ORIGINAL RESEARCH article
Floristic composition, life history traits and phytogeographic distribution of forest vegetation in the western himalaya.
- 1 Department of Ethnobotany, Institute of Botany, Ilia State University, Tbilisi, Georgia
- 2 Department of Botany, Glocal University Saharanpur, Saharanpur, India
- 3 Department of Botany, Government Degree College (Women), Kupwara, India
- 4 Department of Botany, University of Okara, Okara, Pakistan
- 5 Department of Botany, Institute of Life Sciences, State Museum of Natural History, Karlsruhe, Germany
- 6 Department of Food Industries, Faculty of Agriculture, Damietta University, Damietta, Egypt
- 7 Department of Plant Production, College of Food and Agriculture Sciences, King Saud University, Riyadh, Saudi Arabia
Introduction: Scientific documentation of the qualitative forest vegetation parameters of a biogeographical area provides baseline information to guide conservation strategies and design policies for biodiversity management regulations.
Methods: We present one of the most comprehensive qualitative vegetation analyses to evaluate the entire structure and function of an ecosystem in the remote northern part of the Kashmir Himalaya, India. Several multivariate ecological community analyses were conducted after determining the presence of plant species in the various habitats using a random sampling technique.
Results: In total, 155 plant species belonging to 120 genera and 49 families occurred in the area. Asteraceae was the largest family (12% of plant species) followed by Rosaceae (11%). The patterns of species distribution across families were uneven, with 50% of the species belonging to only 7 families, and 23 families being monotypic. In terms of functional groups, the herbaceous growth form dominated. Therophytes were the dominant life form, indicating that the vegetation was disturbed. According to the phytogeographical research, 65% of the species documented in the study area were native, 15% were invasive, 14% naturalized, and 5% being casual. The majority (30%) of exotic species were reported along roadsides. Of all the species found, 39% grew in their natural habitats, such as forests, and 11% were scattered along roadsides. Plant species were grouped in five different clusters based on their floristic similarity. According to the estimated diversity indices, natural forest has the greatest values for Shannon’s and Simpson’s index. We found that the study area serves as the natural habitat for several significant, endangered medicinal plants, including Arnebia benthamii, Bergenia ciliata, Delphinium roylei, Gentiana kurroo, Phytolacca acinosa, Saussurea costus , and Trillium govanianum . Therefore, we recommend that human intervention in natural regeneration efforts be prioritized in these habitats to increase the population of these species.
Conclusion: Examining species features from the perspective of functional groups contributes to our understanding of the ecological aspects of the flora. It may also be useful in developing management plans to ensure long-term management of forest landscapes in this remote Himalayan region.

Introduction
Floristic diversity is an important element of ecosystems ( Hua et al., 2022 ). This diversity includes the plant species diversity of a particular area representing the local flora of a given area ( Qian et al., 2021 ). Taxonomy is concerned with their identification and classification of species ( Barkley et al., 2004 ). Floristic and taxonomic studies provide efficient information about different aspects of an ecosystem, including nomenclature, distribution, ecology and utility of plant species of an area ( Grime et al., 2014 ; Haq et al., 2022d ). While information on plants of many regions has long been available online via checklists ( Safidkon et al., 2003 ), comprehensive floristic studies are still necessary to document the whole plant diversity of any geographic region ( Noss, 1983 ). Lack of such studies strongly limits the scientific inquiry into plants ( Kier et al., 2005 ), and is needed to ultimately understand how plant communities are structured and distributed.
Among the various ecological attributes of plant communities, floristic composition and ecological diversity are the most important ones that are influenced by a variety of biotic and abiotic factors ( Khan et al., 2018 ; Solefack et al., 2018 ). The understanding of linkages among plants diversity and its ecological functions is critical for understanding the adjustment of plant communities, and how they adopt toward specific habitats ( Rahman et al., 2019a ; Altaf et al., 2022 ). Lifeform and habit are the two important physiognomic features that are being widely used while investigating vegetation ( Haq et al., 2019 ). Studying functional plant traits of a given region is an important tool that helps in understanding the relationship between environmental variables and plant community structure and distribution, ultimately revealing the biological functions of individual species in a community ( Vakhlamova et al., 2016 ).
The Himalayan Mountain Range is a prominent biogeographic ecoregion that shows great variation in topography and climate, and thus harbors significant plant diversity ( Olson et al., 2001 ). This mountain ecosystem has been recognized as a global biodiversity hotspot due to its significant biodiversity and huge endemism ( Myers et al., 2000 ). The Kashmir Himalaya, part of Indian Himalayan Region, has been considered as a promising floristic region with high endemism ( Mahar et al., 2009 ). Nonetheless, many remote areas of this biodiversity-rich region have not received much attention as far as floristic and ecological investigations are concerned, which is especially the case of remote mountains region in Kashmir Himalayas. The capability and productivity of the Himalayan forest ecosystem have decreased as a result of numerous stressors, including anthropogenic factors (such as over-exploitation of forest resources and the spread of invasive species), environmental (climate, slope, soil), fire incidences, and declining biological diversity ( Haq et al., 2020 ). Floristic and ecological studies are crucial to advancing our understanding of the distribution and composition of plant communities in biodiversity hotspots. It is crucial and most effective to record qualitative forest vegetation parameters in order to preserve floristic diversity in the future and utilize biological resources sustainably. Keeping this in view, we focused on the following objectives: (i) to record the floristic composition of the vegetation in the region. (ii) What are the patterns of the emergent ecological traits groups in the flora in this region (iii) to find out the contribution of native and alien elements in the region vegetation and (iv) to find out the forest species pool shows habitat filtering in the region.
Given the ecological and economic significance of the forest vegetation under consideration, the findings of this study can guide sustainable biodiversity management and habitat restoration, particularly in invaded habitats in this Himalayan region, with global implications.
Materials and methods
Research area.
The study area one of the remotest Tehsil of District Bandipore, Jammu and Kashmir, India. The Gurez valley is situated in the high-altitude Kashmir Himalaya, about 86 kms from Bandipore and 146 kms from the regions summer capital (Srinagar) ( Figure 1 ). The Gurez valley is at an altitude of ranges from 2,400 to 3,500 masl and is mostly inhabited by Pahari and Gujjar tribes. The study area includes fifteen Panchayats (village administrative units) with about 30,000 inhabitants. Dawar is known as the main town in the area. Due to heavy snowfall (approx. 1.5–1.8 m), the area remains disconnected from the rest of the region for about 6 months a year, as Razdan Pass is completely closed in the winter. It has a dry and mild climate. The valley is vital for wildlife tourism because it is surrounded by high mountains and steep gorges that are drained by the Kishanganga River. It also supports a variety of vegetation, dense forests, and a wealth of species.
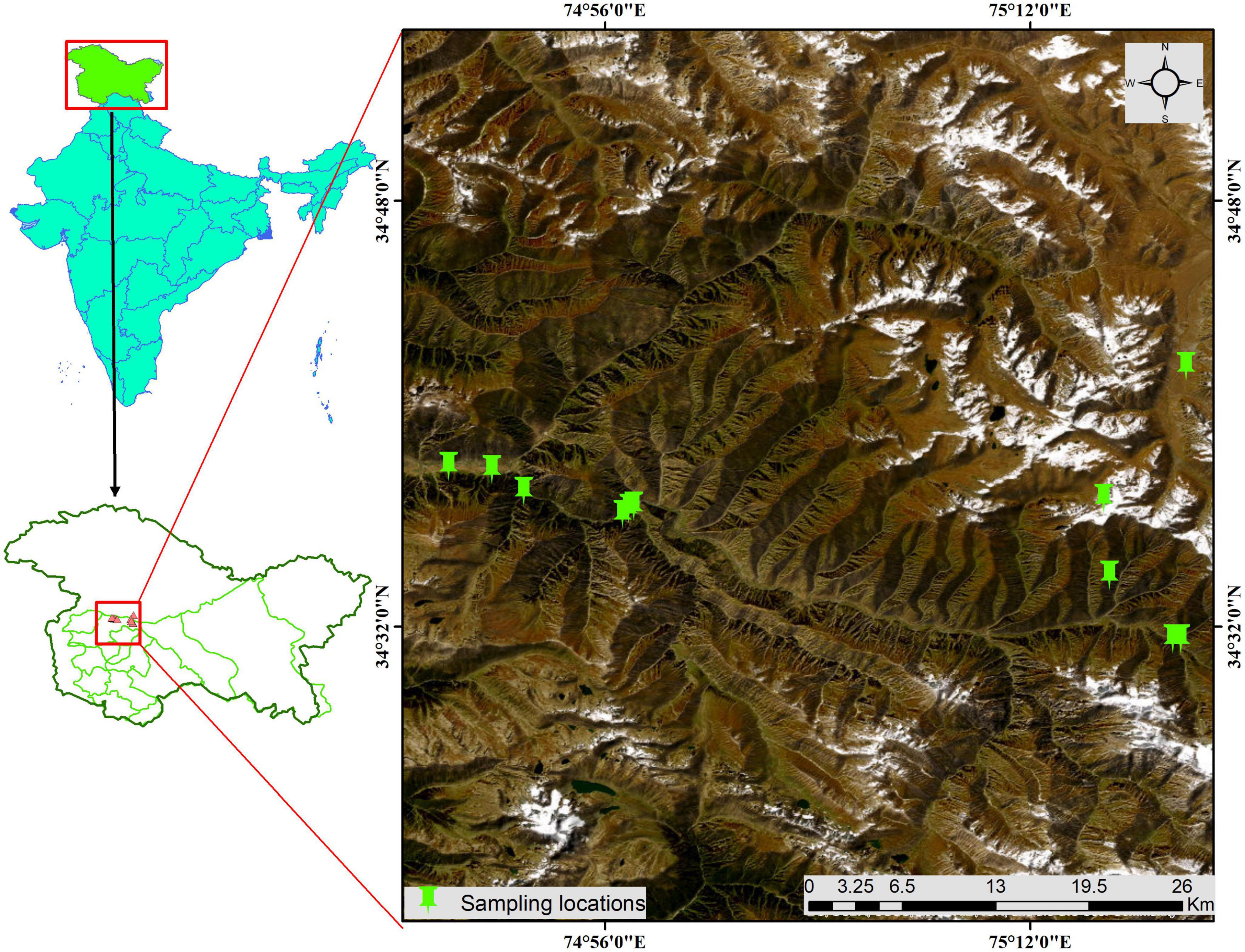
Figure 1. Map of study area (A) India (B) Jammu and Kashmir (C) Bandipora district and point showing the Gurez Valley in the Kashmir Himalaya, India (generated using ArcGIS version,10.5).
Field survey
Frequent surveys were conducted to obtain a better understanding of the research area and field data were collected during in various expeditions 2019–2021. Sites for the floristic studies were chosen using a random sampling technique to guarantee that plant species from various habitats had an equal chance of being sampled. The quadrat method was used to record floristic composition and functional characteristics to improve vegetation documentation ( Haq et al., 2020 ). We set up 36 0.1 ha plots for field sampling across different habitats in the study area. Within each 0.1 ha plot, two 5 m 2 plots were placed in opposite corners estimate shrub diversity. For the herbaceous layer, 5 plots of size 1 m 2 were laid (4 in each corner 0.1 ha plot and one in the center). In total, 32 plots for trees, 64 (2 plots × 32 = 64) plots for shrubs and 160 (5 plots × 32 = 160) plots for herbs were sampled in the present study. Field data about each specimen of plant material was meticulously recorded during field studies. In order to serve as herbarium voucher specimens, plant specimens were collected from the field, identified using taxonomic literature, and then authenticated by comparing the plant specimens with material in the KASH herbarium. Ecological traits (like Raunkiaer’s life-form, plant habit, phyto-geographical, life span) of each species were recorded during sampling, following Raunkiaer (1934) , and Pérez-Harguindeguy et al. (2016) . Furthermore, habitat characteristics of each plant species were categorized as Dry slope (DS), Moist places (MP), Natural forest (FR), Shady places (SP), Riparian vegetation (RV), Rock crevice (RC), Roadside (RS), Crop fields (CF), Vegetable garden (VG) ( Plate 1 ). The range of native phyto-geographically reported plant specimens was acquired by using secondary sources like manuals, Flora and specified internet web pages (GRIN: Germplasm Resource Information Network). 1 Based on the available data, all plant specimens were categorized into native and exotic species.
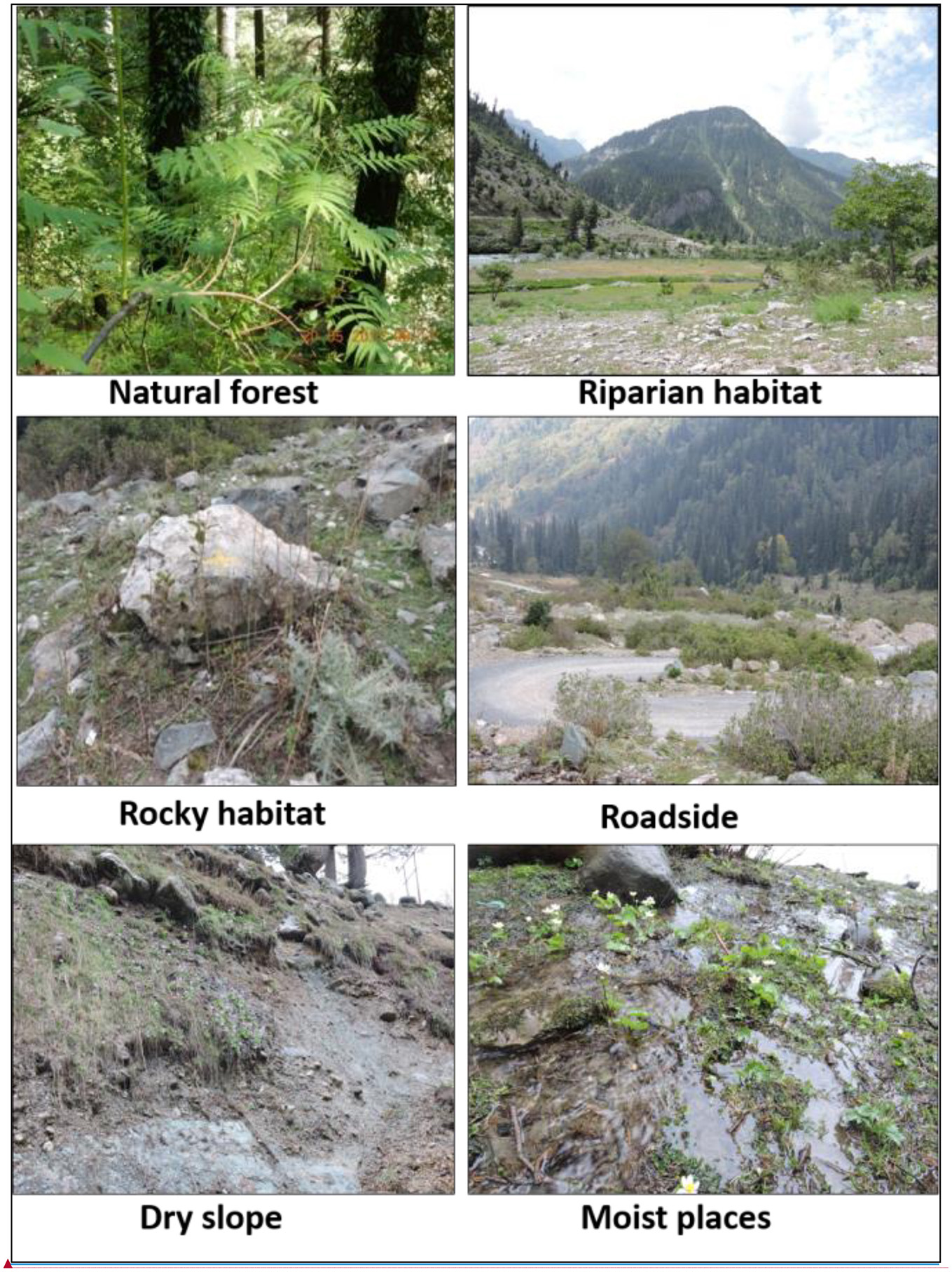
Plate 1. Representation of different habitats in the study area.
Data analysis
To investigate the relationships between ecological variables and plant compositions, heat map and clustering analysis were employed ( Rahman et al., 2019a ; Haq et al., 2021d ). The heat map displayed the distribution of species using presence/absence data, and the clustering algorithm grouped species with similar habitat categories. The Sørensen (1948) similarity coefficient based on presence/absence data was used to identify significant differences among different habitat types and microclimatic similarities ( Dalirsefat et al., 2009 ). In order to identify hypothetical variables (components) that explain the majority of the variance in our multidimensional data, Non-Metric Multidimensional Scaling (NMDS) was utilized. The contribution of various Raunkiaer’s life forms was visualized using chord diagrams generated with the circlize package ( Gu et al., 2014 ). Subsequently, we computed three diversity indices, namely, species richness, Shannon and Simpson, based on presence/absence of species for all habitats, and all plots and analyses were performed using R software version 4.0.0 ( R Core Team, 2020 ).
Floristic composition and distribution
Based on the present investigation, 155 plant species from 120 genera and 49 families were reported in the current study area ( Table 1 ). The distribution of the species among the 49 families was unequal; just 7 families held half of the species, while 42 families held the other half. 23 families were monotypic ( Figure 2 ). The top five plant families were Asteraceae (19 species, or 12% of all species), Rosaceae (17 species, or 11% of all species), Lamiaceae (15 species, or 10%), Ranunculaceae (10 species, or 6%), and Apiaceae (8 species, or 5%) ( Table 1 ).

Table 1. Floristic composition and life span traits of the forest vegetation in Gurez Valley of Kashmir Himalaya, India.
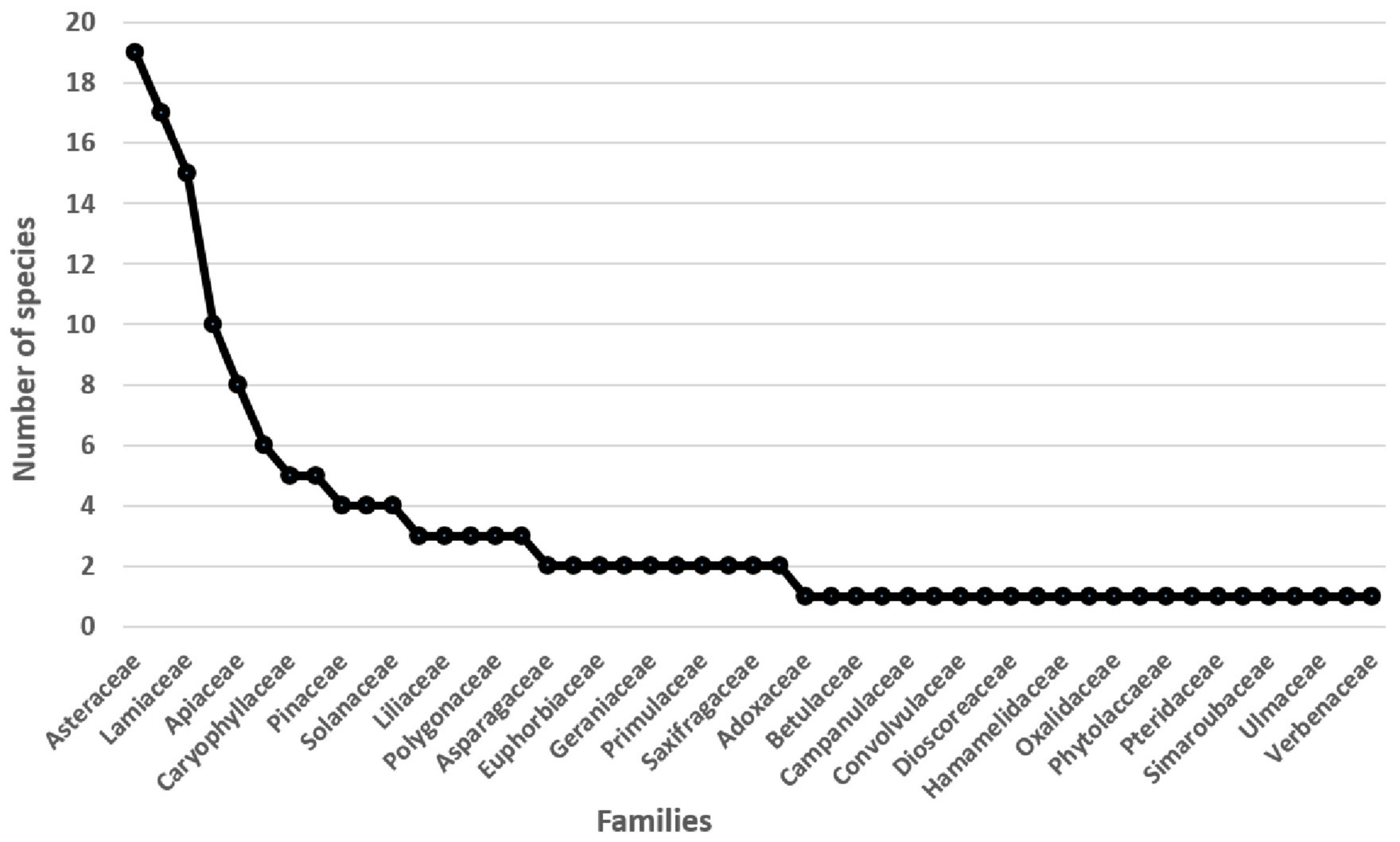
Figure 2. Species- family relationship of documented species in the study area.
Species distribution among growth form types
In the current study, the highest number of plant species (111) were herbaceous plants (72% of all species), followed by 21 tree species (13%), 15 shrubs (10%), four climbers (3%), and four sub-shrubs (2%) ( Table 1 ). Most of the tree species growing in the valley were deciduous (e.g., Aesculus indica, Acer caesium, Betula utilis, Celtis australis, Prunus armeniaca , Prunus cornuta, Populus nigra, and Ulmus villosa ). A few coniferous tree species (e.g., Abies pindrow, Cedrus deodara, Picea smithiana, Pinus wallichiana , and Taxus wallichiana ) were also reported. Shrubs included Rosa webbiana, Berberis lyceum, Rubus ulmifolius, Parrotiopsis jacquemontiana, and Indigofera heterantha. Important medicinal herbs reported from the valley were Ajuga parviflora, Bergenia ciliate, Delphinium roylei, Gentiana kurroo, Heracleum candicans, Phytolacca acinosa, Saussurea costus , Taraxacum officinale , and Trillium govanianum ( Table 1 ). The representative plants species documented in the different habitats of the study area are shown in Plate 2 .
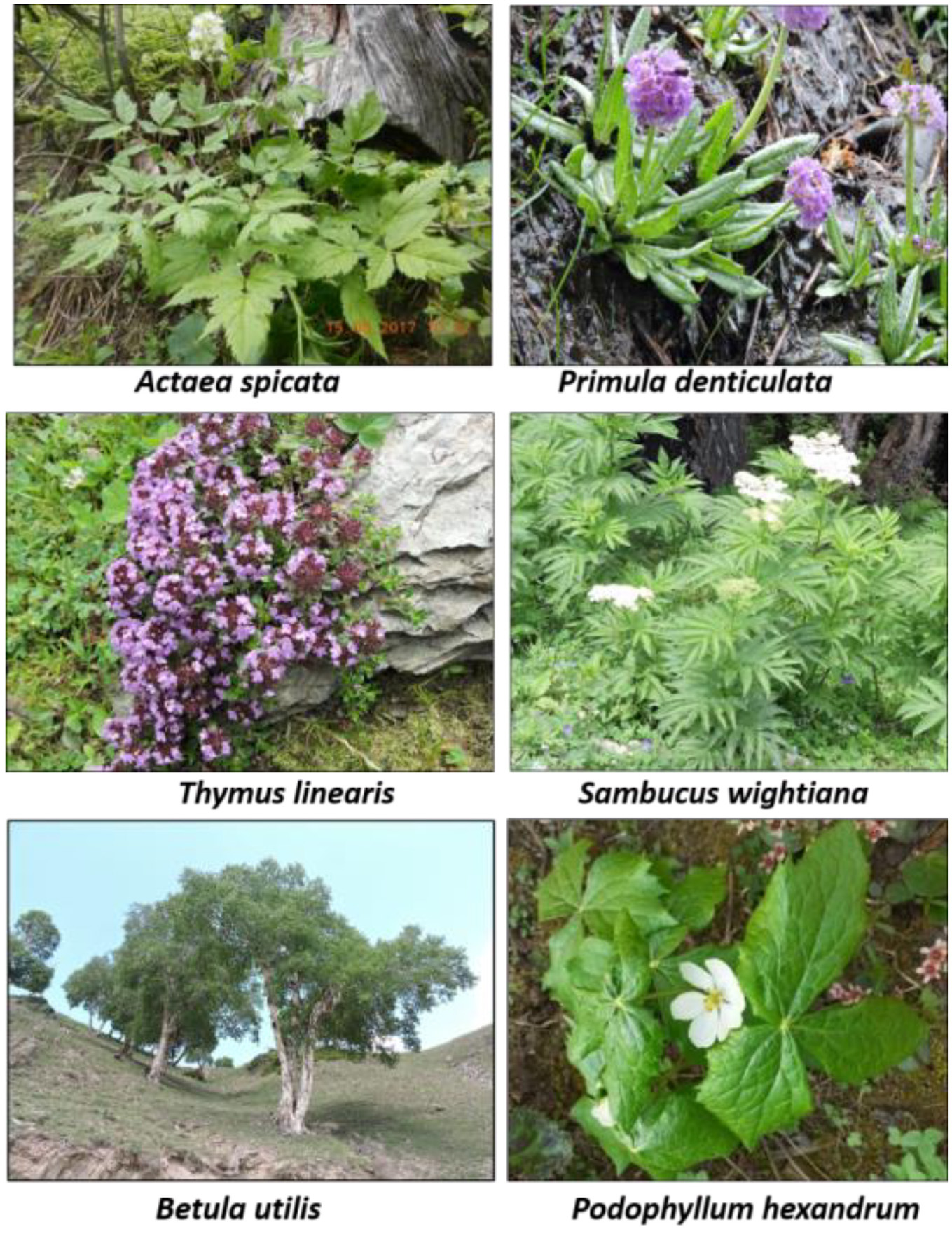
Plate 2. Representative plant species growing in different habitats of the study area.
Species distribution among life-form traits
About 35% of the species (54 species) were therophytes which constituted the dominant life form, followed by 29 species of hemicryptophytes (19%), chamaephytes and geophytes with 15 species each (10%), megaphanerophytes with 13 species (8%), nanophanerophytes with 12 species (8%), cryptophytes and mesophanerophytes with 7 species each (4%), microphanerophytes with 2 species (1%), and parasitic forms with a single species (1%) ( Figure 3 ). Majority of the plant species (129) in the studied valley were represented by perennials (83%) followed by annuals with 23 species (15%), and biennials with three species (2%). According to the phyto-geographical research, 65% of the species documented in the study area were native, with the remaining 15% being invasive, 14% being naturalized, and 5% being casual ( Figure 4 and Table 1 ). The majority (30%) of exotic species were reported on the roadside, followed by natural forests (23%), crop fields (17%), dry slopes (16%), shady areas (5%), moist places, and riparian zones (4% each).
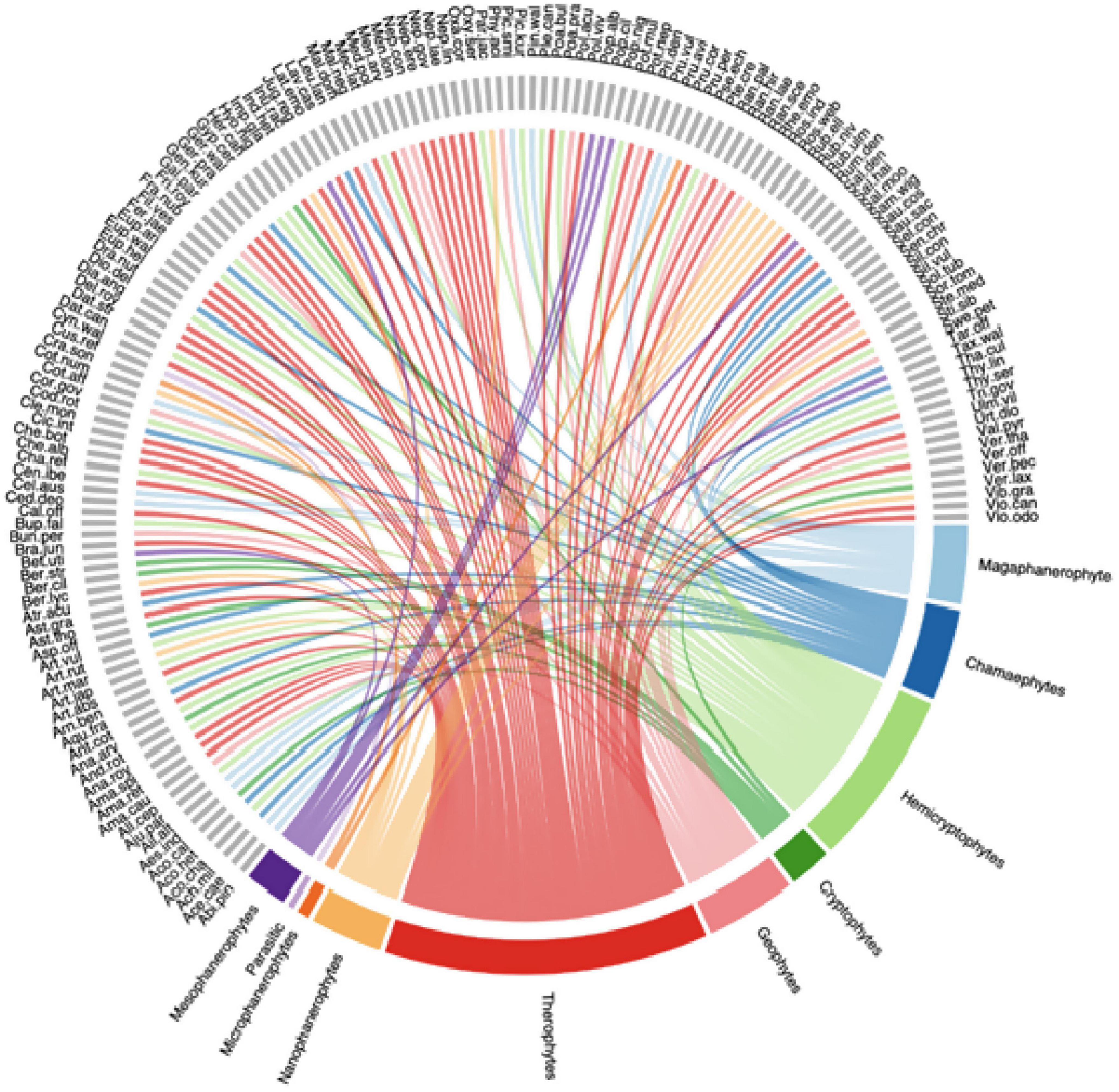
Figure 3. Plant species distribution according to Raunkiaer’s life form of the vegetation in Gurez valley, Kashmir Himalaya, India.
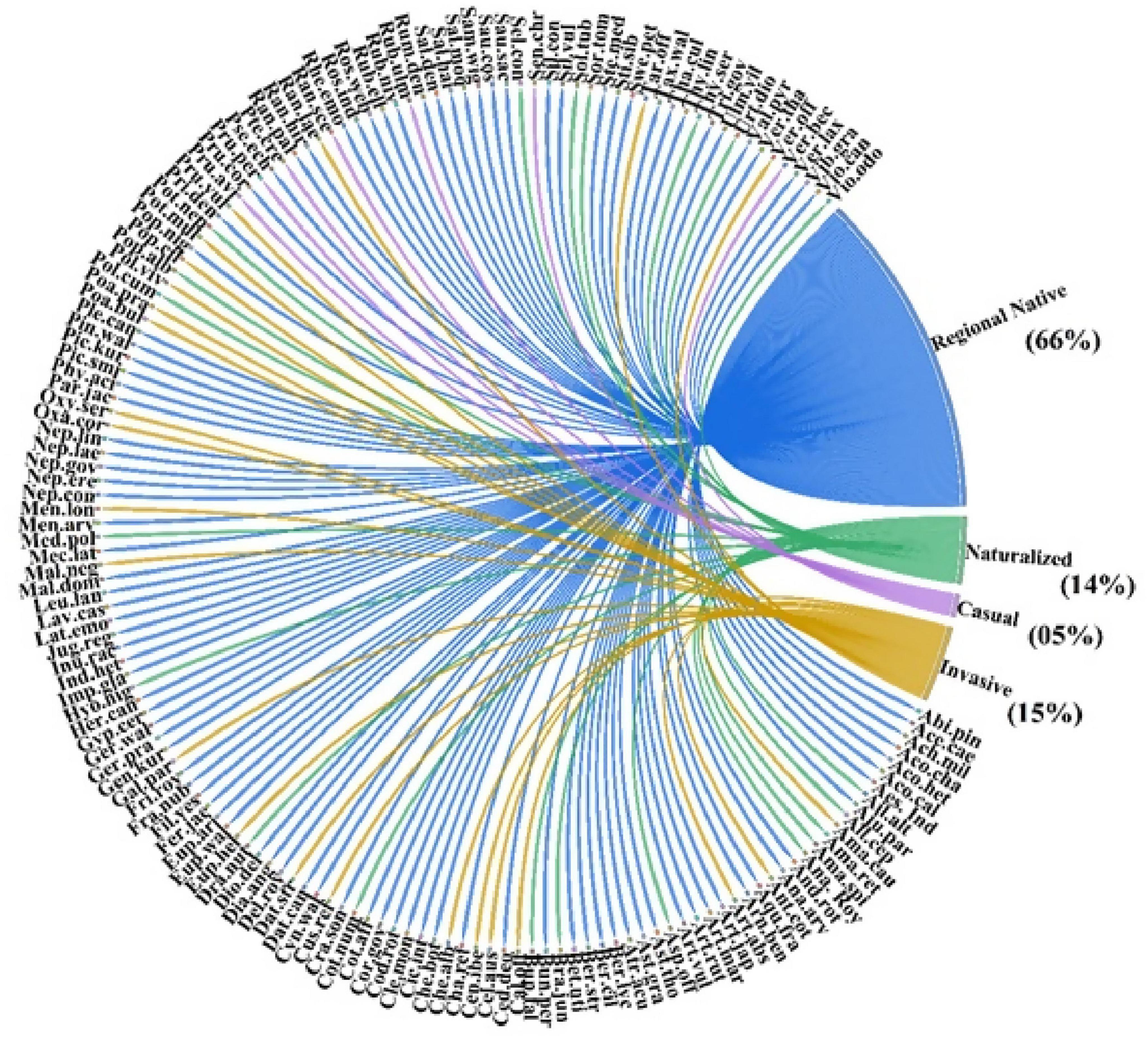
Figure 4. Plant species distribution according to nativity range of the vegetation in Gurez valley, Kashmir Himalaya, India.
