- Subject List
- Take a Tour
- For Authors
- Subscriber Services
- Publications
- African American Studies
- African Studies
- American Literature
- Anthropology
- Architecture Planning and Preservation
- Art History
- Atlantic History
- Biblical Studies
- British and Irish Literature
- Childhood Studies
- Chinese Studies
- Cinema and Media Studies
- Communication
- Criminology
- Environmental Science
- Evolutionary Biology
- International Law
- International Relations
- Islamic Studies
- Jewish Studies
- Latin American Studies
- Latino Studies
- Linguistics
- Literary and Critical Theory
- Medieval Studies
- Military History
- Political Science
- Public Health
- Renaissance and Reformation
- Social Work
- Urban Studies
- Victorian Literature
- Browse All Subjects

How to Subscribe
- Free Trials
In This Article Expand or collapse the "in this article" section Problem Solving and Decision Making
Introduction.
- General Approaches to Problem Solving
- Representational Accounts
- Problem Space and Search
- Working Memory and Problem Solving
- Domain-Specific Problem Solving
- The Rational Approach
- Prospect Theory
- Dual-Process Theory
- Cognitive Heuristics and Biases
Related Articles Expand or collapse the "related articles" section about
About related articles close popup.
Lorem Ipsum Sit Dolor Amet
Vestibulum ante ipsum primis in faucibus orci luctus et ultrices posuere cubilia Curae; Aliquam ligula odio, euismod ut aliquam et, vestibulum nec risus. Nulla viverra, arcu et iaculis consequat, justo diam ornare tellus, semper ultrices tellus nunc eu tellus.
- Artificial Intelligence, Machine Learning, and Psychology
- Counterfactual Reasoning
- Critical Thinking
- Heuristics and Biases
- Protocol Analysis
- Psychology and Law
Other Subject Areas
Forthcoming articles expand or collapse the "forthcoming articles" section.
- Data Visualization
- Executive Functions in Childhood
- Remote Work
- Find more forthcoming articles...
- Export Citations
- Share This Facebook LinkedIn Twitter
Problem Solving and Decision Making by Emily G. Nielsen , John Paul Minda LAST REVIEWED: 26 June 2019 LAST MODIFIED: 26 June 2019 DOI: 10.1093/obo/9780199828340-0246
Problem solving and decision making are both examples of complex, higher-order thinking. Both involve the assessment of the environment, the involvement of working memory or short-term memory, reliance on long term memory, effects of knowledge, and the application of heuristics to complete a behavior. A problem can be defined as an impasse or gap between a current state and a desired goal state. Problem solving is the set of cognitive operations that a person engages in to change the current state, to go beyond the impasse, and achieve a desired outcome. Problem solving involves the mental representation of the problem state and the manipulation of this representation in order to move closer to the goal. Problems can vary in complexity, abstraction, and how well defined (or not) the initial state and the goal state are. Research has generally approached problem solving by examining the behaviors and cognitive processes involved, and some work has examined problem solving using computational processes as well. Decision making is the process of selecting and choosing one action or behavior out of several alternatives. Like problem solving, decision making involves the coordination of memories and executive resources. Research on decision making has paid particular attention to the cognitive biases that account for suboptimal decisions and decisions that deviate from rationality. The current bibliography first outlines some general resources on the psychology of problem solving and decision making before examining each of these topics in detail. Specifically, this review covers cognitive, neuroscientific, and computational approaches to problem solving, as well as decision making models and cognitive heuristics and biases.
General Overviews
Current research in the area of problem solving and decision making is published in both general and specialized scientific journals. Theoretical and scholarly work is often summarized and developed in full-length books and chapter. These may focus on the subfields of problem solving and decision making or the larger field of thinking and higher-order cognition.
back to top
Users without a subscription are not able to see the full content on this page. Please subscribe or login .
Oxford Bibliographies Online is available by subscription and perpetual access to institutions. For more information or to contact an Oxford Sales Representative click here .
- About Psychology »
- Meet the Editorial Board »
- Abnormal Psychology
- Academic Assessment
- Acculturation and Health
- Action Regulation Theory
- Action Research
- Addictive Behavior
- Adolescence
- Adoption, Social, Psychological, and Evolutionary Perspect...
- Advanced Theory of Mind
- Affective Forecasting
- Affirmative Action
- Ageism at Work
- Allport, Gordon
- Alzheimer’s Disease
- Ambulatory Assessment in Behavioral Science
- Analysis of Covariance (ANCOVA)
- Animal Behavior
- Animal Learning
- Anxiety Disorders
- Art and Aesthetics, Psychology of
- Assessment and Clinical Applications of Individual Differe...
- Attachment in Social and Emotional Development across the ...
- Attention-Deficit/Hyperactivity Disorder (ADHD) in Adults
- Attention-Deficit/Hyperactivity Disorder (ADHD) in Childre...
- Attitudinal Ambivalence
- Attraction in Close Relationships
- Attribution Theory
- Authoritarian Personality
- Bayesian Statistical Methods in Psychology
- Behavior Therapy, Rational Emotive
- Behavioral Economics
- Behavioral Genetics
- Belief Perseverance
- Bereavement and Grief
- Biological Psychology
- Birth Order
- Body Image in Men and Women
- Bystander Effect
- Categorical Data Analysis in Psychology
- Childhood and Adolescence, Peer Victimization and Bullying...
- Clark, Mamie Phipps
- Clinical Neuropsychology
- Clinical Psychology
- Cognitive Consistency Theories
- Cognitive Dissonance Theory
- Cognitive Neuroscience
- Communication, Nonverbal Cues and
- Comparative Psychology
- Competence to Stand Trial: Restoration Services
- Competency to Stand Trial
- Computational Psychology
- Conflict Management in the Workplace
- Conformity, Compliance, and Obedience
- Consciousness
- Coping Processes
- Correspondence Analysis in Psychology
- Counseling Psychology
- Creativity at Work
- Cross-Cultural Psychology
- Cultural Psychology
- Daily Life, Research Methods for Studying
- Data Science Methods for Psychology
- Data Sharing in Psychology
- Death and Dying
- Deceiving and Detecting Deceit
- Defensive Processes
- Depressive Disorders
- Development, Prenatal
- Developmental Psychology (Cognitive)
- Developmental Psychology (Social)
- Diagnostic and Statistical Manual of Mental Disorders (DSM...
- Discrimination
- Dissociative Disorders
- Drugs and Behavior
- Eating Disorders
- Ecological Psychology
- Educational Settings, Assessment of Thinking in
- Effect Size
- Embodiment and Embodied Cognition
- Emerging Adulthood
- Emotional Intelligence
- Empathy and Altruism
- Employee Stress and Well-Being
- Environmental Neuroscience and Environmental Psychology
- Ethics in Psychological Practice
- Event Perception
- Evolutionary Psychology
- Expansive Posture
- Experimental Existential Psychology
- Exploratory Data Analysis
- Eyewitness Testimony
- Eysenck, Hans
- Factor Analysis
- Festinger, Leon
- Five-Factor Model of Personality
- Flynn Effect, The
- Forensic Psychology
- Forgiveness
- Friendships, Children's
- Fundamental Attribution Error/Correspondence Bias
- Gambler's Fallacy
- Game Theory and Psychology
- Geropsychology, Clinical
- Global Mental Health
- Habit Formation and Behavior Change
- Health Psychology
- Health Psychology Research and Practice, Measurement in
- Heider, Fritz
- History of Psychology
- Human Factors
- Humanistic Psychology
- Implicit Association Test (IAT)
- Industrial and Organizational Psychology
- Inferential Statistics in Psychology
- Insanity Defense, The
- Intelligence
- Intelligence, Crystallized and Fluid
- Intercultural Psychology
- Intergroup Conflict
- International Classification of Diseases and Related Healt...
- International Psychology
- Interviewing in Forensic Settings
- Intimate Partner Violence, Psychological Perspectives on
- Introversion–Extraversion
- Item Response Theory
- Law, Psychology and
- Lazarus, Richard
- Learned Helplessness
- Learning Theory
- Learning versus Performance
- LGBTQ+ Romantic Relationships
- Lie Detection in a Forensic Context
- Life-Span Development
- Locus of Control
- Loneliness and Health
- Mathematical Psychology
- Meaning in Life
- Mechanisms and Processes of Peer Contagion
- Media Violence, Psychological Perspectives on
- Mediation Analysis
- Memories, Autobiographical
- Memories, Flashbulb
- Memories, Repressed and Recovered
- Memory, False
- Memory, Human
- Memory, Implicit versus Explicit
- Memory in Educational Settings
- Memory, Semantic
- Meta-Analysis
- Metacognition
- Metaphor, Psychological Perspectives on
- Microaggressions
- Military Psychology
- Mindfulness
- Mindfulness and Education
- Minnesota Multiphasic Personality Inventory (MMPI)
- Money, Psychology of
- Moral Conviction
- Moral Development
- Moral Psychology
- Moral Reasoning
- Nature versus Nurture Debate in Psychology
- Neuroscience of Associative Learning
- Nonergodicity in Psychology and Neuroscience
- Nonparametric Statistical Analysis in Psychology
- Observational (Non-Randomized) Studies
- Obsessive-Complusive Disorder (OCD)
- Occupational Health Psychology
- Olfaction, Human
- Operant Conditioning
- Optimism and Pessimism
- Organizational Justice
- Parenting Stress
- Parenting Styles
- Parents' Beliefs about Children
- Path Models
- Peace Psychology
- Perception, Person
- Performance Appraisal
- Personality and Health
- Personality Disorders
- Personality Psychology
- Person-Centered and Experiential Psychotherapies: From Car...
- Phenomenological Psychology
- Placebo Effects in Psychology
- Play Behavior
- Positive Psychological Capital (PsyCap)
- Positive Psychology
- Posttraumatic Stress Disorder (PTSD)
- Prejudice and Stereotyping
- Pretrial Publicity
- Prisoner's Dilemma
- Problem Solving and Decision Making
- Procrastination
- Prosocial Behavior
- Prosocial Spending and Well-Being
- Psycholinguistics
- Psychological Literacy
- Psychological Perspectives on Food and Eating
- Psychology, Political
- Psychoneuroimmunology
- Psychophysics, Visual
- Psychotherapy
- Psychotic Disorders
- Publication Bias in Psychology
- Reasoning, Counterfactual
- Rehabilitation Psychology
- Relationships
- Reliability–Contemporary Psychometric Conceptions
- Religion, Psychology and
- Replication Initiatives in Psychology
- Research Methods
- Risk Taking
- Role of the Expert Witness in Forensic Psychology, The
- Sample Size Planning for Statistical Power and Accurate Es...
- Schizophrenic Disorders
- School Psychology
- School Psychology, Counseling Services in
- Self, Gender and
- Self, Psychology of the
- Self-Construal
- Self-Control
- Self-Deception
- Self-Determination Theory
- Self-Efficacy
- Self-Esteem
- Self-Monitoring
- Self-Regulation in Educational Settings
- Self-Report Tests, Measures, and Inventories in Clinical P...
- Sensation Seeking
- Sex and Gender
- Sexual Minority Parenting
- Sexual Orientation
- Signal Detection Theory and its Applications
- Simpson's Paradox in Psychology
- Single People
- Single-Case Experimental Designs
- Skinner, B.F.
- Sleep and Dreaming
- Small Groups
- Social Class and Social Status
- Social Cognition
- Social Neuroscience
- Social Support
- Social Touch and Massage Therapy Research
- Somatoform Disorders
- Spatial Attention
- Sports Psychology
- Stanford Prison Experiment (SPE): Icon and Controversy
- Stereotype Threat
- Stereotypes
- Stress and Coping, Psychology of
- Student Success in College
- Subjective Wellbeing Homeostasis
- Taste, Psychological Perspectives on
- Teaching of Psychology
- Terror Management Theory
- Testing and Assessment
- The Concept of Validity in Psychological Assessment
- The Neuroscience of Emotion Regulation
- The Reasoned Action Approach and the Theories of Reasoned ...
- The Weapon Focus Effect in Eyewitness Memory
- Theory of Mind
- Therapy, Cognitive-Behavioral
- Thinking Skills in Educational Settings
- Time Perception
- Trait Perspective
- Trauma Psychology
- Twin Studies
- Type A Behavior Pattern (Coronary Prone Personality)
- Unconscious Processes
- Video Games and Violent Content
- Virtues and Character Strengths
- Women and Science, Technology, Engineering, and Math (STEM...
- Women, Psychology of
- Work Well-Being
- Workforce Training Evaluation
- Wundt, Wilhelm
- Privacy Policy
- Cookie Policy
- Legal Notice
- Accessibility
Powered by:
- [185.66.15.189]
- 185.66.15.189
7.3 Problem-Solving
Learning objectives.
By the end of this section, you will be able to:
- Describe problem solving strategies
- Define algorithm and heuristic
- Explain some common roadblocks to effective problem solving
People face problems every day—usually, multiple problems throughout the day. Sometimes these problems are straightforward: To double a recipe for pizza dough, for example, all that is required is that each ingredient in the recipe be doubled. Sometimes, however, the problems we encounter are more complex. For example, say you have a work deadline, and you must mail a printed copy of a report to your supervisor by the end of the business day. The report is time-sensitive and must be sent overnight. You finished the report last night, but your printer will not work today. What should you do? First, you need to identify the problem and then apply a strategy for solving the problem.
The study of human and animal problem solving processes has provided much insight toward the understanding of our conscious experience and led to advancements in computer science and artificial intelligence. Essentially much of cognitive science today represents studies of how we consciously and unconsciously make decisions and solve problems. For instance, when encountered with a large amount of information, how do we go about making decisions about the most efficient way of sorting and analyzing all the information in order to find what you are looking for as in visual search paradigms in cognitive psychology. Or in a situation where a piece of machinery is not working properly, how do we go about organizing how to address the issue and understand what the cause of the problem might be. How do we sort the procedures that will be needed and focus attention on what is important in order to solve problems efficiently. Within this section we will discuss some of these issues and examine processes related to human, animal and computer problem solving.
PROBLEM-SOLVING STRATEGIES
When people are presented with a problem—whether it is a complex mathematical problem or a broken printer, how do you solve it? Before finding a solution to the problem, the problem must first be clearly identified. After that, one of many problem solving strategies can be applied, hopefully resulting in a solution.
Problems themselves can be classified into two different categories known as ill-defined and well-defined problems (Schacter, 2009). Ill-defined problems represent issues that do not have clear goals, solution paths, or expected solutions whereas well-defined problems have specific goals, clearly defined solutions, and clear expected solutions. Problem solving often incorporates pragmatics (logical reasoning) and semantics (interpretation of meanings behind the problem), and also in many cases require abstract thinking and creativity in order to find novel solutions. Within psychology, problem solving refers to a motivational drive for reading a definite “goal” from a present situation or condition that is either not moving toward that goal, is distant from it, or requires more complex logical analysis for finding a missing description of conditions or steps toward that goal. Processes relating to problem solving include problem finding also known as problem analysis, problem shaping where the organization of the problem occurs, generating alternative strategies, implementation of attempted solutions, and verification of the selected solution. Various methods of studying problem solving exist within the field of psychology including introspection, behavior analysis and behaviorism, simulation, computer modeling, and experimentation.
A problem-solving strategy is a plan of action used to find a solution. Different strategies have different action plans associated with them (table below). For example, a well-known strategy is trial and error. The old adage, “If at first you don’t succeed, try, try again” describes trial and error. In terms of your broken printer, you could try checking the ink levels, and if that doesn’t work, you could check to make sure the paper tray isn’t jammed. Or maybe the printer isn’t actually connected to your laptop. When using trial and error, you would continue to try different solutions until you solved your problem. Although trial and error is not typically one of the most time-efficient strategies, it is a commonly used one.
Method | Description | Example |
---|---|---|
Trial and error | Continue trying different solutions until problem is solved | Restarting phone, turning off WiFi, turning off bluetooth in order to determine why your phone is malfunctioning |
Algorithm | Step-by-step problem-solving formula | Instruction manual for installing new software on your computer |
Heuristic | General problem-solving framework | Working backwards; breaking a task into steps |
Another type of strategy is an algorithm. An algorithm is a problem-solving formula that provides you with step-by-step instructions used to achieve a desired outcome (Kahneman, 2011). You can think of an algorithm as a recipe with highly detailed instructions that produce the same result every time they are performed. Algorithms are used frequently in our everyday lives, especially in computer science. When you run a search on the Internet, search engines like Google use algorithms to decide which entries will appear first in your list of results. Facebook also uses algorithms to decide which posts to display on your newsfeed. Can you identify other situations in which algorithms are used?
A heuristic is another type of problem solving strategy. While an algorithm must be followed exactly to produce a correct result, a heuristic is a general problem-solving framework (Tversky & Kahneman, 1974). You can think of these as mental shortcuts that are used to solve problems. A “rule of thumb” is an example of a heuristic. Such a rule saves the person time and energy when making a decision, but despite its time-saving characteristics, it is not always the best method for making a rational decision. Different types of heuristics are used in different types of situations, but the impulse to use a heuristic occurs when one of five conditions is met (Pratkanis, 1989):
- When one is faced with too much information
- When the time to make a decision is limited
- When the decision to be made is unimportant
- When there is access to very little information to use in making the decision
- When an appropriate heuristic happens to come to mind in the same moment
Working backwards is a useful heuristic in which you begin solving the problem by focusing on the end result. Consider this example: You live in Washington, D.C. and have been invited to a wedding at 4 PM on Saturday in Philadelphia. Knowing that Interstate 95 tends to back up any day of the week, you need to plan your route and time your departure accordingly. If you want to be at the wedding service by 3:30 PM, and it takes 2.5 hours to get to Philadelphia without traffic, what time should you leave your house? You use the working backwards heuristic to plan the events of your day on a regular basis, probably without even thinking about it.
Another useful heuristic is the practice of accomplishing a large goal or task by breaking it into a series of smaller steps. Students often use this common method to complete a large research project or long essay for school. For example, students typically brainstorm, develop a thesis or main topic, research the chosen topic, organize their information into an outline, write a rough draft, revise and edit the rough draft, develop a final draft, organize the references list, and proofread their work before turning in the project. The large task becomes less overwhelming when it is broken down into a series of small steps.
Further problem solving strategies have been identified (listed below) that incorporate flexible and creative thinking in order to reach solutions efficiently.
Additional Problem Solving Strategies :
- Abstraction – refers to solving the problem within a model of the situation before applying it to reality.