Habitat-wise distribution
Of all species encountered 39% grew in the natural forests, 17% on dry slopes, 11% in shady places, 10% in moist places, 2% in riparian zones, 3% in rock crevices, and the remaining species were dispersed in highly distributed habitats, e.g., 11% along roadsides, 6% in crop fields, and 1% in vegetables gardens ( Table 1 and Plate 2 ). Based on their floristic similarity, cluster analysis identified five clusters of various habitat types ( Figure 5 ). Natural forests made up the first cluster, dry slopes and roadside vegetation made up the second, wet and shady areas made up the third, crop fields and vegetable gardens made up the fourth, and riparian vegetation and rock crevices made up the fifth ( Figure 5 ).
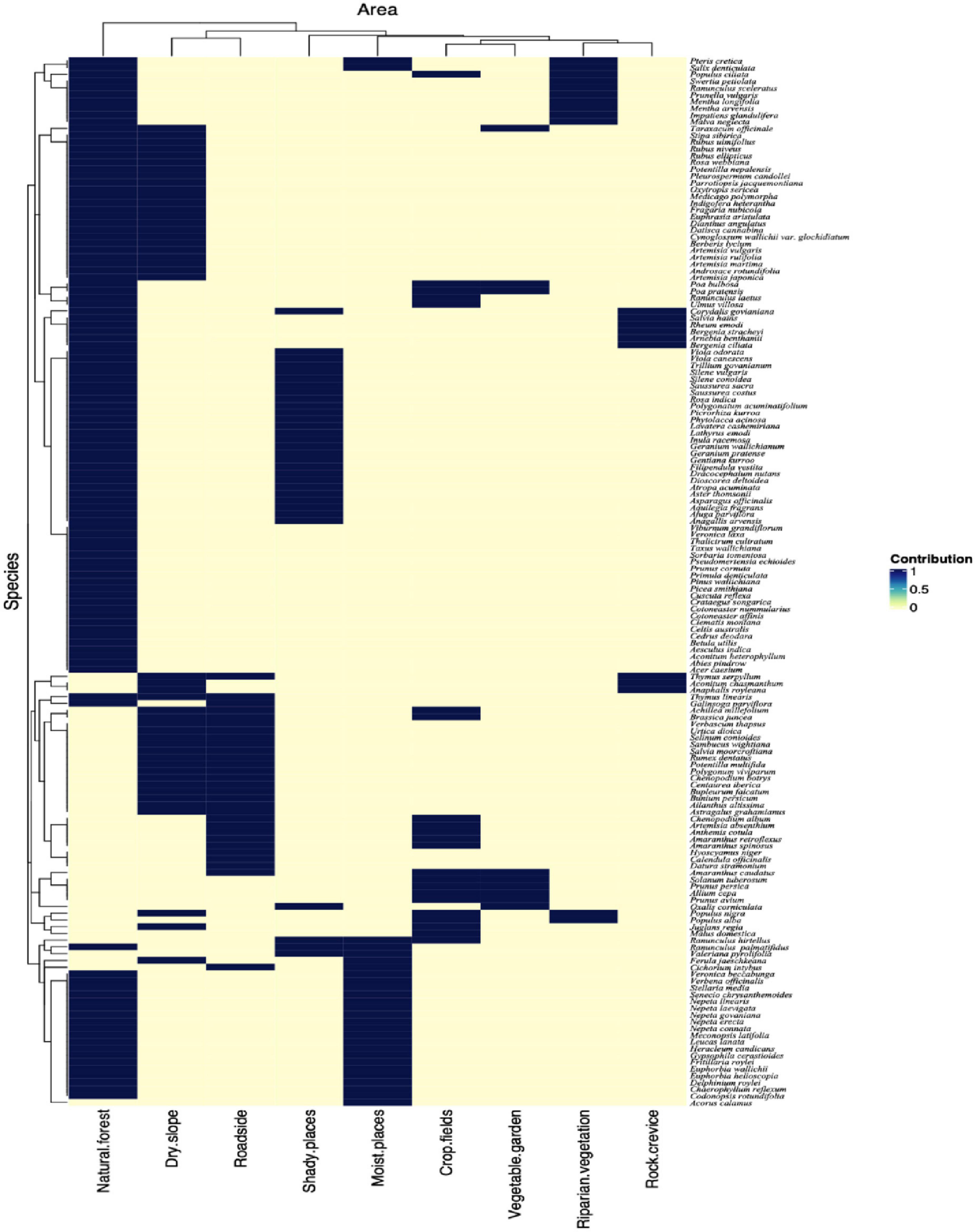
Figure 5. Heat map based on Sørensen’s (1948) similarity index of plant species in Gurez valley, Kashmir Himalaya, India.
Similar to this, the NMDS revealed significant variation in habitat types, with some species groups having a stronger association with particular habitat types than others ( Figure 6 ). In the biplot, five clusters of habitats based on species presence/absence can be identified: dry slope; natural forest; roadside; moist places and rocky crevice; and crop fields, riparian vegetation, shady places, and vegetable gardens. The major plant species richness was detected in rich and optimally moisturized natural forest habitats and middle richness in moist places as well as less distributed habitations.
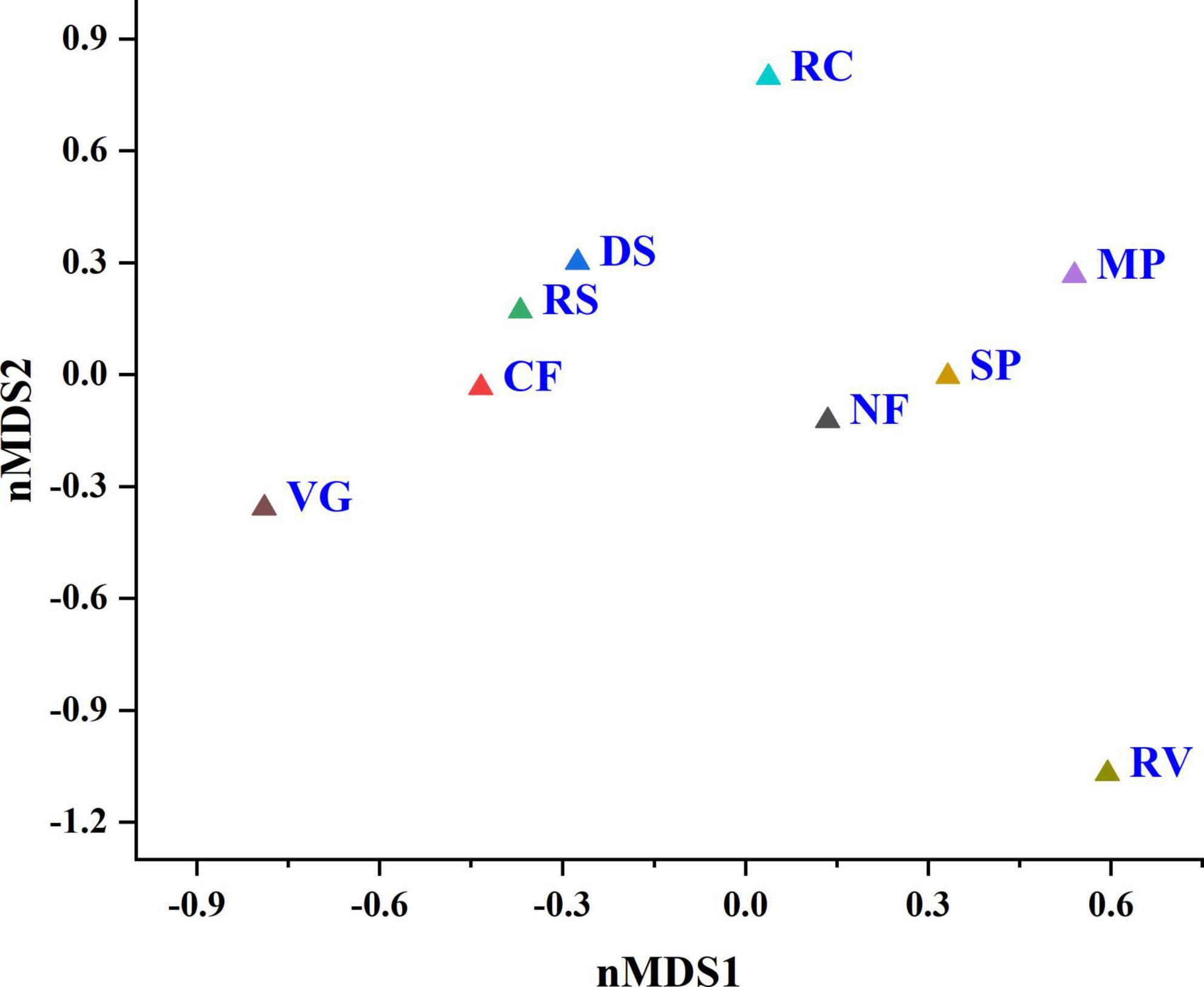
Figure 6. Non-Metric Multidimensional Scaling (NMDS) (plot of different habitat types in Gurez valley, Kashmir Himalaya, India. Full name of habitat is depicted in Table 1 .
According to the estimated diversity indices, Natural forest had the greatest values for all three indices ( Table 2 ). Shannon’s index for this habitat was 4.73, and Simpson’s was 0.99. There were 113 species present. After that, Dry slope and Shady areas held 46 and 31 specie (3.83 and 3.43 for Shannon index, and 0.98 and 0.97 for Simpson index, respectively) ( Table 2 ) lowest values were recorded for Rock crevice and Vegetable garden ( Table 2 ).
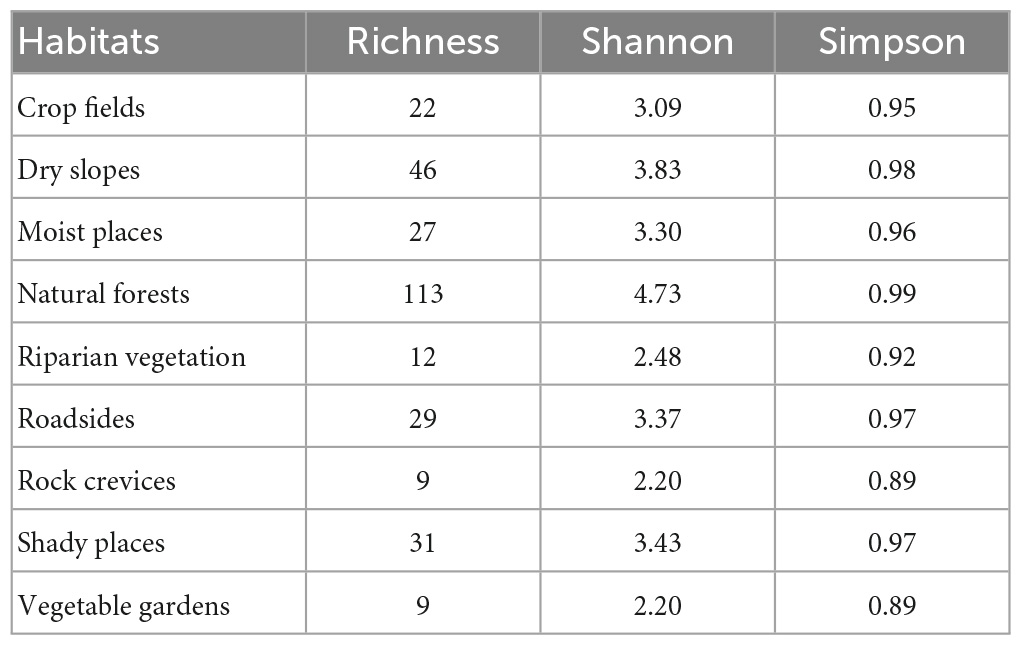
Table 2. Variation of diversity indices between habitat types in Gurez valley, Kashmir Himalaya, India.
In this study, we provide an in-depth evaluation of the qualitative vegetation of remote region of Kashmir Himalaya. The study revealed (i) 155 plant species belonging to 120 genera and 49 families, with (ii) an uneven distribution within families, with 50% of the species belonging to just 7 families and 23 families being monotypic. (iii) Therophytes were the most prevalent form of life, showing that the vegetation was disturbed (iv) Exotic species made up the remaining 35% and most exotic plants were found along roadsides. Finally, (v) natural forests scored the highest values for all three estimated diversity indices, compared to other human-modified habitats. Vegetation analysis helps to develop a comprehensive image of the plant communities of a particular region ( Chhetri and Shrestha, 2019 ). Assessing the floristic diversity of hotspots of biodiversity is essential to understand the conservation status of these areas, which have a significant role in making the conservation strategies as well as policies. The vegetation of region was found to be very diverse due to ecological zonation, different microhabitats, and topographic features. 49 families and 155 species were found in the current investigation. In comparison to past studies conducted in other Himalayan locations ( Qureshi and Bhatti, 2010 ; Semwal et al., 2010 ; Shaheen and Qureshi, 2011 ; Dangwal et al., 2012 ; Singh and Rawat, 2012 ) the number of plant species recorded in the current research area was higher. Numerous interrelated factors, including elevation, regional climate, topography, competition, regional species pool, regional species dynamics, and human activity have an impact on the regional patterns of species richness ( Rahman et al., 2020 ; Mitchell et al., 2023 ). However, Khan et al. (2012) reported a higher number of plant species (198) from the Naran valley forest of Pakistan Himalaya but distributed in a lower number of families (68) when compared to our study. Similarly, Verma and Kapoor (2011) reported 160 vascular plant species belongs to 51 families from Ropa-Giavung valley in the cold deserts of District Kinnaur, Himachal Pradesh, India (also see Manzoor et al., 2016 ; Haq et al., 2022d ; Ullah et al., 2022 ). However, at the region level the species richness of our study was comparatively higher than report in previous studies from Kashmir Himalayas ( Bhat et al., 2014 ). Thus, the slight but predictable fluctuation in species number might be attributed to both abiotic environmental and biotic causes. Apart from elevation, microhabitats that reflect or predict vegetation patterns across landscapes. Together with the previously described findings, our findings demonstrate that while various studies have analyzed the floristic composition of the Himalayan region, each micro-region has unique functional groups that can significantly affect the structuring of the local plant community. Our study showed a smaller number of species than several other studies, but had a larger number of families, which may also represent an important result for the analysis of biodiversity in these regions. Asteraceae, Rosaceae, Lamiaceae, Ranunculaceae, and Apiaceae were reported as the families with the largest number of plant species. Due to wide range of ecological amplitudes, the plant species of Asteraceae are varied in habitats ( Rahman et al., 2019a ; Rashid et al., 2021 ; Waheed et al., 2022 ). Similar findings were observed by Haq et al. (2021d) in Kashmir Himalaya, India and Rahman et al. (2018) in Manoor valley, Pakistan. Many researchers have reported Asteraceae as the dominant family from different regions ( Verma and Kapoor, 2011 ; Hussain et al., 2015 ; Ali et al., 2016 ; Amjad et al., 2017 ; Khan et al., 2018 ; Rahman et al., 2019a ). This demonstrates the family’s strong capacity for adaptation to a variety of environments and temperatures. Additionally, in agreement with our findings, two other studies— Suyal et al. (2010) in the Garhwal Himalaya, India, and Khan et al. (2015) in Kabal (Swat), Pakistan—reported Lamiaceae as the dominant family.
The current study emphasizes the uneven distribution of plant species throughout families, with 23 families being identified as monotypic. The large variety of families, which revealed a varied distribution of flora in the area, is explained by variances in microhabitat, morphological features, life duration, and dynamic ecological niche ( Haq et al., 2022a ; Khoja et al., 2022 ). The diversity and structure of these plant communities can be influenced by various abiotic and biotic factors, which has also been shown by earlier research in the Western Himalayan region, and some species and/or families may not be as able to inhabit particular habitats as others ( Rahman et al., 2018 ). The growth form of trees had higher proportion (13%) than shrubs (10%), an expression of a functioning forest ecosystem ( Khan et al., 2015 ). The present results agreed with Khan et al. (2015) , who found that a large diversity of microhabitats was favorable to tree species in the region. The findings of Sharma and Raina (2018) from other Himalayan forests further support these findings. Herbaceous species (111) were the dominant habit similar to other areas of the Northwestern Himalaya ( Dar and Sundarapandian, 2016 ; Rahman et al., 2019b ; Haq et al., 2021a ; Nafeesa et al., 2021 ). Previous research in the Western Himalayan region has shown that certain species and/or families rarely have the same ability to occupy specific habitats as others, where varied abiotic and biotic effects can alter the diversity and organization of these plant communities ( Haq et al., 2021b ; Nafeesa et al., 2021 ).
It has been observed that the most promising approaches for predicting the species composition of any forest communities revolves around their functional traits. Therefore, studying functional groups usually provides clear information on the direct physiological adaptations of plant communities to particular environments conditions ( Haq et al., 2019 ). The most common life form class were therophytes, followed by hemicryptophytes, magaphanerophytes, chamaephytes, and geophytes. Such biological variety represents the adaptation of plant species to the climatic factors ( Khan et al., 2018 ). Hemi-cryptophytes predominate in the study area due to the cold and mountainous climate. In general, they withstand water scarcity by remaining dry or developing physiological, morphological, and anatomical traits that allow them to tolerate water loss. The fact that therophytes were found as the dominant life form in the studied area, which indicates high biotic disturbance levels on the habitat via grazing, human settlements, agricultural practices, and road constructions, since this life form is commonly associated with the unfavorable dry environmental condition, resulting in adopted strategies for their survival ( Vakhlamova et al., 2016 ). The current findings agreed with Asim et al. (2016) , Rahman et al. (2018 , 2019b ), Wali et al. (2022) who also documented the dominance of therophytes in the respective research areas. The high biotic disturbance significantly alters composition of the herbaceous layer and favored the abundant growth of alien annual weedy species such as such as Anthemis cotula, Amaranthus caudatus, Centaurea iberica, Datura stramonium , and Galinsoga parviflora , all of which are therophytes ( Haq et al., 2021d ). The second most prevalent type of life was hemicryptophytes. The association of hemicryptophytes with a cold, mountainous climate is a likely explanation for their predominance ( Shimwell, 1971 ). Because of the soil and climate in the subalpine zones, chamaephytes are more common ( Khan et al., 2018 ). Some habitats are more suited to plant development than others based on the diversity of species found in the forest environments of different geographic regions ( Medvecká et al., 2018 ). Comparing natural forest habitats to other types of habitat, the current study found that natural forest environments harbored the highest diversity of species. However, anthropogenic disturbances are fragmenting, destroying, and degrading natural forest habitats, which is affecting the composition and layout of forest communities including in this area of the Himalayan region ( Chakraborty et al., 2017 ; Rahman et al., 2022 ). Qureshi and Bhatti (2010) , who recorded the highest number of plant species in natural forest habitats from Pakistan, provide additional support for our findings. Moreover, the resemblance in the plant species pool in human modified habitats provides an evidence toward the indication of ecological filtration that occurs in the region ( Gardner et al., 2009 ). Anthropogenic disturbances common in this Himalayan region include overgrazing, deforestation, over-exploitation and fragmentation of natural intact forests due to linear development such as road networks and transmission lines ( Haq et al., 2022b ). Out of the total reported plant species, 35% were exotic, mostly in human modified habitats. Such numbers are comparable with those documented by Kohli et al. (2004) from the forests of Himachal Pradesh of Indian Himalayas. The most common invasive species growing in forest ecosystems of Jammu and Kashmir included trees like Aesculus indica, Ailanthus altissima, Juglans regia, Populus ciliate , subshrub Sambucus wightiana , and herbs such as Anthemis cotula, Amaranthus caudatus, Centaurea iberica and Datura stramonium ( Haq et al., 2019 , 2023b ). The invasion of plant species is typically facilitated by disturbance because it overwhelms environmental and physical barriers. The parameters that cause the disturbances have been seen to be able to filter the makeup of a community and affect species concurrence by altering resource and safe spot availability ( Davis et al., 2000 ; Haq et al., 2022c , 2023a ). Generally, those species having wide niches and can pass via these filters and likely to overrun newly ecosystems after these are disturbed ( Dukes and Mooney, 1999 ). Furthermore, invasions of exotics into forests might be facilitated by the incursion of other plant species generating conditions that encourage other invasive plant species over native species ( Niu et al., 2007 ). When alien species invade a newly range, innate plant species, adaptation to the new environment, is sometimes evacuated ( van Boheemen et al., 2019 ). The vegetation composition variations rise via a variety of procedure sensuing the disappearance of the diversity of plant species such as communities change from native desired plant species to monospecific positions of the invasive plant species ( Pérez-Ramos et al., 2019 ; Haq et al., 2021c ). Invasive species have broad consequences in affecting potential management to decrease the effects of climate changes ( Kraft et al., 2015 ).
Implications for forest management and policy
According to our findings, 35% of plant species were deemed to be alien, indicating that a large number of non-native species have spread to the inaccessible and isolated Himalaya region. We discovered a high percentage of exotic species (such as Aesculus indica, Ailanthus altissima, Sambucus wightiana, Anthemis cotula, Amaranthus caudatus, Centaurea iberica , and Datura stramonium ) in disturbed forest habitats such as roadsides, and we propose that tunnels, rather than roads, are the best opinions of connectivity in fragile mountain ecosystems. The elements that threaten biodiversity must be minimized in order to keep ecosystems operating and their members intact. Regulations that limit alien species like Anthemis cotula, Artemisia absinthium, Bupleurum falcatum, Cuscuta reflexa, Galinsoga parviflora, and Senecio chrysanthemoides , introductions, and boost recovery, such as modifying structure through enhanced species regeneration and planting native species ( Abies pindrow , Cedrus deodara , Pinus roxburghii, P. wallichiana, Taxus wallichiana, Betula utilis, Ulmus villosa, Trillium govanianum, Ajuga parviflora ). We found that the study area serves as the natural habitat for several significant, endangered medicinal plants, including Arnebia benthamii, Bergenia ciliata, Delphinium roylei, Gentiana kurroo, Phytolacca acinosa, Saussurea costus , and Trillium govanianum . Therefore, we recommend that human intervention in natural regeneration efforts be prioritized in these habitats to increase the population of these species. Finally, existing knowledge of threats to the forest flora can be used to guide management in the face of future climate change. The forest management strategy could be organized so that potential hazards (like forest fragmentation, invasion of exotic species) are addressed before they become a problem. Furthermore, the majority of species recovery initiatives should focus on managing forest restoration in human modified habitats through by planting and reseeding native species like, Ulmus villosa, Betula utilis, Aconitum heterophyllum, Trillium govanianum, Fritillaria roylei, Arnebia benthamii , to lessen susceptibility to future threats in the landscape.
The identification of areas with a high value for biodiversity and the prioritization of these areas for conservation are crucial for preserving biodiversity. The present investigation described qualitative vegetation characteristics in a remote region of the Kashmir Himalaya. The dominance of few families, especially the Asteraceae, is a result of actual and potential dominating invaders in zones with significant disturbance. Therophytes emerged to be the primary form of life in the research area. The presence of a large fraction of therophytes indicates major anthropogenic disruptions. The study examined how environmental factors affect plant communities and placed special emphasis on the idea of habitat filtration as it relates to plant species’ abiotic tolerance. Furthermore, by examining species features from a functional groups standpoint, it may be possible to predict an ecosystem functionality more accurately. The study’s findings suggest that decision-makers and planners should place a greater emphasis on ecologically sustainable development in forest landscapes, considering species composition and the preservation of ecosystem function. Similar approaches should undoubtedly ensure that development activities do not contribute to biodiversity loss in fragile ecosystems.
Data availability statement
The original contributions presented in this study are included in the article/supplementary material, further inquiries can be directed to the corresponding authors.
Author contributions
SH, FL, and AK collected the data. SH and MW analyzed and interpreted the data and results. SH wrote initial draft of the manuscript. SH, RB, EM, HE, and FL revised the manuscript. All authors read and approved the final manuscript.
This study was Researchers Supporting Project (RSP2023R118), King Saud University.
Acknowledgments
We would like to thank Researchers Supporting Project number (RSP2023R118), King Saud University, Riyadh, Saudi Arabia.
Conflict of interest
The authors declare that the research was conducted in the absence of any commercial or financial relationships that could be construed as a potential conflict of interest.
Publisher’s note
All claims expressed in this article are solely those of the authors and do not necessarily represent those of their affiliated organizations, or those of the publisher, the editors and the reviewers. Any product that may be evaluated in this article, or claim that may be made by its manufacturer, is not guaranteed or endorsed by the publisher.
- ^ www.efloras.org
Ali, A., Badshah, L., Hussain, F., and Shinwari, Z. K. (2016). Floristic composition and ecological characteristics of plants of Chail valley, district Swat, Pakistan. Pak. J. Bot. 48, 1013–1026.
Google Scholar
Altaf, A., Haq, S. M., Shabnum, N., and Jan, H. A. (2022). Comparative assessment of phyto diversity in Tangmarg Forest division in Kashmir Himalaya, India. Acta Ecol. Sin. 42, 609–615. doi: 10.1016/j.chnaes.2021.04.009
CrossRef Full Text | Google Scholar
Amjad, M. S., Arshad, M., Page, S., Qureshi, R., and Mirza, S. N. (2017). Floristic composition, biological spectrum and phenological pattern of vegetation in the subtropical forest of Kotli District, AJK, Pakistan. Pure Appl. Biol. 6, 426–447. doi: 10.19045/bspab.2017.60043
Asim, Z. I., Haq, F, Iqbal, A. (2016). Phenology, life form and leaf spectra of the vegetation of Kokarai valley, district swat. J. Bio. Environ. Sci. 9, 23–31.
Barkley, T. M., DePriest, P., Funk, V., Kiger, R. W., Kress, W. J., and Moore, G. (2004). Linnaean nomenclature in the 21st Century: A report from a workshop on integrating traditional nomenclature and phylogenetic classification. Taxon 53, 153–158. doi: 10.2307/4135501
Bhat, F., Mahajan, D. M., Sayyed, MRG, Bhat, A. (2014). Phytosociological measurements and vegetation analysis along an altitudinal gradient of Lolab valley (Kashmir Himalaya. India). WCS’s Natl. J. Inter. Res. 1, 1–13.
Chakraborty, A., Ghosh, A., Sachdeva, K., and Joshi, P. K. (2017). Characterizing fragmentation trends of the Himalayan forests in the Kumaon region of Uttarakhand, India. Ecol. Inform. 38, 95–99. doi: 10.1016/j.ecoinf.2016.12.006
Chhetri, N. B. K., and Shrestha, K. K. (2019). Floristic diversity and important value indices of tree species in lower Kanchenjunga Singhalila Ridge Eastern Nepal. Am. J. Plant Sci . 10, 248–263. doi: 10.4236/ajps.2019.101019
Dalirsefat, S. B., da Silva, A., Meyer, and Mirhoseini, S. Z. (2009). Comparison of similarity coefficients used for cluster analysis with amplified fragment length polymorphism markers in the silkworm Bombyx mori . J. Insect Sci. 9, 1–8. doi: 10.1673/031.009.7101
PubMed Abstract | CrossRef Full Text | Google Scholar
Dangwal, L. R., Singh, T., Singh, A., and Sharma, A. (2012). Plant diversity assessment in relation to disturbances in subtropical chirpine forest of the western Himalaya of district Rajouri, J&K, India. Int. J. Plant Anim. Environ. Sci. 2, 206–213.
Dar, J. A., and Sundarapandian, S. (2016). Patterns of plant diversity in seven temperate forest types of Western Himalaya, India. J. Asia Pac. Biodivers. 9, 280–292. doi: 10.1016/j.japb.2016.03.018
Davis, M. A., Grime, J. P., and Thompson, K. (2000). Fluctuating resources in plant communities: A general theory of invasibility. J. Ecol. 88, 528–534. doi: 10.1046/j.1365-2745.2000.00473.x
Dukes, J. S., and Mooney, H. A. (1999). Does global change increase the success of biological invaders?. Trends Ecol. Evol. 14, 135–139. doi: 10.1016/S0169-5347(98)01554-7
Gardner, T. A., Barlow, R., Chazdon, R. M., Ewers, C. A., Harvey, C. A., Peres, C., et al. (2009). Prospects for tropical forest biodiversity in a human-modified world. Ecol. Lett. 12, 561–582. doi: 10.1111/j.1461-0248.2009.01294.x
Grime, J. P., Hodgson, J. G., and Hunt, R. (2014). Comparative plant ecology: A functional approach to common British species. Berlin: Springer.
Gu, Z., Gu, L., Eils, R., Schlesner, M., and Brors, B. (2014). circlize implements and enhances circular visualization in R. Bioinformatics 30, 2811–2812. doi: 10.1093/bioinformatics/btu393
Haq, S. M., Calixto, E. S., and Kumar, M. (2020). Assessing biodiversity and productivity over a small-scale gradient in the protected forests of Indian Western Himalayas. J. Sustain. 40, 675–694. doi: 10.1080/10549811.2020.1803918
Haq, S. M., Lone, F. A., Kumar, M., Calixto, E. S., Waheed, M., Casini, R., et al. (2023b). Phenology and diversity of weeds in the agriculture and horticulture cropping systems of Indian Western Himalayas: Understanding implications for agro-ecosystems. Plants 12:1222. doi: 10.3390/plants12061222
Haq, S. M., Waheed, M., Khoja, A. A., Amjad, M. S., Bussmann, R. W., Ali, K., et al. (2023a). Measuring forest health at stand level: A multi-indicator evaluation for use in adaptive management and policy. Ecol. Indicat. 150:110225. doi: 10.1016/j.ecolind.2023.110225
Haq, S. M., Malik, Z. A., and Rahman, I. U. (2019). Quantification and characterization of vegetation and functional trait diversity of the riparian zones in protected forest of Kashmir Himalaya, India. Nord. J. Bot. 37:11. doi: 10.1111/njb.02438
Haq, S. M., Calixto, E. S., Rashid, I., and Khuroo, A. A. (2021d). Human-driven disturbances change the vegetation characteristics of temperate forest stands: A case study from Pir Panchal mountain range in Kashmir Himalaya. Trees For. People 6:100134. doi: 10.1016/j.tfp.2021.100134
Haq, S. M., Shah, A. A., Yaqoob, U., and Hassan, M. (2021a). Floristic quality assessment index of the Dagwan stream in Dachigam National Park of Kashmir Himalaya. Proc. Natl. Acad. Sci. India Sec B Biol. Sci. 91, 657–664. doi: 10.1007/s40011-021-01247-w
Haq, S. M., Yaqoob, U., Calixto, E. S., Kumar, M., Rahman, I. U., Hashem, A., et al. (2021b). Long-term impact of transhumance pastoralism and associated disturbances in high-altitude forests of Indian Western Himalaya. Sustainability 13:12497. doi: 10.3390/su132212497
Haq, S. M., Hamid, M., Lone, F. A., and Singh, B. (2021c). Himalayan hotspot with alien weeds: A case study of biological spectrum, phenology, and diversity of weedy plants of high-altitude Mountains in District Kupwara of J&K Himalaya, India. Proc. Natl. Acad. Sci. India Sec. B Biol. Sci. 91, 139–152. doi: 10.1007/s40011-020-01219-6
Haq, S. M., Khan, I., Malik, Z. A., and Singh, B. (2022d). “Plant diversity and species distribution pattern across the Pir Panjal mountain forest range in the Western Himalayas.” in Biodiversity, conservation and sustainability in Asia: Volume 2: Prospects and challenges in south and middle Asia , eds M. Öztürk, S. M. Khan, V. Altay, R. Efe, D. Egamberdieva, F. O. Khassanov (Cham: Springer International Publishing), 67–84.
Haq, S. M., Waheed, M., Bussmann, R. W., and Arshad, F. (2022a). Vegetation composition and ecological characteristics of the forest in the Shawilks Mountain Range from Western Himalayas. Acta Ecol. Sin. doi: 10.1016/j.chnaes.2022.10.008
Haq, S. M., Yaqoob, U., Hamid, S., Hassan, M., Bashir, F., Waheed, M., et al. (2022b). Localized impact of livestock settlements on vegetation patterns in fir forests of Kashmir Himalaya. Acta Ecol. Sin. 42, 407–416. doi: 10.1016/j.chnaes.2022.07.004
Haq, S. M., Amjad, M. S., Waheed, M., Bussmann, R. W., and Proæków, J. (2022c). The floristic quality assessment index as ecological health indicator for forest vegetation: A case study from Zabarwan Mountain Range, Himalayas. Ecol. Indic. 145:109670. doi: 10.1016/j.ecolind.2022.109670
Hua, F., Bruijnzeel, L. A., Meli, P., Martin, P. A., Zhang, J., Nakagawa, S., et al. (2022). The biodiversity and ecosystem service contributions and trade-offs of forest restoration approaches. Science 376, 839–844. doi: 10.1126/science.abl4649
Hussain, F., Shah, S. S., Badshah, L., and Durrani, M. J. (2015). Diversity and ecological characteristics of flora of Mastuj valley, district Chitral, Hindukush range, Pakistan. Pak. J. Bot. 47, 495–510.
Khan, S. M., Page, S., Ahmad, H., Shaheen, H., and Harper, D. M. (2012). Vegetation dynamics in the Western Himalayas, diversity indices and climate change. Sci. Tech. Dev. 31, 232–243.
Khan, W., Khan, S. M., and Ahmad, H. (2015). Altitudinal variation in plant species richness and diversity at Thandiani sub forests division, Abbottabad, Pakistan. JBES 7, 46–53.
Khan, W., Khan, S. M., Ahmad, H., Alqarawi, A. A., Shah, G. M., Hussain, M., et al. (2018). Life forms, leaf size spectra, regeneration capacity and diversity of plant species grown in the Thandiani forests, district Abbottabad, Khyber Pakhtunkhwa, Pakistan. Saudi J. Biol. Sci. 25, 94–100. doi: 10.1016/j.sjbs.2016.11.009
Khoja, A. A., Haq, S. M., Majeed, M., Hassan, M., Waheed, M., Yaqoob, U., et al. (2022). Diversity, ecological and traditional knowledge of pteridophytes in the western Himalayas. Diversity 14:628. doi: 10.3390/d14080628
Kier, G., Mutke, J., Dinerstein, E., Ricketts, T. T., Küper, W., Kreft, H., et al. (2005). Global patterns of plant diversity and floristic knowledge. J. Biogeogr. 32, 1107–1116. doi: 10.1111/j.1365-2699.2005.01272.x
Kohli, R. K., Dogra, K. S., Batish, D. R., and Singh, H. P. (2004). Impact of invasive plants on the structure and composition of natural vegetation of Northwestern Indian Himalayas1. Weed Technol. 18, 1296–1300. doi: 10.1614/0890-037X(2004)018[1296:IOIPOT]2.0.CO;2
Kraft, N. J., Adler, P. B., Godoy, O., James, E. C., Fuller, S., and Levine, J. M. (2015). Community assembly, coexistence and the environmental filtering metaphor. Funct. Ecol . 29, 592–599. doi: 10.1111/1365-2435.12345
Mahar, G., Dhar, U., Rawal, R. S., and Bhatt, I. D. (2009). Implications of location specific data and their usefulness in conservation planning: An example from Indian Himalayan Region (IHR). Biodivers. Conserv . 18, 1273–1286. doi: 10.1007/s10531-008-9450-0
Manzoor, M., Riaz, A., Iqbal, Z., and Mian, A. (2016). Biodiversity of Pir Lasura National Park, Azad Jammu and Kashmir, Pakistan. Sci. Tech. Dev. 82, 182–196.
PubMed Abstract | Google Scholar
Medvecká, J., Jarolímek, I., Hegedüšová, K., Škodová, I., Bazalová, D., Botková, K., et al. (2018). Forest habitat invasions–Who with whom, where and why. For. Ecol. Manag. 409, 468–478. doi: 10.1016/j.foreco.2017.08.038
Mitchell, J. C., Kashian, D. M., Chen, X., Cousins, S., Flaspohler, D., Gruner, D. S., et al. (2023). Forest ecosystem properties emerge from interactions of structure and disturbance. Front. Ecol. Environ. 21:14–23. doi: 10.1002/fee.2589
Myers, N., Mittermeier, R. A., Mittermeier, C. G., Da Fonseca, G. A., and Kent, J. (2000). Biodiversity hotspots for conservation priorities. Nature 403, 853–858. doi: 10.1038/35002501
Nafeesa, Z., Haq, S. M., Bashir, F., Gaus, G., Mazher, M., Anjum, M., et al. (2021). Observations on the floristic, life-form, leaf-size spectra and habitat diversity of vegetation in the Bhimber hills of Kashmir Himalayas. Acta Ecol. Sin. 41, 228–234. doi: 10.1016/j.chnaes.2021.03.003
Niu, H. B., Liu, W. X., Wan, F. H., and Liu, B. (2007). An invasive aster ( Ageratina adenophora ) invades and dominates forest understories in China: Altered soil microbial communities facilitate the invader and inhibit natives. Plant Soil 294, 73–85. doi: 10.1007/s11104-007-9230-8
Noss, R. F. (1983). A regional landscape approach to maintain diversity. BioScience 33, 700–706. doi: 10.2307/1309350
Olson, D. M., Dinerstein, E., Wikramanayake, E. D., Burgess, H. D., Powell, G. V., and Underwood, C. E. (2001). Terrestrial ecoregions of the world: A new map of life on earth a new global map of terrestrial ecoregions provides an innovative tool for conserving biodiversity. BioScience 51, 933–938. doi: 10.1641/0006-3568(2001)051[0933:TEOTWA]2.0.CO;2
Pérez-Harguindeguy, N., Diaz, S., Garnier, E., Lavorel, S., Poorter, H., Jaureguiberry, P., et al. (2016). Corrigendum to: New handbook for standardised measurement of plant functional traits worldwide. Aust. J. Bot. 64, 715–716. doi: 10.1071/BT12225_CO
Pérez-Ramos, I. M., Matías, L., Gómez-Aparicio, L., and Godoy, O. (2019). Functional traits and phenotypic plasticity modulate species coexistence across contrasting climatic conditions. Nat. Commun . 10:2555. doi: 10.1038/s41467-019-10453-0
Qian, H., Zhou, Y., Zhang, J., Jin, Y., Deng, T., and Cheng, S. (2021). A synthesis of botanical informatics for vascular plants in Africa. Ecol. Inform. 64:101382. doi: 10.1016/j.ecoinf.2021.101382
Qureshi, R., and Bhatti, G. R. (2010). Floristic inventory of PAI Forest, Nawab Shah, Sindh, Pakistan. Pak. J. Bot. 42, 2215–2224.
R Core Team (2020). A language and environment for statistical computing . Vienna: R Foundation for Statistical Computing.
Rahman, I. U., Afzal, A., Iqbal, Z., Abd Allah, E., Alqarawi, A. A., Calixto, E. S., et al. (2019a). Role of multivariate approaches in floristic diversity of Manoor Valley (Himalayan Region), Pakistan. Appl. Ecol. Environ. Res. 17, 1475–1498. doi: 10.15666/aeer/1702_14751498
Rahman, I. U., Calixto, E. S., Afzal, A., Iqbal, Z., Ali, N., Ijaz, F., et al. (2019b). “Advanced multivariate and computational approaches in agricultural studies,” in Essentials of bioinformatics , Vol. III, eds K. Hakeem, N. Shaik, B. Banaganapalli, and R. Elango (Cham: Springer), 93–102. doi: 10.1007/978-3-030-19318-8_5
Rahman, I. U., Afzal, A., Iqbal, Z., Bussmann, R. W., Alsamadany, H., Calixto, E. S., et al. (2020). Ecological gradients hosting plant communities in Himalayan subalpine pastures: Application of multivariate approaches to identify indicator species. Ecol. Inform. 60:101162. doi: 10.1016/j.ecoinf.2020.101162
Rahman, I. U., Afzal, A., Iqbal, Z., Ijaz, F., Ali, A., Asif, M., et al. (2018). First insights into the floristic diversity, biological spectra and phenology of Manoor Valley, Pakistan. Pak. J. Bot. 5, 1113–1124.
Rahman, I. U., Hart, R. E., Ijaz, F., Afzal, A., Iqbal, Z., and Calixto, E. S. (2022). Environmental variables drive plant species composition and distribution in the moist temperate forests of Northwestern Himalaya, Pakistan. PLoS One 17:e0260687. doi: 10.1371/journal.pone.0260687
Rashid, I., Haq, S. M., Lembrechts, J. J., Khuroo, A. A., Pauchard, A., and Dukes, J. S. (2021). Railways redistribute plant species in mountain landscapes. J. Appl. Ecol. 58, 1967–1980. doi: 10.1111/1365-2664.13961
Raunkiaer, C. (1934). The life forms of plants and statistical geographical. Oxford: Clarendon Press, 632.
Safidkon, F., R. Kalvandi, M. Atri and M.M. Barazandeh. (2003). Contribution for the characterization of Thymus eriocalyx chemotypes. Int. Mag. Cosmet. Fragr.
Semwal, D. P., Uniyal, P. L., and Bhatt, A. B. (2010). Structure, composition and dominance–diversity relations in three forest types of a part of Kedarnath Wildlife Sanctuary, Central Himalaya, India. Not. Sci. 2, 128–132. doi: 10.15835/nsb234655
Shaheen, H., and Qureshi, R. A. (2011). Vegetation types of Sheosar Lake and surrounding landscape in Deosai Plains of North Pakistan, Western Himalayas. J. Med. Plant Res. 5, 599–603.
Sharma, J., and Raina, A. K. (2018). Quantitative analysis, distributional pattern and species diversity of woody plant species of Lamberi Forest Range, Rajouri, J&K, India. J. Nat. Appl. Sci. 10, 522–527. doi: 10.31018/jans.v10i1.1661
Shimwell, D. W. (1971). The description and classification of vegetation. London: Sedgwick and Jackson, 322.
Singh, G., and Rawat, G. S. (2012). “Depletion of oak ( Quercus spp.) forests in the western Himalaya: Grazing, fuelwood and fodder collection,” in Global perspectives on sustainable forest management , ed. C. A. Okia (Crotia: Intech publisher), 29–42. doi: 10.5772/33554
Solefack, M. C. M., Fedoung, E. F., and Temgoua, L. F. (2018). Factors determining floristic composition and functional diversity of plant communities of Mount Oku forests, Cameroon. Asia Pac. Biodivers. 11, 284–293. doi: 10.1016/j.japb.2018.03.005
Sørensen, T. (1948). A method of establishing groups of equal amplitude in plant sociology based on similarity of species content and its application to analyses of the vegetation on Danish commons. Videnski Selskab Biologiske Skrifter 5, 1–34.
Suyal, S., Sharma, C. M., Gairola, S., Ghildiyal, S., Rana, C., Butola, D. S. (2010). Phyto diversity (angiosperms and gymnosperms) in Chaurangikhal forest of Garhwal Himalaya, Uttarakhand, India. Indian J. Sci. Tech. 3, 267–275. doi: 10.17485/ijst/2010/v3i3.2
Ullah, U., Rahim, F., Jan, H. A., Haq, S. M., Wali, S., and Filimban, F. Z. (2022). A survey of the floristic composition of the Kambat Valley, District Dir Lower, Northern Pakistan. Acta Ecol. Sin. doi: 10.1016/j.chnaes.2022.08.002
Vakhlamova, T., Rusterholz, H. P., Kanibolotskaya, Y., and Baur, B. (2016). Effects of road type and urbanization on the diversity and abundance of alien species in roadside verges in Western Siberia. Plant Ecol. 217, 241–252. doi: 10.1007/s11258-016-0565-1
van Boheemen, L. A., Atwater, D. Z., and Hodgins, K. A. (2019). Rapid and repeated local adaptation to climate in an invasive plant. New Phytol. 222, M614–M627. doi: 10.1111/nph.15564
Verma, R. K., and Kapoor, K. S. (2011). Plant species diversity in Ropa-Giavung valley in cold deserts of District Kinnaur, Himachal Pradesh. Biol. Forum Int. J. 3, 34–43.
Waheed, M., Haq, S. M., Arshad, F., Bussmann, R. W., Iqbal, M., Bukhari, N. A., et al. (2022). Grasses in semi-arid lowlands—community composition and spatial dynamics with special regard to the influence of edaphic factors. Sustainability 14:14964. doi: 10.3390/su142214964
Wali, S., Jan, H. A., Haq, S. M., Calixto, E. S., Bussmann, R. W., and Rahim, F. (2022). Phyto-ecological study of the forests of Shishi Koh Valley, Chitral, Pakistan. Vegetos 35, 1024–1035. doi: 10.1007/s42535-022-00379-2
Keywords : plant invasion, habitat diversity, hotspots of biodiversity, ecological traits, Kashmir Himalaya
Citation: Haq SM, Khoja AA, Lone FA, Waheed M, Bussmann RW, Mahmoud EA and Elansary HO (2023) Floristic composition, life history traits and phytogeographic distribution of forest vegetation in the Western Himalaya. Front. For. Glob. Change 6:1169085. doi: 10.3389/ffgc.2023.1169085
Received: 18 February 2023; Accepted: 15 May 2023; Published: 02 June 2023.
Reviewed by:
Copyright © 2023 Haq, Khoja, Lone, Waheed, Bussmann, Mahmoud and Elansary. This is an open-access article distributed under the terms of the Creative Commons Attribution License (CC BY) . The use, distribution or reproduction in other forums is permitted, provided the original author(s) and the copyright owner(s) are credited and that the original publication in this journal is cited, in accordance with accepted academic practice. No use, distribution or reproduction is permitted which does not comply with these terms.
*Correspondence: Shiekh Marifatul Haq, [email protected] ; Hosam O. Elansary, [email protected]
Disclaimer: All claims expressed in this article are solely those of the authors and do not necessarily represent those of their affiliated organizations, or those of the publisher, the editors and the reviewers. Any product that may be evaluated in this article or claim that may be made by its manufacturer is not guaranteed or endorsed by the publisher.
- Original Article
- Open access
- Published: 19 July 2022
Plant diversity and community analysis of Sele-Nono forest, Southwest Ethiopia: implication for conservation planning
- Alemayehu Kefalew ORCID: orcid.org/0000-0001-8259-9309 1 , 3 ,
- Teshome Soromessa 2 &
- Sebsebe Demissew 1
Botanical Studies volume 63 , Article number: 23 ( 2022 ) Cite this article
5343 Accesses
5 Citations
Metrics details
Studying the floristic diversity of a certain forest is a basic aspect of the design and management of forest vegetation; and consequently this study focused on the plant diversity and community analysis of the Sele-Nono forest. For the current study, plants were sampled from 90 plots using a stratified random sampling technique along the established strata of the study forest. In all the plots, both floristic and environmental data that were relevant to the study were collected following the state of the art. Based on the collected data, the community types, ordination, floristic diversity, and threats to the forest were analyzed using R-package and SPSS software.
Cluster analysis produced seven distinct community types which significantly differed among themselves (Cophentic correlation coefficient = 0.785, P < 0.001) of which community types 2 and 6 were relatively poor; whereas communities 1 and 4 were rich in terms of their species richness and diversity. In addition, Canonical Correspondence Analysis (CCA) suggests that a number of environmental factors such as altitude and slope (topographic factor), OM and N (edaphic factors) and disturbance were the main drivers for the current distribution of plant species and disparity in plant community composition in Sele-Nono forest. Moreover, the study revealed high beta diversity ( \({\beta }_{w}\) >12) of plant species at the landscape level (i.e., throughout the study forest). Deforestation for agricultural land expansion and degradation through selective logging are the main threats to the Sele-Nono forest.
Conclusions
The present study revealed that the Sele-Nono forest is a large and heterogenous forest at the landscape level (150, 325.27 ha; \({\beta }_{w}\) >12). Moreover, it is one of the richest and diverse forest ecosystems in terms of plant biodiversity, and it could qualify to be labeled as a keystone ecosystem. However, currently it is exposed to a variety of threats. We recommend the forest to be developed into a biosphere reserve. We also recommend the prioritization of areas belonging to community types 2 and 6 of the forest for any possible conservation actions so as to maximize species richness and diversity of the native plants of the area.
Introduction
Forest, according to FAO ( 2020 ), is defined as segments of a land spanning more than 0.5 hectares with trees higher than 5 m and a canopy cover of more than 10%, or trees able to reach these thresholds in situ. Forests are crucial habitats for hosting diverse organisms and providing ecosystem services (Wilson 1992 ; Pearce and Pearce 2001 ; Wilson et al. 2012 ; Brockerhoff et al. 2017 ). Globally, they are the dominant ecosystem of the world and cover approximately 30% of the planet’s land area (Olson and Dinerstein 1998 ; Olson et al. 2001 ; FAO, 2010 ). Of the global forests, tropical forests are believed to be the most species-rich ecosystem on earth although it covers only about 5% of the Earth’ s surface (Mutke and Barthlott 2005 ; World Conservation Monitoring Centre (WCMC 1992 ); Wright and Muller-Landau 2006 ; Elliot et al. 2013 ; Wilson et al. 2012 ). The tropical forests are mainly distributed in tropical landmasses such as America, Asia, and Africa which are situated between the tropic of Cancer and the tropic of Capricorn (Thomas and Boltzer 2002 ).
It is said that about 30% of the tropical forest cover is found in Africa, which is accounting for 21% of its total land area and for 16% of the global forest coverage (Whitmore 1997 ; Richards 1996 ; Whitmore 2008 ; Klopper et al. 2002 ; FAO 2006 ). Among the tropical forest ecosystems of Africa, its Afromontane forests are the most species-rich ecosystems housing more than half of African’s flora (Sayer et al. 1992 ; Kuper et al. 2004 ). These Afromontane forests are distributed along with the mountain spots of Cameroon and Sierra Leone in the West to the Ethiopian highlands and the Somalia Ahl Muscat Mountains in the East; and from the Red Sea Hills in the north to the Cape region in the south (White 1978 ; Friis 1992 ; Linder et al. 2005 ). More than 50% of these Afromontane forests are found in Ethiopia (Yalden 1983 ).
Although there is controversy over the precise figure of the former forest cover in Ethiopia, it is thought that about 35–40% of the land area has been covered with forests (EFAP 1994 ). However, by the 1950s the high forests declined to 16% of the total land area (Breitenbach 1963 ) which furthermost rapidly dropped to 3.6% by the 1980s (Egziabher 1991 ). In 2003, the forest cover was estimated to be 2.3% (Tedla 2003 ; WBISPP 2004 ; FAO 2006 ). By now even if the country currently increased its forest coverage due to the new FAO forest definition, the natural forest cover still decline to less than 2% (Kelbessa/Member of the task force in FAO/, Pers. Comm). The remaining major dense forests are found in the moist highlands of Southwest (SW) Ethiopia (MOA 1990 ; Reusing 1998 ; Friis et al. 2011 ).
Given the existed access restrictions of the SW forest due to its unique physical setting, climatic conditions, and biogeographical position, the area was not to the attention of researchers for a long time in the history of botanical explorations compared to other corners of Ethiopian vegetation (Meyer 1965 ; Chaffey 1978b ; Friis 2002 ; Teketay 2004 ; Friis 2009 ). However, by the mid-1900s, which is often considered as a renaissance period by the botanists due to access roads (Tadesse 1993 , 1994 ; Friis 2011 ), some pioneered floristic work on the area became evident (Logan 1946 ; Chaffey 1978a , 1979 ; Friis 1979 ; Friis et al. 1982 ; 1986a , b ). The outcome of these studies discovered the area to have ecological and economic potential and attracted the attention of many other researchers for further studies. The research effort of the Ethiopian botanist and related stakeholders were instrumental in providing additional data to ‘Conservation International’ to consider the area as part of the Eastern Afromontane hotspot so as to give due recognition for being a globally key area for biodiversity conservation(WWF and IUCN 1994 ; Barthlott et al. 1999 ; Kuper et al. 2004 ; Mittermeier et al. 2004 ; Conservation International 2006 ; Vivero et al. 2006 ).
From these early efforts on, vegetation scientists did specific floristic studies and generated enormous data on the diversity, distribution, and conservation status of the existing patches of the area. Some of these studies include the work of Derero ( 1998 ), Kigomo ( 1999 ); Yeshitela and Bekele ( 2002 ), Woldemariam ( 2003 ), Yeshitela and Bekele ( 2003 ), Bekele ( 2003 ), Woldu and Yeshitela ( 2003 ), Berhan and Assefa ( 2004 ), Yeshitela and Shibru ( 2004 ), Ersado and Assefa ( 2004 ), Bekele et al. ( 2004 ), Senbeta et al. ( 2005 ), Denu ( 2006 ), Senbeta ( 2006 ), Schmitt ( 2006 ), Senbeta et al. ( 2007 ), Kelbessa and Soromessa ( 2008 ), Hundera and Deboch ( 2008 ), Hundera and Gadissa ( 2008 ), Woldemariam et al. ( 2008 ); Nune ( 2008 ), Gobeze et al.( 2009 ), Schmitt et al. ( 2010 ), Friis et al. ( 2011 ), Assefa et al. ( 2013 ), Hylander et al.( 2013a , b ), Soromessa and Kelbessa ( 2013 ), Gebrehiwot and Hundera ( 2014 ), Mulugeta et al. ( 2015 ), Addi et al. ( 2016 ), Siraj et al. ( 2017 ), Yasin et al. ( 2018 ), Denu ( 2019 ), Addi et al. ( 2020 ), Dibaba et al. ( 2022 ). Despite these and many other related studies, the area still faces the challenge to conserve its vegetation and is becoming among the highly-threatened ecosystems in Ethiopia. To protect these fragile ecosystems through designing and successfully implementing more appropriate practices it primerly requires complete inventory on the floristic composition and diversity of plants in the area so as to represent ecological changes that may occur over years (Kneeshaw et al. 2000 ; Zumeta and Ellefson 2000 ; Adams and Hulme 2001 ; Larsson and Danell 2001 ; Bekele 2004 ; Schmitt et al. 2010 ). Thus, it would be imperative to conduct further floristic studies for each patch of the SW forest particularly in places that had not been addressed previously.
Literature shows that most of the floristic studies conducted so far in the moist southwest forest are concentrated on the forest fragments of Jimma Zone (from Oromia regional state), Keffa, Sheka, and Benchi Maji Zone (from the South Nation Nationalities and People Regional, (SNNPR) state and Godere District Forest (from Gambella regional state). Studies on the moist forests of Illuababora Zone (Oromia regional state) are very scanty although they largely contribute to the total forest coverage of the SW forest. With this drive, Sele-Nono Forest, which is the largest intact natural forest in Illuababora Zone, was entertained in this study with emphasis on the diversity and community analysis of plants as such information provides the baseline data about the current status of the forest.
Statement of the problem
Sele-Nono forest is registered as one of the forest priority areas of the country which falls within the boundaries of Sele-Anderacha national forest priority areas (NFPAs) (EFAP 1994 ) even if its botanical wealth was not thoroughly assessed by botanists. Moreover, the forest is believed to be one of the few jungle forests in the country with only 5% of it occupied by non-forest land; and hence studying this area would help to design proactive conservation strategies before the area is getting seriously threatened by human impacts as it is economically, climatically and ecologically sensitive. On top of this, the area is a remarkable natural laboratory to investigate the ecological relationships among the vegetation and environment (ecological factors) so as to have a better understanding of species diversity, which is a key parameter to develop appropriate forest conservation measures. Hence, this study was initiated for all these purposes.
General objective
The general objective of this study aims at understanding the plant diversity and community analysis of the Sele-Nono forest.
Specific objectives
The specific objectives were (i) to describe plant community types of Sele-Nono forest and their ecological relationships with some environmental factors (ii) to assess the extent of plant diversity within and along the described plant community types (iii) to identify and rank the existing threats of the forest and recommend possible management directions to prevent from quick rupture.
Material and methods
Description of the study area.
The study was carried out in the Sele-Nono forest, Southwest Ethiopia (between 7 o 38 ′ –8 o 10′N and 34 o 56 ′ –35 o 26′E). It is located in the Sele-Nono District of Illuababora Zone of Oromia Regional State (Zone is an administrative unit in Ethiopia). The district has a total area of 260,000 ha, of which 95% of it is covered by natural forest and it is divided into twenty administrative Kebeles, the smallest administrative units, namely Arbe, Asendabo, Bontu Korma, Decha, Derba, Derbeta, Gemeches, Haro, Kimo, Kombolcha, Kopi, Nono Berbersa, Onose, Qoti, Selase, Sochoso, Tupi, Waka, Welketesa and Yakema. The study area is characterized by undulating terrain with an altitude range of 840 m asl to 2448 m asl. The climate is monsoon-like (i.e., tropical moist climate type with heavy summer rains) with a mean annual precipitation of 1620 mm (concentrated March to December) and a mean annual temperature of 21 °C (Asres 1996 ). According to the Sele-Nono District Agricultural Organization (SNDAO) soils of the area mainly belong to nitosol group with a pH range from 6.4 to 6.6 (SNDAO 2015 ). The vegetation of the area falls into the transitional rainforest and Afromontane rainforest types (Friis 1992 ). The dominant vegetation in the transitional rainforest of the area includes Pouteria atissima, Anthocleista schweinfurthii, Manilkara butugi, Morus mesozygia, Strychnos mitis, Trichilia dregeana, and Trilepisium madascariense ; whereas Pouteria (Aningeria)-adolfi-friederici, Syzygium guineense, Olea welwitschii, Schefflera abyssinica, Croton macrostachyus, Ilex mitis and the likes are very dominant to the Afromontane forest part of the study area (Friis et al. 2011 ). The studied forest is bordered on the Southwest by Gambela region, on the North by Bure, on the Northeast by Halu, and on the Southeast by the Southern Nations, Nationalities, and Peoples Region (SNNPR) (Fig. 1 ).
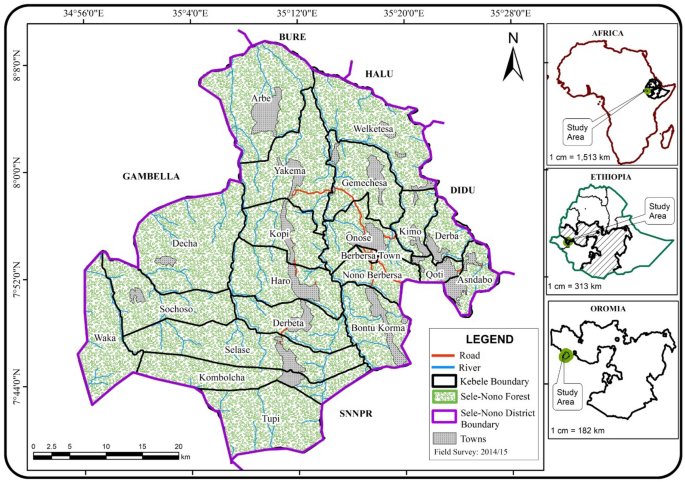
Map of Africa and Ethiopia showing the study area
Research methods
Sampling design.
Preliminary field investigations at Sele-Nono forest in March 2013 have enabled the researcher to employ a stratified random sampling technique for the study. For this purpose, the study area was initially developed using the Digital altitude model (DEM) technique extracted from SRTM (Shuttle Radar Topography Mission) database which has approximately 30 m spatial resolution (Farr and Kobrick 2000 ). Then it was stratified into six strata following the maximum recommended strata number suggested by MacDicken ( 1997 ) based on 275 m altitude differences, and sampling plots were allocated proportionally along with each stratum (Table 1 below).
The allocation of sampling points was done by making geographical grids on the map of the area before going to the field. The grids were made by dividing the map of the study forest in latitude and longitude coordinate using a one-minute (1’) grid-scale, which is equivalent to 1.85 km actual distance on the ground. Then some of the intersection points of the grids from each stratum were randomly chosen as a sample point (sample plot) using the lottery method, and plot numbers were given randomly to each of the sampling points using the same lottery technique (Additional file 1 : Appendix S1). Those plot locations on the map (Fig. 2 ) were placed on the ground of the actual study area using GPS navigation after ridding to the nearest town. Sampling was conducted after checking any category of natural or semi-natural vegetation in the stratum. Agricultural fields and plantations were not sampled.
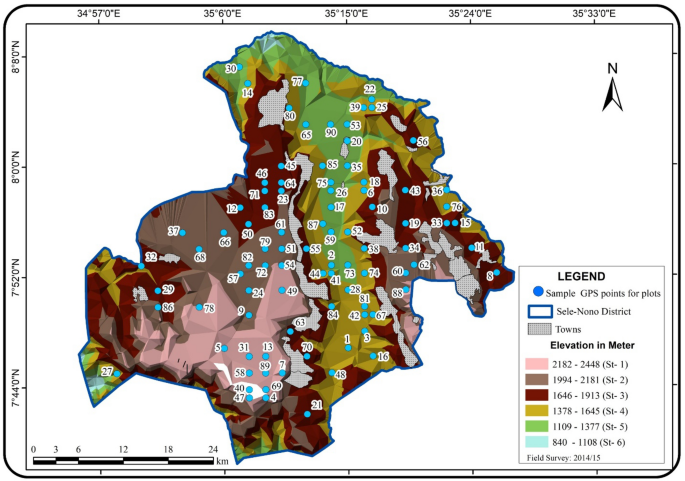
Location of permanent sample plots in the study area. Numbers on the map are plot numbers
Vegetation survey
Field data on the vegetation at Sele-Nono forest was carried out following the releve approach (Braun-Blanquet 1932 ; Van der Maarel 1979 ; Podani 2006 ) from January 2014 to December 2016 escaping the field activities each year between the mid-March to mid-October because of heavy rain on the area. Considering the size of the forest, variation of vegetation, and environmental factors a total of 90 (25 m by 25 m) permanent plots were established in the study area. Plot size was determined using the published table by Van der Maarel ( 2005 ) and based on previous experience from other SW forests of Ethiopia since the minimum area method was difficult to map in the study area. The practical use of a minimum area curve for plot size determination was also criticized for moist tropical forests (Kent and Coker 1992 ; Van der Maarel 2005 ). The 25 m by 25 m plot size was adopted in this study as this size is a trade-off between the 20 m by 20 m (Woldemariam et al. 2002 ; Senbeta et al. 2014 ) and 30 m by 30 m (Kelbessa and Soromessa 2008 ; Mulugeta et al. 2015 ) plot size, which were the most widely used plot sizes for floristic study in SW forests of Ethiopia. All woody individuals with DBH > 2.5 cm and rooted within the quadrat were recorded in each plot. Canopy cover (the percentage of ground area covered or shaded) of all trees (> 1.5 m in height) were assessed in the entire plot (25 m × 25 m), while shrubs were measured in 25 m 2 subplot designed in the center of the main plot. Cover of herbaceous plant species was surveyed in five 1m 2 quadrats within each sample area; four of which were arranged at the four corners of the main plot and one was at the center of the 25 m 2 subplot (Fig. 3 ). The cover-abundance for herbaceous species was estimated by calculating the average cover-abundance of each herb species existing in five subplots established inside a large quadrat. Then, the abundance and/or percentage cover estimates recorded in the field were converted into cover-abundance values (Additional file 2 : Appendix S2) according to 1–9 modified Braun-Blanquet ordinal scale (Van der Maarel 2005 ). Plant species that were not parts of the plots (for trees) or subplots (for shrubs and herbs) but encountered during our field walk were also collected and recorded only as ‘present’ to enrich the floristic composition of the study area; but not included further in the data analysis (Mueller-Dombois and Ellenberg 1974 ).
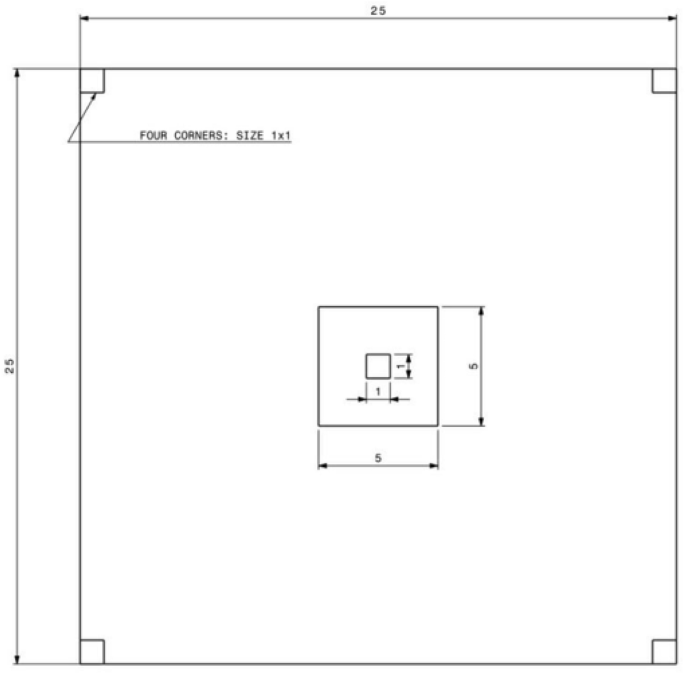
Design of plot layout in the study area (unit in meter, scale 1:600)
Environmental data collection
The environmental factors (altitude, slope, slope orientation (Aspect), disturbance, and soil characteristics) were determined for each of the main plots so as to explain possible differences in plant communities by their corresponding environmental factors. Disturbance on each plot was estimated subjectively on ordinal scales from zero to five following checklist developed for this study (Additional file 3 : Appendix S3). GPS was used to record the altitude and position (latitude and longitude) of the sampled plots. Compass and Clinometer were used to measure aspect and slope respectively. The aspect was codified according to Woldu et al. ( 1989 ). Composite soil samples were collected at a depth of 30 cm by pooling soil samples from all five subplots. These soil samples were air-dried; and analyzed for total nitrogen (N), available phosphorus (P), organic matter (OM), pH, Potassium (K), Calcium (Ca), Magnesium (Mg), Cation exchange capacity (CEC), and texture (sand, silt, and clay) at the National Soil Test Laboratory of Ethiopia.