- Analogy – is using a solution that solves a similar problem.
- Brainstorming – refers to collecting an analyzing a large amount of solutions, especially within a group of people, to combine the solutions and developing them until an optimal solution is reached.
- Divide and conquer – breaking down large complex problems into smaller more manageable problems.
- Hypothesis testing – method used in experimentation where an assumption about what would happen in response to manipulating an independent variable is made, and analysis of the affects of the manipulation are made and compared to the original hypothesis.
- Lateral thinking – approaching problems indirectly and creatively by viewing the problem in a new and unusual light.
- Means-ends analysis – choosing and analyzing an action at a series of smaller steps to move closer to the goal.
- Method of focal objects – putting seemingly non-matching characteristics of different procedures together to make something new that will get you closer to the goal.
- Morphological analysis – analyzing the outputs of and interactions of many pieces that together make up a whole system.
- Proof – trying to prove that a problem cannot be solved. Where the proof fails becomes the starting point or solving the problem.
- Reduction – adapting the problem to be as similar problems where a solution exists.
- Research – using existing knowledge or solutions to similar problems to solve the problem.
- Root cause analysis – trying to identify the cause of the problem.
The strategies listed above outline a short summary of methods we use in working toward solutions and also demonstrate how the mind works when being faced with barriers preventing goals to be reached.
One example of means-end analysis can be found by using the Tower of Hanoi paradigm . This paradigm can be modeled as a word problems as demonstrated by the Missionary-Cannibal Problem :
Missionary-Cannibal Problem
Three missionaries and three cannibals are on one side of a river and need to cross to the other side. The only means of crossing is a boat, and the boat can only hold two people at a time. Your goal is to devise a set of moves that will transport all six of the people across the river, being in mind the following constraint: The number of cannibals can never exceed the number of missionaries in any location. Remember that someone will have to also row that boat back across each time.
Hint : At one point in your solution, you will have to send more people back to the original side than you just sent to the destination.
The actual Tower of Hanoi problem consists of three rods sitting vertically on a base with a number of disks of different sizes that can slide onto any rod. The puzzle starts with the disks in a neat stack in ascending order of size on one rod, the smallest at the top making a conical shape. The objective of the puzzle is to move the entire stack to another rod obeying the following rules:
- 1. Only one disk can be moved at a time.
- 2. Each move consists of taking the upper disk from one of the stacks and placing it on top of another stack or on an empty rod.
- 3. No disc may be placed on top of a smaller disk.
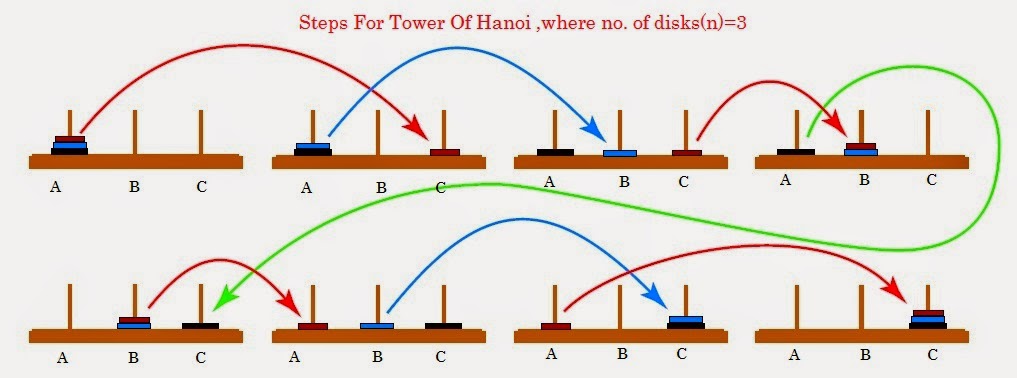
Figure 7.02. Steps for solving the Tower of Hanoi in the minimum number of moves when there are 3 disks.
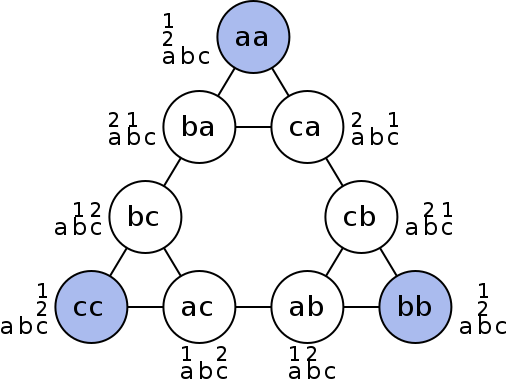
Figure 7.03. Graphical representation of nodes (circles) and moves (lines) of Tower of Hanoi.
The Tower of Hanoi is a frequently used psychological technique to study problem solving and procedure analysis. A variation of the Tower of Hanoi known as the Tower of London has been developed which has been an important tool in the neuropsychological diagnosis of executive function disorders and their treatment.
GESTALT PSYCHOLOGY AND PROBLEM SOLVING
As you may recall from the sensation and perception chapter, Gestalt psychology describes whole patterns, forms and configurations of perception and cognition such as closure, good continuation, and figure-ground. In addition to patterns of perception, Wolfgang Kohler, a German Gestalt psychologist traveled to the Spanish island of Tenerife in order to study animals behavior and problem solving in the anthropoid ape.
As an interesting side note to Kohler’s studies of chimp problem solving, Dr. Ronald Ley, professor of psychology at State University of New York provides evidence in his book A Whisper of Espionage (1990) suggesting that while collecting data for what would later be his book The Mentality of Apes (1925) on Tenerife in the Canary Islands between 1914 and 1920, Kohler was additionally an active spy for the German government alerting Germany to ships that were sailing around the Canary Islands. Ley suggests his investigations in England, Germany and elsewhere in Europe confirm that Kohler had served in the German military by building, maintaining and operating a concealed radio that contributed to Germany’s war effort acting as a strategic outpost in the Canary Islands that could monitor naval military activity approaching the north African coast.
While trapped on the island over the course of World War 1, Kohler applied Gestalt principles to animal perception in order to understand how they solve problems. He recognized that the apes on the islands also perceive relations between stimuli and the environment in Gestalt patterns and understand these patterns as wholes as opposed to pieces that make up a whole. Kohler based his theories of animal intelligence on the ability to understand relations between stimuli, and spent much of his time while trapped on the island investigation what he described as insight , the sudden perception of useful or proper relations. In order to study insight in animals, Kohler would present problems to chimpanzee’s by hanging some banana’s or some kind of food so it was suspended higher than the apes could reach. Within the room, Kohler would arrange a variety of boxes, sticks or other tools the chimpanzees could use by combining in patterns or organizing in a way that would allow them to obtain the food (Kohler & Winter, 1925).
While viewing the chimpanzee’s, Kohler noticed one chimp that was more efficient at solving problems than some of the others. The chimp, named Sultan, was able to use long poles to reach through bars and organize objects in specific patterns to obtain food or other desirables that were originally out of reach. In order to study insight within these chimps, Kohler would remove objects from the room to systematically make the food more difficult to obtain. As the story goes, after removing many of the objects Sultan was used to using to obtain the food, he sat down ad sulked for a while, and then suddenly got up going over to two poles lying on the ground. Without hesitation Sultan put one pole inside the end of the other creating a longer pole that he could use to obtain the food demonstrating an ideal example of what Kohler described as insight. In another situation, Sultan discovered how to stand on a box to reach a banana that was suspended from the rafters illustrating Sultan’s perception of relations and the importance of insight in problem solving.
Grande (another chimp in the group studied by Kohler) builds a three-box structure to reach the bananas, while Sultan watches from the ground. Insight , sometimes referred to as an “Ah-ha” experience, was the term Kohler used for the sudden perception of useful relations among objects during problem solving (Kohler, 1927; Radvansky & Ashcraft, 2013).
Solving puzzles.
Problem-solving abilities can improve with practice. Many people challenge themselves every day with puzzles and other mental exercises to sharpen their problem-solving skills. Sudoku puzzles appear daily in most newspapers. Typically, a sudoku puzzle is a 9×9 grid. The simple sudoku below (see figure) is a 4×4 grid. To solve the puzzle, fill in the empty boxes with a single digit: 1, 2, 3, or 4. Here are the rules: The numbers must total 10 in each bolded box, each row, and each column; however, each digit can only appear once in a bolded box, row, and column. Time yourself as you solve this puzzle and compare your time with a classmate.
How long did it take you to solve this sudoku puzzle? (You can see the answer at the end of this section.)
Here is another popular type of puzzle (figure below) that challenges your spatial reasoning skills. Connect all nine dots with four connecting straight lines without lifting your pencil from the paper:
Did you figure it out? (The answer is at the end of this section.) Once you understand how to crack this puzzle, you won’t forget.
Take a look at the “Puzzling Scales” logic puzzle below (figure below). Sam Loyd, a well-known puzzle master, created and refined countless puzzles throughout his lifetime (Cyclopedia of Puzzles, n.d.).

What steps did you take to solve this puzzle? You can read the solution at the end of this section.
Pitfalls to problem solving.
Not all problems are successfully solved, however. What challenges stop us from successfully solving a problem? Albert Einstein once said, “Insanity is doing the same thing over and over again and expecting a different result.” Imagine a person in a room that has four doorways. One doorway that has always been open in the past is now locked. The person, accustomed to exiting the room by that particular doorway, keeps trying to get out through the same doorway even though the other three doorways are open. The person is stuck—but she just needs to go to another doorway, instead of trying to get out through the locked doorway. A mental set is where you persist in approaching a problem in a way that has worked in the past but is clearly not working now.
Functional fixedness is a type of mental set where you cannot perceive an object being used for something other than what it was designed for. During the Apollo 13 mission to the moon, NASA engineers at Mission Control had to overcome functional fixedness to save the lives of the astronauts aboard the spacecraft. An explosion in a module of the spacecraft damaged multiple systems. The astronauts were in danger of being poisoned by rising levels of carbon dioxide because of problems with the carbon dioxide filters. The engineers found a way for the astronauts to use spare plastic bags, tape, and air hoses to create a makeshift air filter, which saved the lives of the astronauts.
Researchers have investigated whether functional fixedness is affected by culture. In one experiment, individuals from the Shuar group in Ecuador were asked to use an object for a purpose other than that for which the object was originally intended. For example, the participants were told a story about a bear and a rabbit that were separated by a river and asked to select among various objects, including a spoon, a cup, erasers, and so on, to help the animals. The spoon was the only object long enough to span the imaginary river, but if the spoon was presented in a way that reflected its normal usage, it took participants longer to choose the spoon to solve the problem. (German & Barrett, 2005). The researchers wanted to know if exposure to highly specialized tools, as occurs with individuals in industrialized nations, affects their ability to transcend functional fixedness. It was determined that functional fixedness is experienced in both industrialized and nonindustrialized cultures (German & Barrett, 2005).
In order to make good decisions, we use our knowledge and our reasoning. Often, this knowledge and reasoning is sound and solid. Sometimes, however, we are swayed by biases or by others manipulating a situation. For example, let’s say you and three friends wanted to rent a house and had a combined target budget of $1,600. The realtor shows you only very run-down houses for $1,600 and then shows you a very nice house for $2,000. Might you ask each person to pay more in rent to get the $2,000 home? Why would the realtor show you the run-down houses and the nice house? The realtor may be challenging your anchoring bias. An anchoring bias occurs when you focus on one piece of information when making a decision or solving a problem. In this case, you’re so focused on the amount of money you are willing to spend that you may not recognize what kinds of houses are available at that price point.
The confirmation bias is the tendency to focus on information that confirms your existing beliefs. For example, if you think that your professor is not very nice, you notice all of the instances of rude behavior exhibited by the professor while ignoring the countless pleasant interactions he is involved in on a daily basis. Hindsight bias leads you to believe that the event you just experienced was predictable, even though it really wasn’t. In other words, you knew all along that things would turn out the way they did. Representative bias describes a faulty way of thinking, in which you unintentionally stereotype someone or something; for example, you may assume that your professors spend their free time reading books and engaging in intellectual conversation, because the idea of them spending their time playing volleyball or visiting an amusement park does not fit in with your stereotypes of professors.
Finally, the availability heuristic is a heuristic in which you make a decision based on an example, information, or recent experience that is that readily available to you, even though it may not be the best example to inform your decision . Biases tend to “preserve that which is already established—to maintain our preexisting knowledge, beliefs, attitudes, and hypotheses” (Aronson, 1995; Kahneman, 2011). These biases are summarized in the table below.
Bias | Description |
---|---|
Anchoring | Tendency to focus on one particular piece of information when making decisions or problem-solving |
Confirmation | Focuses on information that confirms existing beliefs |
Hindsight | Belief that the event just experienced was predictable |
Representative | Unintentional stereotyping of someone or something |
Availability | Decision is based upon either an available precedent or an example that may be faulty |
Were you able to determine how many marbles are needed to balance the scales in the figure below? You need nine. Were you able to solve the problems in the figures above? Here are the answers.

Many different strategies exist for solving problems. Typical strategies include trial and error, applying algorithms, and using heuristics. To solve a large, complicated problem, it often helps to break the problem into smaller steps that can be accomplished individually, leading to an overall solution. Roadblocks to problem solving include a mental set, functional fixedness, and various biases that can cloud decision making skills.
References:
Openstax Psychology text by Kathryn Dumper, William Jenkins, Arlene Lacombe, Marilyn Lovett and Marion Perlmutter licensed under CC BY v4.0. https://openstax.org/details/books/psychology
Review Questions:
1. A specific formula for solving a problem is called ________.
a. an algorithm
b. a heuristic
c. a mental set
d. trial and error
2. Solving the Tower of Hanoi problem tends to utilize a ________ strategy of problem solving.
a. divide and conquer
b. means-end analysis
d. experiment
3. A mental shortcut in the form of a general problem-solving framework is called ________.
4. Which type of bias involves becoming fixated on a single trait of a problem?
a. anchoring bias
b. confirmation bias
c. representative bias
d. availability bias
5. Which type of bias involves relying on a false stereotype to make a decision?
6. Wolfgang Kohler analyzed behavior of chimpanzees by applying Gestalt principles to describe ________.
a. social adjustment
b. student load payment options
c. emotional learning
d. insight learning
7. ________ is a type of mental set where you cannot perceive an object being used for something other than what it was designed for.
a. functional fixedness
c. working memory
Critical Thinking Questions:
1. What is functional fixedness and how can overcoming it help you solve problems?
2. How does an algorithm save you time and energy when solving a problem?
Personal Application Question:
1. Which type of bias do you recognize in your own decision making processes? How has this bias affected how you’ve made decisions in the past and how can you use your awareness of it to improve your decisions making skills in the future?
anchoring bias
availability heuristic
confirmation bias
functional fixedness
hindsight bias
problem-solving strategy
representative bias
trial and error
working backwards
Answers to Exercises
algorithm: problem-solving strategy characterized by a specific set of instructions
anchoring bias: faulty heuristic in which you fixate on a single aspect of a problem to find a solution
availability heuristic: faulty heuristic in which you make a decision based on information readily available to you
confirmation bias: faulty heuristic in which you focus on information that confirms your beliefs
functional fixedness: inability to see an object as useful for any other use other than the one for which it was intended
heuristic: mental shortcut that saves time when solving a problem
hindsight bias: belief that the event just experienced was predictable, even though it really wasn’t
mental set: continually using an old solution to a problem without results
problem-solving strategy: method for solving problems
representative bias: faulty heuristic in which you stereotype someone or something without a valid basis for your judgment
trial and error: problem-solving strategy in which multiple solutions are attempted until the correct one is found
working backwards: heuristic in which you begin to solve a problem by focusing on the end result

Share This Book
- Increase Font Size
- Bipolar Disorder
- Therapy Center
- When To See a Therapist
- Types of Therapy
- Best Online Therapy
- Best Couples Therapy
- Managing Stress
- Sleep and Dreaming
- Understanding Emotions
- Self-Improvement
- Healthy Relationships
- Student Resources
- Personality Types
- Sweepstakes
- Guided Meditations
- Verywell Mind Insights
- 2024 Verywell Mind 25
- Mental Health in the Classroom
- Editorial Process
- Meet Our Review Board
- Crisis Support
Problem-Solving Strategies and Obstacles
JGI / Jamie Grill / Getty Images
- Application
- Improvement
From deciding what to eat for dinner to considering whether it's the right time to buy a house, problem-solving is a large part of our daily lives. Learn some of the problem-solving strategies that exist and how to use them in real life, along with ways to overcome obstacles that are making it harder to resolve the issues you face.
What Is Problem-Solving?
In cognitive psychology , the term 'problem-solving' refers to the mental process that people go through to discover, analyze, and solve problems.
A problem exists when there is a goal that we want to achieve but the process by which we will achieve it is not obvious to us. Put another way, there is something that we want to occur in our life, yet we are not immediately certain how to make it happen.
Maybe you want a better relationship with your spouse or another family member but you're not sure how to improve it. Or you want to start a business but are unsure what steps to take. Problem-solving helps you figure out how to achieve these desires.
The problem-solving process involves:
- Discovery of the problem
- Deciding to tackle the issue
- Seeking to understand the problem more fully
- Researching available options or solutions
- Taking action to resolve the issue
Before problem-solving can occur, it is important to first understand the exact nature of the problem itself. If your understanding of the issue is faulty, your attempts to resolve it will also be incorrect or flawed.
Problem-Solving Mental Processes
Several mental processes are at work during problem-solving. Among them are:
- Perceptually recognizing the problem
- Representing the problem in memory
- Considering relevant information that applies to the problem
- Identifying different aspects of the problem
- Labeling and describing the problem
Problem-Solving Strategies
There are many ways to go about solving a problem. Some of these strategies might be used on their own, or you may decide to employ multiple approaches when working to figure out and fix a problem.
An algorithm is a step-by-step procedure that, by following certain "rules" produces a solution. Algorithms are commonly used in mathematics to solve division or multiplication problems. But they can be used in other fields as well.
In psychology, algorithms can be used to help identify individuals with a greater risk of mental health issues. For instance, research suggests that certain algorithms might help us recognize children with an elevated risk of suicide or self-harm.
One benefit of algorithms is that they guarantee an accurate answer. However, they aren't always the best approach to problem-solving, in part because detecting patterns can be incredibly time-consuming.
There are also concerns when machine learning is involved—also known as artificial intelligence (AI)—such as whether they can accurately predict human behaviors.