Semi-structured interviews using checklists prepared in advance (Additional file 4 : Appendix S4) were made with the informants of the local people to understand the threats of the forest understudy. For this purpose, a total of 40 informants who have been born or have lived most of their lives in the study area were chosen. 30 of the informants (5 from each stratum) were randomly selected following Kefalew et al. ( 2015 ). The other 10 of the total informants were key informants who were believed to have immense knowledge of the past and present change of the forest; and they were selected based on the recommendation from the local people, local authorities, and development agents (DAs) in the study area.
Plant identification
Plant species that were easily identifiable were recorded on the field. Other species were temporarily stored in a plastic bag; and then pressed and brought to the National Herbarium (ETH) of Addis Ababa University (AAU) where they were dried, deep-frozen, and identified. The identifications were first performed using Natural database for Africa ( 2011 ) and keys of published volumes of Flora of Ethiopia and Eritrea (Edwards et al. 1995 ; Hedberg and Edwards 1989 ; Edwards et al. 1997 ; Edwards et al. 2000 ; Hedberg et al. 2006 ; Hedberg et al. 2009 ) and later supported with identification by comparisons with the already authenticated dried specimen in the Herbarium. At last, all the Latin names of the plant species were confirmed by taxonomic experts in Addis Ababa University (AAU).
Data analysis
A species accumulation curve (SAC) of the data was drawn to ensure that we had collected an adequate number of plots for the study using “library (vegan)” package in R (Oksanen et al . 2011 ) (Fig. 4 ).
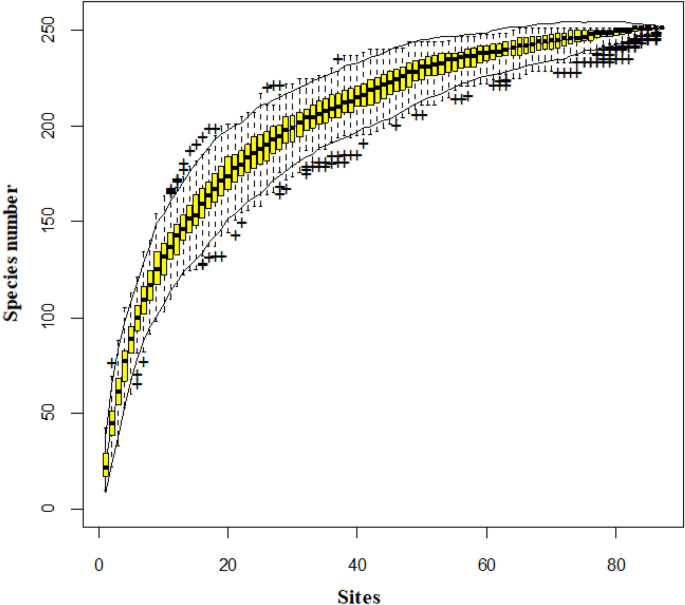
Species accumulation curve showing the relationship between the numbers of sites or quadrats and the number of plant species in Sele-Nono Forest
Agglomerative hierarchical cluster analysis using dendrogram was performed for recognizing and describing plant community types as this tool is the most recommended method of classification in synecology (Van der Mareel 2005). For this purpose, the optimum number of clusters (K) was specified first and foremost using the elbow method by employing a program developed for this purpose in R (R Development Core Team 2011 ). This method is a graphical representation of the total intra-cluster variation [i.e., total within group or cluster sum of square (WSS)] as a function of the number of clusters (Fig. 5 ); and the optimum cluster number is quickly judged by inspecting the elbow (first breakpoint or bend) of the plotted curve with the assumption that adding another cluster doesn’t improve much better the total WSS (Woldu 2017 ). Researchers on the area suggest this elbow method as a fairly clear method, if not a naive solution as it is based on intra-cluster variance (Chebaeva et al. 2020 ; Kongphunphin and Srivanit 2021 ; Nalli et al. 2021 ).
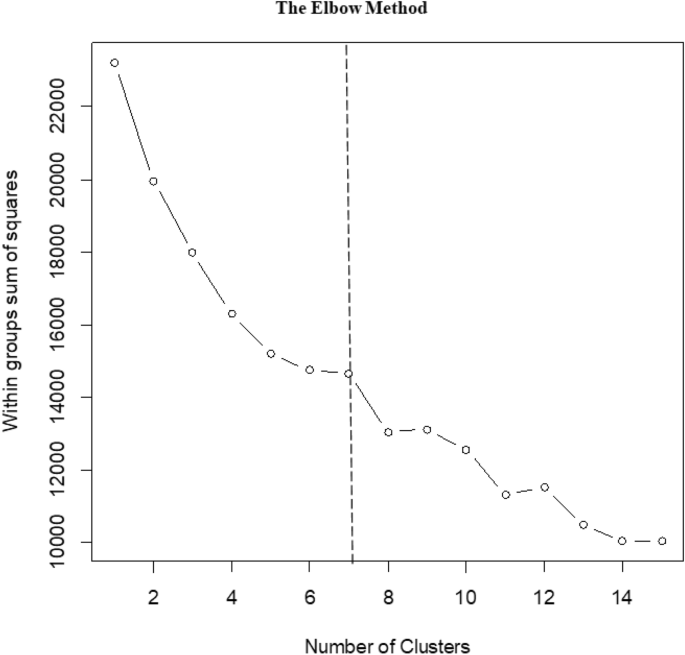
Determination of optimal number of clusters corresponding to plant community types in Sele-Nono forest (Diagram of the Elbow method shows the expected optimal number of clusters. Within group (Cluster) Sum of Squares values represent the sum of squared Euclidean distances between the plots and the centroid. The Elbow is the first breaking point, seen after the flattening of the curve, in our case, 7)
Then the dendrogram was performed using Euclidean distances with the ‘cluster’ and ‘gclus’ packages in R. Euclidean distances was also used in other studies to develop the dendrograms in cluster analysis (Senbeta 2006 ; Woldemariam et al. 2002 ; Kebede et al. 2013 ). The agglomerative strategy used in grouping the clusters was Ward’s method as it is the most recommended clustering strategy to produce reasonably compact clusters (McCune and Grace 2002 ; Woldu 2012 ). The cophenetic correlation coefficient, which is regarded as a criterion of successful classification, was calculated to infer the goodness of fit of the dendrogram. Synoptic table analysis was used to name and describe community types.
Outlier analysis was conducted to drop out rare species from the analysis (species which occur three or fewer times in the data matrix) using ‘the function dropspc in the library labsdv’ (Roberts 2010 ) as rare species are noise to the cluster analysis. The abundance data of the remaining plants were Hellinger transformed prior to analyses to reduce skewness (Woldu 2012 ; Aerts et al. 2006 ). The normality of the environmental variables was checked visually using histograms and with the Shapiro–Wilk normality test. Based on this analysis all the measured environmental variables except the altitude, slope, aspect, and sand data were log10 (x + 1) transformed as the transformation of variables would not affect the outcome of CCA. Mantel test was first applied to see if there were a significant correlation between the species composition and environmental variables (Legendre et al. 2015 ). Constrained ordination technique was then used to display the relative contribution of the measured environmental variables using canonical correspondence analysis (CCA) since a gradient length (GL) on a preliminary detrended correspondence analysis (DCA) showed that the species respond unimodal (GL of 4.07 > 4SD) to environmental gradients (Leps and Smilauer 2003 ). The environmental variables that were only significant in a forward selection procedure (Monte Carlo permutation tests, n = 999) were considered important in explaining the floristic composition of the study area and thus included on the CCA diagram. All data processing was performed using R (R Development Core Team 2011 ).
Plant diversity measures such as Shannon and Wiener ( 1949 ) index of species diversity (H’), richness (S), and evenness (J) were computed in R (R Development Core Team 2011 ) to analyze the status of plant diversity in the established plant community types (Kent and Coker 1992 ; McCune and Grace 2002 ); and to infer the impact of human being on the spatial distribution of the vegetation (Magurran 2004 ; Leitner and Turner 2001 ). Moreover, Whittaker alpha and beta diversity was calculated to measure the heterogeneity of plant species within the clusters. Whittaker beta diversity ( \({\beta }_{w}\) -diversity) was also performed to define the overall diversity of the plant species in the Sele-Nono forest along ecological gradients. Analysis of Variance (ANOVA) followed by post hoc test was also performed to evaluate the significance of variation in mean values of calculated diversities among the established plant community types using SPSS version 20.0 (Onaindia et al. 2004 ). Preference ranking on the major threats of the study area was done following Martin ( 1995 ) to screen and find out the key human impacts which are aggressively threatening the forest. Other qualitative information gathered through informant interviews was used to complement the quantitative analysis.
Floristic composition
A total of 418 vascular plant species representing 108 plant families (Additional file 5 : Appendix S5) were compiled in this study. This floristic list indicates that the Sele-Nono forest constitutes about 6% of the flora of Ethiopia and Eritrea. Out of these species, only 335 species were collected from the plots and the remaining 83 were collected from outside the plots. Although the Sele-Nono forest is characterized by a large number of families (108 families), the top fifteen families (Table 2 ) were the most important ones that contribute about half of the total species composition. Moreover, out of the total species recorded in this study 59 of them (14%) were new records to Illubabur (IL) floristic region in the flora of Ethiopia and Eritrea (Additional file 6 : Appendix S6).
Among the total plant species documented from the forest, 225 (54%) of them were herbs (including ferns and climbers), 120 (29%) were trees, 26 (6%) were lianas and 47 (11%) were shrubs (Fig. 6 ). Field observation in the study area also revealed the large abundance of lianas throughout the forest although their species richness accounts only for 6% of the total floristic composition of the area. Of the total plant species compiled in this study, about 25 (6%) of them were endemics to Ethiopia (Kelbessa et al. 1992 ), and some of these are with some level of IUCN threat category (Vivero et al. 2005 , 2006 ) (Additional file 7 : Appendix S7).
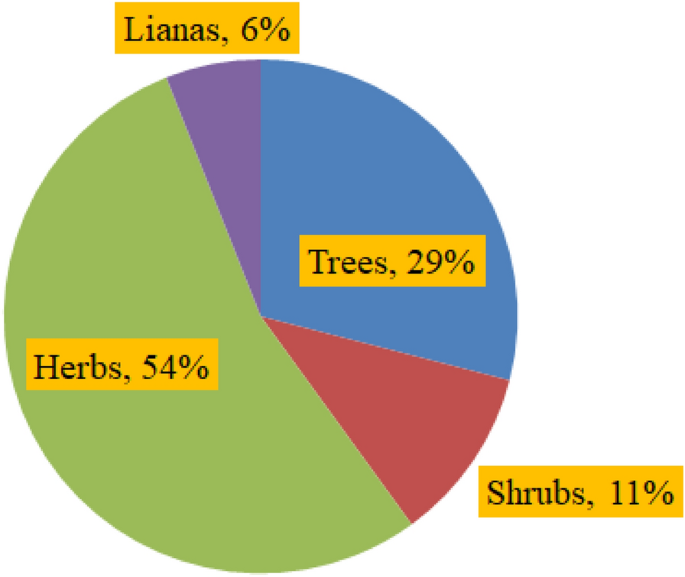
Growth forms of plant species collected in Sele-Nono forest
- Cluster analysis
Outlier analysis excluded eight species leaving 327 species for the analysis (Additional file 8 : Appendix S8). Using agglomerative clustering we were able to visualize 7 clusters in the dendrogram corresponding to 7 plant community types or “associations” that are expected to be naturally occurring in the study area (Fig. 7 ). The dendrogram was proved to include more homogenous plots (i.e., plots of high internal similarity with respect to species) in the same cluster (Cophentic correlation coefficient = 0.785, P < 0.001).
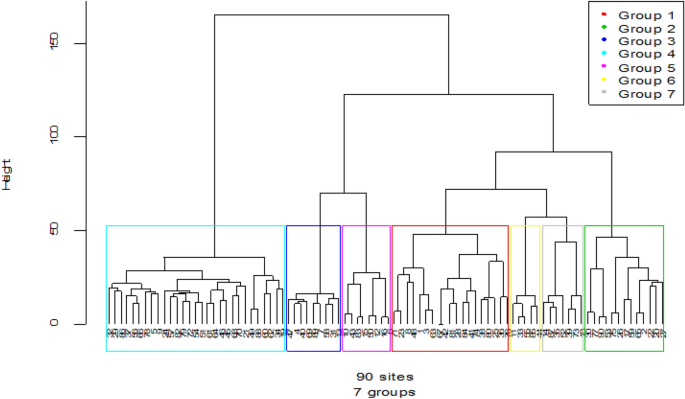
Dendrogram of the vegetation data obtained from hierarchical cluster analysis of Sele-Nono forest using Ward’s method and Euclidean distance (the term ‘Group’ on the legend is synonymous to clusters or plant community types established for the study area, and represented here as C1 to C7 where plots in each community types are presented within the parenthesis as follow: C1(71,23,8,48,1,3,63,67,42,81,28,84,41,74,38,80,25,36,76), C2(30,77,90,53,75,26,17,59,65,22,2,20,27), C3(47,4,40,69,89,70,58,31,13), C4(32,29,86,37,56,66,78,5,9,24,57,82,79,72,54,51,61,64,46,45,68,70,21,49,88,60,62,34,19), C5(10,43,83,15,50,12,16,6), C6 (11,33,55,86,44), C7 (14,87,35,52,39,73,18)
Classification of plant communities in Sele-Nono forest
Seven plant community types were described in the study area and named using two indicators and/or character species identified from synoptic table analysis (Additional file 9 : Appendix S9). Indicator species are diagnostic species that are faithful to one community type only; whereas character species are diagnostic species whose mean cover is high in the cluster type under consideration and relatively low in other types (Kent and Coker 1992 ; Kindt et al. 2011 ). The use of two indicators and/or character species is the most common approach for naming and describing plant association types (Bekele 1993 , 1994 ; Woldu 1999 ; Soromessa et al. 2004 ). The result of synoptic value shows that some of the species are indicator species as they are only faithful to one of the plant community types under consideration and not found in other types. However, many other species occur over wide altitudinal ranges or community types showing a continuous variation of vegetation; but are most dominant in terms of their mean cover within only one of the cluster types.
Accordingly, community one is named as Olea welwitschii — Elaeodendron buchananii community type, and mainly includes the vegetation within the altitude of 1600–1900 m asl in Sele-Nono forest. This community type is often located on steep topographies and spatially different localities such as MOLAYE, YEBECHA, WANGES (all in Onose Kebele), GAGO (in Gemechesa Kebele), QETO (in Bontu-Korma Kebele); KIMO and NONO-BERBERSA Kebele forests; and river lines of CHETA, GURACHA, and SEJA. Representative woody species in this community includes Acanthus eminens, Bersama abyssinica, Clausena anisata, Cordia africana, Dracaena steudneri, Embelia schimperi, Erythrococca trichogyne, Hallea rubrostipulata, Isoglossa somalensis, Lobelia giberroa, Maesa lanceolata, Macaranga capensis, Maytenus gracilipes, Millettia ferruginea, Pittosporum viridiflorum, Rytignia neglecta, Sapium ellipticum, Teclea noblis, Vepris dainellii . Herbaceous species in this community includes Achyranthes aspera, Aframomum corrorima, Desmodium repandum, Hypoestes triflora, Hypoestes forskaolii, Panicum spp, Paullina pinnata, Piper capensis, Tiliacora troupinii, Salvia nilotica .
Community two is Alstonia boonei– Manilkara butugi community type, and often found on the extreme low land of the study area between an altitude ranges of 840–1250 m asl. This community type is often located on gentle terrains of WAKA, ARBE, KOPI, YAKEMA, GEMECHESA WELKKETESA Kebele forests on their lowland extremes. Common woody membership species in this community type includes Acalypha ornate, Anthocleista schwinfurthii, Allophylus macrobotrys, Alstonia boonei, Argomuellera macrophylla, Baphia abyssinica, Celtis zenkeri, Dracaena fragrans, Ficus sycomorus, Garcinia buchananii, Garcinia ovalifolia, Lecaniodiscus fraxinifolius, Manilkara butugi, Milicia excels, Pouteria alnifolia, Pouteria altissima, Saba comorensis, Whitfieldia elongate . Some of the herbaceous species common in this plant community types include Ageratum conyzoides, Achyrospermum schimperi, Phaulopsis imbricata, Panicum atrosanguineum, Isodon schimperi, Plantago palmate, Guizotia scabra, impatiens hochstetteri, Stictocardia beraviensis . Field observation by the authors reveal that this community is disturbed prevalently by the conversion of forest land to cropland (Maize, Enset ventricosum, Colocasea esculenta) through anthropogenic fire. Moreover, it was evident that these areas were heavily disturbed by anthropogenic activities such as charcoaling, logging, the gathering of Dioscorea roots, and the likes. Oral communication with elder people, who were born and live in the area, indicates that the size of the forest area becomes highly diminished than before.
Community three is Arundinaria alpina– Oplismenus hirtellus community type and is found in the uppermost altitudes of the study sites within altitudes of 2300-2448 m asl. It covers the uplands of TUPI and KOMBOLCHA Kebele forest in the study area. Though it is mainly dominated by one species, Arundinaria alpina , it still contains some dominant species, which include Allophylus abyssinicus, Bersama abyssinica, Dracaena afromontana, Galiniera saxifrage, Polyscias fulva, Prunus africana, Schefflera myriantha, Schefflera volkensii, Syzygium guineense subsp. afromontanum, . Herbaceous species include Amauropelta bergiana, Athyrium schimperi, Ajuga integrifolia, Blotiella glabra, Cyathula polycephala, Thunbergia alata, Veronica abyssinica.
Community four is Pouteria adolfi-friederici–Dracaena afromontana community type, and mainly observed between altitude ranges of 1850–2300 m asl in the study area. It covers most parts of the study area such as the forests of DECHA, SOCHOSO, SELASE, DERBETA, and the highlands of WAKA, HARO, KOPI, YAKEMA, BONTU KORMA and GOROSO forest. Common species in this community includes Acanthopale ethio-germanica, Asystasia gangetica, Barleria ventricosa, Albizia gummifera, Allophylus abyssinicus, Brillantaisia madagascariensis, Clausena anisata, Combretum paniculatum, Cyathea manniana, Dalbergia lacteal, Deinbollia kilimandischarica, Embelia schimperi, Galiniera saxifraga, Hippocratea africana, Ilex mitis, Lepidotrichilia volkensii, Maytenus spp., Olea capensis, Oxyanthus speciosus, Pittosporum viridiflorum, Plectranthus garckeanus, Pouteria adolfi-friederici, Rothmannia urcelliformis, Rytignia neglecta, Ruellia prostrate, Teclea nobilis, Vepris dainellii, Schefflera abyssinica, Syzygium guineense . Common herbaceous species included in this community types include Asplenium sandersonii, Arthropteris monocarpa, Celosia argentea, Crassocephalum macropappurm, Desmodium repandum, Dissotis senegambiensis, Elatostemma monticolum, Habenaria holubii, Impatiens ethiopica, Lycopodium c1avatum, Oplismenus hirtellus, Scadox nutans , Thalictrum rhynchocarpum, Tristemma mauritianum, and many others. Interview with District leaders and key informants reveal that these highland forests have been called Kobo forests for honey production by the local people. The Kobo system is a traditional forest management practice in the study area that gives the local people a sense of ownership for a certain block of forests demarcated by big trees and/or other physical features like rivers and small streams and exclusively used for hanging beehives and hunting to sustain their livelihoods.
Community five is Cyperus longibracteatus- Cyperus dereilema community type, and it refers to the vegetation predominantly found on fragile wetlands and other pocket swamp bodies. These are permanently or seasonally flooded areas dominated by grasses and/or sedges between 1640 and 1850 m asl. This community is mainly found in the wetlands (and/or grassland during the dry season) of GOROSO, KIMO, QOTI, ASENDABO, TUPI, GEMECHESA, WELKETESA, and the like. Although the primary vegetation in this community is tall grasses, sedges and ferns, scattered woody species are also notable. The major vascular plant species that characterize the wetland habitat include Cyperus dereilema, Floscopa glomerata, Panicum calvum, Pennisetum macroururn, Pennisetum trachyphyllum, Persicaria setosula, Rumex nepalensis, Snowdenia polystachya, Sporobolus pyramidalis . The grasses grown in this community type are a common source of livestock feed and thatches, and field observation also proved free grazing on these habitats particularly during the dry season as a threat to this community type.
Community six is Coffee arabica- Trichilia dregeana community type, and mainly found within the altitude range of 1600–1800 m asl. It is dominantly observed in ASENDABO, KIMO, DERBA, GEMECHISA, WELKETESA, YAKEMA, KOPI, and the likes. The main plants included in this community are Apodytes dimidiate, Bridelia micranta, Brucea antidysnterica, Cassipourea malosana, Coffea arabica, Croton macrostachyus, Dracaena steudneri, Ehretia cymosa, Erythrococca trichogyne, Euphorbia ampliphylla, Ficus exasperate, Ficus thonningii, Ficus vasta, Ficus ovata, Ficus sur, Hippocratea goetzei, Hippocratea africana, Nuxia congesta, Oncinotis tenuiloba, Paullinia pinnata, Psidium guajava, Polysicas fulva, Ritchiea albersii, Sapium ellepticum, Senna petersiana, Tiliacora troupinii, Trema orientalis, Trichilia dregeana . Field observation showed that this community type is more vulnerable due to forest management (thinning the understory shrubs, logging canopy trees, etc.) for increasing the production of coffee beans for the purpose of household consumption. Moreover, informal interview with local elders showed that most local people prefer this area for the expansion of smallholder coffee agriculture as these areas are average between the highland (where there are not many natural coffee plants) and lowlands (where there is much coffee but unconducive area for a living). As a result, many of them have coffee plots in the forests where they collect coffee beans for selling to subsidize their livelihood. On top of this, expansion of settlements and recent development in road construction in this community type is a major threat to the diversity and richness of plants. As a result, the physiognomy of vegetation in this community is not characterized by continuous cover spacing; rather plants are widely spaced out.
Plant community seven is Trilepisium madagascariense — Morus mesozygia community type and is found at altitudes between 1200 and 1500 m, asl along with the courses of Baro, Genji, and Guracha rivers. Common woody species in this community types includes Acalypha ornate, Albizia grandibractiata, Alblzia schimperiana, Anthocleista schweinfurthii, Argomuellera macrophylla, Baphia abyssinica, Celtis philippensis, Celtis gomphophylla, Cissampelos mucronata, Dioscorea praehensilis, Diospyros abyssinica, Dracaena fragrans, Ficus exasperata, Ficus sycomorus, Gouania longispicta, Justicia bizuneshiae, Justicia ladanoides, Mimosopes kummel, Morus mesozygia, Phoenix reclinata, Strychnos mitis, Trichilia dregeana, Trilepisium madagascariense, Turraea holstii. Common herbaceous species includes Ceropegia cufodontis, Eulophia guineensis, Marantochloa leucantha, Oryra latifolia, Setaria megaphylla.
Diversity analysis
Diversity analysis in vegetation ecology is an important tool to measure or infer the effect of human activity (disturbance) on plant diversity. Accordingly, diversity analysis (species diversity, richness, and evenness) for each cluster was calculated using R as shown in Table 3 . Moreover, Whittaker’s alpha (α) and beta ( \({\beta }_{w}\) ) diversity were also calculated for each cluster (Whittaker 1972 ; Magurran 1988 ) so as to measure the degree of heterogeneity of species composition. Alpha diversity (α-diversity) was calculated as the average species richness per plot (i.e., species density). Beta ( \({\beta }_{w}\) ) diversity was calculated on the basis of the ratio of the total number of species to the average number of species.
Based on the result of the data (Table 3 ), it can be said that cluster 4 is a cluster with high Shannon diversity (H = 4.36) of plant species and is found on the uppermost altitudes of the study area before reaching the bamboo zone. The higher the values of Shannon evenness (J), the more even the plant species are in their distribution within the cluster. Thus, clusters 5, 6 and 7 have a more even distribution of individuals among various species in each cluster (J > 0.90) than the other clusters.
As far as species richness and beta diversity is concerned it can be noted from the above table (Table 3 ) that they are strongly and positively correlated with the number of plots in the communities (Pearson correlation coefficient, r = 0.92). Similarly, there was positive correlation between plot numbers and species diversity (H) (r = 0.75), and strong negative correlation with eveness (J) (r = − 0.95).
On the basis of species richness, cluster 4 ( Pouteria adolfi-friederici– Dracaena afromontana community type) and cluster 1 ( Elaeodendron buchananii- Olea welwitschii community type) contain a large number of species than the other clusters. Actual field observation during the study period revealed that community type 6 is a major coffee growing area and hence most local people prefer it for settlements so as to subsidize their livings; whereas community 5 (pocket wetlands/flooded grasslands) is mainly serving as grazing land for animals particularly during the dry season. Community 3 (the bamboo zone) is the peak and wettest place in the study area.
From the above Whittaker beta diversity analysis (Table 3 ), it can be seen that the degree of variation of vegetation turnover along the plots in each cluster is not so high ( \({\beta }_{w}\) <5) except for cluster 4 ( \({\beta }_{w}\) >5); whereas too low beta diversity was obtained for cluster 6, which is the species-poor plant community type. Whittaker’s beta diversity ( \({\beta }_{w}\) -diversity) index was also calculated in R to assess the overall heterogeneity of plant species in the study area. The result indicates that there is high beta diversity ( \({\beta }_{w}\) = 12.04) of plant species across the community types in the Sele-Nono forest, which leads to the conclusion that each plant community type has a heterogeneous species composition.
Variation on the relative diversity of plants in Sele-Nono forest
Initial analysis of variance (ANOVA) using “aov” approach in R showed that there is a significant difference in diversity such as in species diversity (F = 10.89, P < 0.05), richness (F = 9.83, P < 0.05), and evenness (F = 3.46, P < 0.05) between the plant community types (Fig. 8 ). ANOVA was performed after checking the assumptions of linearity using QQ plot and Shapiro–Wilk normality test (P < 0.05) and homogeneity of variances using Levene statistic (P > 0.05).
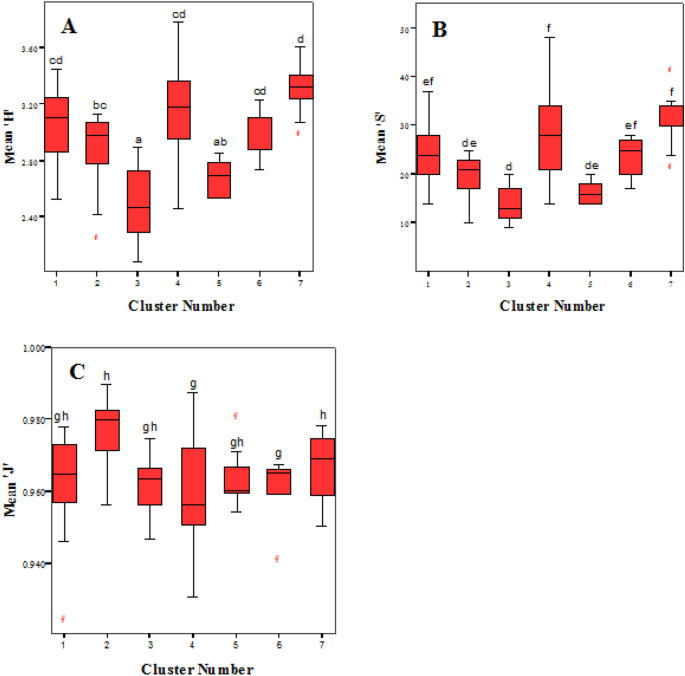
Mean values of plant diversity per plot for the different clusters in the study area. A Variation of mean species diversity. B Variation of mean species richness. C Variation of mean species evenness between the clusters. Boxes represented by the same letters are not significantly different at P < 0.05
From the above figures (Fig. 8 ), it can be revealed that mean species diversity was significantly higher at clusters 1, 4 and 7; and lower at cluster 3 (the community with the alpine bamboo thicket) and cluster 5 (the community with a high level of grazing intensity). Similarly, a significantly large number of species per plot were found in cluster 4 and 7; and cluster 3and 5 has significantly less number of species. Regarding species evenness, significantly high species evenness per plot was revealed at cluster 2 and while significantly low evenness was observed at cluster 4.
Influence of environmental factors on the variation in species composition of plants
The result in this study shows that there is a significant correlation between the species composition and environmental variables (Mantel statistic: r = 0.64, P = 0.001). from the environmental data collected for this study (Additional file 10 : Appendix S10), a forward selection procedure (Monte Carlo permutation tests, n = 999) screened out the following environmental variables (Table 4 ) to be more responsible (P < 0.05) for the distribution of plants and structuring their community composition in the study area. These environmental variables were able to explain 12.5% of the variability in the floristic data as can be understood from the inertia or variance represented by Chisq (Table 4 ).
The correlation of these environmental factors with themselves and their influence on the distribution of vegetation in the study area was projected as follows using the Canonical Correspondence Analysis (CCA) technique (Fig. 9 ).
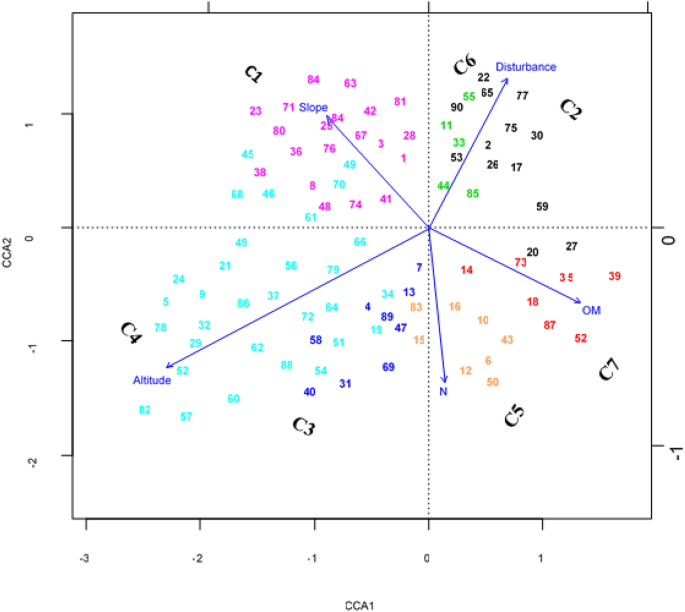
CCA showing the relationship of the environmental factors among themselves and on the distribution of plants in the study area (Numbers inside the ordination diagrams represents plot numbers; and C denotes community number; arrows representing environmental variables; and arrow length shows the strength of the environmental variable)
The first and second ordination axis of the CCA explained 35.51% and 26.08% of the constrained variation respectively, leading to capture and explain a cumulative of 61.59% of the constained inertia. From the above ordination diagram (Fig. 9 ) it can be seen that altitude was strongly associated with species composition as indicated by the long arrow in the ordination diagram indicating that it is the main environmental factor responsible for the spatial variation of vegetation in the study area. The plant communities that are distributed in the higher altitude of the study area (C1, C3 andC4), which all together form part of Afromontane forest were ordinated to the left side of the diagram; whereas the clusters that are distributed to the lower altitude (C2 and C7), which altogether forms part of the transitional rainforest were ordinated at the positive end of axis 1. A negative correlation between altitude and disturbance was also evident from the ordination diagram. Vegetation of the swampy areas (C5) has a direct relationship with the nitrogen content of the soil, whereas Morus mesozygia—Trilepisium madagascariense community type (C7) is mainly constrained by the high organic matter content of the soil in the area.
Threat to vegetation of Sele-Nono forest
The semi-structured interview conducted with the informants reveals that the forest vegetation of the study area is depleting from time to time basically due to deforestation and forest degradation. The following are common reasons summarized through the interview,
Expansion of Agricultural lands (conversion of forest land use to farming land)
Expansion of settlements/urban boundaries due to population growth
Fire (causing a wide distraction of vegetation and affecting the regeneration. It is often set deliberately either for farm clearing, or driving out bees during honey collection from the traditional beehives or may get out of control. Sometimes by smokers leaving butts of cigarettes unextinguished in the forest)
Over-grazing particularly for herbaceous plants (because it hinders regenerations, decreases species diversity, and if it is heavily grazed it paves ways for erosion by wind, water etc.)
Clearing of forests for wide and far-reaching road constructions
Thinning of understorey shrubs other than Coffea for improving coffee bean productivity (i. e., conversion of “forest-coffee systems” into “semi-forest coffee systems” as defined by Woldemariam 2003 )
Selective removal of trees and/shrubs for construction purposes, timbering, charcoal production, harvesting of fuelwood, etc. Some of the species frequently preferred for logging by the local people include Pouteria adolfifriederici, Apodytes dimidiate, Celtis africana, Cordia africana, Croton macrostachyus, Ekebergia capensis, Prunus africana, Olea welwitschii, Polyscias fulva and Syzygium guineense . These logging practices have been reported to exist in the study forest since the time that dates back beyond the knowledge of the local people. The current participatory forest management (PFM) approach practicing in the study area was reported by clan leaders at least in limiting the uncontrolled logging.