Heuristics are shortcut strategies that people can use to solve a problem at hand. These "rule of thumb" approaches allow you to simplify complex problems, reducing the total number of possible solutions to a more manageable set.
If you find yourself sitting in a traffic jam, for example, you may quickly consider other routes, taking one to get moving once again. When shopping for a new car, you might think back to a prior experience when negotiating got you a lower price, then employ the same tactics.
While heuristics may be helpful when facing smaller issues, major decisions shouldn't necessarily be made using a shortcut approach. Heuristics also don't guarantee an effective solution, such as when trying to drive around a traffic jam only to find yourself on an equally crowded route.
Trial and Error
A trial-and-error approach to problem-solving involves trying a number of potential solutions to a particular issue, then ruling out those that do not work. If you're not sure whether to buy a shirt in blue or green, for instance, you may try on each before deciding which one to purchase.
This can be a good strategy to use if you have a limited number of solutions available. But if there are many different choices available, narrowing down the possible options using another problem-solving technique can be helpful before attempting trial and error.
In some cases, the solution to a problem can appear as a sudden insight. You are facing an issue in a relationship or your career when, out of nowhere, the solution appears in your mind and you know exactly what to do.
Insight can occur when the problem in front of you is similar to an issue that you've dealt with in the past. Although, you may not recognize what is occurring since the underlying mental processes that lead to insight often happen outside of conscious awareness .
Research indicates that insight is most likely to occur during times when you are alone—such as when going on a walk by yourself, when you're in the shower, or when lying in bed after waking up.
How to Apply Problem-Solving Strategies in Real Life
If you're facing a problem, you can implement one or more of these strategies to find a potential solution. Here's how to use them in real life:
- Create a flow chart . If you have time, you can take advantage of the algorithm approach to problem-solving by sitting down and making a flow chart of each potential solution, its consequences, and what happens next.
- Recall your past experiences . When a problem needs to be solved fairly quickly, heuristics may be a better approach. Think back to when you faced a similar issue, then use your knowledge and experience to choose the best option possible.
- Start trying potential solutions . If your options are limited, start trying them one by one to see which solution is best for achieving your desired goal. If a particular solution doesn't work, move on to the next.
- Take some time alone . Since insight is often achieved when you're alone, carve out time to be by yourself for a while. The answer to your problem may come to you, seemingly out of the blue, if you spend some time away from others.
Obstacles to Problem-Solving
Problem-solving is not a flawless process as there are a number of obstacles that can interfere with our ability to solve a problem quickly and efficiently. These obstacles include:
- Assumptions: When dealing with a problem, people can make assumptions about the constraints and obstacles that prevent certain solutions. Thus, they may not even try some potential options.
- Functional fixedness : This term refers to the tendency to view problems only in their customary manner. Functional fixedness prevents people from fully seeing all of the different options that might be available to find a solution.
- Irrelevant or misleading information: When trying to solve a problem, it's important to distinguish between information that is relevant to the issue and irrelevant data that can lead to faulty solutions. The more complex the problem, the easier it is to focus on misleading or irrelevant information.
- Mental set: A mental set is a tendency to only use solutions that have worked in the past rather than looking for alternative ideas. A mental set can work as a heuristic, making it a useful problem-solving tool. However, mental sets can also lead to inflexibility, making it more difficult to find effective solutions.
How to Improve Your Problem-Solving Skills
In the end, if your goal is to become a better problem-solver, it's helpful to remember that this is a process. Thus, if you want to improve your problem-solving skills, following these steps can help lead you to your solution:
- Recognize that a problem exists . If you are facing a problem, there are generally signs. For instance, if you have a mental illness , you may experience excessive fear or sadness, mood changes, and changes in sleeping or eating habits. Recognizing these signs can help you realize that an issue exists.
- Decide to solve the problem . Make a conscious decision to solve the issue at hand. Commit to yourself that you will go through the steps necessary to find a solution.
- Seek to fully understand the issue . Analyze the problem you face, looking at it from all sides. If your problem is relationship-related, for instance, ask yourself how the other person may be interpreting the issue. You might also consider how your actions might be contributing to the situation.
- Research potential options . Using the problem-solving strategies mentioned, research potential solutions. Make a list of options, then consider each one individually. What are some pros and cons of taking the available routes? What would you need to do to make them happen?
- Take action . Select the best solution possible and take action. Action is one of the steps required for change . So, go through the motions needed to resolve the issue.
- Try another option, if needed . If the solution you chose didn't work, don't give up. Either go through the problem-solving process again or simply try another option.
You can find a way to solve your problems as long as you keep working toward this goal—even if the best solution is simply to let go because no other good solution exists.
Sarathy V. Real world problem-solving . Front Hum Neurosci . 2018;12:261. doi:10.3389/fnhum.2018.00261
Dunbar K. Problem solving . A Companion to Cognitive Science . 2017. doi:10.1002/9781405164535.ch20
Stewart SL, Celebre A, Hirdes JP, Poss JW. Risk of suicide and self-harm in kids: The development of an algorithm to identify high-risk individuals within the children's mental health system . Child Psychiat Human Develop . 2020;51:913-924. doi:10.1007/s10578-020-00968-9
Rosenbusch H, Soldner F, Evans AM, Zeelenberg M. Supervised machine learning methods in psychology: A practical introduction with annotated R code . Soc Personal Psychol Compass . 2021;15(2):e12579. doi:10.1111/spc3.12579
Mishra S. Decision-making under risk: Integrating perspectives from biology, economics, and psychology . Personal Soc Psychol Rev . 2014;18(3):280-307. doi:10.1177/1088868314530517
Csikszentmihalyi M, Sawyer K. Creative insight: The social dimension of a solitary moment . In: The Systems Model of Creativity . 2015:73-98. doi:10.1007/978-94-017-9085-7_7
Chrysikou EG, Motyka K, Nigro C, Yang SI, Thompson-Schill SL. Functional fixedness in creative thinking tasks depends on stimulus modality . Psychol Aesthet Creat Arts . 2016;10(4):425‐435. doi:10.1037/aca0000050
Huang F, Tang S, Hu Z. Unconditional perseveration of the short-term mental set in chunk decomposition . Front Psychol . 2018;9:2568. doi:10.3389/fpsyg.2018.02568
National Alliance on Mental Illness. Warning signs and symptoms .
Mayer RE. Thinking, problem solving, cognition, 2nd ed .
Schooler JW, Ohlsson S, Brooks K. Thoughts beyond words: When language overshadows insight. J Experiment Psychol: General . 1993;122:166-183. doi:10.1037/0096-3445.2.166
By Kendra Cherry, MSEd Kendra Cherry, MS, is a psychosocial rehabilitation specialist, psychology educator, and author of the "Everything Psychology Book."
InstructionalDesign.org
Home » Learning Domains » Problem-Solving
Problem-Solving
Theories of problem-solving are dominated by the work of Newell & Simon on GPS (General Problem Solver) . This work established the information processing paradigm for the study of problem-solving and the concepts of “means-ends-analysis” and “problem space”. According to the GPS framework, problem-solving involves the identification of subgoals and the use of methods (especially heuristics) to satisfy the subgoals.
The Gestalt psychologist Wertheimer also conducted research on problem-solving and emphasized the importance of understanding the structure (i.e., the relationship among parts) of the problem. In his lateral thinking theory, DeBono stressed the importance of looking at a problem with a fresh perspective.
Schoenfeld presents a theory of problem-solving in mathematics that involves four aspects: resources, heuristics, control, and beliefs. Although this framework was specifically developed for mathematical problem-solving, it seems more generally applicable. Bransford et al. present a problem-solving approach to the use of hypermedia in their anchored instructional theory.
Problem-solving skills appear to be related to many other aspects of cognition (Frederiksen, 1984) such as schema (the ability to remember similar problems), pattern recognition (recognizing familiar problem elements) and creativity (developing new solutions). The issue of transfer is highly relevant to problem solving. A good summary of problem-solving research as it applies to instruction is provided by Tuma & Rief (1980). Problem-solving skills are fundamental to many professional domains such as engineering or medicine .
- Frederiksen, N. (1984). Implications of cognitive theory for instruction in problem solving. Review of Educational Research, 54 (3), 363-407.
- Tuma, D. & Reif, F. (1980). Problem Solving and Education. Hillsdale, NJ: Erlbaum.
Problem-Solving Theory: The Task-Centred Model
- Living reference work entry
- First Online: 12 April 2022
- Cite this living reference work entry
- Blanca M. Ramos 5 &
- Randall L. Stetson 6
Part of the book series: Social Work ((SOWO))
605 Accesses
This chapter examines the task-centred model to illustrate the application of problem-solving theory for social work intervention. First, it provides a brief description of the problem-solving model. Its historical development and key principles and concepts are presented. Next, the chapter offers a general overview of the crisis intervention model. The task-centred model and crisis intervention share principles and methods drawn from problem-solving theory. The remainder of the chapter focuses on the task-centred model. It reviews its historical background, viability as a framework for social work generalist practice, as well as its applicability with diverse client populations and across cultural settings. The structured steps that guide task-centred implementation throughout the helping process are described. A brief critical review of the model’s strengths and limitations is provided. The chapter concludes with a brief summary and some closing thoughts.
This is a preview of subscription content, log in via an institution to check access.
Access this chapter
Institutional subscriptions
Similar content being viewed by others
Conceptual framework for task shifting and task sharing: an international Delphi study
Action in Counselling: A Contextual Action Theory Perspective
Brieland D (1977) Historical overview. Soc Work 22(5):341–346. http://www.jstor.org.libezproxy2.syr.edu/stable/23712810
Google Scholar
Coady N, Lehmann P (2016) The problem-solving model: a framework for integrating the science and art of practice. In: Lehmann P, Coady N (eds) Theoretical perspectives for direct social work practice: a generalist-eclectic approach, 3rd edn. Springer
Chapter Google Scholar
D’Zurilla TJ, Goldfried MR (1971) Problem solving and behavior modification. J Abnorm Psychol 78(1):107–126. https://doi.org/10.1037/h0031360
Article Google Scholar
Dattilio F (1998) Cognitive behavioral therapy. In: Dattilio M (ed) Case studies in couple and family therapy: systems and cognitive perspectives. Guilford, New York, pp 62–82
Dohert W (1981) Cognitive processes in intimate conflicts: extending attribution theory. Am J Fam Ther 9:3–12
Duckword G (1967) A project in crisis intervention. Soc Casework 48(4):227–231
Fortune AE (2012) Development of the task-centered model. In: Rzepnicki TL, McCracken SG, Briggs HE (eds) From task-centered social work to evidence-based and integrative practice: reflections on history and implementation. Oxford University Press, pp 15–39
Fortune AE, Reid WJ (2011) Task-centered social work. In: Turner F (ed) Social work treatment: interlocking theoretical approaches, 6th edn. Oxford University Press, New York, pp 513–532
Fortune AE, McCallion P, Briar-Lawson K (Eds.) (2010) Social work practice research for the 21st century. New York: Columbia University Press
Fortune AE, Ramos BM, Reid WJ (2022) Task-Centered practice. In: Lisa Rapp-McCall, Kevin Corcoran & Albert R. Roberts, (eds.), Social workers’ desk reference, 4th edn Oxford University Press, New York
Fortune AE, Ramos BM, Reid WJ (2022) Task-Centered Practice. In: Lisa Rapp-McCall, Kevin Corcoran, Albert R Roberts, (Eds.). Social Workers’ Desk Reference, 4th edition. New York: Oxford University Press
Garfield SL (1994) Research on client variables in psychotherapy. In: Bergin A, Garfield S (eds) Handbook of psychotherapy and behavior change, 4th edn. Wiley, New York, pp 190–228
Golan N, Carey H, Hyttinnen E (1969) The emerging role of the social worker in the psychiatric emergency service. Community Ment Health J 5(1):55–61
Gorey KM, Thyer BA, Pawfuck DE (1998) Differential effectiveness of prevalent social work practice models: a meta-analysis. Soc Work 43:269–278
Hollis F (1970) The psychosocial approach to the practice of casework. In: Theories of social casework. University of Chicago Press, pp 33–75
Hoyt MF (2000) Some stories are better than others: doing what works in brief therapy and managed care. Brunner/Mazel, Philadelphia
Hubble M, Duncan B, Miller S (1999) Introduction. In: Hubble M, Duncan B, Miller S (eds) The heart and soul of change: what works in therapy. American Psychological Association, Washington, DC
Huh NS, Koh YS (2010) Task-centered practice in South Korea. In: Fortune AE, McCallion P, Briar-Lawson K (eds) Social work practice research for the 21st century. Columbia University Press, New York, pp 235–239
Jagt N, Jagt L (2010) Task-centered practice in the Netherlands. In: Fortune AE, McCallion P, Briar-Lawson K (eds) Social work practice research for the 21st century. Columbia University Press, New York, pp 208–212
Lo TW (2010) Task-centered practice in Hong Kong. In: Fortune AE, McCallion P, Briar-Lawson K (eds) Social work practice research for the 21st century. Columbia University Press, New York, pp 240–244
Malouff JM, Thorsteinsson EB, Schutte NS (2007) The efficacy of problem-solving therapy in reducing mental and physical health problems: a meta-analysis. Clin Psychol Rev 27(1):46–57
Marsh P (2010) Task-centered practice in Great Britain. In: Fortune AE, McCallion P, Briar-Lawson K (eds) Social work practice research for the 21st century. Columbia University Press, New York, pp 203–2007
Marsh P, Doel M (2005) The task-centred book. Routledge, Abingdon/New York
Book Google Scholar
Miley K, O’Melia M, DuBois (2017) Generalist social work practice: an empowering approach. Allyn & Bacon, Boston
Morris B (1968) Crisis intervention in a public welfare agency. Soc Casework 49(10):612–617
Naleppa M (2010) Task-centered practice in Germany. In: Fortune AE, McCallion P, Briar-Lawson K (eds) Social work practice research for the 21st century. Columbia University Press, New York, pp 213–216
Nezu AM, Nezu CM, D’Zurilla T (2012) Problem-solving therapy: a treatment manual. Springer
Nichols M, Schwartz R (2001) Family therapy. Allyn and Bacon, Needham Heights
Parad HJ (1958) Ego psychology and dynamic casework. Family Association of America, New York
Parad H (1965) Preventive casework: problems and implications. In: Parad H (ed) Crisis intervention: selected readings. Family Service Association of America, New York
Parad H (1966) The use of time-limited crisis interventions in community mental health programming. Soc Serv Rev 40(3):275–282
Parad H, Capland G (1960) A framework for studying families in crisis. Soc Work 5(3):3–15
Parad H, Parad G (1968) A study of crisis oriented planned short-term treatment. Soc Casework 49(6):346–355
Payne M (2014) Modern social work theory, 3rd edn. Palgrave Macmillan, Basingstoke
Perlman HH (1957) Social casework: a problem-solving process. University of Chicago Press, Chicago
Poal P (1990) Introduction to the theory and practice of crisis intervention. Quadernos Psicol 10:121–140
Ramos BM, Garvin C (2003) Task centered treatment with culturally diverse populations. In: Tolson E, Reid W, Garvin C (eds) Generalist practice: a task centered approach, pp. Columbia University Press, New York, pp 441–463
Ramos B, Tolson E (2016) The task-centered model. In: Lehmann P, Coady N (eds) Theoretical perspectives for direct social work practice: a generalist-eclectic approach, 3rd edn. Springer
Regehr C (2017) Crisis theory and social work treatment. In: Turner F (ed) Social work treatment: interlocking theoretical approaches. Oxford University Press
Reid WJ (1992) Task strategies: an empirical approach to social work practice. Columbia University Press, New York
Reid WJ, Epstein L (eds) (1972) Task-centered casework. Columbia University Press, New York
Reid W, Ramos B (2002) Intervención “Centrada en la Tarea”, un Modelo de Práctica de Trabajo Social. Rev Treball Soc 168:6–22
Reid WJ, Shyne AW (1969) Brief and extended casework. Columbia University Press, New York
Roberts A (2005) Bridging the past and present to the future of crisis intervention and case management. In: Roberts A (ed) Crisis intervention handbook: assessment, treatment, and research, 3rd edn. Oxford University Press
Rooney RH (2010) Task-centered practice in the United States. In: Fortune AE, McCallion P, Briar-Lawson K (eds) Social work practice research for the 21st century. Columbia University Press, New York, pp 195–202
Ruben D (1998) Social exchange theory: dynamics of a system governing the dysfunctional family and guide to assessment. J Contemp Psychother 8(3):307–325
Schatz MS, Jenkins LE, Sheafor BW (1990) Milford redefined: a model of initial and advanced generalist social work [Article]. J Soc Work Educ 26(3):217–231. https://doi.org/10.1080/10437797.1990.10672154
Strean HS (1968) Some reactions of case workers to the war on poverty. J Contemp Psychother 1:43–48
Strickler M (1965) Applying crisis theory in a community clinic. Soc Casework 46:150–154
Studt E (1968) Social work theory and implication for the practice of methods. Soc Work Educ Report 16:22–46
Tolson R, Reid W, Garvin C (2003) Generalist practice: a task-centered approach, 2nd edn. Columbia University Press, New York
Trotter C (2010) Task-centred practice in Australia. In Fortune AE, McCallion P, Briar-Lawson K (Eds.), Social work practice research for the 21st century, 235–239. New York: Columbia University Press
Watzlawick P, Bervin J, Jackson D (1967) Pragmatics of human communication. W.W. Norton, New York
Download references
Author information
Authors and affiliations.
State University of New York at Albany, Albany, NY, USA
Blanca M. Ramos
State University of New York at Oswego, Oswego, NY, USA
Randall L. Stetson
You can also search for this author in PubMed Google Scholar
Corresponding author
Correspondence to Blanca M. Ramos .
Editor information
Editors and affiliations.
School of Human Services and Social Work, Griffith University, Meadowbrook, QLD, Australia
Dorothee Hölscher
School of Social Sciences, UNSW Sydney, Sydney, NSW, Australia
Richard Hugman
Donna McAuliffe
Rights and permissions
Reprints and permissions

Copyright information
© 2022 Springer Nature Singapore Pte Ltd.
About this entry
Cite this entry.