Moreover, actual field observation (Fig. 10 ) supported the interview result.
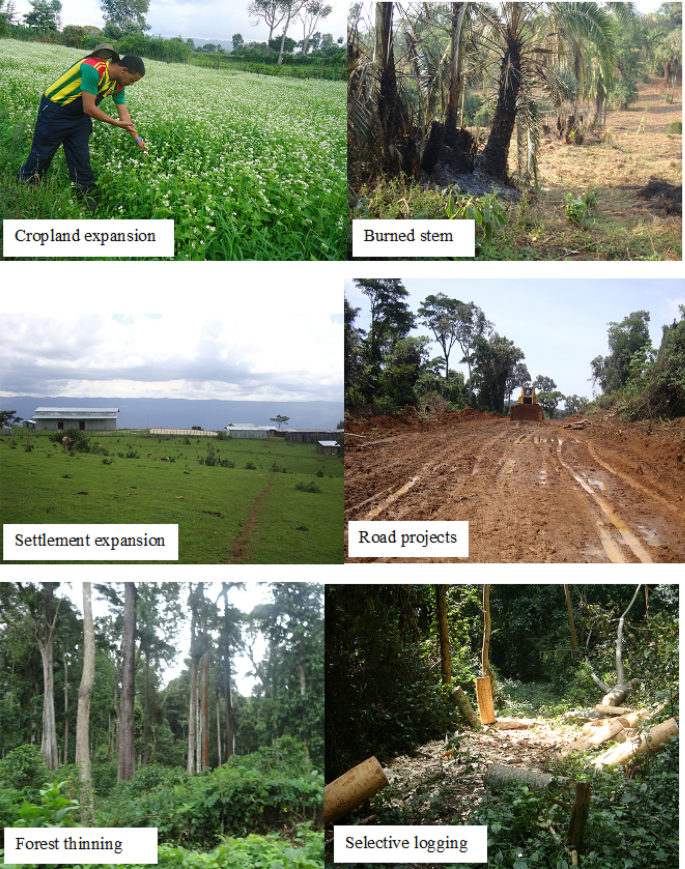
Common threat to the vegetation of Sele-Nono Forest
To indicate the above listing of environmental degradation in order of their impact, preference ranking was applied by taking ten key informants following Martin ( 1995 ) (Table 5 ).
From the above table (Table 5 ) it can be seen that the study forest is primarily threatened by forest clearing for agriculture purposes and forest degradation through selective removals of woody species and forest thinning practices for optimizing coffee bean production. Moreover, settlement expansion or population increment and road projects are also notable threats to the Sele-Nono forest. Overgrazing and fire were found to be the least serious threat to the plant biodiversity of the Sele-Nono forest.
Floristic composition of Sele-Nono Forest
It is frequently claimed that understanding floristic composition is the primary phase for the management of forest ecosystems (Kneeshaw et al. 2000 ; Simberloff 2001 ; Sanjit and Bhatt 2005 ; Ssegawa and Nkuutu 2006 ; Negesse and Woldearegay 2022 ). In agreement with this sentiment, this study showed that the Sele-Nono forest sheltered a large number of vascular plants (418 species) falling into diverse plant families (108 families) and considerable growth forms (herbs, shrubs, lianas, and trees). The finding of this study also demonstrates that the Sele-Nono forest contains species for moist montane on the highlands plant community types (C1 and C4) and transitional rainforest characteristic species (Friis et al. 2011 ) on the lowland plant community types (C2 and C7) suggesting that Sele-Nono forest belongs to moist montane and transitional rain forest, which had not been included on the vegetation map of Ethiopia developed by Friis ( 1992 ).
The finding of this study also revealed that the Sele-Nono forest contains more species than the species reported for Bonga forest (220 species) by Schmitt et al. ( 2010 ), Gura Ferda forest (196 species) by Denu ( 2006 ), Masha forest (130 species) by Assefa et al. ( 2013 ), Yayu forest (220 species) by Woldemariam et al. ( 2002 ), Sheko forest (374 species) by Senbeta et al. ( 2007 ), Gesha and Sayilem forest (300 species) by Addi et al. ( 2020 ), Gerba Dima forest (180 species) by Dibaba et al. ( 2022 ). This indicates that the current study area is one of the richest forest ecosystems in the southwest forest of Ethiopia. This may be attributed to its largness in size (about 150,000 ha forest area) that goes with the ecological concept of “largness and singularity” in the theory of island biogeography which focuses on the overarching importance of single but large natural reserves or habitates to harbor more number of species than if it would have been divided into several small natural habitates with the same total area (Diamond 1975 ; Whittaker and Fernandez—Palacios 2007 ; Triantis and Bhagwat 2011 ; Wilson and MacArthur 2016 ). The large number of species records in Sele-Nono may also be attributed to prsecence of relatively less anthropogenic pressure to the forest due to low population number (CSA 2007 ) and inaccessible roads.
The richest plant families reported in the current study area are Asteraceae, Fabaceae, Acanthaceae, Euphorbiaceae, Rubiaceae, and the like. Most of these families are also among the top families in the flora of Ethiopia and Eritrea (Kelbessa et al. 1992 ) in general and in the different vegetation patches of the moist southwest forests in particular (Yeshitela and Bekele 2002 , Woldemariam 2003 , Yeshitela and Bekele 2003 ; Senbeta et al. 2005 ; Kelbessa and Soromessa 2008 ; Addi et al. 2020 ; Dibaba et al. 2022 ). The prevalence of the family Asteraceae in the study area indicate that the forest might have been under a certain level of disturbance as plants of the Asteraceae family are often ruderal (early colonizers); and normally prefer open and disturbed lands to grow (Malcolm 1994 ; Turner 1996 ; Tadesse 2004 ; Awoke et al. 2022 ).
This study also reports the presence of a large number of trees and shrubs next to herbs. This may suggest that there was less selective utilization of tree species as opposed to some other forest, for instance, the Bishan Gari/Kimphee natural reserve in Southern Ethiopia (Senbeta and Teketay 2003 ). Moreover, the higher floristic composition of the forest floor may be a consequence of low grazing intensity in the forest (Onaindia et al. 2004 ). The occurrence of a large proportion of herbs is a common phenomenon in other moist forests of Ethiopia (Addi et al. 2016 ; Senbeta et al. 2007 ). Moreover, the relatively high number of liana species in the forest may indicate that there have been encroachments followed by continuous disturbances in the area (Putz 1984 ; Villagra et al. 2013 ). The presence of high numbers of lianas has also been noted elsewhere in the moist southwest forests of Ethiopia (Senbeta et al. 2005 ) and elsewhere in moist forests of Africa (Girma 2011 ; Addo-Fordjour et al. 2013 ; Uwalaka et al. 2021 ).
The finding in this study also reported that about 6% of the total plant species compiled in this study are endemics to Ethiopia (Additional file 2 : Appendix S2). The restriction of these taxa is probably a consequence of limited dispersal ability. The low endemicity of plants is a common phenomenon for the vegetation of the moist SW forest of Ethiopia in particular (Friis 2009 ) and for the vegetation of the country at large (Kelbessa et al. 1992 ).
Community types and diversity of plants in Sele-Nono forest
For the purpose of plant biodiversity assessments of a forest ecosystem, ecologists often recommend studying it at a certain finest floristic unit so as to draw a better picture of the richness and diversity of plant species of an area (Mueller-Dombois and Ellenberg 1974 ; Kent and Coker 1992 ). In line with this sentiment, this study established and described seven plant community types. None of these community types were poor with respect to vascular plant diversity (H > 1.5). According to Kent and Coker ( 1992 ), the optimal value of the Shannon diversity Index (H) lies between 1.5 and 3.5. This diversity of vascular plant species is comparable with other moist forests of Ethiopia (Nigatu and Tadesse 1989 ; Lulekal et al. 2008 ; Gurmessa et al. 2013 ; Kebede et al. 2013 ).
Among the community types described in this study community types 1 and 4, which are mainly represented by the moist evergreen Afromontane forest (MAF) parts of the study area, had relatively high species diversity and richness of plants. The high diversity and richness of plants in the moist evergreen Afromontane forest of Ethiopia were also reported by Friis ( 2011 ). One possible reason for having a high richness and diversity of plants for these community types in the current study area could be a consequence of the large sample size. Another possibility may be the altitude gradient since these community types were found at reasonably high altitudes that provide optimal environmental conditions required for their physiological needs and favor vegetation growth (Rosenzweig 1995 ; Schmitt et al. 2013 ). Moreover, the tradition of the local people named as KOBO system might have contributed for the higher richness and diversity of plants in these plant community types as this culture emphasizes on the use of the forest for traditional apicultural practices and other non-timber forest products. The same observations was made in the previous study on the area (Tamene 2010 ) and elsewhere in the southwest forest (Wood et al. 2019 ). This communal culture has also been reported elsewhere to have conservation value in other southwest forests of Ethiopia (Tadesse and Woldemariam 2007 ; Woldemariam and Getaneh 2011 ). On the contrary, lower richness and diversity of plants were found in the second community type (C2) which was found in the lower altitude of the study area (840–1200 m, asl). Possible reason could be high level of disturbance and effect of local climatic variation. Moreover, geographical isolation might also have contributed to the low diversity of plants in this forest since this community type is bordered by large tracts of dry and lowland vegetation of Gambella Regional state (Friis 1992 ).
The findings of this study revealed that community types 3, 5, and 6 are with relatively low species richness and diversity of plants. The low diversity and richness of species in community type 3, which was mainly represented on the peak of mount TUPI (in Tupi Kebele) of the study area, might be a consequence of the chilly climatic nature that may be too harsh to permit other plants to grow well except few species such as the alpine bamboo. Moreover, the low richness and diversity of plants in this community type may be attributed to its small size. Inline with this Rahbek ( 1997 ) reported that areas on top of conical mountains are not only cooler but they are also smaller; and are often poor in plant species. The low richness and diversity of species in community type 5 may be associated with the absence of a number of woody plants. This absence might be due to the fact that the stagnant water in the swampy places restricts the root growth of woody plants due to poor soil aeration. Lower diversity of the existing species in this community type could also be attributed to the anthropogenic influences such as over-harvesting of grasses and sedges important for beautifying floors in the culture of the local people mainly for holidays, and for making roofs of the huts in the rural areas. Moreover, this plant community type is the sole place for grazing; and consequently, these places are over-grazed by livestock. Similar observations on the wetlands of southwest forests of Ethiopia were noted in previous studies (Woldu 1999 ; Hailu 2003 ; Woldu and Yeshitela 2003 ; Tolossa et al. 2022 ). The low diversity and richness of plant species in community type 6 might also be attributed to their occurrence close to settlements that were mainly situated at 1600–1800 m asl. Studies elsewhere also suggested that areas close to settlements are often prone to poor diversity of plants (Fahrig 2003 ; Somashekar et al. 2003 ; Specht et al. 2015 ). The preference of the local people to settle at this altitude zone (or community type) is perhaps a consequence of the occurrence of rich coffee plant resources, which is an important cash crop in the study area (Ango et al. 2020 ). According to the report in Wikipedia Free Encyclopedia ( 2015 ), the use of Coffee beans as a cash crop in the area is believed to start during the time of Dejazmach Ganame, governor of Illubabur during the time of Minilik II (1889–1913). The dominancy of wild coffee plants in this altitude zone may be attributed to its dispersal limitation and species selection. Given this community type is more abundant in wild coffee ( Coffea arabica ), which is a flagship species at the international level, it would help ecologists to consider this matter under any possible conservation activities. This altitude zone is also reported to be a dominant habitat for naturally growing coffee ( C. arabica ) population in other Afromontane rainforests of Ethiopia (Teketay 1999 ; Woldemariam et al. 2002 ; Senbeta and Denich 2006 ; Schmitt et al. 2009 ; Geeraert et al. 2019 ; Hwang et al. 2020 ).
In this study we also noted a positive and strong correlation between sample plots and species number of the identified communities and consequently with species diversity and beta diversity. Similar observation were noted from the findings of similar works elsewhere in the country (Tadesse et al. 2017 ; Awoke et al. 2022 ; Sewale and Mammo 2022 ). This is perhabs the larger the plot numbers along a specific gradient the higher will be the probability of having diverse ecological niche that will be responsible for more number of species to exist (Peterson et al. 2011 ).
Based on the beta diversity analysis the study area can be regarded as a heterogenous forest ( \({\beta }_{w}\) = 12.04) at landscape level (Woldu 2017 ). This could be due to the spatial and environmental heterogeneity of the area. At plant community level cluster 4 is the more heterogeneous ( \({\beta }_{w}\) > 5) forest part indicating a high turnover of plant species among the sample plots. This can be further illustrated in the box plot that shows high variability of data set between the samples. The reason for high beta diversity or variability between the samples could be the prescence of large sample size in this community type and relatively large elevation gradient. On the other hand, community 6 and7 scored the lowest beta diversity indicating each of these community types are relatively homogenous, and characterized by high compositional similarity in the sampled plots as it can also be noted from high Shannon evenness value (J = 0.92). This is still associated with the fewer number of sample plots found in the community types. Similar annotations were indicated by Yastrebov ( 1996 ) and Jurasinski et al. ( 2009 ).
Influence of environmental factors on the variation in species composition of plants in Sele-Nono forest
It is known that the vegetation cover of a given area is developed due to its long-term interaction with biotic and abiotic factors. Consequently, the finding of this study showed that the existing composition and diversity of plant species in the Sele-Nono forest is partially (12.5%) judged by environmental factors such as terrain variable (altitude and slopes), edaphic variables such as total nitrogen (N) and organic matter (OM) and disturbance factors.
The findings of this study show that altitude is a substantial factor for the high richness and diversity of plants on highland forests, particularly in community type 4 of the study area; and for the low richness and diversity of plants in community type 3. This may be attributed to the influence of altitude to build favorable microclimates (temperature and moisture) at the altitude range of 1850–2300 m asl for optimal growth of plants; and hostile microclimate on the extreme altitude (2300–2448 m asl) to limit the growth of plants in the study area due to the induced change to the soil environment (soil chemistry, nutrient release, organic matter decomposition). A similar explanation was given by Schmitt et al. ( 2013 ) about vegetation variation along an altitude gradient in moist montane forests of Ethiopia and elsewhere (Kabrick et al. 2008 ; Fonge et al. 2013 ).In this regard, altitude is often claimed as an important factor that has a strong influence on the vegetation diversity of moist southwest forest of Ethiopia in particular (Woldu et al. 1989 ; Nune 2008 ; Schmitt et al. 2010 ; Friis et al. 2011 ) and most montane ecosystem of the world in general (Lovett 1993 ; Eilu et al. 2004 ; Cirimwami et al. 2019 ; Asefa et al. 2020 ).
The finding of this study also shows that slope is an important environmental factor for community type 1 (C1) to harbor a large number of plant species. This may be attributed to the improperness of these areas to access it for use such as for plowing and crop cultivation, which are often regarded as serious threats to forests (Woldemariam 2003 ; Tadele et al. 2014 ; Asefa et al. 2020 ).
The study also showed that a high level of disturbance was one of the chief environmental factors near the lower limit of the study area. Clearing of forests and their conversion into croplands, and selective removal of trees were the main disturbance factors in C2 and C6 respectively. These factors mainly lead to the decline in species richness and diversity of plants in these community types. This could be because the clearing of forests and conversion into croplands in the study area is expected to reduce litter production, increase soil erosion rates and decomposition of organic matters (OMs) by oxidation (Lawton et al. 2001 ; Fahrig 2003 ); whereas disturbance through selective exploitation of canopies creates openings in the forest that may alter the local microclimate which would dry the soil rapidly and cause loss of nutrients through run-off (Berry et al. 2008 ; Ruger et al. 2008 ; Jiren et al. 2020 ). In both cases, disturbance reduces the natural stability of the study area which subsequently might impede the recovery of trees and shrubs. Results of many other studies in Ethiopia (MOA 1990 ) and elsewhere (Lovett 1999 ; Aubert et al. 2003 ; Onaindia et al. 2004 ; Rad et al. 2018 ; Chapagain et al. 2021 ) also shows similar findings in that anthropogenic disturbance leads to low species richness.
This study has also assessed the influence of edaphic factors on the diversity of plants. The influence of soil factors on floristic composition was also noted elsewhere (Infante-Mata et al. 2011 ). Accordingly, this study revealed that soil organic matter (OM), and total nitrogen contents (N) were the most significant edaphic factors in the Sele-Nono forest for the distribution of plants. Among the plant community types described in the lower altitude of the study area, (< 1500 m asl) community type 7 is with a high diversity of species. This might be related to the high organic matters (OM)content of the area, which could give the soil a buffering capacity by resupplying the soil solution when important nutrients are removed by uptake or leaching (Cole 1995 ; McCauley et al. 2017 ) and hence contribute for the rapid growth of plants. The high temperature that favors the decomposition of forest litter might have resulted in to have high-level of soil organic matter (OM) in this community type (community type 7). The presence of high temperature in this altitude zone of the moist southwest forest was also evident by Friis et al. ( 2011 ). In relation to these assumptions, vegetation ecologists have claimed that, unlike dry forests where moisture is the most decisive factor for decomposition and turnover rates, so does is the temperature in moist forests. On the contrary total nitrogen content of the soil is a more responsible edaphic factor for the vegetation pattern in community type 3, which is mainly represented by the vegetation of swampy lands. This may be partly because the wetlands/swampy areas are often presented as symmetric ball/cup-shaped curves circumscribed by hilly slopes terrains, and flooded with the runoff rains washed to gorges and downslopes during the rainy seasons. These conditions may form nitrate pollutions, especially when mixed with animal dugs leftover during the dry season while they were grazing. A similar observation was found in the Bonga forest by Nune ( 2008 ). Nitrogen in its nature is easily leachable from the soil along sloppy terrains that are exposed to high precipitation (Batjes and Dijkshoorn 1998 ; Widowati and De Neve 2016 ).
Threats to Sele-Nono forests
It is well-known that disturbance influences species composition and diversity of a forest ecosystem. However, not all aspects of disturbances are equally threatening the composition of forest vegetation. Thus, it is essential to know which disturbances are critical to the forest to bring meaningful changes in the conservation aspect (Simberloff 2001 ; Onaindia et al. 2004 ). Accordingly, this study found that clearing of forests for cropland expansion by the local people followed by selective removal of trees was the most notorious threat factor in the Sele-Nono forest. Clearing of forests for agricultural expansion is also reported as the main threatening factor accounting for the loss of 60% of tropical moist forests (Wright 2010 ; Chakravarty et al. 2012 ). However, this finding contradicts previous reports for other southwest forests of Ethiopia (e.g. Sheka and Bonga forest) that reported the conversion of forest land into commercial tea plantation as a leading threat of the forest ecosystem (Woldu 1999 ; Woldemariam and Getaneh 2011 ; Senbeta et al. 2013 ). This is because unlike the forests in Sheka and Bonga, the conversion of forest land into other commercial investments such as tea plantations in Sele-Nono is prohibited by the rule of the District administration office.
Moreover, the uncontrolled selective removal of trees that was reported as the second main threat in this study is also reported as the main threat to other southwest forests of Ethiopia (Ersado 2001 ; Abebe 2002 ). The presence of abundant number of disturbance-associated plant species such as Asteraceae and lianas in the current study may indicate that the area might have faced selective logging. Similar assertions were reported in other moist forests of Ethiopia (Nigatu and Tadesse 1989 ; Berhan and Assefa 2004 ; Senbeta et al. 2005 ; Lulekal et al. 2008 ) and elsewhere in tropical forests (Putz 1984 ; Gerwing 2002 ) who described the abundance of vines and/or Asteraceae plants in openings of a disturbed forest through selective logging. It is said that there was uncontrolled forest exploitation during the past times since the 1950s in the southwest forest mainly for the purpose of timber and timber-related purposes (Chaffey 1979 ; Ersado 2001 ; Tadesse and Woldemariam 2007 ). Most of the species reported in this study to be preferred for timber and timber-related purposes by the local people were also mentioned in other southwest forests of Ethiopia (Chaffey 1979 ; EFAP 1994 ; Schmitt et al. 2010 ; Desalegn et al. 2012 ; Dibaba et al. 2022 ). Some of these species are Pouteria adolfifriederici, Apodytes dimidiate, Celtis africana, Cordia africana, Croton macrostachyus, Ekebergiacapensis, Prunus africana, Olea welwitschii, Polyscias fulva, and Syzygium guineense . However, the current participatory forest management (PFM) system in the study area is playing a better opportunity in at least controlling the uncontrolled logging practices that have been prevailing in the forest. Moreover, thinning of forests for optimizing coffee bean productivity is also a substantial factor for the degradation of forests in areas where coffee growing is abundant. This threat is also a common phenomenon in other southwest forests of Ethiopia (Friis 1979 ; Teketay 1999 ; Woldemariam et al. 2002 ; Senbeta and Denich 2006 ; Schmitt et al. 2009 ; Ango et al. 2020 ).
Moreover, the finding of this study shows that clearing of forests for settlement expansion due to growing population is another source of threat to the study area. In regard to this finding Yilak and Debelo ( 2019 ) indicated that settlement and population growth are the main causes for forest degradation (decline of forest quality) and deforestation in the forests of southwest Ethiopia. On top of this, the recently wide-spreading new road construction projects in the study forest is an emerging threat of plant biodiversity in the Sele-Nono forest as it involves clearing of trees and fragment habitat. Similar observation was found elsewhere (Olander et al. 1998 ; Ledec and Posas 2003 ; Bera et al. 2006 ; Caliskan 2013 ).On the other hand, over-grazing and fire are among the least notorious threats to the study area. This could be attributed to the moist nature of the forest that could extinguish fire incidences. Moreover, the long rainy season permits rehab the grazed land. These are also reported as the least damaging threat in other moist forests elsewhere (Chakravarty et al. 2012 ; Tranquilli et al. 2014 ).
Conclusion and recommendation
The present study revealed that the Sele-Nono forest covering 150, 325.27 ha is characterized by mosaic of landscapes. Some of these landscapes have an intact and more protected vegetation as in for example in community types 1 and 4, which may be due to their occurance on suitable habitate and the role of the traditional knowledge of the local people to the forest (also called the KOBO system). On the contrary, some part of the forest especially those that are represented by community type 2 and 6 were more degraded. It can be also concluded that Sele-Nono forest at the landscape level is a reservoir of diverse vascular plants (414 species with high Shannon and beta diversity; H > 3, \({\beta }_{w}\) > 12). Of which 25 of them (6%) were endemic. Given the presence of a high number of plant species along with flagship species such as coffee and other ecological assets it could qualify to be labeled as a keystone ecosystem. Among the environmental factor treated in this study, altitude plays a key ecological role to influence the spatial distribution of plants in the Sele-Nono forest. Human-induced disturbance such as deforestation for cropland expansion and settlements and degradation through selective logging and thinning forest were major threats for the study area.
Recommendations
Sele-Nono forest, being a large forest and rich plant biodiversity shall be developed as a Biosphere Reserve as such system provide the possibility to compartmentalize the forest into core zone, transition zone and buffer zone. This approach further would provide adequate protection to the forest and suitable use of the resources for the communities around the forest.
Provision of environmental education to strengthen the existing participatory forest management (PFM) and KOBO system to the local people to minimize uncontrol logging in the forest.
The endangered and endemic plant species identified in this study shall be a prior species for conservation by the nearby higher institutions and other plant conservation affiliated research centers.
Among the plant community types established in this study, Cluster 2 and 6 shall be among the prioritized area for any possible protection as these areas have been under high level of threat.
Designing future vegetation monitoring studies are essential to evaluate the status of the forest and take appropriate actions for any significant changes that may occur in the forest (temporal effect).
Availability of data and materials
The data used and analyzed in this study can be provided from the respective author for scientifc, non-proft purpose.
Abebe T (2002) The influence of selective logging on residual stand and regeneration in a tropical rainforest in south-western Ethiopia. Acta Univ Agric Suec Silv 261:1–68
Google Scholar
Adams WM, Hulme D (2001) If community conservation is the answer in Africa, what is the question? Oryx 35(3):193–200
Article Google Scholar
Addi A, Soromessa T, Kelbessa E, Dibaba A, Kefalew A (2016) Floristic composition and plant community types of Agama Forest, an “Afromontane Forest” in Southwest Ethiopia. J Ecol Nat Environ 8(5):55–69
Addi A, Soromessa T, Bareke T (2020) Plant diversity and community analysis of Gesha and Sayilem Forest in Kaffa Zone, southwestern Ethiopia. Biodiversitas 21:2878–2888
Addo-Fordjour P, Rahmad ZB, Amui J, Pinto C, Dwomoh M (2013) Patterns of liana community in a tropical diversity and stracture in a tropical rainforest reserve, Ghana: effects of human disturbance. Afr J Ecol 51(2):217–227
Aerts R, Van Overtveld K, Haile M, Hermy M, Deckers J, Muys B (2006) Species composition and diversity of small Afromontane forest fragments in northern Ethiopia. Plant Ecol 187:127–142
Ango TG, Hylander K, Borjeson L (2020) Processes of forest cover change since 1958 in the coffee-producing areas of Southwest Ethiopia. Land 9:278
Asefa M, Cao M, He Y, Mekonnen E, Song X, Yang J (2020) Ethiopian vegetation types, climate and topography. Plant Diversity 42:302–311
Article PubMed PubMed Central Google Scholar
Asres T (1996). Agroecological Zones of South western Ethiopia. PhD. Thesis, University of Trier, Germany
Assefa A, Demissew S, Woldu Z (2013) Floristic composition, structure and regeneration status of Masha forest, south-west Ethiopia. Afr J Ecol 52:151–162
Aubert M, Alard D, Bureau F (2003) Diversity of plant assemblages in managed temperate forests: a case study in Normandy (France). For Ecol Manag 175:321–337
Awoke N, Nemomissa S, Bekele T (2022) Floristic composition and diversity of vascular plants in remnant patches of Arsi-Bale Massif of Oromia Regional state, Ethiopia. Int J Ecol. https://doi.org/10.1155/2022/6693679
Barthlott W, Biedinger N, Braun G, Feig F, Kier G, Mutke J (1999) Terminological and methodological aspects of the mapping and analysis of global biodiversity. Acta Bot Fenn 162:103–110
Batjes NH, Dijkshoorn JA (1998) Carbon and nitrogen stocks in the soils of the Amazon region. Geoderma 88:273–286
Bekele T (1993) Vegetation ecology of remnant Afromontane forests on the Central Plateau of Shewa, Ethiopia. Acta Phytogeogr Suec 79:1–59
Bekele T (1994) Phytosociology and ecology of a humid Afromontane forests on the Central Plateau of Shewa, Ethiopia. J Veg Sci 5:87–98
Bekele T (2003) The potential of Bonga forest for certification—a case study. http://www.pfmp-farmsos.org . Accessed on 10/01/2019
Bekele T, Yeshitela K, Dessie S, Gunther H (2004) Priority woody species of the moist montane forests of southwest Ethiopia consideration for conservation. In: Girma B, Yeshitela K, Bekele T (eds) Proceedings of a National Conference on forest resources of Ethiopia: status, challenges and opportunities. 27–29 November 2002. Institute of Biodiversity Conservation, Addis Ababa
Bera SK, Basumatary SK, Agarwal A, Ahmed M (2006) Conversion of forest land in Garo Hills, Meghalaya for construction of roads: A threat to the environment and biodiversity. Curr Sci 9(3):281–284
Berhan G, Assefa Y (2004) Conservation status of Boginda forest in the SNNPR region, Kefa Zone, Gimbo Woreda, Ethiopia. In: Girma B, Yeshitela K, Bekele T (eds) Proceedings of a National Conference on forest resources of ethiopia: status, challenges and opportunities. 27–29 November 2002. Institute of Biodiversity Conservation, Addis Ababa
Berry NJ, Phillips OL, Ong RC, Hamer KC (2008) Impacts of selective logging on tree diversity across a rainforest landscape: the importance of spatial scale. Landsc Ecol 23:915–929
Braun-Blanquet J (1932) Plant sociology: the study of plant communities. McGraw-Hill, New York
Breitenbach VF (1963) Forests and woodlands of Ethiopia: A geobotanical contribution to the knowledge of the principal plant communities of Ethiopia with special regard to forestry. Ethiop for Rev 1:5–16
Brockerhoff EG, Barbaro L, Castagneyrol B et al (2017) Forest biodiversity, ecosystem functioning and the provision of ecosystem services. Biodivers Conserv 26:3005–3035
Caliskan E (2013) Environmental impacts of forest road construction on mountain terrain. J Environ Health Sci Eng 10:23
Chaffey DR (1978a). Southwest Ethiopia forest inventory project. A glossary of vernacular names of plants in southwest Ethiopia with special reference to forest trees. Ministry of Overseas Development. Land Resources Development Centre. Project report 26:1–75 and map
Chaffey DR (1978b) Southwest Ethiopia Forest Inventory Project. An Inventory of Tiro Forest. Ministry of Overseas Development, Land Resources Development Centre. Project report. 30:1–60
Chaffey DR (1979) Southwest Ethiopia forest inventory project: a reconnaissance inventory of southwest Ethiopia. Ministry of Overseas Development. Land resources development centre. Project report 31 :1–316 and map
Chakravarty S, Ghosh SK, Suresh CP, Dey AN, Shukla G (2012) Deforestation: causes, effects and control strategies, global perspectives on sustainable forest management. In: Okia CA (ed) Sustainable forest management. Intech, Shanghai, pp 1–30
Chapagain U, Chapagain BP, Nepal S, Manthey M (2021) Impact of disturbances on species diversity and regeneration of Nepalese Sal (Shorea robusta) forests managed under different management regimes. Earth 2:826–844
Chebaeva N, Lettner M, Wenger J, Schoggl J, Hesser F, Holzer D, Stern T (2021) Dealing with the eco-design paradox in research and development projects: The concept of sustainability assessment levels. J Clean Prod. https://doi.org/10.1016/j.jclepro.2020.125232
Cirimwami L, Doumenge C, Kahindo JM, Amani C (2019) The efect of elevation on species richness in tropical forests depends on the considered lifeform: results from an East African mountain forest. Trop Ecol 60:473–484
Cole DW (1995) Soil nutrient supply in natural and managed forests. Plants Soil. 168(1):43–53
Conservation International (2006) Biodiversity Hotspots. Conservation International. Washington D.C. http://www.biodiversityhotspots.org . Accessed on 12/05/2018
CSA (2007) Summary and statistical report of the 2007 population and housing census. Population size by age and sex. Federal Democratic Republic of Ethiopia Census Commission
Denu, D. (2006). Floristic composition and ecological study of Bibita Forest (Gura Ferda) Southwest Ethiopia. M.Sc. Thesis, Addis Ababa University
Denu D (2019) Plant Species Richness and Diversity in Beda-Buna Coffee Forest, Jimma Zone, Oromia Regional State, Southwest Ethiopia, Ethiopian. J Educ Sci 15(1):1–17
Derero A (1998). Natural regeneration in a broad-leaved Afromontane rainforest, South West Ethiopia. M.Sc. Thesis. Swedish University of Agricultural Sciences, Uppsala, Sweden
Desalegn G, Abegaz A, Teketay D, Gezahgne A (2012) Commercial Timber Species in Ethiopia: characteristics and uses-a handbook for forest industries, construction and energy sectors, foresters and other stakeholders. Addis Ababa University Press, Addis Ababa
Diamond JM (1975) The island dilemma: lessons of modern biogeographic studies for the design of natural reserves. Biol Cons 7(2):129–146
Dibaba A, Soromessa T, Warkineh B (2022) Plant community analysis along environmental gradients in moist afromontane forest of Gerba Dima, South-Western Ethiopia. Ecol Evol 22:12
Edwards S, Tadesse M, Hedberg I (1995) Flora of Ethiopia and Eritrea. In: Edwards S, Tadesse M, Hedberg I (eds) Part 2. The National Herbarium, vol 2. Addis Ababa University, Uppsala
Edwards S, Demissew S, Hedberg I (1997) Flora of ethiopia and eritrea. In: Edwards S, Demissew S, Hedberg I (eds) ) The national herbarium, vol 6. Addis Ababa University, Uppsala
Edwards S, Tadesse M, Demissew S, Hedberg I (2000) Flora of Ethiopia and Eritrea. In: Edwards S, Tadesse M, Demissew S, Hedberg I (eds) Part 1. The National Herbarium, vol 2. Addis Ababa University, Uppsala
EFAP (1994) Ethiopian Forestry Action Program (EFAP). EFAP, Addis Ababa
Egziabher TBG (1991) Diversity of Ethiopian flora. In: Egziabher TBG (ed) Plant genetic resources of Ethiopia. Cambridge University Press, Cambridge, p 7581
Eilu G, Hafashimana DL, Kasenene JM (2004) Density and species diversity of trees in four tropical forests of the Albertine rift, western Uganda. Divers Distrib 10:303–312
Elliot S, Blakesley D, Hardwick K (2013) Restoring tropical forests: a practical guide. Royal Botanic Gardens, Kew
Ersado M (2001) An inventory of woody plant species diversity in Bonga forest. Unpublished report. IBCR, FAPGRD, GTZ, FGRCP, Addis Ababa
Ersado M, Assefa Y (2004) Structural characteristics of the moist montane forests in south west Ethiopia. In: Girma B, Yeshitela M, Bekele T (eds) Proceedings of a national conference on forest resources of ethiopia: status, challenges and opportunities. 27–29 november 2002. Institute of Biodiversity Conservation, Addis Ababa
Fahrig L (2003) Effects of habitat fragmentation on Biodiversity. Annu Rev Ecol Evol Syst 34:487–515
FAO (2006). Global forest resources assessment 2005: Progress towards sustainable forest management. FAO Forestry Paper 147
FAO (2010). Forest Resources Assessment: Global Tables, Rome. www.fao/forestry/fra/fra2010/en/ . Accessed on 3/7/2017
FAO (2020) Global Forest Assessment (FRA) 2020: terms and definitions. Forest resources assessment working paper 188, Rome
Farr TG, Kobrick M (2000) Shuttle Radar topography mission produces a wealth of data. Eos Trans Am Geophys Un 81:583–583
Fonge BA, Tchetcha DJ, Nkembi L (2013) Diversity, distribution, and abundance of plants in Lewoh-Lebang in the Lebialem highlands of Southwestern Cameroon. Int J Biodiv. https://doi.org/10.1155/2013/642579
Friis I (1979) The wild populations of Coffea arabica L. and the cultivated coffee. Proceedings of the IXth Plenary Meeting of AETFAT: 63–68
Friis I (1986a) The forest vegetation of Ethiopia. Symb Bot Ups 26(2):31–47
Friis I (1986b) Ethiopia in regional phytogeography. Symb Bot Ups 26(2):31–47
Friis I (1992) Forest and forest trees of Northeast Tropical Africa. Kew Bull Add Ser 15:1–396
Friis I (2002) Progress with the botanical exploration of Ethiopia and Eritrea during the last forty years. Syst Geogr Plants 71(2):165–186
Friis I (2009) The scientific study of the flora of Ethiopia andEritrea up to the beginning of the Ethiopian flora Project (1980). In: Hedberg I, Friis I, Persson E (eds) Flora of Ethiopia and Eritrea. The National Herbarium, vol 8. Addis Ababa University, Addis Ababa, pp 5–25
Friis I (2011) Botanical collecting activity in the area of the Flora of Ethiopia and Eritrea during the “motor period.” Acta Univ Ups Symb Bot Ups 35(2):45–68
Friis I, Rasmussen FN, Vollesen K (1982) Studies in the flora and vegetation of southwest Ethiopia. Opera Bot 63:8–70
Friis I, Demissew S, Breugel PV (2011) Atlas of the potential vegetation of Ethiopia. Addis Ababa University Press, Addis Ababa
Gebrehiwot K, Hundera K (2014) Species composition, Plant Community structure and Natural regeneration status of Belete Moist Evergreen Montane Forest, Oromia Regional state, Southwestern Ethiopia. Momona Ethiop J Sci 6(1):97–101
Geeraert L, Berecha G, Honnay O, Aerts R (2019) Organoleptic quality of Ethiopian Arabica coffee deteriorates with increasing intensity of coffee forest management. J Environ Manag 231:282–288
Article CAS Google Scholar
Gerwing JJ (2002) Degradation of forests through logging and fire in the eastern Brazilian Amazon. For Ecol Manage 157:131–141
Girma A (2011) Plant communities, species diversity, seedling bank and resprouting in nandi forests, Kenya. PhD Thesis. University of Koblenz-Landau, Germany
Gobeze T, Bekele M, Lemenih M, Kassa H (2009) Participatory forest management and its impacts on Livelihoods and forest status: the case of Bonga forest in Ethiopia. Int for Rev 11(3):346
Gurmessa F, Soromessa T, Kelbessa E (2013) Floristic composition and community analysis of Komto Afromontane Moist Forest, East Wollega Zone, West Ethiopia. Sci Technol Arts Res J 2(2):58–69
Hailu A (2003) Wetlands research in south-western Ethiopia: the experience of the Ethiopian Wetlands Research Programme. In: Abebe Y, Geheb K (eds) Wetlands of Ethiopia. Proceedings of a seminar on the resources and status of Ethiopia’s Wetlands. International Union for Conservation of Nature and Natural Resources, Nairobi, pp 37–48
Hedberg I, Edwards S (1989) Flora of Ethiopia. In: Hedberg I, Edwards S (eds) The National Herbarium, vol 3. Addis Ababa University, Addis Ababa
Hedberg I, Kelbessa E, Edwards S, Demissew S, Persson E (2006) Flora of Ethiopia and Eritrea. In: Hedberg I, Kelbessa E, Edwards S, Demissew S, Persson E (eds) The National Herbarium, vol 5. Addis Ababa University, Addis Ababa
Hedberg I, Friis I, Persson E (2009) Flora of Ethiopia and Eritrea. In: Hedberg I, Friis I, Persson E (eds) The National Herbarium, vol 8. Addis Ababa University, Addis Ababa
Hundera K, Deboch B (2008) Woody Species Composition and Structure of the Gurra Farda Forest, SNNPR, South Western Ethiopia, Ethiopian. J Educ Sci 3:43–54
Hundera K, Gadissa T (2008) Woody species composition and structure of the Belete forest, Jimma zone, South Western Ethiopia, Ethiopian. J Biol Sci 7:1–15
Hwang B, Hundera K, Mekuria B, Wood A, Asfaw A (2020) Intensified management of coffee forest in Southwest Ethiopia detected by landsat imagery. Forests 11:422
Hylander K, Nemomissa S, Enkosa W (2013a) Edge effects on understory epiphytic ferns and epiphyllous bryophytes in moist afromontane forests of Ethiopia. Polish Botan J 58(2):555–563
Hylander K, Nemomissa S, Delrue J, Enkosa W (2013b) Effects of coffee management on deforestation rates and forest integrity. Conserv Biol 27(5):1031–1040
Article PubMed Google Scholar
Infante-Mata D, Moreno-Casasola P, Madero-Vega C, Castillo-Campos G, Warner BG (2011) Floristic composition and soil characteristics of tropical freshwater forested wetlands of Veracruz on the coastal plain of the Gulf of Mexico. For Ecol Manag 262:1514–1531
Jiren TS, Hanspach J, Schultner J, Fischer J, Bergsten A, Senbeta F, Hylander K, Dorresteijn I (2020) Reconciling food security and biodiversity conservation: participatory scenario planning in southwestern Ethiopia. Ecol Soc 25(3):24
Jurasinski G, Retzer V, Beierkuhnlein C (2009) Inventory, differentiation, and proportional diversity: a consistent terminology for quantifying species diversity. Oecologia 159(1):15–26.