Ramos, B.M., Stetson, R.L. (2022). Problem-Solving Theory: The Task-Centred Model. In: Hölscher, D., Hugman, R., McAuliffe, D. (eds) Social Work Theory and Ethics. Social Work. Springer, Singapore. https://doi.org/10.1007/978-981-16-3059-0_9-1
Download citation
DOI : https://doi.org/10.1007/978-981-16-3059-0_9-1
Received : 24 December 2021
Accepted : 25 January 2022
Published : 12 April 2022
Publisher Name : Springer, Singapore
Print ISBN : 978-981-16-3059-0
Online ISBN : 978-981-16-3059-0
eBook Packages : Social Sciences Reference Module Humanities and Social Sciences Reference Module Business, Economics and Social Sciences
- Publish with us
Policies and ethics
- Find a journal
- Track your research
*BRAND NEW* Self-paced Introduction to Counting & Probability. Enroll today!
Something appears to not have loaded correctly.
Click to refresh .

- Liberty University
- Jerry Falwell Library
- Special Collections
- < Previous
Home > ETD > Doctoral > 5880
Doctoral Dissertations and Projects
Examining the lived experiences of educators using different levels of support for teaching math to students with learning disabilities in math computation and problem-solving for teachers at public cyber charter high schools in the northeastern united states: a transcendental phenomenological study.
LeeAnn E. McCullough , Liberty University Follow
School of Education
Doctor of Philosophy in Education (PhD)
Mary Catherine Strickland
cognitive load theory, specific learning disability, math computation, math problem-solving, Sweller, learning theory
Disciplines
Mathematics | Special Education and Teaching
Recommended Citation
McCullough, LeeAnn E., "Examining the Lived Experiences of Educators Using Different Levels of Support for Teaching Math to Students with Learning Disabilities in Math Computation and Problem-Solving for Teachers at Public Cyber Charter High Schools in the Northeastern United States: A Transcendental Phenomenological Study" (2024). Doctoral Dissertations and Projects . 5880. https://digitalcommons.liberty.edu/doctoral/5880
The purpose of this transcendental phenomenological study was to describe the lived experiences of educators using different levels of support for teaching math to students with learning disabilities in math computation and problem-solving for teachers at public cyber charter high schools in the Northeastern United States. The theory guiding this study was Sweller’s cognitive load theory, as it explained the learning process of students with learning disabilities and how educators developed instructional methods that complement the learner’s needs. The central research question was, “What is the lived experience of 9-12th-grade mathematics teachers in supporting students with differing learning abilities in math computation and problem-solving?” This study design was based on the transcendental phenomenological methodology of Moustakas (1994). The participants included 12 high school cyber teachers of general and special education students with specific learning disabilities in math computation and math problem-solving. Data were gathered through a journal prompt, individual interviews, and focus groups. The data analysis identified trends in the data and highlighted the successes and failures of various approaches used in the classroom. Three themes emerged from the study: enhanced engagement and learning environment, cognitive load and assessment strategies, and supportive and inclusive instructional practices. This study uncovered educators' lived experiences using personalized instructional strategies, interactive and adaptive technology, and instructional design approaches to reduce learners’ cognitive load. This research provided insights into high school educators' experiences using these methods of teaching math in cyber classrooms to students with disabilities.
Since August 09, 2024
Included in
Mathematics Commons , Special Education and Teaching Commons
- Collections
- Faculty Expert Gallery
- Theses and Dissertations
- Conferences and Events
- Open Educational Resources (OER)
- Explore Disciplines
Advanced Search
- Notify me via email or RSS .
Faculty Authors
- Submit Research
- Expert Gallery Login
Student Authors
- Undergraduate Submissions
- Graduate Submissions
- Honors Submissions
Home | About | FAQ | My Account | Accessibility Statement
Privacy Copyright
- Comment Comments
- Save Article Read Later Read Later
How Chain-of-Thought Reasoning Helps Neural Networks Compute
March 21, 2024

Writing out intermediate steps can make it easier to solve problems.
Nick Slater for Quanta Magazine
Introduction
Your grade school teacher probably didn’t show you how to add 20-digit numbers. But if you know how to add smaller numbers, all you need is paper and pencil and a bit of patience. Start with the ones place and work leftward step by step, and soon you’ll be stacking up quintillions with ease.
Problems like this are easy for humans, but only if we approach them in the right way. “How we humans solve these problems is not ‘stare at it and then write down the answer,’” said Eran Malach , a machine learning researcher at Harvard University. “We actually walk through the steps.”
That insight has inspired researchers studying the large language models that power chatbots like ChatGPT. While these systems might ace questions involving a few steps of arithmetic, they’ll often flub problems involving many steps, like calculating the sum of two large numbers. But in 2022, a team of Google researchers showed that asking language models to generate step-by-step solutions enabled the models to solve problems that had previously seemed beyond their reach. Their technique, called chain-of-thought prompting, soon became widespread, even as researchers struggled to understand what makes it work.
Now, several teams have explored the power of chain-of-thought reasoning by using techniques from an arcane branch of theoretical computer science called computational complexity theory. It’s the latest chapter in a line of research that uses complexity theory to study the intrinsic capabilities and limitations of language models. These efforts clarify where we should expect models to fail, and they might point toward new approaches to building them.
“They remove some of the magic,” said Dimitris Papailiopoulos , a machine learning researcher at the University of Wisconsin, Madison. “That’s a good thing.”
Training Transformers
Large language models are built around mathematical structures called artificial neural networks. The many “neurons” inside these networks perform simple mathematical operations on long strings of numbers representing individual words, transmuting each word that passes through the network into another. The details of this mathematical alchemy depend on another set of numbers called the network’s parameters, which quantify the strength of the connections between neurons.
To train a language model to produce coherent outputs, researchers typically start with a neural network whose parameters all have random values, and then feed it reams of data from around the internet. Each time the model sees a new block of text, it tries to predict each word in turn: It guesses the second word based on the first, the third based on the first two, and so on. It compares each prediction to the actual text, then tweaks its parameters to reduce the difference. Each tweak only changes the model’s predictions a tiny bit, but somehow their collective effect enables a model to respond coherently to inputs it has never seen.
Researchers have been training neural networks to process language for 20 years. But the work really took off in 2017, when researchers at Google introduced a new kind of network called a transformer.
“This was proposed seven years ago, which seems like prehistory,” said Pablo Barceló , a machine learning researcher at the Pontifical Catholic University of Chile.
What made transformers so transformative is that it’s easy to scale them up — to increase the number of parameters and the amount of training data — without making training prohibitively expensive. Before transformers, neural networks had at most a few hundred million parameters; today, the largest transformer-based models have more than a trillion. Much of the improvement in language-model performance over the past five years comes from simply scaling up.
Transformers made this possible by using special mathematical structures called attention heads, which give them a sort of bird’s-eye view of the text they’re reading. When a transformer reads a new block of text, its attention heads quickly scan the whole thing and identify relevant connections between words — perhaps noting that the fourth and eighth words are likely to be most useful for predicting the 10th. Then the attention heads pass words along to an enormous web of neurons called a feedforward network, which does the heavy number crunching needed to generate the predictions that help it learn.
Real transformers have multiple layers of attention heads separated by feedforward networks, and only spit out predictions after the last layer. But at each layer, the attention heads have already identified the most relevant context for each word, so the computationally intensive feedforward step can happen simultaneously for every word in the text. That speeds up the training process, making it possible to train transformers on increasingly large sets of data. Even more important, it allows researchers to spread the enormous computational load of training a massive neural network across many processors working in tandem.
To get the most out of massive data sets, “you have to make the models really large,” said David Chiang , a machine learning researcher at the University of Notre Dame. “It’s just not going to be practical to train them unless it’s parallelized.”
However, the parallel structure that makes it so easy to train transformers doesn’t help after training — at that point, there’s no need to predict words that already exist. During ordinary operation, transformers output one word at a time, tacking each output back onto the input before generating the next word, but they’re still stuck with an architecture optimized for parallel processing.
As transformer-based models grew and certain tasks continued to give them trouble, some researchers began to wonder whether the push toward more parallelizable models had come at a cost. Was there a way to understand the behavior of transformers theoretically?
The Complexity of Transformers
Theoretical studies of neural networks face many difficulties, especially when they try to account for training. Neural networks use a well-known procedure to tweak their parameters at each step of the training process. But it can be difficult to understand why this simple procedure converges on a good set of parameters.
Rather than consider what happens during training, some researchers study the intrinsic capabilities of transformers by imagining that it’s possible to adjust their parameters to any arbitrary values. This amounts to treating a transformer as a special type of programmable computer.
“You’ve got some computing device, and you want to know, ‘Well, what can it do? What kinds of functions can it compute?’” Chiang said.
These are the central questions in the formal study of computation. The field dates back to 1936, when Alan Turing first imagined a fanciful device , now called a Turing machine, that could perform any computation by reading and writing symbols on an infinite tape. Computational complexity theorists would later build on Turing’s work by proving that computational problems naturally fall into different complexity classes defined by the resources required to solve them.
In 2019, Barceló and two other researchers proved that an idealized version of a transformer with a fixed number of parameters could be just as powerful as a Turing machine. If you set up a transformer to repeatedly feed its output back in as an input and set the parameters to the appropriate values for the specific problem you want to solve, it will eventually spit out the correct answer.
That result was a starting point, but it relied on some unrealistic assumptions that would likely overestimate the power of transformers. In the years since, researchers have worked to develop more realistic theoretical frameworks.
One such effort began in 2021, when William Merrill , now a graduate student at New York University, was leaving a two-year fellowship at the Allen Institute for Artificial Intelligence in Seattle. While there, he’d analyzed other kinds of neural networks using techniques that seemed like a poor fit for transformers’ parallel architecture. Shortly before leaving, he struck up a conversation with the Allen Institute for AI researcher Ashish Sabharwal , who’d studied complexity theory before moving into AI research. They began to suspect that complexity theory might help them understand the limits of transformers.
“It just seemed like it’s a simple model; there must be some limitations that one can just nail down,” Sabharwal said.
The pair analyzed transformers using a branch of computational complexity theory, called circuit complexity, that is often used to study parallel computation and had recently been applied to simplified versions of transformers. Over the following year, they refined several of the unrealistic assumptions in previous work. To study how the parallel structure of transformers might limit their capabilities, the pair considered the case where transformers didn’t feed their output back into their input — instead, their first output would have to be the final answer. They proved that the transformers in this theoretical framework couldn’t solve any computational problems that lie outside a specific complexity class. And many math problems, including relatively simple ones like solving linear equations, are thought to lie outside this class.
Basically, they showed that parallelism did come at a cost — at least when transformers had to spit out an answer right away. “Transformers are quite weak if the way you use them is you give an input, and you just expect an immediate answer,” Merrill said.
Thought Experiments
Merrill and Sabharwal’s results raised a natural question — how much more powerful do transformers become when they’re allowed to recycle their outputs? Barceló and his co-authors had studied this case in their 2019 analysis of idealized transformers, but with more realistic assumptions the question remained open. And in the intervening years, researchers had discovered chain-of-thought prompting, giving the question a newfound relevance.
Merrill and Sabharwal knew that their purely mathematical approach couldn’t capture all aspects of chain-of-thought reasoning in real language models, where the wording in the prompt can be very important . But no matter how a prompt is phrased, as long as it causes a language model to output step-by-step solutions, the model can in principle reuse the results of intermediate steps on subsequent passes through the transformer. That could provide a way to evade the limits of parallel computation.
Meanwhile, a team from Peking University had been thinking along similar lines, and their preliminary results were positive. In a May 2023 paper, they identified some math problems that should be impossible for ordinary transformers in Merrill and Sabharwal’s framework, and showed that intermediate steps enabled the transformers to solve these problems.
In October, Merrill and Sabharwal followed up their earlier work with a detailed theoretical study of the computational power of chain of thought. They quantified how that extra computational power depends on the number of intermediate steps a transformer is allowed to use before it must spit out a final answer. In general, researchers expect the appropriate number of intermediate steps for solving any problem to depend on the size of the input to the problem. For example, the simplest strategy for adding two 20-digit numbers requires twice as many intermediate addition steps as the same approach to adding two 10-digit numbers.
Examples like this suggest that transformers wouldn’t gain much from using just a few intermediate steps. Indeed, Merrill and Sabharwal proved that chain of thought only really begins to help when the number of intermediate steps grows in proportion to the size of the input, and many problems require the number of intermediate steps to grow much larger still.
The thoroughness of the result impressed researchers. “They really pinned this down,” said Daniel Hsu , a machine learning researcher at Columbia University.
Merrill and Sabharwal’s recent work indicates that chain of thought isn’t a panacea — in principle, it can help transformers solve harder problems, but only at the cost of a lot of computational effort.
“We’re interested in different ways of getting around the limitations of transformers with one step,” Merrill said. “Chain of thought is one way, but this paper shows that it might not be the most economical way.”
Back to Reality
Still, researchers caution that this sort of theoretical analysis can only reveal so much about real language models. Positive results — proofs that transformers can in principle solve certain problems — don’t imply that a language model will actually learn those solutions during training.
And even results that address the limitations of transformers come with caveats: They indicate that no transformer can solve certain problems perfectly in all cases. Of course, that’s a pretty high bar. “There might be special cases of the problem that it could handle just fine,” Hsu said.
Despite these caveats, the new work offers a template for analyzing different kinds of neural network architectures that might eventually replace transformers. If a complexity theory analysis suggests that certain types of networks are more powerful than others, that would be evidence that those networks might fare better in the real world as well.
Chiang also stressed that research on the limitations of transformers is all the more valuable as language models are increasingly used in a wide range of real-world applications, making it easy to overestimate their abilities.
“There’s actually a lot of things that they don’t do that well, and we need to be very, very cognizant of what the limitations are,” Chiang said. “That’s why this kind of work is really important.”
Get highlights of the most important news delivered to your email inbox
Comment on this article
Quanta Magazine moderates comments to facilitate an informed, substantive, civil conversation. Abusive, profane, self-promotional, misleading, incoherent or off-topic comments will be rejected. Moderators are staffed during regular business hours (New York time) and can only accept comments written in English.

Next article
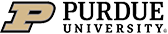
A Mathematical Theory of Communication with Graphs and Symbols
This work will introduce a channel conceptualization and possible coding scheme for Graph-and-Symbol (GS) Communication. While Claude Shannon’s mathematical model for communication employed graphs to describe relationships and confusability among traditional time-sequenced signals, little work as been done to describe non-linear communication with graphs where we transmit and receive physical structures of information. The principal contribution of this work is to introduce a mathematical framework for communication with graphs which have symbols assigned to vertices. This looks like a molecule, and so we may think of these messages as coded forms of molecular communication.
At this time, many problems in this area will (and may remain) computationally intractable, but as the field of graph theory continues to develop, new tools and techniques may emerge to solve standing problems in this new subfield of communication.
Graphs present two difficulties: first, they contain ambiguities among their vertices and do not have an a priori canonical ordering, and second, the relationships among graphs lack structural regularities which we see in traditional error control coding lattices. There are no Galois fields to exploit over graph-based codes as we have with cyclic codes, for example. Furthermore, the shear number of graphs of order n grows so rapidly that it is difficult to account for the neighborhoods around codewords and effectively reduce communication errors which may occur. The more asymmetric a graph is, the more orderings on symbols it can support. However, asymmetries complicate the computation of channel transition probabilities, which are the cornerstone of all communication theory.
In the prologue, the reader will be introduced to a new educational tool for designing traditional binary cyclic codes.
1 through 10 will detail the development of Graph-and-Symbol (GS) Commu- nication to date followed by two example codes which demonstrate the power of structuring information on graphs.
Chapter 13 onward will review the preliminary work in another area of research, disjoint from the main body. It is included here for posterity and special interests in applying graphs to solving other problems in signal processing. It begins with an introduction of spacetime raythic graphs. We propose a new chamfering paradigm for connecting neighboring pixels which approximates solutions to the eikonal equation. We show that some raythic graphs possess structures with multiple, differing solutions to eikonal wavefront propagation which are essential to the construction of the Umbral Transform. This umbral transform emulates ray casting effects, such as shadows and diffraction within an image space, from a network-flow algorithm.
This work may be duplicated in whole or in part for educational purposes only. All other rights of this work are reserved by the author, Timothy Arthur Terlep Jr., of Rose-Hulman Institute of Technology, Terre Haute, IN (effective August 2024), and subject to the rules and regulations of the Graduate School of Purdue University.
Readers may contact the author with any comments and questions at [email protected]
Degree Type
- Doctor of Philosophy
- Electrical and Computer Engineering
Campus location
- West Lafayette
Advisor/Supervisor/Committee Chair
Advisor/supervisor/committee co-chair, additional committee member 2, additional committee member 3, usage metrics.
- Data communications
- Molecular, biological, and multi-scale communications
- Signal processing
- Communications engineering not elsewhere classified
- Engineering education
- Spatial data and applications
- Coding, information theory and compression
- Combinatorics and discrete mathematics (excl. physical combinatorics)
A Heuristic Philosophical Discourse on Various Applications of Abstract Differential Geometry in Quantum Gravity Research † † thanks: This paper is a philosophical distillation of the basic concepts and central results of this author’s ongoing research project, spanning the last three decades, of applying Anastasios Mallios’s Abstract Differential Geometry to the ‘persistently stubborn’ problem of formulating a conceptually sound, mathematically consistent and calculationally finite Quantum Theory of Gravity. The paper will be posted at the General Relativity and Quantum Cosmology website www.arXiv.org/gr-qc before October 2024. This work may be viewed as the philosophical résumé and aftermath (:after the Maths!) of the following published papers [ 62 , 63 , 64 , 73 , 74 , 75 , 76 , 77 , 78 , 79 , 84 , 85 ] , as well as a sequel to the Dodecalogue paper [ 79 ] in the aftermath of the recent publication [ 80 ] and the pre-prints [ 81 , 82 , 83 ] , which are currently work-in-progress in the pipeline. In turn, a longer version of the paper will constitute a chapter in a research monograph type of book that we had and have been working on, in collaboration with the late Professor Anastasios Mallios, since 2003 [ 65 ] .
In the present paper, we outline and expound the fundamental and novel qualitative-cum-philosophical premises, principles, ideas, concepts, constructions and results that originate from our ongoing research project of applying the conceptual panoply and the technical machinery of Abstract Differential Geometry (ADG) to various persistently outstanding issues in Quantum Gravity (QG) [ 62 , 63 , 64 , 73 , 74 , 75 , 76 , 77 , 78 , 79 , 84 , 85 ] . This paper may be regarded as a sequel to the paper [ 79 ] in the aftermath of the paper [ 80 ] , which is currently in press. At the end of the paper, we discuss the potential philosophical repercussions of two possible future research routes that the main stream of our applications of ADG to QG may bifurcate towards in view of three independent, but overlapping, research papers that are currently under development [ 81 , 82 , 83 ] .