Kabrick JM, Dey DC, Jensen RG, Wallendorf M (2008) The role of environmental factors in oak decline and mortality in the Ozark Highlands. For Ecol Manage 255:1409–1417
Kebede M, Yirdaw E, Luukkanen O, Lemenih M (2013) Plant community analysis and effect of environmental factors on the diversity of woody species in the moist Afromontane forest of Wondo Genet, South Central Ethiopia. Biodiv Res Conserv 29:63–80
Kefalew A, Asfaw Z, Kelbessa E (2015) Ethnobotany of medicinal plants in Ada’a District, East Shewa Zone of Oromia Regional State, Ethiopia. J Ethnobiol Ethnomed 11:25
Kelbessa E, Soromessa T (2008) Interfaces of regeneration, structure, diversity and uses of some plant species in Bonga forest: a reservoir for wild coffee gene pool. SINET Ethiop J Sci 31(2):121–134
Kelbessa E, Demissew S, Woldu Z, Edwards S (1992) Some threatened endemic plants of Ethiopia. In: Edwards S, Zemede A (eds) Botany 2000: East and Central Africa, NAPERICA Monograph Series No 2. Addis Ababa University, Addis Ababa, pp 33–35
Kent M, Coker P (1992) Vegetation description and analysis: a practical approach. Wiley, Chichester
Kigomo B (1999) Considerations and procedure for priority setting in a national forest genetic resources conservation and management programme. In: Edwards S, Demise A, Bekele T, Gunther H (eds) Proceedings of the national forest genetic resources conservation strategy development workshop June 21–22, Addis Ababa Ethiopia. IBCR, Addis Ababa
Kindt R, van Breugel P, Lilleso JPB, Bingham M, Demissew D, Dudley C, Friis I, Gachathi F, Kalema J, Mbago F, Minani V, Moshi HN, Mulumba J, Namaganda M, Ndangalasi HJ, Ruffo CK, Jamnadass R and Graudal L (2011). Potential natural vegetation of Eastern Africa: description and tree species composition for forest potential natural vegetation types, (volume 2). Forest and Landscape Working Papers no. 62–2011, ISBN 978–87–7903–551–5
Klopper RR, Smith GF, van Rooy J (2002) The biodiversity of Africa. In: Biaijanath H, Singh Y (eds) Rebirth of science in Africa: a shared vision for life and environmental sciences. Umdaus Press, Hatfield, pp 60–86
Kneeshaw DD, Leduc A, Drapeau P, Gauthier S, Pare D, Carignan R, Doucet R, Bouthillier L, Messier C (2000) Development of integrated ecological standards of sustainable forest management at an operational scale. For Chron 76:481–493
Kongphunphin C, Srivanit M (2021) A multi-dimensional clustering applied to classify the typology of urban public parks in Bangkok Metropolitan Area, Thailand. Sustainability 13:11426. https://doi.org/10.3390/su132011426
Kuper W, Sommer JH, Lovett JC, Mutke J, Linder HP, Beentje HJ, Van Rompaey RSAR, Chatelain C, Sosef M, Barthlott W (2004) Africa’s hotspots of biodiversity redefined. Ann Mo Bot Gard 91(4):525–535
Larsson S, Danell K (2001) Science and the management of boreal forest biodiversity. Scand J for Res 3:5–9
Lawton RO, Nair US, Pielke RA, Welch RM (2001) Climatic impact of tropical lowland deforestation on nearby montane cloud forests. Science 294:584–587
Article CAS PubMed Google Scholar
Ledec G, Posas P (2003) Biodiversity conservation in roads projects: lessons from World Bank experience in Latin America. Transp Res Rec. https://doi.org/10.3141/1819a-29
Legendre P, Fortin M, Borcard D (2015) Should the Mantel test be used in spatial analysis? Methods Ecol Evol 6:1239–1247
Leitner W, Turner WR (2001) Measurement and analysis of biodiversity. In: Levin SA (ed) Encyclopaedia of biodiversity, vol 4. Academic Press, Princeton, pp 123–144
Chapter Google Scholar
Leps J, Smilauer P (2003) Multivariate analysis of ecological data using CANOCO. Cambridge University Press, Cambridge
Book Google Scholar
Linder HP, Lovett J, Mutke J, Barthlott W, Jürgens N, Rebelo T, Küper W (2005) A numerical re-evaluation of the sub-Saharan phytochoria of mainland Africa. Plant diversity and complexity patterns: local, regional, and global dimensions. In: Linder HP (ed) Proceedings of an International symposium held. Royal Danish Academy of Sciences and Letters in Copenhagen, Copenhagen
Logan WEM (1946) An introduction to the forests of central and southern Ethiopia. Imp for Inst Paper 24:1–58
Lovett JC (1993) Eastern Arc moist forest flora. In: Lovett JC, Wasser SK (eds) Biogeography and ecology of the rain forests of eastern Africa. Cambridge University Press, Cambridge, pp 33–55
Lovett JC (1999) Tanzanian forest tree plot diversity and altitude. J Trop Ecol 15:689–694
Lulekal E, Kelbessa E, Bekele T, Yinger H (2008) Plant species composition and structure of the Mana Angetu moist montane forest, South-eastern Ethiopia. J East Afr Nat Hist 97(2):165–185
MacDicken K (1997) A guide to monitoring carbon storage in forestry and agroforestry projects. Forest carbon monitoring program. Winrock International, Arlington
Magurran AE (1988) Ecological diversity and its measurement. Chapman and Hall, London
Magurran AE (2004) Measuring biological diversity. Blackwell Publishing, Malden
Malcolm JR (1994) Edge effects in central Amazonian forest fragments. Ecology 75:2438–2445
Martin GJ (1995) Ethnobotany: a method manual. Chapman and hall, London
McCauley A, Jones C, Olson-Rutz K (2017) Nutrient management: soil pH and organic matter. Montana State University, Bozeman
McCune B, Grace JB (2002) Analysis of ecological communities. MjM Software Design, Gleneden Beach
Meyer FG (1965) Notes on wild Coffea arabica from Southwestern Ethiopia, with some historical considerations. Econ Bot 19:136–151
Mittermeier RA, Robles Gil P, Hoffmann M, Pilgrim J, Brooks T, Mittermeier CG, Lamoreux J, da Fonseca GAB (2004) Hotspots revisited. Cemex Books on Nature, Mexico City
MOA (1990) Ministry of agriculture: study on forest resource base: identification, conservation and rational use. State forest conservation and development department. Internal report. Addis Ababa
Mueller-Dombois D, Ellenberg H (1974) Aims and methods of vegetation ecology. Wiley, New York
Mulugeta Y, Bekele T, Kelbessa E (2015) Floristic composition, species diversity and vegetation structure of Gera moist montane forest, Jimma Zone of Oromia National Regional State, Southwest Ethiopia, Ethiopian. J Biol Sci 14(1):45–68
Mutke J, Barthlott W (2005) Patterns of vascular plant diversity at continental to global scales. Biologiske Skrift 55:521–537
Nalli G, Amendola D, Perali A, Mostarda L (2021) Comparative analysis of clustering algorithms and moodle plugin for creation of student heterogeneous groups in online university courses. Appl Sci 11:5800. https://doi.org/10.3390/app11135800
Natural Database for Africa (2011). Version 2.0. NDA is a species based versatile database developed by Ermias Dagne, Ethiopia
Negesse G, Woldearegay M (2022) Floristic diversity, structure and regeneration status of Menfeskidus Monastery forest in Berehet District, North Shoa, central Ethiopia. Trees for People. https://doi.org/10.1016/j.tfp.2022.100191
Nigatu N, Tadesse M (1989) An Ecological study of the vegetation of the Harenna forest, Bale, Ethiopia. SINET Ethiop J Sci 12:63–93
Nune S (2008). Flora Biodiversity Assessment in Bonga, Boginda and Mankira forest, Kefa, Ethiopia. A report prepared for Ethiopian Wildlife and Natural History Society, Addis Ababa
Oksanen J, Blanchet FG, Kindt R, Legendre P, Minchin PR, O'hara RB, Simpson GL, Solymos P, Stevens MH. H. Wagner. (2011). Vegan: community ecology package. R package version 2.0–1
Olander LP, Scatena FN, Silver WL (1998) Impact of disturbance initiated by road construction in a subtropical cloud forest in the Luquillo experimental forest, Puerto Rico. For Ecol Manag 109:33–49
Olson DM, Dinerstein E (1998) The global 200: a representation approach to conserving the earth’s most biologically valuable ecoregions. Conserv Biol 3:502–515
Olson DM, Dinerstein E, Wikramanayake ED, Burgess ND, Powell GVN, Underwood EC, D’Amico JA, Itoua I, Strand HE, Morrison JC, Loucks CJ, Allnutt TF, Ricketts TH, Kura Y, Lamoreux JF, Wettengel WW, Hedao P, Kassem KR (2001) Terrestrial ecoregions of the world: a new map of life on earth. Bioscience 51:933–938
Onaindia M, Dominguez I, Albizu I, Garbisu C, Amezaga T (2004) Vegetation diversity and vertical structure as indicators of forest disturbance. For Ecol Manag 195:341–354
Pearce D and Pearce C (2001) The value of forest ecosystems. A Report to the Secretariat Convention on Biological Diversity (CBD)
Peterson AT, Soberón J, Pearson RG, Anderson RP, Martínez-Meyer E, Nakamura M, Araújo MB (2011) Ecological niches and geographic distributions. In: Levin SA, Horn HS (eds) Monographs in population biology. Princeton University Press, Princeton
Podani J (2006) Braun-Blanquet’s legacy and data analysis in vegetation science. J Veg Sci 17:113–117
Putz FE (1984) The natural history of Lianas on Barro Colorado Island, Panama. Ecology 65(6):1713–1724
R Development Core Team (2011). R: A language and environment for statistical computing. R Foundation for Statistical Computing, 360 Vienna, Austria. ISBN 3-900051-07-0,. http://www.Rproject.org/
Rad JE, Valadi G, Salehzadeh O, Maroofi H (2018) Effects of anthropogenic disturbance on plant composition, plant diversity and soil properties in oak forests. Iran J for Sci 64(8):358–370
CAS Google Scholar
Rahbek C (1997) The relationship among area, altitude, and regional species richness in neotropical birds. Am Nat 149:875–902
Reusing, M. (1998). Monitoring of Natural High Forests in Ethiopia. Government of the Federal Democratic Republic of Ethiopia, Ministry of Agriculture, Natural resources management and regulatory department. In cooperation with GTZ, Addis Ababa, Ethiopia
Richards PW (1996) The tropical rainforest: an ecological study, 2nd edn. Cambridge University Press, Cambridge
Roberts DW (2010) labdsv: ordination and multivariate analysis for ecology. R package version 1.4–1. http://CRAN.Rproject.org/package=labdsv
Rosenzweig ML (1995) Species diversity in space and time. Cambridge University Press, Cambridge
Ruger N, Williams-Linera G, Kissling WD, Huth A (2008) Long-term impacts of fuelwood extraction on a tropical montane cloud forest. Ecosystems 11:868–881
Sanjit L, Bhatt D (2005) How relevant are the concepts of species diversity and species richness? J Biosci 30:557–560
Sayer JA, Harcourt CS, Collins NM (1992) The Conservation Atlas of Tropical Forests, Africa. Macmillan Publishers, Basingstoke
Schmitt CB (2006) Montane rainforest with wild Coffea arabica in the Bonga region (SW Ethiopia): plant diversity, wild coffee management and implications for conservation. In: Schmitt CB (ed) Ecology and development series, vol 47. Cuvillier Verlag, Gottingen
Schmitt CB, Senbeta F, Denich M, Preisinger H, Boehmer HJ (2009) Wild coffee management and plant diversity in the montane rainforest of south-western Ethiopia. Afr J Ecol 48:78–86
Schmitt CB, Denich M, Demissew S, Friis I, Boehmer HJ (2010) Floristic diversity in fragmented Afromontane rainforests: Altitudinal variation and conservation importance. Appl Veg Sci 13:291–304
Schmitt CB, Senbeta F, Woldemariam T, Rudner M, Denich M (2013) Importance of regional climates for plant species distribution patterns in moist Afromontane forest. J Veg Sci 24(3):553–568
Senbeta F (2006) Biodiversity and ecology of Afromontane rainforests with wild Coffea arabica L. populations in Ethiopia. In: Senbeta F (ed) Ecology and development series, vol 38. Cuvillier Verlag, Gottingen
Senbeta F, Denich M (2006) Effects of wild coffee management on species diversity in the Afromontane rainforests of Ethiopia. For Ecol Manage 232:68–74
Senbeta F, Teketay D (2003) Diversity, community types and population Structure of woody plants in Kimphee forest: a unique nature reserve in Southern Ethiopia. Eth J Biol Soc 2(2):169–187
Senbeta F, Schmitt C, Denich M, Demissew S, Vlek PLG, Preisinger H, Woldemariam T, Teketay D (2005) The diversity and distribution of lianas in the Afromontane rainforests of Ethiopia. Divers Distrb 11:443–452
Senbeta F, Woldemariam T, Demissew S, Denich M (2007) Floristic diversity and composition of Sheko forest, southwest Ethiopia. SINET Ethiop J Sci 6(1):11–42
Senbeta F, Woldemariam T, Denich M, Kelbessa E (2013) Diversity of useful plants in the coffee forests of Ethiopia. Ethnobot Res Appl 11:49–69
Senbeta F, Schmitt C, Woldemariam T, Boehmer HJ, Denich M (2014) Plant diversity, vegetation stracture and relationship between plant communities and environmental variables in the Afromontane forests of Ethiopia. SINET Ethiop J Sci 37(2):113–130
Sewale B, Mammo S (2022) Analysis of floristic composition and plant community types in Kenech natural forest, Kaffa Zone, Ethiopia. Trees for People. https://doi.org/10.1016/j.tfp.2021.100170
Shannon CE, Wiener W (1949) The mathematical theory of communication. University of Illinois, Chicago
Simberloff D (2001) Management of boreal forest biodiversity-a view from the outside. Scand J for Res 3:105–118
Siraj M, Zhang K, Demissew S, Woldu Z (2017) Floristic composition and plant community types in Maze National Park, southwest Ethiopia. Appl Ecol Environ Res 15(1):245–262
SNDAO (2015). Unpublished report from Sele-Nono district agricultural organization
Somashekar RK, Raj MB, Nagaraja BC (2003) Impact of human settlement on forest structure and composition in Kudremukh national park, Western Ghats, south India. XII World Forestry Congress, Quebec City
Soromessa T, Kelbessa E (2013). Diversity, ecology and regeneration studies of Bonga, Borana and Chilimo forests of Ethiopia. Saarbrucken, Lambert Academic Publishing. ISBN 978-3-659-41509-8
Soromessa T, Teketay D, Demissew S (2004) Ecological study of the vegetation in Gamo Gofa zone, Southern Ethiopia. Trop Ecol 45(2):209–221
Specht MJ, Pinto SRR, Albuquerque UP, Tabarelli M, Melo FPL (2015) Burning biodiversity: fuelwood harvesting causes forest degradation in human-dominated tropical landscapes. Glob Ecol Conserv 3:200–209
Ssegawa P, Nkuutu DN (2006) Diversity of vascular plants on Ssese Islands in Lake Victoria, Central Uganda. Afr J Ecol 44:22–29
Tadele D, Lulekal E, Damtie D, Assefa A (2014) Floristic diversity and regeneration status of woody plants in Zengena Forest, a remnant montane forest patch in north-western Ethiopia. J for Res 25(2):329–336
Tadesse M (1993) A survey of the evergreen forests of Ethiopia. In: Berhanu AG (ed) Proceedings of the national workshop on setting forestry research: priorities in Ethiopia. Forestry Research Center, Addis Abeba, pp 265–291
Tadesse M (1994) History of botanical exploration in Ethiopia. In: Lepage C (ed) Actes de la Xe Conference Internationale des Etudes Ethiopiennes. Societe Francaise pour les Etudes Ethiopiennes, Paris, pp 529–540
Tadesse M (2004) Asteraceae (Compositae). In: Hedberg I, Friis I, Edwards S (eds) Flora of Ethiopia and Eritrea, part 2, vol 4. Addis Ababa University and Uppsala University, Uppsala, pp 1–408
Tadesse D, Woldemariam T (2007) Customary forest tenure in southwest Ethiopia. For Trees Livelihood. 17(4):325–338
Tadesse Z, Kelbessa E, Bekele T (2017) Floristic composition and plant community analysis of vegetation in Ilu Gelan district, West Shewa Zone of Oromia region, Central Ethiopia. Trop Plant Res 4(2):335–350
Tamene B (2010). The impact of traditional forest management practices on composition, structure and diversity of woody species of sele-nono natural forest and its contribution to the local community, illubabor Zone of Oromia Regional State, Southwest Ethiopia. M. Sc thesis in forestry (unpublished), Hawassa University, Ethiopia
Tedla S (2003) Ethiopian environmental condition: today and twenty-five years from now. Econ Focus 5:17–34
Teketay D (1999) History, botany and ecological requirements of coffee. Walia 20:2850
Teketay D (2004) Forestry research in Ethiopia: past, present and future. In: Girma B, Yeshitela K, Bekele T (eds) Proceedings of a National conference on forest resources of Ethiopia: status, challenges and opportunities 27–29 November 2002. Institute of Biodiversity Conservation, Addis Ababa
Thomas SC and Baltzer JL (2002). Tropical Forests. Encyclopaedia of life sciences. Nature Publishing Group. www.els.net . Accessed on 11/8/2020
Tolossa T, Bakala F, Alemkere A, Fite Y (2022) Benefits of Wetlands and Attitudes of Local Communities towards Wetland Conservation in the Southwest Ethiopia. Wetl Conserv. https://doi.org/10.3897/arphapreprints.e85603
Tranquilli S, Abedi-Lartey M, Abernethy K, Amsini F, Asamoah A, Balangtaa C (2014) Protected areas in tropical Africa: assessing threats and conservation activities. PLoS ONE 9(12):e114154. https://doi.org/10.1371/journal.pone.0114154
Article CAS PubMed PubMed Central Google Scholar
Triantis KA, Bhagwat SA (2011) Applied Island biogeography. In: Ladle RJ, Whittaker RJ (eds) Conservation biogeography. Blackwell Publishing Ltd, Padstow, pp 190–223
Turner IM (1996) Species loss in fragments of tropical rainforest; a review of the evidence. J Appl Ecol 33:200–219
Uwalaka NO, Borisade TV, Rufai AB (2021) Liana abundance and colonization in a tropical moist secondary lowland rainforest in Nigeria. Taiwania 66(2):174–183
Van der Maarel E (1979) Transformation of cover abundance values in phytosociology and its effects on community. Vegetation 39:97–114
Van der Maarel E (2005) Vegetation ecology—an overview. In: van der Maarel E (ed) Vegetation ecology. Blackwell Publishing, Padstow, pp 1–51
Villagra BLP, Gomes EPC, Burnham RJ, Neto SR (2013) Diversity and abundance of climbers from the Atlantic forest, south-eastern Brazil. Biodiv Conserv 22:2505–2517
Vivero JL, Kelbessa E, Demissew S (2005) The red list of endemic trees & shrubs of Ethiopia and Eritrea. Fauna and Flora International, Cambridge
Vivero JL, Kelbessa E, Demissew S (2006) Progress on the Red List of plants of Ethiopia and Eritrea: conservation and biogeography of endemic flowering taxa. In: Ghazanfar SA, Beentje HJ (eds) Taxonomy and ecology of african plants, their conservation and sustainable use. Royal Botanic Gardens, Kew, pp 761–778
WBISPP (Woody Biomass Inventory and Strategic Planning Project) (2004). Forest Resources of Ethiopia. Addis Ababa, Ethiopia
WCMC (World Conservation Monitoring Center) (1992) Global biodiversity: status of the earth’s living resources. Chapman and Hall, London
White F (1978) The Afromontane region. In: Werger MJA (ed) Biogeography and ecology of Africa. Junk, The Hague
Whitemore TC (1997) Tropical forest disturbance, disappearance and species loss. In: Laurence WL, Bierregaard RO (eds) Tropical forest remnants. University of Chicago Press, Chicago, pp 1–3
Whitemore TC (2008) An introduction to tropical forests, 2nd edn. Oxford University Press, New York
Whittaker RH (1972) Evolution and measurement of species diversity. Taxon 21:213–251
Whittaker RJ, Fernandez Palacios JM (2007) Island biogeography: ecology, evolution, and conservation, 2nd edn. Oxford University Press, Oxford
Widowati LR, De Neve S (2016) Nitrogen dynamics and nitrate leaching in intensive vegetable rotations in Highlands of Central Java, Indonesia. J Trop Soils 21(2):67–78
Wiki En (2015). Sele-Nono district. Wikipedia free encyclopaedia. Retrieved on 8 Oct 2016
Wilson EO (1992) The Diversity of Life. Belkneys Press of Harvard University, Cambridge
Wilson EO, MacArthur RH (2016) The theory of Island biogeography. Princeton University Press, Princeton
Wilson JB, Peet RK, Dengler J, Partel M (2012) Plant species richness: the world records. J Veg Sci 23:796–802
Woldemariam T (2003) Vegetation of the Yayu forest in SW Ethiopia: impacts of human use and implications for in situ conservation of wild Coffea arabica L. populations. In: Woldemariam T (ed) Ecology and development series, vol 10. Cuvillier Verlag, Gottingen
Woldemariam T, Getaneh F (2011) Sheka forest biosphere reserve nomination form UNESCO-MAB. National Committee Federal Democratic Republic of Ethiopia, Addis Ababa
Woldemariam T, Denich M, Teketay D, Vlek PLG (2002) Human impacts on Coffea arabica genetic pool in Ethiopia and the need for its in situ conservation. In: Engels J, Ramanatha Rao V, Brown AH, Jackson M (eds) Managing plant genetic diversity. CAB International, Oxon, pp 237–247
Woldu Z (1999) Forests in the vegetation types of Ethiopia and their status in the geographical context. In: Edwards S, Abebe D, Bekele T, Gunther H (eds) Proceedings of the national forest genetic resources conservation strategy development workshop, June 21–22, 1999. Kenya Forestry Research Institute, Addis Ababa, pp 1–48
Woldu Z (2012) Environmental and ecological data analysis: basics, concepts and methods. Lambert Academic Publishing, Sunnyvale
Woldu Z (2017) Comprehensive and ecological data analysis: basics, concepts and methods. Addis Ababa University, Addis Ababa
Woldu Z, Yeshitela K (2003) Wetland plants in Ethiopia with examples from Illubabor, south-western Ethiopia. In: Abebe Y, Geheb K (eds) Wetlands of Ethiopia. Proceedings of a Seminar on the Resources and Status of Ethiopia’s Wetlands. International Union for Conservation of Nature and Natural Resources (IUCN), Nairobi, pp 49–66
Woldu Z, Feoli E, Nigatu L (1989) Partitioning an altitudeal gradient of vegetation from south eastern Ethiopia by probabilistic methods. Vegetation 81:189–198
Wood A, Tolera M, Snell M, O’Hara P, Hailu A (2019) Community forest management (CFM) in south-west Ethiopia: maintaining forests, biodiversity and carbon stocks to support wild coffee conservation. Glob Environ Chang. https://doi.org/10.1016/j.gloenvcha.2019.101980
Wright SJ (2010) The future of tropical forests. Ann NY Acad Sci 1195:1–27
Wright SJ, Muller-Landau HC (2006) The future of tropical forest species. Biotropica 38:287–301
WWF and IUCN (1994) Centres of plant diversity a guide and strategy for their conservation, vol 3. IUCN Publications Unit, Cambridge
Yalden DW (1983) The extent of high ground in Ethiopia compared to the rest of Africa. SINET Ethiop J Sci 6:35–39
Yasin H, Kebebew Z, Hundera K (2018) Woody species diversity, regeneration and socioeconomic benefits under natural forest and adjacent coffee Agroforests at Belete forest, southwest Ethiopia. Ekológia (bratislava) 37(4):380–391
Yastrebov AB (1996) Different types of heterogeneity and plant competition in monospecific stands. Oikos 75: 89–97
Yeshitela K, Bekele T (2002) Plant community analysis and ecology of Afromontane and transitional rainforest vegetation of Southwestern Ethiopia. SINET Ethiop J Sci 25:155–175
Yeshitela K, Bekele T (2003) The woody species composition and structure of Masha Anderacha forest, Southwestern Ethiopia. J Biol Soc Ethiopia 2(1):31–48
Yeshitela K, Shibru S (2004) Floristic composition and phytogeographic comparison of moist montane forests of Southwest Ethiopia. In: Girma B, Yeshitela K, Bekele T (eds) Proceedings of a national conference on forest resources of ethiopia: status, challenges and opportunities 27–29 November 2002. Addis Ababa, Ethiopia
Yilak D, Debelo DG (2019) Impacts of human resettlement on forests of Ethiopia: the case of Chamen-Didhessa Forest in Chewaka district, Ethiopia. J Hortic for 11(4):70–77
Zumeta DC, Ellefson PV (2000) Conserving the biological diversity of forests: program and organizational experiences of state governments in the United States. Environ Manag 26:393–402
Download references
Acknowledgements
The authors would like to thank the people in and around the Sele-Nono forest for their cooperation during data collection.
This research has been funded by Addis Ababa University and Debre Markos University (Both in Ethiopia).
Author information
Authors and affiliations.
Department of Plant Biology and Biodiversity Management, the National Herbarium, Addis Ababa University, P. O. Box 3434, Addis Ababa, Ethiopia
Alemayehu Kefalew & Sebsebe Demissew
Center for Environmental Science, Addis Ababa University, P. O. Box 1176, Addis Ababa, Ethiopia
Teshome Soromessa
Department of Biology, Debre Markos University (DMU), P. O. Box 269, Debre Markos, Ethiopia
Alemayehu Kefalew
You can also search for this author in PubMed Google Scholar
Contributions
Research design, data collection, data analysis, writing drats manuscripts AK; review and editing, supervision TS and SD. All authors read and approved the final manuscript.
Corresponding author
Correspondence to Alemayehu Kefalew .
Ethics declarations
Ethics approval and consent to participate.
Not applicable, the study involves no human participants.
Consent for publication
Not applicable.
Competing interests:
The authors declare no conflict of interest.
Additional information
Publisher's note.
Springer Nature remains neutral with regard to jurisdictional claims in published maps and institutional affiliations.
Supplementary Information
Additional file 1: appendix s1..
GPS points for sample plots used for the study.
Additional file 2: Appendix S2.
Modified Braun-Blanquet scale for cover-abundance values (Van der maarel, 2005).
Additional file 3: Appendix S3.
Checklist used to determine level of disturbance of a sample plot in Sele-Nono forest.
Additional file 4: Appendix S4.
Semi-structured interview checklist.
Additional file 5: Appendix S5.
Lists of plant species recorded from Sele-Nono forest.
Additional file 6: Appendix S6.
new species records to Illubabur (IL) floristic region in the flora of Ethiopia and Eritrea.
Additional file 7: Appendix S7.
Endemic plant recorded from Sele-Nono forest.
Additional file 8: Appendix S8.
Outlier species excluded from the analysis.
Additional file 9: Appendix S9.
Synoptic value of plants species for each clusters.
Additional file 10: Appendix S10.
Enviromental data recorded for each sample plot in Sele-Nono forest.