PACS numbers : 04.60.-m, 04.20.Gz, 04.20.-q
Key words : Natural Philosophy of Quantum Gravity and Quantum Yang-Mills Gauge Theories; Abstract Differential Geometry; sheaf theory; sheaf cohomology; category theory; topos theory; geometric prequantisation; canonical quantisation; background spacetime manifold independence; quantum gravity as a purely quantum gauge theory; gauge theory of the third kind; third sheaf cohomological quantisation of gravity and gauge theories
1 Prolegomena-cum-Motivation: Why Adopt a Philosophical Stance in Quantum Gravity Research?
Quantum Gravity (QG), very broadly speaking, is an attempt to unite the laws of Physics that describe dynamics at large (:cosmological) scales—as encoded in Einstein’s General Relativity (GR) equations for the gravitational field that guides the motion of large material objects, with the dynamical laws for the other three fundamental forces that guide matter fields and their quantum particles at small (:subatomic) scales—as encoded in Quantum Theory and its application to Special Relativistic field physics, commonly known as Quantum Field Theory (QFT). 1 1 1 In this paper, we use the terms QFT and Quantum Gauge (QGT) or Quantum Yang-Mills Theories (QYMT) of matter interchangeably.
There is currently a plethora of various and glaringly diverse approaches to QG, and it is not the ‘vain’ or ‘quixotic’ aim of this paper to list them all herein, let alone to review their conceptual and technical import or their successes and shortcomings. On the one hand, there is no unanimous agreement on what the ‘right’ or ‘correct’ approach to QG is and the diversity of the various different approaches—from string theory [ 25 , 91 ] , 2 2 2 For current developments in this field, see the second reference above [ 91 ] . to loop quantum gravity [ 2 , 3 , 4 , 44 ] 3 3 3 For an exhaustive modern exposition of loop quantum gravity, see the last reference [ 44 ] above. and causal sets [ 6 , 93 , 94 , 95 ] , for example—exemplifies exactly that.
On the other hand, it would be informative for the reader to list a triplet of very general predicates and characteristics— theory making ‘imperatives’ as it were—that the desired and hitherto elusive Quantum Theory of Gravity should possess, properties that all the aforementioned diverse approaches to QG aim or aspire to satisfy in one way or another:
Economy (E): Conceptual clarity and depth, as well as economy and simplicity of the underlying fundamental physical theory construction principles ;
Mathematical Consistency (M): Internal mathematical consistency (:self-consistency), mathematical representability and predictive power, technical innovation and efficacy, wide ranging utility and versatility of application, and, as a bonus, abstract mathematical simplicity and beauty ; 4 4 4 With respect to this last point, we tacitly assume that‘ beauty , especially of the mathematical kind, is in the eye of the beholder ’—in this case, of the theoretician/mathematician—hence it is largely of a subjective aesthetic nature .
Finiteness (F): Calculational freedom from unphysical (observable) infinities, anomalies and ‘singularities’ of all kinds at which the aforementioned physical laws might seem to break down, hence are deemed to become unphysical, uninformative, unpredictive and, ultimately, practically useless and therefore obsolete .
We will henceforth refer to the triplet of desirable QG traits above by the acronym EMF1 .
‘Mission Statement’: Our philosophical discourse in the present paper will focus on showing, arguing and discussing, with extensive references to the existing published literature, that the ADG-theoretic approach to QG, hitherto to be referred to as ADG-gravity , goes a long way towards satisfying the EMF1 triptych of theory making imperatives above.
At the same time, the basic ‘justification’ for engaging in a philosophical discourse about the import of ADG-gravity in QG research is another triplet EMF2 :
Explication (E): Explanation and interpretation of new concepts, techniques and results from applying ADG in QG research ;
Mathematical Efficacy (M): Discussion of the mathematical power of ADG in addressing and resolving certain key QG problems and issues associated with EMF1 ;
Future Prospects and Developments (F): Discussion of future prospects for QG theory growth and development, as well as anticipation in what direction will QG research move in view of the new ideas and theoretical paradigms that ADG brings forth.
Expository Declaration 1: In the sections that follow, whenever we discuss and analyse a qualitative-philosophical aspect of ADG-gravity, at the end of the discussion we will mark it by boldface markers in brackets like, for example, (F1): this would mean that the qualitative/philosophical characteristic of ADG-gravity being analysed and discussed satisfies the Finiteness 1 aspect of the EMF1 triplet above.
We would like to kick-off our philosophical discourse on applications of ADG in QG with a very telling quote of Gerard ’t Hooft just after the turn of the new millennium [ 98 ] , which kind of gives a raison d’être and a raison de faire to our endeavours herein:
(Q1) “ …The problems of quantum gravity are much more than purely technical ones. They touch upon very essential philosophical issues 5 5 5 Our emphasis. … ”
the basic idea behind the quotation above is that it motivates us to go beyond the ‘technicalities’ of various formal conceptual and mathematical issues in current QG research, and discuss the deeper semantics and philosophical nature underlying, or even possibly transcending, those conceptual and mathematical ‘technicalities’. Which brings us to the second Expository Declaration of this paper.
Expository Declaration 2: It is a conscious decision and choice of this author not to include a single quantitative expression ( e.g. , technical mathematical formula) in the present paper, which is purely of a qualitative (discursive) character. For concise definitions of formal technical concepts and their associated mathematical formulae/modelling/equations, the reader will be directly referred to the relevant published literature.
Expository Declaration 3: Our philosophical treatise below is organised in short sections that end with ‘ Aphorisms ’—short ‘statements’ that distill the main philosophical gist of each section.
2 General Relativity is formulated by the Classical Differential Geometry on a Pointed Smooth Base Spacetime Manifold
∙ ∙ \bullet ∙ General Relativity (GR), the classical theory of gravity, is inextricably tied to a 𝒞 ∞ superscript 𝒞 \mathcal{C}^{\infty} caligraphic_C start_POSTSUPERSCRIPT ∞ end_POSTSUPERSCRIPT -smooth ( alias , differential) base spacetime manifold M 𝑀 M italic_M for its mathematical formulation via Classical Differential Geometry (CDG)—the Newtonian Calculus based geometry of differential manifolds.
∙ ∙ \bullet ∙ In turn, the differential manifold M 𝑀 M italic_M , as a geometrical point-set, is equivalent to the structure algebra sheaf 𝒞 ∞ ( M ) superscript 𝒞 𝑀 \mathcal{C}^{\infty}(M) caligraphic_C start_POSTSUPERSCRIPT ∞ end_POSTSUPERSCRIPT ( italic_M ) of germs of smooth coordinate functions of its points.
∙ ∙ \bullet ∙ In GR, the dynamical law of gravity is formulated as Einstein’s nonlinear partial differential equations of a 𝒞 ∞ superscript 𝒞 \mathcal{C}^{\infty} caligraphic_C start_POSTSUPERSCRIPT ∞ end_POSTSUPERSCRIPT -smooth spacetime metric g μ ν subscript 𝑔 𝜇 𝜈 g_{\mu\nu} italic_g start_POSTSUBSCRIPT italic_μ italic_ν end_POSTSUBSCRIPT (and its derivatives), whose 10 10 10 10 components are supposed to represent the gravitational field potentials.
∙ ∙ \bullet ∙ Accordingly, GR’s Priciple of General Covariance (PGC) is mathematically represented by the group Diff ( M ) Diff 𝑀 \mathrm{Diff}(M) roman_Diff ( italic_M ) of (active) diffeomorphisms of M 𝑀 M italic_M . 6 6 6 By definition, a diffeomorphism of a smooth manifold M 𝑀 M italic_M is an automorphism of M 𝑀 M italic_M that preserves its differential geometric (:smooth) structure, as the latter is effectively encoded in the structure algebra sheaf 𝒞 ∞ ( M ) superscript 𝒞 𝑀 \mathcal{C}^{\infty}(M) caligraphic_C start_POSTSUPERSCRIPT ∞ end_POSTSUPERSCRIPT ( italic_M ) of smooth coordinate functions of M 𝑀 M italic_M ’s point events, as noted above.
Aphorism 1. GR uses CDG to formulate the gravitational dynamics as a differential equation for the smooth metric (and its derivatives) on a background geometrical differential spacetime manifold M 𝑀 M italic_M . In turn, the PGC of GR is represented by the spacetime diffeomorphism group Diff ( M ) Diff 𝑀 \mathrm{Diff}(M) roman_Diff ( italic_M ) of the underlying 𝒞 ∞ superscript 𝒞 \mathcal{C}^{\infty} caligraphic_C start_POSTSUPERSCRIPT ∞ end_POSTSUPERSCRIPT -smooth manifold M 𝑀 M italic_M .
2.1 Feynman’s ‘Fancy-Schmanzy’ Differential Geometry and Isham’s No-Go of Differential Geometry in Quantum Gravity
Arguably, the smooth background geometrical spacetime manifold, whether curved or flat, is responsible for both the singularities of the smooth gravitational field g μ ν subscript 𝑔 𝜇 𝜈 g_{\mu\nu} italic_g start_POSTSUBSCRIPT italic_μ italic_ν end_POSTSUBSCRIPT of GR [ 22 , 23 , 27 , 28 , 29 , 30 , 71 ] , as well as for the pestilential non-renormalisable unphysical infinities that assail QFT on flat Minkowski spacetime [ 26 , 5 ] .(F1)
Mainly due to these pathologies, QG researchers as early as Feynman and as recently as Isham, have questioned altogether the use of CDG on a smooth spacetime manifold, whether flat (QFT) or curved (GR), as the appropriate mathematical framework via which to formulate QG.
In this line of thought, we first recall from Bryan Hatfield’s Quantum Gravity Foreword to Feynman’s Lectures on Gravitation [ 19 ] the following telling excerpt:
(Q2) “…Thus it is no surprise that Feynman would recreate general relativity from a non-geometrical viewpoint. The practical side of this approach is that one does not have to learn some ‘ fancy-schmanzy ’ (as he liked to call it) differential geometry in order to study gravitational physics. (Instead, one would just have to learn some quantum field theory.) However, when the ultimate goal is to quantize gravity, Feynman felt that the geometrical interpretation just stood in the way. From the field theoretic viewpoint, one could avoid actually defining—up front—the physical meaning of quantum geometry, fluctuating topology, space-time foam, etc. , and instead look for the geometrical meaning after quantization… Feynman certainly felt that the geometrical interpretation is marvellous, ‘ but the fact that a massless spin- 2 2 2 2 field can be interpreted as a metric was simply a coincidence that might be understood as representing some kind of gauge invariance’ 7 7 7 Our emphasis of Feynman’s words as quoted by Bryan Hatfield. …”
And he further added categorimatically in [ 18 ] that:
(Q3) “ …the simple ideas of [differential] geometry, extended down to infinitely small, are wrong. ”
While more recently, Chris Isham firmly posited in [ 31 ] :
(Q4) “ …at the Planck-length scale, differential geometry is simply incompatible with quantum theory…[so that] one will not be able to use differential geometry in the true quantum-gravity theory… ”
We may distill the above to our second Aphorism:
Aphorism 2. All the anomalies and pathologies of GR and QFT in the form of singularities and other unphysical infinities originate from the a priori assumption of a background differential manifold as a geometrical model for spacetime.(F1)
Thus, we can combine Aphorisms 1 and 2 to the following ‘ vicious circle ’ statement:
Fundamental Vicious Circle. If we wish to formulate the dynamical laws of QG as differential equations proper, it seems that we have to use the concepts and techniques of CDG on a smooth manifold. However, the latter is responsible for both the singularities of GR and the unphysical the infinities of QFT—sites in the spacetime manifold where the laws of physics appear to break down or lead to unphysical infinities for important observable quantities; hence, we seem to arrive at an impasse. 8 8 8 The reader should note here that, in the three quotes above, both Feynman and Isham question the Mathematical Efficacy of CDG in QG ( M1,F1, M2).
3 Enter ADG
Below, we itemise the basic tenets of ADG:
∙ ∙ \bullet ∙ Abstract Differential Geometry is more of a Leibnizian (:relational), rather than Newtonian (:geometrical), purely algebraic (:sheaf-theoretic and homological algebraic) way of doing differential geometry (:Calculus) [ 15 , 8 ] , without at all recourse to or dependence on a pointed, smooth geometric locally Euclidean background space (:a 𝒞 ∞ superscript 𝒞 \mathcal{C}^{\infty} caligraphic_C start_POSTSUPERSCRIPT ∞ end_POSTSUPERSCRIPT -smooth manifold) for its concepts, technical machinery and constructions thereof [ 47 , 48 , 53 ] .(M2)
∙ ∙ \bullet ∙ The most fundamental concept of ADG is that of connection ( viz. generalised differential) 𝒟 𝒟 \mathcal{D} caligraphic_D acting on a vector sheaf ℰ ℰ \mathbf{\mathcal{E}} caligraphic_E over a suitably algebraized, by a certain so-called algebra structure sheaf 𝐀 𝐀 \mathbf{A} bold_A of generalised coordinates or what Mallios coined ‘ arithmetics , arbitrary topological space X 𝑋 X italic_X as a linear and Leibnizian sheaf morphism. The pair ( 𝒟 , ℰ ) 𝒟 ℰ (\mathcal{D},\mathbf{\mathcal{E}}) ( caligraphic_D , caligraphic_E ) is coined an ADG-field .(M1,M2)
∙ ∙ \bullet ∙ Based on the concept of connection, ADG erects the whole edifice of CDG (plus more), but in the manifest absence of a background geometrical manifold.
∙ ∙ \bullet ∙ Thus, a new, entirely algebraic (:relational) notion of geometry emerges, whereby, geometry does not pertain to the configuration states (‘shape’) and measurement of objects living in an a priori posited (:postulated) ether-like background space [ 20 ] , but rather, it derives from the algebraic (:dynamical) relations between the objects that live on that ‘space’. 9 9 9 These ‘objects’ are the very ADG-connection fields acting on the sections of the vector sheaves involved.
∙ ∙ \bullet ∙ The last bullet point put in more physical terms: physical geometry (: physical ‘spacetime’ ) is not a priori posited like the differential spacetime manifold M 𝑀 M italic_M of GR. Rather, it derives from the dynamics (:the differential equations involving the connection field 𝒟 𝒟 \mathcal{D} caligraphic_D ) of the ‘objects’ (:the dynamical physical fields themselves).(E2,M2)
We may distill the essential gist of the bullets above to the following Aphorism:
Aphorism 3. From the ADG-theoretic perspective, physical geometry (or physical ‘spacetime’) derives from, or is the outcome of, the algebraic dynamical relations between the ADG-fields (:the physical laws, which are formulated categorically as equations between he relevant sheaf morphisms that the ADG-connection fields correspond to). 10 10 10 In this regard, one may think of the more commonly used mathematical term ‘ solution space ’ derived from a set of (differential) equations set up (on a manifold) by the usual CDG-means. That ‘solution space’ is the ‘physical geometry’. (E2,M2)
4 The Point of Pointlessness and Finiteness: the ADG Evasion of Spacetime Singularities and the Management of Infinities
∙ ∙ \bullet ∙ In the homological algebraic (:category-theoretic) setting of ADG, the singular, ideal and physically unrealistic notion of a geometrical point 11 11 11 The ‘ physically unrealistic ’ nature of a geometrical point (:an ideal spacetime event, so to speak) can be appreciated if one considers the fact that one cannot localise an ‘event’ (:measure the value of, say, the gravitational field at a point) with more accuracy than the Planck length without creating a black hole (:think for example of the inner Schwartzschild singularity right at the point-mass source, where the gravitational field blows up without bound. is meaningless.(F1)
∙ ∙ \bullet ∙ Mutatis mutandis for the continuous infinity of point-events that the smooth spacetime manifold accommodates: for example, the non-renormalisable infinities of QFT in Minkowski spacetime effectively arise from the fact that one can in principle pack an uncountable infinity of events (:field values) in a finite spacetime volume.(F1)
∙ ∙ \bullet ∙ In both the point of pointlessness and finiteness, ADG has been applied towards formulating on the one hand a locally finite, causal and quantal version of Lorentzian vacuum Einstein gravity and free Yang-Mills theories, and on the other, the same dynamical equations are seen to hold over spaces that are everywhere dense with singularities of the most unmanageable kind from the point of view of ADG [ 50 , 51 , 55 , 56 , 62 , 63 , 64 , 66 , 67 , 68 , 75 ] .
∙ ∙ \bullet ∙ The results above are due to the purely algebraic and background pointed continuous spacetime manifold independent character of ADG [ 47 , 48 , 53 , 79 ] .
Again, we may distill the essential gist of the bullets above to the following Aphorism, our fourth one:
Aphorism 4. We can formulate the dynamical equations of Einstein and Yang-Mills over highly pathological and problematic spaces, especially when viewed from the smooth background spacetime manifold perspective of CDG. Thus, singularities (and their associated infinities) are not insuperable obstacles and ‘sites’ where the differential equations that represent the dynamical field laws of Nature appear to break down. Not only we can evade them by ADG-theoretic means, but also we can ‘calculate’ (:do Calculus!) in their very presence, in spite of them. The inherently algebraic differential geometric mechanism of ADG is genuinely background smooth spacetime independent, hence it does stumble on its inherent anomalies and pathologies [ 50 , 51 , 55 , 56 , 62 , 63 , 64 , 66 , 67 , 68 , 75 ] . (E1,M1,F1,E2,M2) Thus, by ADG-theoretic means we are able to evade the ‘vicious circle’ statement that we made earlier after the first two aphorisms, as well as to question both Feynman’s (Q2,3) and Isham’s (Q4) doubts about using differential geometric ideas in QG research.
4.1 ADG Gravity: Einstein’s Purely Algebraic Description of Reality in the Quantum Deep
In the Philosophy of Physics there is a well established view that Einstein in vain pursued his Unified Field Theory 12 12 12 Einstein had originally coined it Unitary Field Theory instead [ 17 ] . research on a continuous spacetime manifold in spite of the inherently finitistic and algebraic description of Physical Reality at subatomic scales that Quantum Theory brought about.
The following couple of quotations from Einstein’s The Meaning of Relativity [ 17 ] testify to that:
(Q5) “ …One can give good reasons why reality cannot at all be represented by a continuous field. From the quantum phenomena it appears to follow with certainty that a finite system of finite energy can be completely described by a finite set of numbers . 13 13 13 Our emphasis. This does not seem to be in accordance with a continuum theory, and must lead to an attempt to find a purely algebraic theory for the description of reality 14 14 14 Our emphasis. … ”
and a similar quote from [ 17 ] that also mentions singularities:
(Q6) “ …Is it conceivable that a field theory 15 15 15 Here, Einstein was implicitly alluding to his Unitary Field Theory, which, according to his vision, could hopefully ‘explain away’ quantum phenomena. permits one to understand the atomistic and quantum structure of reality? Almost everybody will answer this question with ‘no’. But I believe that at the present time nobody knows anything reliable about it. This is so because we cannot judge in what manner and how strongly the exclusion of singularities reduces the manifold of solutions. We do not possess any method at all to derive systematically solutions that are free of singularities 16 16 16 Our emphasis. … ” [ 17 ]
In connection with the two Einstein quotes (Q5,6) above, with our fifth Aphorism next, which closes this section, we kill two birds with one stone:
Aphorism 5. ADG is an entirely algebraic method for formulating gravity and quantum Yang-Mills theories of matter field theoretically and finitistically by evading singularities and without any dependence on a background geometrical spacetime continuum, with its all inherent singularities and associated unphysical smooth field infinities [ 64 , 75 , 78 , 79 , 80 ] .
4.2 Sheaf Theory and the Transition from Local to Global
At the basis of ADG ( alias , The Geometry of Vector Sheaves ), lie the purely algebraic methods of sheaf theory [ 7 , 47 , 48 , 53 ] . Unlike the geometry of smooth vector bundles, which features prominently in the geometrisation of Physics that gauge theory brought about [ 24 ] , sheaf theory has been slow in coming in QG research.
Structures closely related to sheaves are special type of categories called topoi [ 46 , 45 ] , which are pointless spaces having their own internal logic. Topoi have been applied to both quantum logic [ 9 , 10 , 11 , 12 ] and quantum spacetime structures, including QG research [ 35 , 36 , 37 , 38 , 39 , 40 , 76 , 77 , 80 , 69 , 70 ]
In this regard, very early on, Rudolph Haag [ 26 ] intuited the great import that sheaf theory could bring to QFT as sheaves are structures tailor-cut to encode and transmit information (from local measurements of quantum observables, for example) from local to global in QFT:
(Q7) “ Germs. We may take it as the central message of Quantum Field Theory that all information characterizing the theory is strictly local i.e. expressed in the structure of the theory in an arbitrarily small neighborhood of a point . 17 17 17 Our emphasis. For instance in the traditional approach the theory is characterized by a Lagrangean density. Since the quantities associated with a point are very singular objects, it is advisable to consider neighborhoods. This means that instead of a fiber bundle one has to work with a sheaf. The needed information consists then of two parts: first the description of the germs, secondly the rules for joining the germs to obtain the theory in a finite region 18 18 18 Again, emphasis is ours. … ”
Indeed, the vector and algebra sheaves involved in ADG and their associated topoi have been used very successfully in analysing the structure of the algebras of local quantum observables and how these stitch up from local to global [ 105 , 106 ] . Moreover, the ADG sheaves and their associated topoi have been applied to address important issues in QG research [ 76 , 77 , 79 , 70 , 69 , 80 ] .
5 Revisiting Feynman: Gravity as Gauge Theory
Returning to the Feynman quote in Section 2, we wish to dwell a bit on his remark that the fact that the gravitational field was identified with the smooth spacetime metric g μ ν subscript g μ ν g_{\mu\nu} italic_g start_POSTSUBSCRIPT italic_μ italic_ν end_POSTSUBSCRIPT of the CDG-based Riemannian geometry on a differential spacetime manifold, was an ‘accident’ of theory making . 19 19 19 That is to say, Einstein formulated GR as the dynamics of the metric, ‘because’ he used the CDG-based Riemannian geometry of a smooth base spacetime manifold [ 71 ] . Rather, Feynman intuited that:
The deeper character of gravity is that it is a gauge force, much like the other three fundamental forces, while the methods of CDG would be ineffective in the QG deep. 20 20 20 Similarly to what Isham said in the quote following Feynman’s.
In subsequent developments in GR, we were able to cast gravity as a gauge theory in the new Ashtekar variables involving a spin-Lorentzian gravitational connection [ 2 ] and apply the new, first-order formalism 21 21 21 The Ashtekar formalism in terms of the tetrad e μ subscript 𝑒 𝜇 e_{\mu} italic_e start_POSTSUBSCRIPT italic_μ end_POSTSUBSCRIPT and the spin-connection 𝒜 μ subscript 𝒜 𝜇 \mathcal{A}_{\mu} caligraphic_A start_POSTSUBSCRIPT italic_μ end_POSTSUBSCRIPT dynamical variables is coined first order , while the GR of Einstein is based solely on the smooth spacetime metric g μ ν subscript 𝑔 𝜇 𝜈 g_{\mu\nu} italic_g start_POSTSUBSCRIPT italic_μ italic_ν end_POSTSUBSCRIPT as its sole dynamical variable. to a new candidate for (canonical) QG called Loop Quantum Gravity [ 3 , 44 ] . Albeit, on the one hand, the metric was still implicitly involved in the dynamics in the guise of the vierbein comoving tetrad e μ subscript 𝑒 𝜇 e_{\mu} italic_e start_POSTSUBSCRIPT italic_μ end_POSTSUBSCRIPT , while the smooth spacetime manifold was still present as a geometrical background in order for the canonical formalism to be applied by the methods of CDG. 22 22 22 With all the problematic issues and pathologies that this dependence causes to QG (such as the Diff ( M ) Diff 𝑀 \mathrm{Diff}(M) roman_Diff ( italic_M ) constraint problem and the problem of time [ 32 , 33 , 34 ] .
The words in the quote above leave us with the following quandary, posited below as a rhetorical question in the light of ADG:
Is there a way to view gravity as a gauge theory, as Feynman inuited and envisaged, while still be able to apply to it differential geometric ideas, methods and techniques in spite of Feynman’s and Isham’s No-Go of CDG in QG research?
Which brings us to the next subsection about the ADG perspective on gravity as a gauge theory.
5.1 Enter ADG: Gravity as Pure Gauge Theory of the Third Kind
One of the central results of the application of ADG to QG is that:
Aphorism 6: Gravity is a pure gauge theory, without recourse to an underlying (smooth) spacetime manifold structure for either its mathematical formulation or its physical interpretation. Gravity involves the dynamics of the ADG-theoretic Einstein field ℱ E i n s t = ( ℰ , 𝒟 ) subscript ℱ E i n s t ℰ 𝒟 \mathcal{F}_{Einst}=(\mathbf{\mathcal{E}},\mathcal{D}) caligraphic_F start_POSTSUBSCRIPT italic_E italic_i italic_n italic_s italic_t end_POSTSUBSCRIPT = ( caligraphic_E , caligraphic_D ) , which is simply a connection 𝒟 𝒟 \mathcal{D} caligraphic_D on a suitable vector sheaf ℰ ℰ \mathbf{\mathcal{E}} caligraphic_E . The dynamical Einstein equations are derived from a variational principle applied on the ADG-version of the Einstein-Hilbert action functional [ 53 , 64 , 80 ] . 23 23 23 By ‘ pure gauge theory ’ above, it is meant that the sole dynamical variable in the theory is the gravitational connection 𝒟 𝒟 \mathcal{D} caligraphic_D , acting on the sections of a suitable vector sheaf ℰ ℰ \mathbf{\mathcal{E}} caligraphic_E , and nothing else. The corresponding formalism has been coined half-order formalism , to distinguish it from the first-order formalism of Ashtekar and its Loop QG outgrowth, and of course from the usual second-order formalism of the original Einstein theory (GR). (E1,M1,F1)
6 Field Solipsism and Functoriality: The Point of Spacetimelessness, Generalised Principle of General Covariance and a Different Perspective on the ‘Measurement Problem’
A geometrical point is mathematically an ideal and physically an unrealistic (:singular) entity. We discussed earlier how the sheaf-theoretic ADG and its pointless topos-theoretic extension evade the pointed background geometrical spacetime continuum of events of GR. We also noted how the ADG-gravitational connection field is the sole dynamical variable in the theory, while the underlying spacetime metric g μ ν subscript 𝑔 𝜇 𝜈 g_{\mu\nu} italic_g start_POSTSUBSCRIPT italic_μ italic_ν end_POSTSUBSCRIPT of the usual CDG-based GR is manifestly absent from our theory. This on the one hand is supposed to depict the pure gauge character of ADG-gravity à-la Feynman, and on the other, to support the aforementioned ADG-field solipsism: that is to say, that
the ADG-gravitational dynamics does not need or depend at all on a background differential spacetime manifold for either its differential geometric formulation as a differential equation proper, or for its physical interpretation. ADG-gravity is a genuinely background independent theory. The result is that the sole dynamical variable in ADG-gravity is the gravitational Einstein ADG-field ℱ E i n s t = ( ℰ , 𝒟 ) subscript ℱ 𝐸 𝑖 𝑛 𝑠 𝑡 ℰ 𝒟 \mathcal{F}_{Einst}=(\mathbf{\mathcal{E}},\mathcal{D}) caligraphic_F start_POSTSUBSCRIPT italic_E italic_i italic_n italic_s italic_t end_POSTSUBSCRIPT = ( caligraphic_E , caligraphic_D ) , a feature that has been coined field solipsism and the Einstein-Hilbert variational action principle dynamics that ℱ E i n s t = ( ℰ , 𝒟 ) subscript ℱ 𝐸 𝑖 𝑛 𝑠 𝑡 ℰ 𝒟 \mathcal{F}_{Einst}=(\mathbf{\mathcal{E}},\mathcal{D}) caligraphic_F start_POSTSUBSCRIPT italic_E italic_i italic_n italic_s italic_t end_POSTSUBSCRIPT = ( caligraphic_E , caligraphic_D ) obeys has been coined ADG-field autodynamics (:autonomous gravitational field dynamics), with no dependence whatsoever on a background spacetime manifold with its inherent gravitational singularities and unphysical field infinities [ 64 , 76 , 78 , 79 , 80 ] .(E1,M1,F1,E2,M2)
The discussion above brings to the forefront one very telling Einstein quote from [ 16 ] :
(Q8) “ Time and space are modes by which we 24 24 24 Our emphasis. think, not conditions in which we live. ”
Space and time are human inventions convenient for representing, localising and quantifying our measurements of physical observable entities.
Which brings us to the idea of spacetime point coordinates , or equivalently, spacetime determinations/localisations/measurements of events or field-values . The locution of every point field-value or event in the spacetime manifold of GR is supposed to be determined (:measured) by four real spacetime coordinates (:coordinate functions) with respect to a given coordinate system (measurement frame of location).
The Principle of General Covariance (PGC) of GR mandates that the law of gravity (:Einstein’s equations) is generally covariant; that is to say, it is invariant under any arbitrary general coordinate transformation. 25 25 25 Technically, we say that the group of symmetries of GR is G L ( 4 , ℝ ) 𝐺 𝐿 4 ℝ GL(4,\mathbb{R}) italic_G italic_L ( 4 , blackboard_R ) , the group of general linear transformations of the locally Euclidean (: ℝ 4 superscript ℝ 4 \mathbb{R}^{4} blackboard_R start_POSTSUPERSCRIPT 4 end_POSTSUPERSCRIPT ) spacetime manifold M 𝑀 M italic_M . Equivalently, we may state the PGC of GR as the Kleinian symmetry group of the background 𝒞 ∞ superscript 𝒞 \mathcal{C}^{\infty} caligraphic_C start_POSTSUPERSCRIPT ∞ end_POSTSUPERSCRIPT -smooth spacetime manifold M 𝑀 M italic_M , as follows:
The symmetry group of GR is the group Diff ( M ) Diff 𝑀 \mathrm{Diff}(M) roman_Diff ( italic_M ) of differentiable automorphisms (:diffeomorphisms) of the background smooth spacetime manifold M 𝑀 M italic_M .
By contrast, in ADG-gravity, where we have no background spacetime manifold and the ADG-field autodynamics is purely gauge ,
The gauge symmetry group sheaf is the principal sheaf 𝒜 u t 𝐀 ℰ 𝒜 𝑢 subscript 𝑡 𝐀 ℰ {\mathcal{A}}ut_{\mathbf{A}}\mathbf{\mathcal{E}} caligraphic_A italic_u italic_t start_POSTSUBSCRIPT bold_A end_POSTSUBSCRIPT caligraphic_E of structure sheaf 𝐀 𝐀 \mathbf{A} bold_A -automorphisms of the associated vector sheaf ℰ ℰ \mathbf{\mathcal{E}} caligraphic_E of the ADG-Einstein field ℱ E i n s t = ( ℰ , 𝒟 ) subscript ℱ 𝐸 𝑖 𝑛 𝑠 𝑡 ℰ 𝒟 \mathcal{F}_{Einst}=(\mathbf{\mathcal{E}},\mathcal{D}) caligraphic_F start_POSTSUBSCRIPT italic_E italic_i italic_n italic_s italic_t end_POSTSUBSCRIPT = ( caligraphic_E , caligraphic_D ) [ 64 , 76 , 78 , 79 , 80 ] .
whereby, as noted earlier, 𝐀 𝐀 \mathbf{A} bold_A is the structure sheaf of generalised arithmetics or coordinates in the theory.
In other words, the ADG-gravitational dynamics, which is formulated entirely categorically in terms of the connection 𝒟 𝒟 \mathcal{D} caligraphic_D sheaf morphism, is respected by (:‘remains invariant under’) all our generalised measurements (:arithmetics, event coordinate determinations) encoded in the structure sheaf 𝐀 𝐀 \mathbf{A} bold_A . The gravitational dynamics ‘sees through’ all our coordinate measurements (:spacetime event localisations) in A A \mathbf{A} bold_A [ 79 , 80 ] .
6.1 The Issue of Functoriality
In [ 80 ] it has been shown that the aforesaid PGC of GR, which is tantamount to 𝐀 𝐀 \mathbf{A} bold_A -invariance in ADG-gravity , is an example of the Functoriality of ADG-gravity . In other words, since the dynamics is categorically represented as equations involving the connection sheaf morphism 𝒟 𝒟 \mathcal{D} caligraphic_D of the ADG-Einstein field ℱ E i n s t = ( ℰ , 𝒟 ) subscript ℱ 𝐸 𝑖 𝑛 𝑠 𝑡 ℰ 𝒟 \mathcal{F}_{Einst}=(\mathbf{\mathcal{E}},\mathcal{D}) caligraphic_F start_POSTSUBSCRIPT italic_E italic_i italic_n italic_s italic_t end_POSTSUBSCRIPT = ( caligraphic_E , caligraphic_D ) , the PGC is represented by functors that preserve the relevant categories. 26 26 26 In category-theoretic parlance, a functor between two categories, is a map or transformation that respects the objects and arrows of the two categories. In [ 80 ] , in continuation and extension of [ 58 , 59 , 60 ] , it was shown and argued that the relevant functors are, in fact, special types of 𝐀 𝐀 \mathbf{A} bold_A -preserving functors (or 𝐀 𝐀 \mathbf{A} bold_A -morphisms) called geometric morphisms , which preserve the ‘geometric’ structure of the vector sheaves involved in the dynamics.
We distill all the foregoing discussion into our seventh Aphorism below:
Aphorism 7. In ADG-Gravity, the dynamics is purely gauge and background spacetime manifold independent and functorial, while the PGC is functorially represented in terms of the principal group sheaf of automorphisms of the relevant vector sheaves as 𝐀 𝐀 \mathbf{A} bold_A -invariance citemall14,mall15,mall13,rap15. The latter simply means that the ADG-field dynamics, which in its Einstein-Hilbert action expression involves the curvature of the connection which is an ⊗ 𝐀 subscript tensor-product 𝐀 \otimes_{\mathbf{A}} ⊗ start_POSTSUBSCRIPT bold_A end_POSTSUBSCRIPT -tensor, remains invariant (or ‘unperturbed’) by our generalised coordinate ‘measurements’ that are organised in the structure algebra sheaf 𝐀 𝐀 \mathbf{A} bold_A .(E1,M1,F1,E2,M2)
Thus, all our generalised measurements are represented in ADG as sections of the structure algebra sheaf 𝐀 𝐀 \mathbf{A} bold_A . 27 27 27 The reader should note here that the structure sheaf 𝐀 𝐀 \mathbf{A} bold_A is supposed to be a sheaf of abelian (:commutative) algebras. This reflects our primitive assumption that we always measure commutative numbers , while in the Quantum Theory it is supposed to be the ADG-version of Bohr’s Correspondence principle: although quantum observables may be noncommutative q-numbers, our measurements thereof are commutative c-numbers . In turn, the ADG-gravitational dynamics, since it is 𝐀 𝐀 \mathbf{A} bold_A -functorial, 28 28 28 Or as Mallios originally coined it: 𝐀 𝐀 \mathbf{A} bold_A -invariant . is not ‘disturbed’ at all by our generalised field measurements in 𝐀 𝐀 \mathbf{A} bold_A . Furthermore, the ⊗ 𝐀 subscript tensor-product 𝐀 \otimes_{\mathbf{A}} ⊗ start_POSTSUBSCRIPT bold_A end_POSTSUBSCRIPT -functorial ADG-gravitational field dynamics does not break down in any differential geometrical sense in the presence of any type of singularity that may be encoded in the structure sheaf 𝐀 𝐀 \mathbf{A} bold_A .
The pair of observations above, namely that:
The ADG-gravitational dynamics is unperturbed by our generalised measurements in 𝐀 𝐀 \mathbf{A} bold_A ; and,
The ADG-gravitational dynamics does not break down in any (differential geometric) sense by any kind of ‘singularities’ or ‘anomalies’ present in 𝐀 𝐀 \mathbf{A} bold_A ,
reflect what we have elsewhere called the Principle of ADG-Field Realism [ 78 , 79 , 80 ] . Which brings us to the last section.