Rights and permissions
Open Access This article is licensed under a Creative Commons Attribution 4.0 International License, which permits use, sharing, adaptation, distribution and reproduction in any medium or format, as long as you give appropriate credit to the original author(s) and the source, provide a link to the Creative Commons licence, and indicate if changes were made. The images or other third party material in this article are included in the article's Creative Commons licence, unless indicated otherwise in a credit line to the material. If material is not included in the article's Creative Commons licence and your intended use is not permitted by statutory regulation or exceeds the permitted use, you will need to obtain permission directly from the copyright holder. To view a copy of this licence, visit http://creativecommons.org/licenses/by/4.0/ .
Reprints and permissions
About this article
Cite this article.
Kefalew, A., Soromessa, T. & Demissew, S. Plant diversity and community analysis of Sele-Nono forest, Southwest Ethiopia: implication for conservation planning. Bot Stud 63 , 23 (2022). https://doi.org/10.1186/s40529-022-00353-w
Download citation
Received : 02 December 2021
Accepted : 02 July 2022
Published : 19 July 2022
DOI : https://doi.org/10.1186/s40529-022-00353-w
Share this article
Anyone you share the following link with will be able to read this content:
Sorry, a shareable link is not currently available for this article.
Provided by the Springer Nature SharedIt content-sharing initiative
- Canonical correspondence analysis
- Community types
- Multivariate technique
- Sele-Nono Forest
Theses and Dissertations Collection
Open Access Repository of University of Idaho Graduate ETD
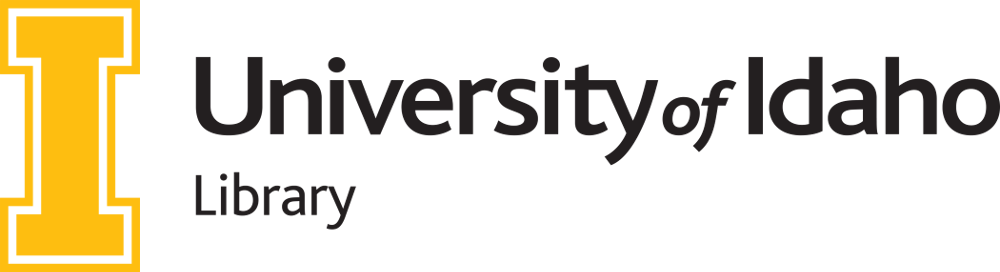
- Disentangling drivers of floristic diversity in island systems using phylogenetic...
Disentangling drivers of floristic diversity in island systems using phylogenetic approaches
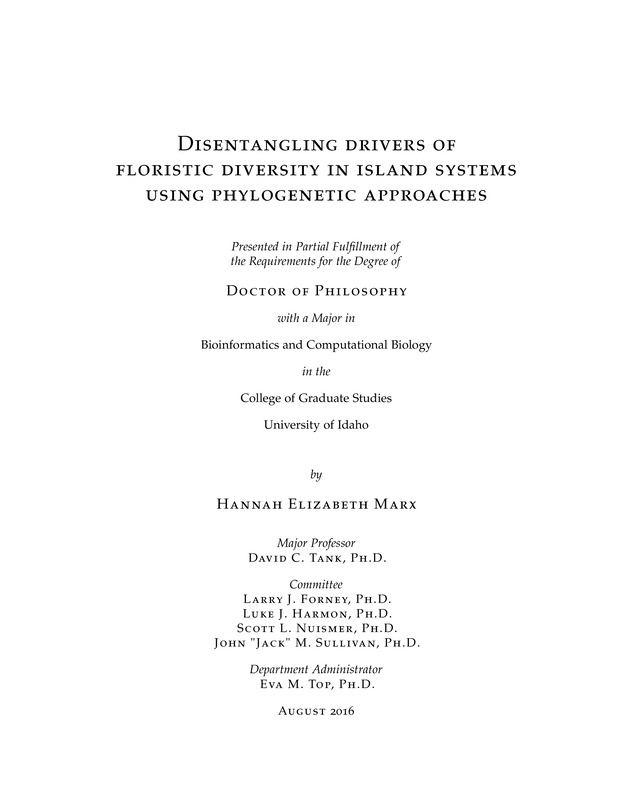
Marx, Hannah Elizabeth. (2016). Disentangling drivers of floristic diversity in island systems using phylogenetic approaches. Theses and Dissertations Collection, University of Idaho Library Digital Collections. https://www.lib.uidaho.edu/digital/etd/items/marx_idaho_0089e_10882.html
The complexity of nature is awe inspiring. Identifying patterns is the first step towards understanding how this complexity arose and predicting what will come of it in the future. There are many ways to describe diversity. Measures that include phylogenetic diversity can provide a foundation for assessing hypotheses about ecological processes driving the patterns we observe. In this dissertation, I explore how incorporating the evolutionary history of coexisting species can help to disentangle patterns of diversity at macro-ecological scales. Islands have provided ideal study systems for evolutionary studies since the beginning of the field. I turn to the classical natural "experiments" that island-like systems provide, where many replicates of simplified microcosms represent the global variation in biology and geography.
I begin by introducing a few ways phylogenetic methods have been employed to understand diversity patterns, and discuss some of the challenges and pitfalls of this approach. I explain how evolutionary history can be used to interpret processes driving diversity in species assemblages at macro-scales when specific hypotheses are used with explicit null models. In summary, I postulate about how to improve the community phylogenetic approach with consistent comparable methods, more sophisticated null models, and novel datasets to quantify and qualify diversity.
Darwin's Naturalization Conundrum presents a clear hypothesis to address macro-ecological patterns of species invasion. In the second chapter, I quantify patterns in both functional trait differences and phylogenetic distances between invasive species and the native flora they invaded across 80 uninhabited islands in the San Juan Islands. I compare these trait-based and evolutionary distance-based measures of diversity, and discuss their implications for understanding invasion dynamics. Across the archipelago, invasives are closely related to natives but differ in ecologically relevant functional traits. These opposing trends may be informing particular stages in the invasion process.
Approaching the limits of plant life, mountains inspired Humboldt to synthesize his thoughts about how species diversity changes across space, and alpine summits constitute some of the last frontiers for biodiversity surveys. To understand the floristic patterns on pristine "sky islands" across the French Alps, I use nested species pools and explicit null models to assess the environmental, historical, and neutral processes that could contribute to the phylogenetic patterns we observe. Although extreme environmental conditions on mountain summits are expected to pose a strong filter and induce phylogenetic clustering of closely related species, we find that stochastic models of speciation, colonization, and local extinction can explain the phylogenetic patterns just as well. This demonstrates the importance of clear hypothesis testing to infer processes that generate phylogenetic diversity patterns.
The primary obstacle to a synthesis of phylogenetic patterns of diversity observed in natural communities or multi-lineage assemblies are comparable methods, especially for phylogeny reconstruction. In the final chapter, I introduce one novel approach for collecting sequence data for well-resolved community phylogenies. High-throughput sequencing approaches can be utilized to overcome one obstacle for describing diversity in remote regions---efficient genetic sequencing of entire species assemblages. I illustrate the efficacy of this approach by describing the diversity of a remote alpine flora in Central Idaho. Similar to the French Alps, stochastic patterns are dominant within and between summits. This suggests that although adaptations to extreme alpine environments drive diversity within particular lineages, species-neutral processes might dictate macro-ecological patterns at regional scales.
Together, these chapters exemplify how the evolutionary history of species assemblages can unravel dominant ecological and evolutionary processes that generate such elaborate complexity observed in nature. As more data are compiled and better models are developed, we continue to unify the patchwork of species distributions and might someday reach our ultimate goal---to provide a predictive framework for anticipating diversity dynamics across the globe.
Contact us about this record
Investigating the Role of Local Floristic Diversity in Provisioning Wild Bees in Pennsylvania Apple Orchards

- Request paper in alternate format.
- Research article
- Open access
- Published: 07 March 2017
Exploring the floristic diversity of tropical Africa
- Marc S. M. Sosef 1 ,
- Gilles Dauby 2 , 12 , 21 ,
- Anne Blach-Overgaard 3 ,
- Xander van der Burgt 4 ,
- Luís Catarino 5 ,
- Theo Damen 6 ,
- Vincent Deblauwe 2 , 7 , 8 , 22 , 23 ,
- Steven Dessein 1 ,
- John Dransfield 4 ,
- Vincent Droissart 7 , 9 , 20 ,
- Maria Cristina Duarte 5 ,
- Henry Engledow 1 ,
- Geoffrey Fadeur 7 ,
- Rui Figueira 10 , 11 ,
- Roy E. Gereau 9 ,
- Olivier J. Hardy 12 ,
- David J. Harris 13 ,
- Janneke de Heij 14 , 15 ,
- Steven Janssens 1 ,
- Yannick Klomberg 14 , 16 ,
- Alexandra C. Ley 17 ,
- Barbara A. Mackinder 4 , 13 ,
- Pierre Meerts 7 , 18 ,
- Jeike L. van de Poel 14 ,
- Bonaventure Sonké 8 ,
- Tariq Stévart 1 , 7 , 9 ,
- Piet Stoffelen 1 ,
- Jens-Christian Svenning 3 ,
- Pierre Sepulchre 19 ,
- Rainer Zaiss 20 ,
- Jan J. Wieringa 6 , 14 &
- Thomas L. P. Couvreur 2 , 8 , 14
BMC Biology volume 15 , Article number: 15 ( 2017 ) Cite this article
18k Accesses
122 Citations
48 Altmetric
Metrics details
Understanding the patterns of biodiversity distribution and what influences them is a fundamental pre-requisite for effective conservation and sustainable utilisation of biodiversity. Such knowledge is increasingly urgent as biodiversity responds to the ongoing effects of global climate change. Nowhere is this more acute than in species-rich tropical Africa, where so little is known about plant diversity and its distribution. In this paper, we use RAINBIO – one of the largest mega-databases of tropical African vascular plant species distributions ever compiled – to address questions about plant and growth form diversity across tropical Africa.
The filtered RAINBIO dataset contains 609,776 georeferenced records representing 22,577 species. Growth form data are recorded for 97% of all species. Records are well distributed, but heterogeneous across the continent. Overall, tropical Africa remains poorly sampled. When using sampling units (SU) of 0.5°, just 21 reach appropriate collection density and sampling completeness, and the average number of records per species per SU is only 1.84. Species richness (observed and estimated) and endemism figures per country are provided. Benin, Cameroon, Gabon, Ivory Coast and Liberia appear as the botanically best-explored countries, but none are optimally explored. Forests in the region contain 15,387 vascular plant species, of which 3013 are trees, representing 5–7% of the estimated world’s tropical tree flora. The central African forests have the highest endemism rate across Africa, with approximately 30% of species being endemic.
Conclusions
The botanical exploration of tropical Africa is far from complete, underlining the need for intensified inventories and digitization. We propose priority target areas for future sampling efforts, mainly focused on Tanzania, Atlantic Central Africa and West Africa. The observed number of tree species for African forests is smaller than those estimated from global tree data, suggesting that a significant number of species are yet to be discovered. Our data provide a solid basis for a more sustainable management and improved conservation of tropical Africa’s unique flora, and is important for achieving Objective 1 of the Global Strategy for Plant Conservation 2011–2020.
Documenting the distribution of biodiversity is the first and most fundamental step for effective conservation and sustainable utilisation of natural resources for the future [ 1 ]. Tropical Africa [ 2 ] (Fig. 1 ) is home to some of the most important species-rich biodiversity regions in the world [ 3 ]. From the second largest extent of continuous rain forest in the world, the Congo basin, to the Namib dessert, tropical Africa is a land of strong biodiversity contrasts [ 4 ]. Yet, it has already lost large amounts of its ‘wilderness areas’ [ 5 ], i.e. areas where ecological and evolutionary processes are little affected by human disturbance. In addition, future climate change is expected to have important negative effects on sub-Saharan ecosystems, with an estimated 90% of species loosing part or most of their areas of suitable climate by 2085 [ 6 ]. These results call for immediate international policies to be put in place [ 5 , 7 , 8 ]; however, they will be hard to achieve without a better understanding of plant diversity and its distribution.
Distribution of botanical records across tropical Africa. Number of specimens ( a ) and observed species richness ( b ) per 0.5° sampling units. Dashed lines represent the limits for tropical Africa as defined in our study. Map based on georeferenced herbarium records, silica gel samples and plot data
Herbaria around the world are the keepers of such essential data for plants [ 7 , 9 , 10 ]. Together, they curate a vast databank of about 350,000,000 physical dried plant specimens [ 11 ]. Any conserved specimen provides unique proof of where and when a particular species was present. With ongoing and intensified efforts of natural history institutions to digitize their scientific heritage, the possibility of providing large amounts of data to a much wider audience has rapidly expanded over the last few years [ 12 – 16 ] (see [ 17 ] for an overview of the number of scanned herbarium specimens in the world’s largest virtual herbaria).
Relying on such data, several large-scale initiatives have been undertaken focusing on, for example, the tree flora of the Amazon basin [ 18 – 20 ], enabling access to more detailed estimates on plant diversity and distribution in this species-rich region. To date, however, our knowledge of tropical plant distribution remains limited [ 21 – 23 ] and tropical Africa is no exception to the rule [ 24 , 25 ]. Indeed, total species estimates for different countries, regions and biomes are lacking or are approximate estimates at best. For example, White [ 26 ] in his much-cited The Vegetation of Africa provided broad estimates of plant species diversity for each of his recognised ‘phytochoria’, numbers that are still used today. However, these values were not based on any formal analyses of biodiversity data, but rather on expert opinion. In addition, there have been numerous efforts to estimate the total number of tree species across the tropics, either in general for the tropics [ 27 ] or for specific regions such as the Amazon basin [ 20 ]. Few estimates of total tree diversity for Africa have been advanced. Using extrapolations from global plot data, Slik et al. [ 27 ] estimated a minimum number of 4626–5984 tree species for Africa (including Madagascar). In general, tropical Africa is deemed to be botanically less diverse than regions at similar latitudes in South America and South East Asia [ 27 – 29 ]. Numerous hypotheses exist which try to explain this ‘odd man out’ pattern (reviewed in [ 28 ]) but no clear conclusive explanation has yet been provided.
During the last 15 years, efforts to database tropical African plants have been undertaken, but generally with the purpose of analysing large scale phytogeographical patterns across Africa [ 30 – 32 ]. Thus, these datasets are characterised by an overrepresentation of well-known and comparatively widespread species. More recently, Stropp et al. [ 25 ] extracted and analysed all flowering plant data openly available via the Global Biodiversity Information Facility (GBIF, http://www.gbif.org ) for Africa (including Madagascar). They concluded that the quality and completeness of species-occurrence data of flowering plant species available through Biodiversity Information Systems such as GBIF is low. In addition, over half of the records they retrieved did not relate to tropical Africa but to South Africa and Madagascar. Thus, to date, large uncertainties remain about plant species diversity and distribution across tropical Africa. The digitization and georeferencing of major African-specialised herbarium collections have substantially progressed these past years [ 16 , 17 ]. When these independent efforts are compiled and concatenated into a single quality-checked comprehensive mega-database, they will provide new and detailed insights into tropical African plant diversity and distribution as never achieved before [ 33 ].
The goal of this paper is to document the main floristic patterns and information gaps for tropical Africa. For this, we use the largest and highest quality mega-database of vascular plant species distributions ever compiled for tropical Africa (RAINBIO, [ 33 ]). First, we explore the spatial distribution of records and species diversity statistics at regional and country levels and ask the following questions: how is plant species diversity distributed across tropical Africa? How many plant species are there in tropical African forests? How is this diversity partitioned in terms of growth form? Which regions have the highest floristic turnover rates? Second, we analyse the level of botanical exploration across tropical Africa and ask the following questions: have tropical African countries been adequately explored? What regions are well sampled and which are not? Can we identify areas where future sampling would be the most efficient? Finally, we conclude with a series of recommendations in order to improve our knowledge of the floristic diversity of tropical Africa.
Data and study area
All analyses were performed using different subsets (i.e. with or without silica gel information, see below) of the RAINBIO database [ 33 ]. In short, RAINBIO is a compilation of:
publicly available datasets mainly from international herbaria;
personal ones such as datasets on palms, legumes, orchids, Rubiaceae, Marantaceae, flora of Gabon, flora of the Dzanga-Sangha region (Central African Republic);
tree plot inventory data from Gabon;
georeferenced silica-dried samples for tree species from Central Africa.
The data were checked automatically and manually via experts for quality in several ways, including geographic and taxonomic standardisation, merging of duplicate records (originating from different datasets), and exclusion of cultivated/non-native plants. A full description of RAINBIO, how it was compiled and how the data were checked and verified can be found in Dauby et al. [ 33 ]. The RAINBIO dataset contains important additions and a significant increase in data quality compared to the data available through the GBIF (see, [ 25 ] for biodiversity analyses of data only available via GBIF), and has a strong focus on tropical Africa [ 33 ].
Our study area is tropical Africa, which Klopper et al. [ 2 ] broadly defined as sub-Saharan Africa and excludes southern Africa and Madagascar (Fig. 1 , roughly between 16°N and 20°S). For delimitating this study area, we relied on the ecoregions defined by Olson et al. [ 34 ] and used the eco-region ‘south Saharan steppe and woodlands’ as the northern limit and ecoregions ‘Namibian savanna woodlands’, ‘Kalahari xeric savanna’, ‘Kalahari Acacia-Baikiaea woodland’, ‘Highveld grasslands’ and ‘Drakensberg montane grassland’ as the southern limits. We filtered the RAINBIO dataset so species having all of their occurrence records outside this region were excluded.
Sampling unit size
In our study, we used two different sampling unit (SU) sizes. First, we simply used a ‘fixed-size’ SU of 0.5° (ca. 55 × 55 km at the equator). For certain analyses, however, we used an ‘adaptive resolution’ SU method [ 35 ]. This approach adapts the size of the SU as a function of a user-defined threshold of minimum occurrence records (see [ 35 ] for details). This method allows one to consider smaller SUs where record density is high, while increasing SUs size where records are sparsely distributed. The advantage is that record numbers remain broadly constant across SUs. In order to create an adaptive SU grid we uploaded the filtered RAINBIO database to the Infomap Bioregions [ 35 ] website ( http://bioregions.mapequation.org ). We used the following parameters: maximum cell capacity = 1000; minimum cell capacity = 500; maximum cell size = 8°; minimum cell size = 0.5°. The SU grid was then downloaded as a shapefile and processed in the R environment for excluding unsuitable areas (ocean and lakes) in each SU.
Species diversity patterns
Species richness was estimated at two different levels: (1) for each fixed-size SU of 0.5° containing at least 100 records, and (2) at country level. Only herbarium specimens were included for this estimation. Plot data and silica gel-dried DNA samples were excluded because of their stronger focus on particular taxa, which are often over-sampled and would introduce a bias. In each case, an observed and estimated total species number was calculated. For the observed species number per country we used georeferenced and non-georeferenced specimens. As species richness estimation is strongly affected by sampling efforts, we computed two complementary richness estimators. First, we calculated a non-parametric species richness estimator [ 36 ], known as the Chao1 [ 37 ], Ŝ , defined as:
Where a 1 is the number of species represented by one specimen (singleton), a 2 is the number of species represented by two specimens (doubleton), S obs is the observed number of species and N is the total number of specimens. Second, we estimated species richness using a subsampling procedure. This approach has proven to be robust when dealing with incomplete and heterogeneous sampling [ 38 – 40 ]. We used the Nielsen’s estimator N e [ 41 ] of effective number of species [ 39 ], which corresponds to a nearly unbiased estimator of the popular Gini-Simpson diversity index [ 37 ] converted into effective number of species [ 38 ].
This estimator is defined as [ 41 ]:
Where N is the total number of specimens, p i is the frequency of the i th species and S is the observed number of species. This estimator does not estimate total species richness, but expresses the diversity in terms of ‘effective number of species’. In addition to its good statistical properties [ 38 ], this metric has the advantage of satisfying the ‘replication principle’ [ 37 ]. This basically means that the ratio of diversity values reflects ‘true’ differences in diversity just as actual species richness would do [ 42 ].
Floristic turnover rates
We assessed the level at which floristic composition changes in space by computing a ‘neighbourhood’ species turnover rate applying a three-step procedure (Additional file 1 ). For this parameter, we used the adaptive resolution SU grid as defined above.
Step 1. For a focal SU, a convex hull was drawn around all specimen occurrences it contained. A given buffer of distance μ was added to the hull convex. All other SUs with at least 500 records included in this buffered area were selected for comparison.
Step 2. The pairwise floristic similarity was computed as 1– β sim between all selected SUs. The turnover index β sim was defined as [ 43 ]: \( {\beta}_{sim}\frac{min\left( b, c\right)}{a+ min\left( b, c\right)} \) where b and c are the number of species restricted to the first and second SU, respectively, while a is the number of species shared by the two cells.
Step 3. The geographical distance among all pairs of selected cells was computed based on centroids of occurrences within each SU.
The decay of the floristic similarity with geographical distance was then approximated by a linear model. From this model, we extract the halving distance which was the geographical distance at which the floristic similarity was reduced to 50% [ 44 ]. Hence, low halving distances indicated high floristic turnover per distance unit. By changing the distance which determines the neighbourhood SUs that are taken into account ( μ ), one can investigate different scales of floristic turnover (small μ = fine-scale; large μ = large-scale) (see Additional file 1 for illustration of the method). Here, we investigate two values of μ : μ = 1°, representing a meso-scale floristic turnover, and μ = 2°, representing a large scale floristic turnover.
Sampling completeness
Two estimates of sampling completeness were computed. First, the relative exploration was estimated as the percentage of species already discovered: observed species divided by the estimate of total number of species by Chao1. Second, the sampling coverage estimator of [ 45 ] was calculated as:
A sampling coverage of 1 indicates that all species have been collected twice or more, while for a value of 0 all species are known by a single record only.
We identified the ‘best-explored’ countries, namely countries with a relative exploration value above 0.85 and sampling coverage higher than 0.95. However, this does not imply they were botanically well known, but rather, compared to others, they had the best data available to date. Figures for countries where the number of specimens was lower than the estimated number of species were deemed unreliable and thus not included in the results; obviously, these countries were poorly explored (or our data for them was largely incomplete).
Using adaptive resolution SUs we identified three major categories of SUs depending on their level of sampling and coverage. First, we defined ‘well-sampled units’ (WSU) representing SUs with a minimum collection density of 100 records for 100 km 2 [ 46 ] and a sampling coverage estimator equal to or higher than 0.5. Second, we defined ‘top sampled units’ (TSU) as the top 25% of SUs with the highest density records and sampling coverage estimator equal to or higher than 0.5 and excluding WSUs. Third, we proposed a number of ‘priority sampling units’ (PSU) defined as a SU where meso-scale species turnover (see Floristic turnover rates section) was estimated to be high (the top 20% of SUs with lower halving distances), but where sampling completeness was low (smaller than 0.5). Thus, PSUs translate the idea that sampling should focus on poorly known areas with sharp floristic gradients. For example, SUs with low coverage but located in low turnover regions will be important to explore but might not lead to the addition of many new species for the region, if a nearby SU has already been well sampled. This approach should be viewed as complementary to other methods that have identified data-deficient areas in Africa [ 24 , 25 ].
Finally, we estimated the level of sampling completeness across all tropical African species. For that, we assessed the number of specimens for each species as a function of the number of fixed-sized SUs occupied by that species. A scatter plot was then produced where each point represented a species, and the slope represented the average number of specimens per grid cell for all species. In order to have an idea of the historical evolution of this value through time, we generated the same plots but at 13 different time slices in the past, going back every 10 years until 1900.
All computations were done within the R statistical software (R Core Team 2015) using the vegan package [ 47 ] for the Chao1 estimator, entropart [ 48 ] for the sampling completeness estimator, betapart [ 49 ] for the β sim computation and an R function built by G. Dauby to estimate the Nielsen parameter (Additional file 2 ).
Estimation of species distributions
The distribution surface, or range size, of each species was estimated by calculating the surface within the convex hull formed by all specimen localities of that species. Large salt water bodies were excluded from the surface measurements. Species with a single georeferenced specimen were assigned an arbitrary range size of 1 km 2 . For species known from only two georeferenced specimens, the range size was estimated arbitrarily by multiplying the distance between the two localities by 0.1.
Growth form diversity
Most species (91%) recorded in RAINBIO were able to be categorised into nine different growth form types (tree, shrub, herb, liana, vine, aquatic herb, epiphyte, mycoheterotroph and parasitic) [ 33 ]. For detailed information on how this was achieved, please see [ 33 ]. In short, main categories were automatically assigned through an ad hoc custom R script by extracting keywords for each habit from available herbarium specimen label note information. A total of 4751 species names (22% of the total filtered dataset, see below) were manually checked by experts in order to confirm automatic assignments (when two or more habits were suggested, 2823 species) and to fill in missing assignments (1928 species). Besides these specific cases, a large majority of habit assignments were confirmed (but no proportion can be provided). We considered species to be woody if they were classified as trees, shrubs or lianas, and as herbaceous when they were classified as herbs, vines, aquatics or mycoheterotrophs (parasitic species were not included because they are a mixture of both woody and herbaceous species). For each adaptive resolution SU we generated the total number of species, and the ratio of the number of species in each of five growth forms: (1) herbs, vines and mycoheterotrophs, (2) shrubs, (3) liana, (4) trees, and (5) epiphytes.
African tropical forest diversity
In order to provide species diversity values for African tropical forests, we used the map of Mayaux et al. [ 50 ] depicting land cover types across Africa and Madagascar for the year 2000. The map consists of 27 different land cover layers. We selected six of them that consisted mostly, or originally, of tropical forests, namely evergreen forests, degraded evergreen forests, submontane forests, montane forests, swamp forests and mosaic forest-croplands. The original resolution of the map is 1 km 2 . We aggregated the selected land cover at a resolution of 0.1° (ca. 11 km 2 ) because our georeferenced records are generally not that precise. The resulting forest area layer was then arbitrarily divided into a West African block (west to the Dahomey Gap), a central African block (east of the Dahomey Gap, west of the East African rift), and an eastern African block (east of the East African rift) (Additional file 3 ). Using these subunits we estimated the number of records, species richness, and number of sub-endemics (considered as a species with 90% or more of its records located within the forest layer) in total and for five different growth forms defined above for African forests in general and for each forest bloc (west, central and east).
Spatial distribution of data
The original unfiltered RAINBIO database comprises distribution data for 25,356 species of vascular plants, 3158 genera and 273 families from a total of 614,022 records. The filtered dataset for tropical Africa that was used for our analyses had a total of 609,776 specimens representing 22,577 species. This represents the largest and highest quality dataset ever compiled for tropical African plants. The records are well distributed but heterogeneous across the continent (Fig. 1a ). The highest collecting efforts are concentrated in West Africa (Liberia to Benin), south-western Cameroon, Equatorial Guinea, Gabon, the region covering the eastern part (Kivu) of Democratic Republic of the Congo (DRC) plus Rwanda and Burundi, and the Eastern Arc Mountain range in Kenya and Tanzania (Fig. 1a ). Although to a lesser extent, records are also well represented in western and south-eastern DRC and Ethiopia. In contrast, there are comparatively few records in most of the Sahelian region (Senegal to South Sudan), the Ogaden (south-eastern Ethiopia), the Central African Republic, Nigeria, the Republic of Congo and Angola.
Our data indicates that just 21 SUs of 0.5° contain more than 3000 records or 100 records per 100 km 2 (Additional file 4 ). The observed species richness highlights several regions (Fig. 1b ), with the following areas standing out: the Nimba Mountains in north Liberia, the Cameroonian Volcanic Line in western Cameroon, the regions around Kribi, Bipindi and Yaoundé in Cameroon, Rio Muni (continental part of Equatorial Guinea), several areas in Gabon such as the surroundings of Libreville and Makokou, around Yangambi in DRC, the Eastern Arc Mountain range in Tanzania, Abidjan in Ivory Coast and the south of Benin (Fig. 1b ). The observed species richness is highly correlated with specimen density (Pearson correlation R = 0.91; compare Fig. 1a ). Estimated total species richness for each SU based on the Chao1 (Fig. 2a ) is highly correlated to both the number of records per SU (Fig. 1a ; Pearson correlation R = 0.85) and the observed species richness (Fig. 1b , Pearson correlation R = 0.91). In contrast, the Nielsen diversity estimator computed for each 0.5° SU (Fig. 2b ) is much less correlated with specimen density (Pearson correlation R = 0.28) than the Chao1 estimator.
Estimated botanical diversity of tropical Africa. a Estimated species diversity based on the Chao1 estimator for each 0.5° sampling units (SUs) with more than 100 records. b Effective number of species estimated using the Nielsen statistic per 0.5° SUs with more than 100 records. Dashed lines represent the limits for tropical Africa as defined in our study. This map is based on georeferenced herbarium records, silica gel samples and plot data
A total of 3438 species have been collected just once and 8026 collected less than 5 times, while 3172 species have been collected more than 50 times (Additional file 5 a). A similar picture is found when looking at the number of 0.5° SUs occupied per species (Additional file 5 b). Over 4294 species are only recorded in a single SU (narrow endemics), while 1607 species occur in 50 SUs or more (widespread). The range size distribution of all species (Additional file 5 c) shows a relatively low number of exceptionally widespread species (i.e. more than 16,777,216 km 2 ). Species with an ‘intermediate’ range size (i.e. between 8192 and 16,777,216 km 2 ) are most common, while species with small distribution areas are again less common. However, because of the quadratic scale used on the x-axis (to show more detail in the lower categories), the picture actually shows an ever increasing number of species along the x-axis.
The average number of records collected per species per SU is 1.84, calculated as the slope of the linear fit of the numbers of specimens for each species as a function of the number of SUs it occupies (Fig. 3 ). Species that cluster on the lower left of the graph are limited in distribution while those to the right are comparatively widespread. Species that fall above the red line are collected more often than average while those under the red line are relatively under-collected. Rare species (left end of the plot) that fall above the red line are either locally common (clustered) or have been well-collected (e.g. by collectors focussing on specific groups), while those below the red line are comparatively scattered. Widespread species (right end of the plot) falling above the red line are comparatively abundant (widespread common) or have a positive collecting bias, those below the red line occur as scattered individuals (widespread rare) or are otherwise inconspicuous (minute or seasonal plants, like small saprophytes) or more difficult to collect (palms, large trees, aquatic plants or plants only flowering in the canopy) or have a negative collecting bias (e.g. weeds).
Spatial distribution of specimens per species. Each dot represents a species with its number of specimens against the number of 0.5° sampling units (SUs) it occupies. The slope of the red line (linear regression) indicates the average number of specimens per SU for all species. The grey line (slope equal to one) indicates when the number of specimens equals the number of occupied SUs
Temporal distribution of collections
Collecting dates ranged from 1782 to 2015. Collecting intensity across tropical Africa through time has generally increased, though with lower collecting efforts in the early 1980s, to 2005, after which there has been a significant decline to the present (Fig. 4a ). Collecting intensity per country shows different histories, with different periods of intense and low collecting efforts (Additional file 6 ). For example, DRC knew its highest period of botanical collections from the 1930s to the 1960s (Additional file 6 ). In contrast, Benin has experienced intense collecting over the last 20 years (Additional file 6 ). Other countries, such as Cameroon, have known a sustained and important collecting intensity since the 1960s up until 2010.
Temporal distribution of collecting efforts. a Number of herbarium records for tropical Africa per 5-year slices from 1782 to 2015. b Temporal evolution of the average number of specimens per SU for all species. Plots based on georeferenced herbarium records only
By plotting the average number of records collected per species per SU in function of 10-year time slices over 130 years, the slope is increasing, except for two periods (1941–1950 and 1961–1970) where the slope decreased (Fig. 4b ). This is corroborated by a steady decrease of newly explored SUs since 1980 (Additional file 7 ).
In order to have a ‘time lapse’ view of the collecting history throughout tropical Africa, we plotted the year of the oldest collection per SU from 1780 up to 2015 (Fig. 7 , see Additional file 8 ). The first regions to be prospected (shades of blue in Fig. 5 ) are along the coast lines of East Africa (Kenya and Tanzania), central Africa (Cameroon, Equatorial Guinea, Gabon and Nigeria) and West Africa (Benin, Ghana, Guinea, Ivory Coast and Sierra Leone). Main rivers, such as the Congo River, were also explored during that time as well as mountain regions such as the Eastern Arcs in Tanzania, the Cameroonian Volcanic Range in Cameroon and the Crystal mountains in Gabon. The map also reveals areas that have been prospected only recently (shades of red in Fig. 5 ) such as the north of Benin, the Republic of Congo and the Haut-Ogooué region in Gabon. Some large areas that are still to be botanically explored (white in Fig. 5 ) are present along the southern margin of the Sahara, in eastern Chad, Sudan and South Sudan, northern and eastern Angola, and parts of the Ogaden desert in Ethiopia.
Time lapse of botanical collecting history across tropical Africa. The map represents the date of the first botanical collection made within each 0.5° sampling unit. Dashed lines represent the limits for tropical Africa as defined in our study. Map based on georeferenced herbarium records, silica gel samples and plot data. An animated gif version of this map is available at: http://rainbio.cesab.org
Tropical African turnover rates
High large-scale turnover rates (low halving distance values, Fig. 6a ) are present in areas with high topographical heterogeneity, for example, along the Cameroonian Volcanic Range, the Albertine Rift or around the Nimba Mountains area, and forest-savannas transitional areas. The meso-scale turnover map (Fig. 6b ) shows broadly similar areas, but also some striking differences. Some additional heterogeneous areas are identified, such as the regions around Libreville in Gabon and around Kribi and Bipindi in Cameroon, while others become homogeneous at the large scale.