7 Gauge Field Theory of the Third Kind and its Third Quantisation
The last philosophical issue of ADG-gravity that we would like to discuss in this paper is two-fold:
∙ ∙ \bullet ∙ Gauge Field Theory of the Third Kind. We discussed earlier how from an ADG-theoretic perspective gravity is regarded as a gauge theory . We noted that the ADG-formalism may be coined half-order formalism , to distinguish it from the original second-order formalism of Einstein, whereby the dynamical variable is the smooth Riemannan spacetime metric g μ ν subscript 𝑔 𝜇 𝜈 g_{\mu\nu} italic_g start_POSTSUBSCRIPT italic_μ italic_ν end_POSTSUBSCRIPT , or from the more recent first-order formalism of Ashtekar, whereby the gravitational dynamical variables are the spin-connection 𝒜 μ subscript 𝒜 𝜇 \mathcal{A}_{\mu} caligraphic_A start_POSTSUBSCRIPT italic_μ end_POSTSUBSCRIPT and the vierbein frame e μ subscript 𝑒 𝜇 e_{\mu} italic_e start_POSTSUBSCRIPT italic_μ end_POSTSUBSCRIPT . In ADG-gravity, the sole dynamical variable is the Einstein (connection) field ℱ E i n s t = ( ℰ , 𝒟 ) subscript ℱ 𝐸 𝑖 𝑛 𝑠 𝑡 ℰ 𝒟 \mathcal{F}_{Einst}=(\mathbf{\mathcal{E}},\mathcal{D}) caligraphic_F start_POSTSUBSCRIPT italic_E italic_i italic_n italic_s italic_t end_POSTSUBSCRIPT = ( caligraphic_E , caligraphic_D ) .
As such, the ADG-based gauge-theoretic formulation of gravity, without recourse to any background spacetime manifold, has been called pure gauge field autodynamics [ 64 , 78 , 79 , 80 ] .
The second denomination gauge theory of the third kind comes from the observation that the first U ( 1 ) 𝑈 1 U(1) italic_U ( 1 ) gauge (or scale) theory for the electromagnetic and the gravitational field due to Weyl [ 103 ] was a global gauge theory , 29 29 29 In the sense that Weyl showed that non-spacetime localised (global) gauge/scale invariance implies the conservation of electric charge in much the same way that general coordinate invariance leads to the conservation of energy and momentum in gravitational dynamics. while the gauge theories underlying the three fundamental forces (other than gravity) of the Standard Model 30 30 30 That is, the electromagnetic (with local gauge group U ( 1 ) 𝑈 1 U(1) italic_U ( 1 ) ), the weak nuclear (with local gauge group S U ( 2 ) 𝑆 𝑈 2 SU(2) italic_S italic_U ( 2 ) ) and the strong nuclear (with local gauge group S U ( 3 ) 𝑆 𝑈 3 SU(3) italic_S italic_U ( 3 ) ) forces. are flat Minkowski space localised gauge theories [ 24 ] .
By contrast, aside from its half-order formalism, our ADG-based gauge-theoretic formulation of gravity (:ADG-gravity), although local by its sheaf-theoretic character, is not background spacetime localised, since there is no background spacetime manifold to localise and solder it on to begin with .(E1,M1, F1,E2,M2)
∙ ∙ \bullet ∙ Third Quantisation. In [ 78 , 79 ] , and recently in [ 80 ] , a third canonical type of ADG-field quantisation scenario was proposed according to which certain local, sheaf cohomological characteristic forms for both the vector sheaf part ℰ ℰ \mathbf{\mathcal{E}} caligraphic_E and the connection part 𝒟 𝒟 \mathcal{D} caligraphic_D of the ADG-theoretic vacuum Einstein field ℱ E i n s t = ( ℰ , 𝒟 ) subscript ℱ 𝐸 𝑖 𝑛 𝑠 𝑡 ℰ 𝒟 \mathcal{F}_{Einst}=(\mathbf{\mathcal{E}},\mathcal{D}) caligraphic_F start_POSTSUBSCRIPT italic_E italic_i italic_n italic_s italic_t end_POSTSUBSCRIPT = ( caligraphic_E , caligraphic_D ) were seen to obey canonical type of Heisenberg non-commutation relations, albeit, explicitly not parametrised by a background spacetime manifold. 31 31 31 In the sense that they are not equal-time commutation relations which, in the usual canonical QG scenario, would have been required to obey some global hyperbolicity type of foliation of the background spacetime manifold into time-parametrised 3-dimensional spacelike hypersurfaces. This is to be expected as our ADG-gravity does not depend at all on an external (background) spacetime manifold, as well as to be desired, as our third quantisation scenario would be expected to ‘algebraically close’ within the autonomous and ‘solipsistic’ ADG-theoretic Einstein field ℱ E i n s t = ( ℰ , 𝒟 ) subscript ℱ 𝐸 𝑖 𝑛 𝑠 𝑡 ℰ 𝒟 \mathcal{F}_{Einst}=(\mathbf{\mathcal{E}},\mathcal{D}) caligraphic_F start_POSTSUBSCRIPT italic_E italic_i italic_n italic_s italic_t end_POSTSUBSCRIPT = ( caligraphic_E , caligraphic_D ) .
We distill these remarks to the following eighth Aphorism:
Aphorism 8. There are no external geometrical structures, such as a background spacetime manifold, in our theory: all there is is ℱ E i n s t = ( ℰ , 𝒟 ) subscript ℱ E i n s t ℰ 𝒟 \mathcal{F}_{Einst}=(\mathbf{\mathcal{E}},\mathcal{D}) caligraphic_F start_POSTSUBSCRIPT italic_E italic_i italic_n italic_s italic_t end_POSTSUBSCRIPT = ( caligraphic_E , caligraphic_D ) and its Yang-Mills counterparts ℱ Y M = ( ℰ , 𝒟 ) subscript ℱ Y M ℰ 𝒟 \mathcal{F}_{YM}=(\mathbf{\mathcal{E}},\mathcal{D}) caligraphic_F start_POSTSUBSCRIPT italic_Y italic_M end_POSTSUBSCRIPT = ( caligraphic_E , caligraphic_D ) ; hence, if the autonomous (:autodynamical) ADG-fields are to be quantum (or quantised) in any way, they should be quantum (or quantised) from within themselves, not from without [ 78 , 79 , 80 ] .(E1,M1,F1,E2,M2)
8 Brief Philosophical Musings on the Future of ADG-Gravity
This author’s current research on ADG-gravity focuses on the following three fronts:
To organise the recently discovered ‘ time-asymmetric algebras ’ in [ 81 ] 32 32 32 These algebras originally appeared, in primitive form, in this author’s Ph.D. thesis [ 72 ] , in which the early seeds for a time-asymmetric quantum spacetime structure and gravity were planted. into vector sheaves à-la ADG and, by employing the rich differential geometric mechanism of ADG, explore the possibility of developing a time-asymmetric Dirac equation on the resulting sheaves, possibly with ADG-gravity coupled to it [ 83 ] .(F2)
The project above dovetails snugly with our current musings in [ 82 ] , where we apply ADG to develop a time-asymmetric version of the vacuum Einstein equations for a finitary spin-Lorentzian gravitational connection [ 73 , 74 , 62 , 63 , 64 ] on Finkelstein’s quantum net as originally worked out by Steve Selesnick [ 86 , 87 , 88 , 89 , 90 ] , and relate this asymmetry to the fundamental asymmetry that Penrose has for many years anticipated that the true QG theory should account for [ 41 ] .(F2)
The main philosophical query that will arise from the three papers above [ 81 , 82 , 83 ] is that the anticipated fundamental time-asymmetry of the true QG theory may not only be traced back to time-asymmetric initial conditions for the Universe, 33 33 33 Like Penrose’s Weyl curvature hypothesis . but also it may be due to the fundamentally time-asymmetric quantum gravitational dynamics themselves (:time-asymmetric vacuum Einstein equations for ADG-gravity).(F2)
The quest continues…
Acknowledgments
I am greatly indebted to Professors Goro Kato (Department of Mathematics, California Polytechnic Institute, San Luis Obispo) and Steve Selesnick (Department of Mathematics, University of St Louis, Missouri) for numerous stimulating exchanges on a plethora of topics in Mathematics, Physics, Philosophy and Poetry after a long hiatus period of personal reflection and research course re-evaluation and re-adjustment.
< . > < . > < . > < . > < . > < . > < . > < . > < . > <.><.><.><.><.><.><.><.><.> < . > < . > < . > < . > < . > < . > < . > < . > < . >
The present paper is lovingly dedicated to my parents, George and Helen Raptis , whose unceasing moral and material support of my research quests has never been diminished by the passage of time, no matter what its toll on both their ageing bodies and their lucid minds.
Last but not least, the unceasing ‘moral’ support of my lovely family: Kathleen, Francis, James and Cookie , is also warmly aknowledged, especially their patience and understanding in putting up with me over the years.
- [1] Aristotle, The Nicomachean Ethics , Oxford World’s Classics, Oxford University Press (2009).
- [2] Ashtekar, A., New Variables for Classical and Quantum Gravity , Physical Review Letters, 57, 2244 (1986).
- [3] Ashtekar, A., Quantum Gravity: What and Why? , in Asymptotic Quantization , a volume based on the author’s 1984 Naples Lectures, Monographs and Textbooks in Physical Science, Lecture Notes 2, Bibliopolis, Naples (1987).
- [4] Ashtekar, A., Quantum Gravity: A Mathematical Physics Perspective , pre-print (1994); hep-th/9404019.
- [5] Auyang, S. Y., How is Quantum Field Theory Possible? , Oxford University Press, New York-Oxford (1995).
- [6] Bombelli, L., Lee, J., Meyer, D. and Sorkin, R. D., Space-Time as a Causal Set , Physical Review Letters, 59, 521 (1987).
- [7] Bredon, G. E., Sheaf Theory , McGraw-Hill, New York (1967).
- [8] Brown, R. C., The Tangled Origins of the Leibnizian Calculus: A Case Study of a Mathematical Revolution , World Scientific (2012).
- [9] Butterfield, J., Hamilton, J. and Isham, C. J., A Topos Perspective on the Kochen-Specker Theorem: III. Von Neumann Algebras as the Base Category , International Journal of Theoretical Physics, 39, 2667 (2000).
- [10] Butterfield, J. and Isham, C. J., A Topos Perspective on the Kochen-Specker Theorem: I. Quantum States as Generalized Valuations , International Journal of Theoretical Physics, 37, 2669 (1998).
- [11] Butterfield, J. and Isham, C. J., A Topos Perspective on the Kochen-Specker Theorem: II. Conceptual Aspects and Classical Analogues , International Journal of Theoretical Physics, 38, 827 (1999).
- [12] Butterfield, J. and Isham, C. J., Some Possible Roles for Topos Theory in Quantum Theory and Quantum Gravity , Foundations of Physics, 30, 1707 (2000).
- [13] Clark, P., Philosophy of Science Today , Clarendon Press, Oxford (2003).
- [14] Dirac, P. A. M., Quantized Singularities in the Electromagnetic Field , Proceedings of the Royal Society London A , 133, 60 (1931).
- [15] Edwards, C. H. Jr, The Historical Development of the Calculus , Springer-Verlag, Berlin-Heidelberg-New York (1982).
- [16] Einstein, A., Albert Einstein: Philosopher-Scientist , The Library of Living Philosophers, 7, Schilpp, P. A. (Ed.), Evanston, III (1949).
- [17] Einstein, A., The Meaning of Relativity , 5th edition, Princeton University Press, Princeton (1956).
- [18] Feynman, R. P., The Character of Physical Law , Penguin Books, London (1992).
- [19] Feynman, R. P., Feynman Lectures on Gravitation , notes by Morinigo, F. B. and Wagner, W. G., Hatfield, B. (Ed.), Penguin Books, London (1999).
- [20] Finkelstein, D., Theory of Vacuum in The Philosophy of Vacuum , Saunders, S. and Brown, H. (Eds.), Clarendon Press, Oxford (1991).
- [21] Finkelstein, D. R., Quantum Relativity: A Synthesis of the Ideas of Einstein and Heisenberg , Springer-Verlag, Berlin-Heidelberg-New York (1996).
- [22] Geroch, R., What is a singularity in General Relativity? , Annals of Physics, 48, 526 (1968).
- [23] Geroch, R., Local Characterization of Singularities in General Relativity , Journal of Mathematical Physics, 9, 450 (1968).
- [24] Göckeler, M. and Schücker, T., Differential Geometry, Gauge Theories and Gravity , Cambridge University Press, Cambridge (1990).
- [25] Green, M. B., Schwartz J. H. and Witten, E., Superstring Theory: Introduction (Volume 1) , Cambridge University Press (2012). 34 34 34 Online publication of the original book.
- [26] Haag, R., Local Quantum Physics: fields, particles, algebras , 2nd edition, Springer-Verlag, Berlin-Heidelberg-New York (1996).
- [27] Hawking, S. W., Singularities in the universe , Physical Review Letters, 17, 444 (1966).
- [28] Hawking, S. W., Breakdown of predictability in gravitational collapse , Physical Review, D14, 2460 (1976).
- [29] Hawking, S. W. and Ellis, G. F. R., The Large Scale Structure of Space-Time , Cambridge University Press, Cambridge (1973).
- [30] Hawking, S. W. and Penrose, R., The Singularities of Gravitational Collapse and Cosmology , Proceedings of the Royal Society London A, 314, 529 (1970).
- [31] Isham, C. J., Canonical groups and the quantization of geometry and topology , in Conceptual Problems of Quantum Gravity , Ashtekar, A. and Stachel, J. (Eds.), Birkhäuser, Basel (1991).
- [32] Isham, C. J., Canonical Quantum Gravity and the Problem of Time , in Integrable Systems, Quantum Groups, and Quantum Field Theories , Kluwer Academic Publishers, London-Amsterdam (1993); gr-qc/9210011.
- [33] Isham, C. J., Prima Facie Questions in Quantum Gravity , pre-print (1993); gr-qc/9310031.
- [34] Isham, C. J., Structural Issues in Quantum Gravity , Plenary Session Lecture given at the GR14 Conference (Florence, Italy), pre-print (1995); gr-qc/9510063.
- [35] Isham, C. J., Topos Theory and Consistent Histories: The Internal Logic of the Set of All Consistent Sets , International Journal of Theoretical Physics, 36, 785 (1997).
- [36] Isham, C. J., Some Reflections on the Status of Conventional Quantum Theory when Applied to Quantum Gravity , in The Future of Theoretical Physics and Cosmology: Celebrating Stephen Hawking’s 60th Birthday , Gibbons, G. W., Shellard, E. P. S. and Rankin, S. J. (Eds.), Cambridge University Press, Cambridge (2003); quant-ph/0206090.
- [37] Isham, C. J., A new approach to quantising space-time: I. Quantising on a general category , Advances in Theoretical and Mathematical Physics, 7, 331 (2003); gr-qc/0303060.
- [38] Isham, C. J., A new approach to quantising space-time: II. Quantising on a category of sets , Advances in Theoretical and Mathematical Physics, 7, 807 (2004); gr-qc/0304077.
- [39] Isham, C. J., A new approach to quantising space-time: III. State vectors as functions on arrows , Imperial College pre-print TP/2-03/15 (2003); gr-qc/0306064.
- [40] Isham, C. J., Quantising on a category , to appear in A Festschrift for James Cushing (2005); quant-ph/0401175.
- [41] Jacobs, T. and Maes, C., Reversibility and Irreversibility within the Quantum Formalism , pre-print (2005); quant-ph/0508041.
- [42] Kiefer, K., On a Quantum Weyl Curvature Hypothesis , pre-print (2022); gr-qc/2111.02137v2.
- [43] Leibniz, G. W., Discourse on Metaphysics and the Monadology , translated by Montgomery, G. R., Great Books in Philosophy Series, Prometheus Books, Amherst, New York (1992).
- [44] Lu, L. and May, P. A., Step-by-Step Canonical Quantum Gravity: Part I: Ashtekar’s New Variables , pre-print (2024); gr-qc/2401.06863v1.
- [45] MacLane, S., Categories for the Working Mathematician , Graduate Texts in Mathematics Series, Springer-Verlag, New York (1971).
- [46] MacLane, S. and Moerdijk, I., Sheaves in Geometry and Logic: A First Introduction to Topos Theory , Springer-Verlag, New York (1992).
- [47] Mallios, A., Geometry of Vector Sheaves: An Axiomatic Approach to Differential Geometry , vols. 1-2, Kluwer Academic Publishers, Dordrecht (1998). 35 35 35 There is also a Russian translation of this 2-volume book by MIR Publishers, Moscow (vol. 1, 2000 and vol. 2, 2001).
- [48] Mallios, A., On an Axiomatic Treatment of Differential Geometry via Vector Sheaves. Applications , Math. Jap. (International Plaza), 48, 93 (1998). (invited paper)
- [49] Mallios, A., On an axiomatic approach to geometric prequantization: A classification scheme à la Kostant-Souriau-Kirillov , J. Math. Sci. (NY), 95, 2648 (1999). (invited paper)
- [50] Mallios, A., Abstract Differential Geometry, General Relativity and Singularities , in Unsolved Problems in Mathematics for the 21st Century: A Tribute to Kiyoshi Iséki’s 80th Birthday , Abe, J. M. and Tanaka, S. (Eds.), 77, IOS Press, Amsterdam (2001). (invited paper)
- [51] Mallios, A., Remarks on “singularities” (2002) (pre-print); gr-qc/0202028.
- [52] Mallios, A., On Localizing Topological Algebras , in Topological Algebras and Their Applications , Arizmendi, H., Bosch, C., and Palacios, L. (Eds), Contemporary Mathematics, AMS, 341 (2004) (pre-print); gr-qc/0211032.
- [53] Mallios, A., Modern Differential Geometry in Gauge Theories. Vol.I: Maxwell Fields, Vol.II: Yang-Mills Fields , 2-volume continuation (including abstract integration theory) of [ 47 ] , Birkhäuser, Boston-Basel-Berlin (Vol. I 2005, Vol. II 2006).
- [54] Mallios, A., K-Theory of topological algebras and second quantization , Acta Universitatis Ouluensis–Scientiae Rerum Naturalium, A408, 145 (2004); math-ph/0207035.
- [55] Mallios, A., Abstract Differential Geometry, Singularities and Physical Applications , in Topological Algebras with Applications to Differential Geometry and Mathematical Physics , in Proceedings of a Fest-Colloquium in Honour of Professor Anastasios Mallios (16–18/9/1999) , Strantzalos, P. and Fragoulopoulou, M. (Eds.), Department of Mathematics, University of Athens Publications (2002).
- [56] Mallios, A., Quantum gravity and “singularities” , Note Mat., 25, 57 (2006) (invited paper); physics/0405111.
- [57] Mallios, A., Geometry and physics today , in a Special Proceedings issue for Glafka–2004: Iconoclastic Approaches to Quantum Gravity , Raptis, I. (Ed.), Int. J. Theor. Phys. 45, 1552 (2006) (invited paper); physics/0405112.
- [58] Mallios, A., 𝒜 𝒜 \mathcal{A} caligraphic_A -Invariance: An axiomatic approach to quantum relativity , Int. J. Theor. Phys., 47, 1929 (2008).
- [59] Mallios, A., Relational mathematics: A response to quantum gravity , Publications Ecole Norm. Supér., Takaddoum, Rabat, Morocco 2007, pp. 61-68 (2010).
- [60] Mallios, A., On Utiyama’s Theme Through “ 𝒜 𝒜 \mathcal{A} caligraphic_A -Invariance” , Complex Analysis and Operator Theory, 6, 775 (2012).
- [61] Mallios, A., Bohr’s correspondence principle (:the commutative substance of the quantum), abstract (:axiomatic) quantum gravity, and functor categories , Manuscript/pre-print (2012).
- [62] Mallios, A. and Raptis, I., Finitary Spacetime Sheaves of Quantum Causal Sets: Curving Quantum Causality , Int. J. Theor. Phys., 40, 1885 (2001); gr-qc/0102097.
- [63] Mallios, A. and Raptis, I., Finitary Čech-de Rham Cohomology , Int. J. Theor. Phys., 41, 1857 (2002); gr-qc/0110033.
- [64] Mallios, A. and Raptis, I., Finitary, Causal and Quantal Vacuum Einstein Gravity , Int. J. Theor. Phys., 42, 1479 (2003); gr-qc/0209048.
- [65] Mallios, A. and Raptis, I., 𝒞 ∞ superscript 𝒞 \mathcal{C}^{\infty} caligraphic_C start_POSTSUPERSCRIPT ∞ end_POSTSUPERSCRIPT -Smooth Singularities Exposed: Chimeras of the Differential Spacetime Manifold , research monograph (2005) (in preparation); gr-qc/0411121. 36 36 36 Two years’ old version posted at gr-qc.
- [66] Mallios, A. and Rosinger, E. E., Abstract Differential Geometry, Differential Algebras of Generalized Functions and de Rham Cohomology , Acta Appl. Math., 55, 231 (1999).
- [67] Mallios, A. and Rosinger, E. E., Space-Time Foam Dense Singularities and de Rham Cohomology , Acta Appl. Math., 67, 59 (2001).
- [68] Mallios, A. and Rosinger, E. E., Dense Singularities and de Rham Cohomology , in Topological Algebras with Applications to Differential Geometry and Mathematical Physics , in Proceedings of a Fest-Colloquium in Honour of Professor Anastasios Mallios (16–18/9/1999) , Strantzalos, P. and Fragoulopoulou, M. (Eds.), Department of Mathematics, University of Athens Publications (2002).
- [69] Mallios, A. and Zafiris, E., Topos-theoretic Relativization of Physical Representability and Quantum Gravity , pre-print (2007); gr-qc/0610113.
- [70] Mallios, A. and Zafiris, E., Differential Sheaves and Connections: A Natural Approach to Physical Geometry , Series on Concrete and Applicable Mathematics, Vol. 18, World Scientific (2015).
- [71] Misner, C. W., Thorne, K. and Wheeler, J. A., Gravitation , Freeman Publishers, San Francisco (1973).
- [72] Raptis, I., Axiomatic Quantum Timespace Structure: A Preamble to the Quantum Topos Conception of the (Minkowski) Vacuum , Theoretical Physics Group, Physics Department, The University of Newcastle upon Tyne, United Kingdom (1998).
- [73] Raptis, I., Algebraic Quantization of Causal Sets , Int. J. Theor. Phys., 39, 1233 (2000); gr-qc/9906103.
- [74] Raptis, I., Finitary Spacetime Sheaves , Int. J. Theor. Phys., 39, 1703 (2000); gr-qc/0102108.
- [75] Raptis, I., Finitary-Algebraic ‘Resolution’ of the Inner Schwarzschild Singularity , Int. J. Theor. Phys., 45, (6) (2006) (to appear); gr-qc/0408045.
- [76] Raptis, I., Finitary Topos for Locally Finite, Causal and Quantal Vacuum Einstein Gravity , Int. J. Theor. Phys., 46, 688 (2007); gr-qc/0507100.
- [77] Raptis, I., ‘Iconoclastic’ Categorical Quantum Gravity , published in a Special Proceedings issue for Glafka–2004: Iconoclastic Approaches to Quantum Gravity , Raptis, I. (Ed.), Int. J. Theor. Phys., 45, 1495 (2006); gr-qc/0509089.
- [78] Raptis, I., ‘Third’ Quantization of Vacuum Einstein Gravity and Free Yang-Mills Theories , Int. J. Theor. Phys., 46, 1137 (2007); gr-qc/0606021.
- [79] Raptis, I., A Dodecalogue of Basic Didactics from Applications of Abstract Differential Geometry to Quantum Gravity , Int. J. Theor. Phys., 46, 3009 (2007); gr-qc/0607038.
- [80] Raptis, I., Functoriality in Finitary Vacuum Einstein Gravity and Free Yang-Mills Theories from an Abstract Differential Geometric Perspective , invited paper contribution to a Special Memorial Volume titled Physical Geometry: Unravelling the Weave of Quantum Geometry in memory of Professor Anastasios Mallios, edited by Dr Elias Zafiris (Editor-in-Chief), Technical University of Vienna Press (September 2024); gr-qc/2401.13283v3 (vol. 3).
- [81] Raptis, I., Multiplicatively Ordered and Directed Hybrid δ 𝛿 \delta italic_δ -Jordan-Lie Superalgebra , paper submitted to the Journal of Algebra and its Applications (2024); math-ph/2405.01181v3 (vol. 3).
- [82] Raptis, I., “ Gravity from Light ”. Gravity and Electrodynamics Viewed in the Purely Gauge-Theoretic Light of Abstract Differential Geometry: Tracing the sub-Planckian Origins of Inertial Mass , pre-print in preparation (August-October 2024); gr-qc/??? (to be posted duly at the e-archives).
- [83] Raptis, I., Three Potential Physical Applications of a Hybrid δ 𝛿 \delta italic_δ -Jordan-Lie Superalgebra: Extended Supersymmetry, Time-Irreversible Free Generative Linear Semigroups, and the Time-Asymmetric Dirac Equation , pre-print in preparation (August-October 2024); gr-qc/??? (to be posted duly at the e-archives).
- [84] Raptis, I. and Zapatrin, R. R., Quantization of discretized spacetimes and the correspondence principle , Int. J. Theor. Phys., 39, 1 (2000); gr-qc/9904079.
- [85] Raptis, I. and Zapatrin, R. R., Algebraic description of spacetime foam , Class. Quant. Grav., 20, 4187 (2001); gr-qc/0102048.
- [86] Selesnick, S. A, Second quantization, projective modules and local gauge invariance , Int. J. Theor. Phys., 22, 29 (1983).
- [87] Selesnick, S. A., Gauge fields on the quantum net , Journal of Mathematical Physics, 36, 5465 (1995).
- [88] Selesnick, S. A., Quanta, Logic and Spacetime , World Scientific (2003).
- [89] Selesnick, S. A., Computing the Lagrangians of the Standard Model II. The Ghost Term , Int. J. Theor. Phys., 55, 4999 (2016).
- [90] Selesnick, S. A., Emergent geometry, emergent forces , Class. Quant. Grav., 34, 195010 (2017).
- [91] Sen A. and Zwiebach B., String Field Theory: A Review , pre-print (2024); gr-qc/2405.19421v2.
- [92] Sklar, L., The Philosophy of Science : A Collection of Essays , Sklar, L. (Ed.), Vols. 1–5, Garland Publishers, New York and London (2000).
- [93] Sorkin, R. D., Does a Discrete Order Underlie Spacetime and its Metric? in Proceedings of the Third Canadian Conference on General Relativity and Relativistic Astrophysics , Cooperstock, F. and Tupper, B. (Eds.), World Scientific, Singapore (1990).
- [94] Sorkin, R. D., A Specimen of Theory Construction from Quantum Gravity , in The Creation of Ideas in Physics , Leplin, J. (Ed.), Kluwer Academic Publishers, Dordrecht (1995); gr-qc/9511063.
- [95] Sorkin, R. D., Forks in the Road, on the Way to Quantum Gravity , International Journal of Theoretical Physics, 36, 2759 (1997); gr-qc/9706002.
- [96] Stachel, J. J., The Other Einstein: Einstein Contra Field Theory , in Einstein in Context , Beller, M., Cohen, R. S. and Renn, J. (Eds.), Cambridge University Press, Cambridge (1993).
- [97] Thiemann, T., Introduction to Modern Canonical Quantum General Relativity , pre-print (2001); gr-qc/0110034.
- [98] ’t Hooft, G., Obstacles on the Way Towards the Quantization of Space, Time and Matter , ITP-University of Utrecht, pre-print SPIN-2000/20 (2001).
- [99] Vassiliou, E., On Mallios’ 𝒜 𝒜 \mathcal{A} caligraphic_A -connections as connections on principal sheaves , Note di Matematica, 14, 237 (1994).
- [100] Vassiliou, E., Connections on principal sheaves , in New Developments in Differential Geometry , Szenthe, J. (Ed.), Kluwer Academic Publishers, Dordrecht (1999).
- [101] Vassiliou, E., On the geometry of associated sheaves , Bulletin of the Greek Mathematical Society, 44, 157 (2000).
- [102] Vassiliou, E., Geometry of Principal Sheaves , Mathematics and Its Applications, Vol. 578, Springer (2005).
- [103] Weyl, H., Gravitation and Electricity , in The Principle of Relativity , Dover Publications, New York (1952).
- [104] Wheeler, J. A., Singularity and Unanimity , General Relativity and Gravitation, 8, 713 (1977).
- [105] Zafiris, E., Quantum observables algebras and abstract differential geometry , Int. J. Theor. Phys., bf 46, 319 (2007).
- [106] Zafiris, E., Physical Principles of Functorial Gauge Localization and Dynamics. With a View Toward Quantum General Relativity. , Monograph in preparation (2012).
- [107] Zapatrin, R. R., Finitary Algebraic Superspace , International Journal of Theoretical Physics, 37, 799 (1998).
- [108] Zapatrin, R. R., Incidence algebras of simplicial complexes , Pure Mathematics and its Applications (2002) (to appear); math.CO/0001065.
- [109] Zapatrin, R. R., Continuous limits of discrete differential manifolds , pre-print (2001). 37 37 37 This pre-print can be retrieved from Roman Zapatrin’s personal webpage, at: www.isiosf.isi.it/ ∼ zapatrin .

IMAGES
COMMENTS
the general problem solving theory. AI is the study and devel-opment of computer programs to solve problems. An AI program uses a finite set of functions to work from the prob-lem state to the solution state. Many simple puzzles and problems in logic were successfully completed with AI pro-grams, supporting the idea that problem-solving success
The General Problem Solver (GPS) was a theory of human problem solving stated in the form of a simulation program (Ernst & Newell, 1969; Newell & Simon, 1972). This program and the associated theoretical framework had a significant impact on the subsequent direction of cognitive psychology. It also introduced the use of productions as a method ...
General Problem Solver ( GPS) is a computer program created in 1957 by Herbert A. Simon, J. C. Shaw, and Allen Newell ( RAND Corporation) intended to work as a universal problem solver machine. In contrast to the former Logic Theorist project, the GPS works with means-ends analysis. [ 1]
ter on problem solving, however they prescribe only general problem-solving strategies as solu-tions. Gagne, Briggs, and Wager (1992) acknowl-edged that problem-solving learning is difficult and suggest only a brief template for applying the events of instruction in the same way they treat concept-learning and rule-learning out-comes.
In general, problems are regularly present, ... The research also looks at three theories: engagement theory, problem-solving theory, and narrative-centered learning theory. These ideas are ...
This chapter provides a revised review of the psychological literature on reasoning and problem solving. Four classes of deductive reasoning are presented, including rule (mental logic) theories, semantic (mental model) theories, evolutionary theories, and heuristic theories. Major developments in the study of reasoning are also presented such ...
The current bibliography first outlines some general resources on the psychology of problem solving and decision making before examining each of these topics in detail. Specifically, this review covers cognitive, neuroscientific, and computational approaches to problem solving, as well as decision making models and cognitive heuristics and biases.
The nature of human problem solving has been studied by psychologists for the past hundred years. Early conceptual work of German Gestalt psychologists (e.g., Duncker, 1935; Wertheimer, 1959) and experimental research on problem solving in the 1960s and 1970s typically operated with relatively simple, laboratory tasks (e.g., Duncker's famous "X-ray" problem; Ewert and Lambert's 1932 ...
The problem solving is a personal and aimed process. That means that the activities done by an individual during the problem solving process are led to his/her personal aim (Mayer and Wittrock, 2006). An individual has to identify the problem first and then seek for possible solutions (Mayer and Wittrock, 2006).
problem solving proposed in the preceding chapters. Janet E. Davidson is Associate Professor of Psychology at Lewis & Clark College. She conducts research on several aspects of problem solving, including the roles that insight and metacognitive skills play in problem solving. Robert J. Sternberg is IBM Professor of Psychology and Education at
A heuristic is another type of problem solving strategy. While an algorithm must be followed exactly to produce a correct result, a heuristic is a general problem-solving framework (Tversky & Kahneman, 1974). You can think of these as mental shortcuts that are used to solve problems. A "rule of thumb" is an example of a heuristic.
Problem-solving is a vital skill for coping with various challenges in life. This webpage explains the different strategies and obstacles that can affect how you solve problems, and offers tips on how to improve your problem-solving skills. Learn how to identify, analyze, and overcome problems with Verywell Mind.
Abstract. This chapter follows the historical development of research on problem solving. It begins with a description of two research traditions that addressed different aspects of the problem-solving process: (1) research on problem representation (the Gestalt legacy) that examined how people understand the problem at hand, and (2) research on search in a problem space (the legacy of Newell ...
Problem Solving as of 1985 - a retrospective view . In theoretical terms, what . MPS . offered in 1985 was a . framework. for the analysis of the success of failure problem solving attempts, in mathematics and hypothetically in all problem solving domains. "Problem solving" at its most general was defined as trying
Herbert Simon (1972). These authors provided a formal, computational theory for such problems called the General Problem Solver (GPS), and this theory was one of the first formal information processing theories to be developed in cognitive psychology. A move problem is posed to solvers in terms of a clearly defined representation consisting of a
Cognitive—Problem solving occurs within the problem solver's cognitive system and can only be inferred indirectly from the problem solver's behavior (including biological changes, introspections, and actions during problem solving).. Process—Problem solving involves mental computations in which some operation is applied to a mental representation, sometimes resulting in the creation of ...
In general, effective problem-solving strategies include the following steps: Define the problem. Come up with alternative solutions. Decide on a solution. Implement the solution. Problem-solving ...
The goal of research on problem solving is a general theory, that is, a set of pr inciples that . capture the essential properties of the cognitive processes by which human beings solve .
Theories of problem-solving are dominated by the work of Newell & Simon on GPS (General Problem Solver). This work established the information processing paradigm for the study of problem-solving and the concepts of "means-ends-analysis" and "problem space". According to the GPS framework, problem-solving involves the identification of subgoals and the use of methods (especially ...
Many theories have been proposed that attempt general problem solving, yet the final goal of achiev-ing human-level intelligence has been unsuccess-ful. Some authors have proposed guidelines and roadmaps for this search [17, 12]. One of the ear-liest theories that focus on general problem solving was proposed in [14] and focuses in decomposing re-
General Overview. The task-centred model is a problem-solving, empirically based, short-term practice model. It was developed by social work educators Bill Reid and Laura Epstein and was intended for practice with various client populations, including clients from historically oppressed, diverse backgrounds.An underlying premise of the task-centred model is that life circumstances inevitably ...
The term "problem" defines J. Linhart (1976, p. 385) as: . a) problem is an interactive relation between a subject and its surroundings, which incorporates the inner conflict that is solved by the subject by searching of transitions from initial condition to the final condition. b) the existence of a conflict causes the dynamics of an activity ...
General Overview. The task-centred model is a problem-solving, empirically based, short-term practice model. It was developed by social work educators Bill Reid and Laura Epstein (1972) and was intended for practice with various client populations, including clients from historically oppressed, diverse backgrounds.
Art of Problem Solving AoPS Online. Math texts, online classes, and more for students in grades 5-12. Visit AoPS Online ‚ Books for Grades 5-12 ...
The purpose of this transcendental phenomenological study was to describe the lived experiences of educators using different levels of support for teaching math to students with learning disabilities in math computation and problem-solving for teachers at public cyber charter high schools in the Northeastern United States. The theory guiding this study was Sweller's cognitive load theory, as ...
While there is a widespread belief that artificial general intelligence (AGI) -- or even superhuman AI -- is imminent, complex problems in expert domains are far from being solved. We argue that such problems require human-AI cooperation and that the current state of the art in generative AI is unable to play the role of a reliable partner due to a multitude of shortcomings, including ...
In general, researchers expect the appropriate number of intermediate steps for solving any problem to depend on the size of the input to the problem. For example, the simplest strategy for adding two 20-digit numbers requires twice as many intermediate addition steps as the same approach to adding two 10-digit numbers.
This work will introduce a channel conceptualization and possible coding scheme for Graph-and-Symbol (GS) Communication. While Claude Shannon's mathematical model for communication employed graphs to describe relationships and confusability among traditional time-sequenced signals, little work as been done to describe non-linear communication with graphs where we transmit and receive ...
In this sense, the GPD is stronger in the fracture problems. (2) Nonlocal microscopic two-point interaction: Compared to the traditional theories, GPD is derived based on the nonlocal vector calculus and the microscopic constitutive equation which presents a more general mathematical form and provides a considerable physical foundation. The ...
4.2 Sheaf Theory and the Transition from Local to Global; 5 Revisiting Feynman: Gravity as Gauge Theory. 5.1 Enter ADG: Gravity as Pure Gauge Theory of the Third Kind; 6 Field Solipsism and Functoriality: The Point of Spacetimelessness, Generalised Principle of General Covariance and a Different Perspective on the 'Measurement Problem'