Floristic turnover rates across tropical Africa. Values based on adaptive resolution sampling unit (explanation see text). Pairwise floristic similarity is computed as 1–β sim turnover index using two values of μ (see section in Methods and Additional file 1 ). a Meso-scale floristic turnover rate with μ = 1°; b Large-scale turnover rate with μ = 2°. Dashed lines represent the limits for tropical Africa as defined in our study. Maps based on georeferenced herbarium records, silica gel samples and plot data
Botanical exploration intensity
The observed number of species and level of exploration of a selection of countries are provided in Table 1 . The estimated botanically best-explored countries (Table 1 , relative exploration index ≥ 0.85) are Cameroon, Benin, Gabon, Liberia and Ivory Coast. When looking at the sampling completeness, DRC and Tanzania can be added to this list (Table 1 ). The botanically least explored countries (relative exploration index ≤ 0.65) are Angola, Somalia, Botswana, Republic of Congo, Guinea-Bissau and Zimbabwe.
Our results show that big portions of tropical Africa remain poorly explored, which is illustrated by the numerous large grey coloured SUs (areas that do not attain our threshold values for adequate botanical exploration; Fig. 7 ) located in, for example, Angola, DRC or Nigeria. Only 34 variable sized SUs can be qualified as being ‘well sampled’ (density above 100 records/100 km 2 and coverage over 0.5 WSUs). These are mainly concentrated in Cameroon, Gabon and Equatorial Guinea. The TSUs are mainly located in Atlantic central Africa (Cameroon, Gabon and Equatorial Guinea), parts of Tanzania, parts of the Kivu region, and south Benin. Finally, we highlight several regions as PSUs, mainly occurring in regions in West Africa, along the Cameroon Volcanic Line, eastern Gabon, and the Eastern Arcs and coastal forests of Tanzania. It is important to underline that this does not mean that other areas are not a priority, but simply indicates that, based on our data, the exploration of these SUs is more likely to improve our knowledge about tropical African plant biodiversity.
Level of botanical exploration across tropical Africa. Based on an adaptive resolution sampling units (SUs). This map shows priority SUs calculated based on a turnover rate using μ = 1°. Grey SUs represent SUs that did not meet any of our threshold limits (see text for explanation) and thus highlight SUs that are poorly documented. Dashed lines represent the limits for tropical Africa as defined in our study. Map based on georeferenced herbarium records, silica gel samples and plot data
Growth form information
Growth form data were recorded for 21,901 species or 97% of the filtered species list of RAINBIO. Our results suggest that the number of herbaceous species is around the same as the number of woody species (Table 2 ). The geographic distribution of plant growth form types across tropical Africa in terms of the proportion of species of a certain type shows contrasting patterns (Fig. 8 ). The herbaceous growth form has a high proportion in drier regions (Sahel, East Africa) where savannah prevails (Fig. 8a ). In contrast, the tree and liana growth forms have high ratios within the rain forest regions where climate seasonality is lowest (Fig. 8c, d ). Shrubs recorded average proportions in the Sahel region and high proportions in the horn of Africa region, for example, Somalia and northern Kenya (Fig. 8b ). Finally, epiphytes recorded high proportions in São Tomé and Príncipe, and montane areas such as the Cameroon Volcanic Line, Crystal Mountains area in northwest Gabon or Nimba Mountains in Liberia (Fig. 8e ).
Distribution of growth form diversity across tropical Africa. Based on an adaptive resolution sampling units (SUs; for explanation see text). Proportion of species of a given growth form type occurring in each SU. a Proportion of herbs; b Proportion of shrubs; c Proportion of lianas; d Proportion of trees; e Proportion of epiphytes. Dashed lines represent the limits for tropical Africa as defined in our study. Map based on georeferenced herbarium records, silica gel samples and plot data
When limited to tropical African forests (Additional file 3 ), RAINBIO records relate to a total of 15,387 vascular plant species, of which 3013 are scored as trees, 5755 as herbs, 1637 as lianas and 3158 as shrubs (Table 3 ). As expected, the central African forests represent the most species rich block with 10,306 species, followed by the east African forests with 6789 species and West Africa with 4396 species. The endemism rate for Central African forests is 29.1% (2997 out of 10,306 species endemic), 7.4% for east African forests (504 out of 6789 species) and 11.4% West African forests (503 out of 4396 species). The top 20 most species-rich families found in tropical African forests are provided in Table 4 .
The RAINBIO mega-database
The basis of the current analyses relies on data available through the RAINBIO mega-database [ 33 ]. As indicated in the methods section, we suggest that RAINBIO is the largest and most accurate database for tropical African plant distributions, as we undertook numerous data validation routines, automatic as well as manual via numerous African flora taxonomic experts. Recently, another dataset has been published [ 51 ], comprising 3.1 million records for 40,401 vascular plant species across the whole of Africa. However, this dataset was assembled to test a new method of identifying areas of important biodiversity conservation (Star ratings) and has not been specifically used to explore tropical African plant biodiversity.
We stress that, like all existing biodiversity databases, RAINBIO is not perfect. First, specific data associated with specimens can be erroneous, for example, misidentifications or errors in geo-referencing. Nevertheless, by having expert taxonomists validate large parts of the identifications and by treating information from duplicated herbarium specimens in a systematic manner (see recommendations section), we significantly limited errors and improved the overall quality of RAINBIO. Second, several important herbaria were not directly included such as the Muséum National d’Histoire Naturelle (P), the Conservatoire et Jardin botaniques, Geneva (G), the East African herbarium (EA) and partly the Royal Botanical Gardens, Kew (K) (acronyms follow, [ 11 ]). In general, specimens within those herbaria have not yet been databased or were not available at the time of this project. The impact of missing data has yet to be explored and could affect the results in various regions such as East Africa (EA, K) or West/Central Africa (P). However, it is important to underline that (1) a significant part of the data within these herbaria are indirectly available in RAINBIO (via duplicates distributed to other herbaria or via specimen databased with the framework of regional/country floras (e.g. [ 52 , 53 ]) or monographic revisions, information of which is included in RAINBIO), and that (2) our paper explores general tropical African plant biodiversity patterns for which RAINBIO provides a sound representation of all data.
The floristic diversity of tropical Africa
Botanical records across tropical Africa are well distributed but highly heterogeneous (Fig. 1a ). Continental or regional scale biodiversity data are typically distributed in an uneven and patchy way [ 20 , 24 , 40 ], related to differences in accessibility (presence of roads or rivers), focus of researchers, projects [ 54 ] or to colonial history. In tropical Africa, areas of higher record density are partly explained by focused collecting and databasing efforts of the main contributing herbarium institutions (acronyms follow [ 11 ]): BRLU for Equatorial Guinea, São Tomé and Príncipe and Gabon; MO for the Eastern Arc Mountains in Tanzania and Gabon; WAG for West Africa, Cameroon, Gabon and Ethiopia; BR for western DRC and the Kivu-Rwanda-Burundi region; and K for western Cameroon. In contrast, low record density is not only due to low collecting efforts (Central African Republic, Republic of Congo or Angola) but also to incomplete digitization and georeferencing of existing records, for example, in Angola [ 55 ] and DRC [ 56 ].
For tropical Africa, we record occurrences for 22,577 species, 2810 genera and 258 families of vascular plants. These values are lower than previous estimates for the region. Indeed, Klopper et al. [ 2 ] recorded a total of 32,424 angiosperm taxa, while Govaerts [ 57 ] estimated around 29,887 species for the same region. However, these values are not entirely comparable. First, they are based on databases (checklists) of published names (e.g. African Plant Checklist and Database Project; Kew World Checklist) rather than from herbarium specimens. Due to new insights in taxonomy, a significant number of those names have been marked as synonyms in our list, although we have also added many newly published species names. Second, Klopper et al.’s [ 2 ] values are for taxa (thus including infraspecific names) and for angiosperms only, while Govaerts’ [ 57 ] are restricted to species and seed plants (gymnosperms and angiosperms). Our values are for species and all vascular plants (pteridophytes, gymnosperms and angiosperms), and based on herbarium specimens complemented with silica gel data and plot inventories. Most dubious names or names of doubtful taxonomic status still present in checklists do not have any specimens linked to them in herbarium databases.
Several species-rich areas across tropical Africa (Fig. 2a ) are highlighted based on the Chao1 estimator (but see below), namely the Cameroon/Gabon forests, including Mont Cameroon, the east Kivu region and the Eastern Arc Mountains in Tanzania, with a less pronounced diversity centred in Katanga (south-eastern DRC). These results are consistent with those of several previous studies [ 30 – 32 ], but our results are derived from a significantly larger dataset. Larger areas with high species and specimen records can be linked to specific plant inventory efforts, for example, in most of Gabon [ 52 ], the Cameroon Volcanic Line in western Cameroon [ 58 – 60 ], Benin [ 53 ] and the Eastern Arc mountains [ 61 ]. Some small-scale instances of high species richness (isolated red SUs in Fig. 2a ) can be either attributed to single-person sampling campaigns (e.g. in south-western Tanzania, where A.F. Stolz (1871–1917) made ca. 2500 plant collections; in south-western DRC, where Masens Da Musa generally collected each species only once [ 62 ]; or in western Ghana, with specimens mainly collected by C. Jongkind) or by focused sampling and digitization efforts for the area, namely Yangambi (North-central DRC), where intense field work was undertaken by Belgian collectors combined with the digitization of the BR type specimens and large parts of the Yangambi general collections [ 63 ]; or the Nimba Mountains area (border between Liberia, Ivory Coast and Guinea), well documented by Adam [ 64 ], with more recent records from MO and WAG contained in RAINBIO.
The estimated species richness per SU based on Chao1 (Fig. 2a ) is highly correlated to both the number of records (Fig. 1a ) and the observed species richness (Fig. 1b ) per SU. This is a well-known artefact of biodiversity analyses [ 39 , 40 ] and is likely biased by the heterogeneous sampling effort. Part of the correlations can also be explained by collector’s behaviour, tending to collect in areas of known high species richness [ 24 , 54 ]. The Nielsen diversity estimator was proposed to correct for such heterogeneous sampling effort [ 39 ]. When computed for each 0.5° and 1° SUs (Fig. 2b ), this effective number of species is much less correlated with specimen density (Pearson correlation R = 0.28). Despite that high species diversity is confirmed in Lower Guinea and the Eastern Arc Mountains, there are some discrepancies between richness patterns shown by the Chao1 and Nielsen estimators. For example, estimated species richness based on Chao1 in the Kivu and Katanga regions of DRC and several areas in Lower Guinea are similar, while this pattern is not observed when using the Nielsen estimator. This suggests the Chao1 does indeed more strongly reflect the heterogeneous sample size rather than the true species diversity. Other differences are the high effective number of species observed in several areas in Ethiopia and West Africa, localities that were not emphasised by the Chao1; it is likely that these are artefacts in the Nielsen estimator. For example, the very high effective number of species detected in western Ghana (red SU, Fig. 2b ) is probably due to the intense collections of a single collector (C. Jongkind) in that area. Large parts of Gabon yield a very low Nielsen score, which must be a flaw in the Nielsen estimator, since several SUs were identified as well as top sampled units (WSU or TSU; Fig. 7 ), and our data (Fig. 1b ) confirm they are very species rich. It is nevertheless important to highlight that the Nielsen and Chao1 estimators are not fully adequate for herbarium specimens because they rely on the relative abundance of species within each SU (even if only singletons and doubletons are taken into account for Chao1 estimator), which is estimated by the number of specimens per species. However, the number of specimens per species is not a good proxy of the species’ population size [ 54 ]. Indeed, a collector generally does not collect plants at random, but usually tends to collect as many different species as possible. This would explain the unexpected high values of Nielsen estimator and Chao1 observed in SUs where many specimens were collected by a single or very few collectors. The SU in western Ghana with mainly collections by C. Jongkind does give an artefact in Nielsen, but not in Chao1, indicating that such collector-biases are not dealt with in the same way.
The diversity of tropical African forests
Our study documents a total of 15,387 vascular plant species occurring in tropical African forests (excluding Madagascar) of which ca. 30% are strict endemics (Table 3 ). To date, only rough estimates have been published, and these have generally not been restricted to forested regions but rather to certain major bioregions such as phytochoria [ 3 , 31 , 65 ]. White [ 26 ] estimated a total of 8000 plant species occurring in the Guineo-Congolian regional centre of endemism, 80% of which were endemics. This centre of endemism concerns West and Central Africa and encompasses species occurring in other ecosystems than forests such as savannahs and mountains. The values advanced by White [ 65 ] are based mainly on his expert opinion with little underlying quantitative information.
Adding to previous estimates of the tropical tree flora [ 20 , 27 ], our results suggest a total of 3013 tree species recorded for African forests (36% strict endemics). This estimate is below the recently estimated minimum number of 4626–5984 trees for Africa [ 27 ], but it provides, for the first time, a number based on solid underlying quantitative biodiversity data. However, the values of Slik et al. [ 27 ] include Madagascar and are based on forest plots where a tree is identified as having a diameter above breast height (DBH) of more than 10 cm. Recent studies have underlined that a significant portion of tree species diversity in African forests have a DBH smaller than 10 cm [ 66 , 67 ]. In our analyses, a species was considered a tree if explicitly mentioned on the specimen labels or coded as such [ 33 ], thus also includes species with DBHs smaller than 10 cm.
Our results confirm the observed ‘odd man out’ pattern [ 29 ] of relatively lower diversity of African forest tree species when compared to the Neotropics or South-East Asia [ 20 , 27 , 28 , 68 ]. For example, there are 11,676 tree species (DBH > 10 cm) recorded for the Amazon basin, more than three and a half times our figure for tropical Africa. Overall, the tropical African forest tree flora represents between 5% and 7.5% of the estimated total number of tree species for the whole of the tropics (between 40,000 and 53,000 tropical tree species, [ 26 ]). The numbers documented here, though likely underestimates, provide for the first time values for plant diversity in tropical African forests. As indicated above, significant discrepancies between ‘true’ floral diversity and estimated/counted number of species in a given region is a common problem in the tropics, as rare species will be hard to find or document [ 20 ].
Country-level diversity patterns
We provide, for the first time, basic plant biodiversity values for several countries across tropical Africa (Table 1 ). These values are based on the available data within RAINBIO, and thus should not be taken as definite. Nevertheless, they provide important insights into the plant diversity of these countries as well as levels of exploration. For some countries, these values are quite close to previous estimates. For example, Onana [ 69 ] recorded a total of 7850 vascular plant species for Cameroon, whereas we report 6883 species with an estimated total species richness of 8015. The higher recorded total species number of Onana [ 69 ] could be related to synonymy not taken into account during that study (Onana personal communication). Another example is for São Tomé and Príncipe [ 70 ], where a total of 803 native flowering plant species (excluding 301 introduced species) plus a single endemic gymnosperm species have been recorded. This value closely matches our estimates (Table 1 ), which could reflect the inclusion of the vast majority of São Tomé and Príncipe records from herbaria such as BRLU and LISC in our study [ 33 ].
Our study identifies several botanically ‘best-explored’ countries with Cameroon, Benin and Gabon in the top three (Table 1 ). For some of those countries (i.e. Benin, Gabon and Liberia) a large percentage of existing specimen data (over 90%) is available in RAINBIO and thus could reflect values close to reality. Even for these countries, the average specimen density is well below the threshold of 100 records/100 km 2 (19 for Benin, 35 for Gabon and 16 for Liberia; Table 1 ). In addition, the estimated discover rate (percentage of species yet to be documented based on our estimate of total species richness) varies between 14% and 19% for the top seven countries (Table 1 ). Thus, even for the top best-explored countries new species are expected to be described or have already been collected but not yet identified. Data from the International Plant Name Index (IPNI, www.ipni.org ) for the period 2000 to 2015 reveals that 8, 162 and 38 new species were described from Benin, Gabon and Liberia, respectively (0.3%, 2.7% and 1.5% of their estimated species richness, respectively). For Gabon, 4710 species were recorded in 2005 [ 52 ], meaning that no less than 526 (10%) new species or new species records have been added these past 11 years (5236 species recorded in RAINBIO). New species and even new genera are still regularly described for Gabon, even in well sampled areas [ 71 ] such as the Crystal Mountains National Park [ 67 , 72 – 77 ]. Interestingly, São Tomé and Príncipe show the highest concentration of records (375/100 km 2 , Table 1 ) yet is not amongst the best-explored countries in our study. This could be linked to the relatively high level of singletons when compared to the other most “well-explored” countries, with 233 species known from a single record out of 3598 total records (6.5%, Table 1 ). The Chao and Jost [ 45 ] estimator for the degree of sampling completeness adds DRC and Tanzania to the list of best-explored countries (Table 1 ). However, this seems counterintuitive when looking at the high number of singletons for those countries and the difference between the observed and estimated number of species (Table 1 ). This can be explained by the high number of specimens available for these two countries, which reduces the effect of the high number of singletons. Importantly, our results underline that even though these countries might appear well explored, there are still lots of efforts to provide in terms of botanical exploration and databasing. This is a common situation across the tropics, for example, in South America [ 20 ], where for even some of the best collected countries such as Ecuador, significant collecting efforts remain to be done in order to fully record its floral diversity [ 40 ]. To date, as far as we know, no tropical country (except Singapore) has yet claimed it should be considered as being botanically well-known.
In contrast, for some countries our data is far from complete as underlined by the low values of degree of completeness such as Angola, Somalia, Botswana, Republic of Congo, Guinea-Bissau and Zimbabwe. For some countries, the low value is explained by a lack of available data in digital format. For example, Figueiredo et al. [ 55 ] mentioned the occurrence of 6735 species of vascular plants in Angola, whereas RAINBIO records only 2262 species (Table 1 ). In the same way, the Republic of Congo is suggested to contain 4538 vascular plant species [ 78 , 79 ], whereas our dataset records just 2403 (Table 1 ). Despite this basic check-list, the Republic of Congo remains botanically one of the least known countries in tropical Africa [ 79 ]; a result that is also confirmed here (Table 1 ).

Botanical exploration of tropical Africa
A vital question in biodiversity inventories has been to evaluate the level of botanical exploration across the tropics. Campbell and Hammond [ 46 ] suggested that a minimal level of botanical exploration of a tropical region should be at least 100 specimens per 100 km 2 . Our data indicate that just 21 of the 0.5° SUs reach this threshold (Additional file 4 , 0.5° represents a surface of about 3000 km 2 around the equator, thus 3000 specimens per SU). In addition, we find that just 34 variable sized SUs (Fig. 7 ) are well sampled (defined as SUs with record density higher than 100 records/100 km 2 and C N > 0.5). This is much less than the number identified by Stropp et al. [ 25 ] based on the analysis of GBIF data (1002 SUs of 0.25° with more than 200 records). However, most of these SUs were located in South Africa, a region not included in our study. Moreover, this difference could be linked to the data (RAINBIO versus GBIF, although RAINBIO contains more data for tropical Africa) or the difference in our definitions of well-sampled SUs (larger SUs (0.5° versus 0.25°), different density thresholds and a different method to calculate the sampling coverage, C N ). In addition, we defined TSUs, which highlight the 25% SUs with the best data available when compared to the others (Fig. 7 ). TSUs are different from WSUσ in that they show SUs where most botanical exploration has occurred relative to others. Finally, the need for additional botanical exploration is also reflected by the very high number of species known from less than five records (Additional file 5 a) or occurring in a single SU (Additional file 5 b); this is a characteristic outcome of tropical biodiversity inventories [ 52 , 80 , 81 ]. For our dataset, this can partly be explained by the targeted scanning of type specimens within the Global Plant Initiative ([ 63 ], https://plants.jstor.org ), artificially increasing the number of species known from a single collection. Still, overall, our results confirm that tropical Africa remains severely under sampled [ 24 , 25 ], even when using the largest homogenised dataset ever complied for the region to date [ 33 ]. Similar results are reported for other tropical regions such as the Amazon basin [ 20 ] or countries such as Ecuador [ 40 ].
In a world of limited resources, an important question is how to identify priority sampling units [ 24 ], e.g. SUs where we could expect to significantly add to our knowledge of the African flora. This is not a trivial question, as potentially any SU across Africa deserves more in depth exploration. Several past studies have identified data-deficient areas using different methods. Küper et al. [ 24 ] qualified data-deficient areas as the difference or the ratio between predicted species richness (based on species distribution modelling of ca. 5000 species) and the documented species richness. Stropp et al. [ 25 ] identified areas of ‘acute data deficiency’ as areas that maximise the distance between well-sampled SUs based on the assumption that floristic similarity decreases with distance. Both these approaches are valid in their own right, underlining regions either containing potentially many uncollected species or regions that maximise the collection of new records for a whole region, respectively. Here, we used a concept similar to Stropp et al. [ 25 ] to identify a set of PSUs. Indeed, floristic similarity does decrease with distance; however, this relationship is not linear, as certain regions will experience higher floristic turnover rates than others (Fig. 6 ) [ 32 ]. We suggest that regions of higher floristic turnover should be more thoroughly explored as they will potentially uncover higher levels of botanical novelties. We defined a PSU as being an SU with an estimated high turnover rate relative to other neighbouring SUs associated with a low sampling coverage. High floristic turnover rates were identified (Fig. 6 ) mainly in montane areas (Cameroon Volcanic Line, Nimba Mountains, Eastern Arcs) and areas of vegetation transitions (e.g. coastal regions in West, Central and East Africa, Katanga in south-eastern DRC, Haut-Ogooué in Gabon). Not all coastal regions are identified as having a high turnover (e.g. Liberia), but the high turnover observed along the southern coast of Gabon is corroborated by Harris et al. [ 82 ]. Our suggested PSUs (Fig. 7 ) highlight very different areas than those suggested by Stropp et al. [ 25 ] and agree more with the priority areas suggested by Küper et al. [ 24 ]. Indeed, we identify PSUs, for example, in Guinea (Nimba Mountains), Cameroon (the Volcanic Line area above Mount Cameroon and the northern part of Korup National Park), Gabon (Haut-Ogooué), and the Eastern Arc Mountains and coastal forests in Tanzania. Areas of low estimated floristic turnover (Fig. 6 ) [ 32 ], such as the Congo Basin, do not contain any PSUs, in contrast to Stropp et al. [ 25 ]. Even though one could criticise our approach as it relies on the estimation of turnover rates, we applied a variable SU size approach which allows comparison of SUs based on similar amount of data [ 35 ]. All approaches to identify PSUs can be viewed as complementary but focusing on different aspects/priorities of biodiversity exploration.
Plant growth form dominance across tropical Africa
Data on growth form in the tropics is scarce, but some studies show that the contribution of herbaceous species to tropical forest diversity is between 18% and 44% [ 83 – 85 ]. Our data suggests that 43.8% of species are herbaceous across Tropical Africa. This also underlines that savanna and montane vegetation types are well represented in RAINBIO, despite the initial emphasis of our data towards forested regions. It has been shown that on global or continental scales plant growth form is linked with climatic variables [ 19 , 86 , 87 ]. Although no formal analyses are undertaken here, our data underline general patterns possibly linked to climate (Fig. 8 ). For example, herb species are dominant in the drier parts of Africa (Senegal, Burkina Faso, Malawi, Tanzania, Zimbabwe) whereas trees and lianas are dominant in countries with significant areas of tropical forests (e.g. Republic of Congo, Gabon, south of Cameroon). In addition, with most of their surface being at higher elevations, Ethiopia, Rwanda and Burundi harbour vegetation with much higher proportions of herbaceous species.
As indicated above, the herbaceous component (Fig. 8a ) gives a clear pattern related to the drier regions (Sahel, East Africa) where savanna prevails. Rwanda, Burundi, south-eastern DRC (Katanga), Zambia and Ethiopia, which are dominated by savanna and/or highland vegetation, also stand out as containing a high proportion of herbaceous species. Interestingly, a large New World dataset for geographical trait patterns did not reveal montane areas (such as the Andes or the Rocky Mountains) as exceptionally dominated by herb species [ 19 ]. The moderately high ratio of herb species locally observed on the coast of Ghana and the western tip of Gabon (Port-Gentil) (Fig. 8a ) relate to land surface occupied by coastal savanna where the rest of those grid cells are sea, showing that marginal SUs with only little land surface can easily result in seemingly odd results.
Shrub dominance (Fig. 8b ) is in general more equally distributed than herb, liana and tree dominance; shrubs can occur in nearly all vegetation types. The Eastern Africa Coastal Forest ecoregion is strikingly dominated by shrubs, known for its mosaic of forest, savannas and wetlands [ 88 – 90 ]. High shrub dominance is also observed in the Horn of Africa. The low proportion of shrub species observed in montane areas is not corroborated by a study on New World plants [ 19 ].
Liana dominance (Fig. 8c ) shows a very clear pattern, being high within the major rain forest block (the Guineo-Congolian region). In addition, lianas form a high proportion of growth forms in the semi-deciduous forests of south-eastern Cameroun and south-eastern Gabon, as well as in the highly fragmented forests of Upper Guinea [ 91 ], while the wettest forests in coastal Gabon, Cameroon and Liberia contain proportionally fewer liana species. This might be explained by the wettest forests having a closed canopy all year round, rendering the understory very dark, while in the drier forests, with some of the trees being semi-deciduous, the forest floor will have some periods with more light, enabling liana seedlings to gain the energy to climb to the canopy. In contrast to west and central Africa, the drier semi-deciduous East African coastal and Eastern Arc forests contain proportionally fewer liana species, which remains unexplained.
The tree component (Fig. 8d ) is highest within the rain forest regions where climate seasonality is lowest, which thus is in line with the findings of Engemann et al. [ 19 ] for the Neotropics. Gabon, having over 80% of its surface covered by possibly the most species-rich lowland rain forest of Africa [ 92 ], shows the highest proportion of tree species and the lowest proportion of herb species. Interestingly, similar high dominance in tree species is observed in northern Mozambique and south-eastern Tanzania. Recent collections from that area were made in the framework of the tree flora of Mozambique [ 93 ], creating a potential bias towards tree records. The Katanga region (south-eastern DRC) comes out as an area with an extremely poor tree species component.
Overall, the proportion of epiphytes (Fig. 8e ) is highest in montane areas such as Nimba Mountains (northern Liberia), Mount Cameroon and the Bamenda Highlands (Western Cameroon), Crystal Mountains (north-west Gabon), Monte Alen (Rio Muni), the Albertine Rift region and the Eastern Arc Mountains. Orchids, which constitute 73% of all epiphytes species recorded in RAINBIO, having better dispersal abilities than most other groups, would have been favoured by elevation and humidity gradients in mountainous areas [ 94 , 95 ]. The exceptionally high proportion of epiphytes observed on the island of Príncipe (up to 30% of species) is probably biased by the activities of several collectors with a strong interest in orchids [ 96 ]. Certainly, many more areas are actually under-collected for epiphytes, which are generally difficult to reach in the canopy.
Collecting history
Collecting intensity steadily increased through time in tropical Africa at least up to the end of the 20th century (Fig. 4a ). This trend, also underlined by other studies [ 25 ], could be linked to the growing notion of the importance of our environment and its diversity for economic reasons as well as the well-being of mankind and our planet [ 97 ]. We can clearly see the effect of both world wars (1914–1918, 1939–1945; Fig. 4a ), where collecting intensity dropped, but then quickly increased afterwards. The lower number of specimens recorded for the 21st century is worrying but could be partly explained by a lag in specimen digitization [ 25 ]. However, we do believe this observation to reflect a true trend. Thus, even though our data shows that our knowledge of tropical botanical diversity is far from sufficient (Fig. 7 ), we observe an alarming trend of diminishing exploration efforts across tropical Africa (Fig. 4a ). We also identified two decades (1941–1950 and 1961–1970) where significant botanical collecting took place in SUs never visited or very poorly collected before (reflected by the negative slope in Fig. 4b ). For 1961–1970 this is indeed explained by a record newly inventoried SUs (Additional file 7 ). Whereas for the 1941–1950 decade, this trend remains unexplained as comparatively fewer new SUs were inventoried.
When we study the geographical distribution of collecting efforts through time (time lapse) in tropical Africa (Fig. 5 ), we note that, until 1900, important collecting activity took place notably in Sierra Leone, the coasts of Cameroon and Gabon, in DRC along the Congo River and its main tributaries, and in East Africa in the Mombassa to Dar-es-Salaam coastal region. These corresponded to relatively easy accessible areas along the coast lines or along major river systems. Then, from 1900 to after the Second World War, the most significant botanical explorations took place in Liberia, Ghana, DRC and Mozambique. This was followed, between 1945 and 1975, by intensified collecting in virtually all regions, but notably in Ivory Coast, Cameroon, Gabon, the Albertine Rift region and Ethiopia. Finally, in the period after 1975, several regions were explored for the first time, such as northern Benin, while gaps were filled notably in Cameroon, Gabon, Kenya and Tanzania. It also is apparent that, in certain areas, there was no collecting at all, often due to periods of war (e.g. Liberia 1980–2000 and Angola 1975–2002), or the clear drop in collecting in DRC after independence (1960) when various Belgian institutions stopped their explorative work.
In general, we observe that collecting efforts per country are linked with both the political situation in a region as well as specific Flora programs. Each country has its own particular collecting history (Additional file 6 ). For Benin (Additional file 6 a), collecting activities greatly increased in the 1990s and 2000s related to a program to support their National Herbarium and publish a diagnostic Flora for the country [ 53 ]. In Cameroon (Additional file 6 b), collecting has been intense and fairly stable from the 1960s onward, initiated by the activities of René Letouzey (1918–1989) [ 98 ]. The graph for DRC (Additional file 6 c) shows the significant efforts by Belgian botanists during the colonial period 1890–1960 (despite the fact that the data on the majority of these collections is not yet available in electronic form), during which the production of the Flore d’Afrique Centrale started [ 56 , 99 ]. This is followed by a rapid decline in collecting activity after the country became independent in 1960. For Gabon (Additional file 6 d), a steep increase in collecting efforts coincides with the start of the intensive research program by the Plant Taxonomy department of Wageningen University (The Netherlands) in the early 1970s, also attracting other research groups in the course of time [ 52 ]. This provided the baseline data for the production of the Flore du Gabon [ 100 ]. For Ivory Coast (Additional file 6 e), the vast majority of collections were made after World War II, undertaken by local botanists (e.g. L. Aké Assi 1931–2014) but also British, French, Dutch and Swiss researchers [ 101 , 102 ]. Collecting intensity dropped considerably after the turmoil in the 2000s. Collecting in Liberia (Additional file 6 f) increased due to the efforts of Dutch collectors during the 1960s and 1970s. Then, after a period of virtual inactivity and civil war, renewed efforts took place, mainly by a single Dutch collector (C. Jongkind) within the context of various conservation projects and environmental impact studies [ 103 ]. Finally, Tanzania (Additional file 6 g) shows a steady increase in collecting effort after World War II, mainly by Tanzanian, American, British and Scandinavian botanists and also in the light of the production of the Flora of Tropical East Africa [ 61 ].
To conclude, we provide three main recommendations in order to improve our understanding of the distribution of plant diversity in tropical Africa.
Improve data exchange between datasets
The effort to combine several big datasets as well as more non-public specific ones has been a major undertaking [ 33 ]. It has proven to be possible, not overly complicated, though fairly time consuming. For tropical Africa, downloading available data from GBIF will exclude significant records [ 25 ] mainly from non-public databases from institutions that are either not participating in GBIF or have not yet shared all their data openly. We did not undertake detailed comparisons between GBIF and RAINBIO data as this was out of the scope of this article. Even though GBIF represents the most important source of open data for species distribution information, it has been shown that data quality and reliability may be low, especially in the tropics [ 104 , 105 ]. When compiling specimen data from different sources, one is faced with the ‘duplicate problem’, since plant specimens have several duplicates distributed to different herbaria some are prone to having different identifications and databasing quality, complicating the concatenation of data. Estimates suggest that just under 10% of all records in GBIF are potential duplicates [ 106 ]. Taking into account duplicates when compiling data from different institutes has yet to meet a good automatic solution and has rarely been done in large plant datasets. In the case of RAINBIO, different datasets were identified as ‘expert taxonomic benchmarks’, i.e. datasets verified by specialists for a given family, against which all duplicates were compared too and updated. We identified 11% of records with at least one duplicate. Similar approaches to deal with this problem have been undertaken, but at smaller scales (e.g. [ 105 ]). In order to maximise the usefulness of botanical biodiversity data more efforts should focus on resolving this limitation and finding appropriate automatic ways of taking duplicate information into account, especially from important online repositories such as GBIF that contain potentially high levels of duplicated information. Thus, besides past and ongoing major efforts to independently database herbarium specimens and upload them to GBIF, synthesising these datasets must remain a priority.
Improve data reliability
Separate, but related to the above point, the reliability of the data is important as it will directly influence the outcome of analyses [ 12 , 20 , 104 , 105 ]. It is well known and understood that natural history specimen datasets have several potential errors [ 20 , 54 , 107 ]. Two major sources of errors can influence the analyses of such data.
First, wrong species identification of specimens will be an important source of error in any dataset. We estimate that the identification error rate in an average herbarium collection can be as high as 10% or even up to 58% for specific groups [ 108 ], although in the latter figure specimens with a synonymous but otherwise correct name were also counted as errors. In a dataset of 4000 individual trees of the genus Inga , 7% of the identifications proved to be wrong [ 109 ]. By comparing an expert selection of identified duplicates, the identification accuracy in combined botanical datasets like ours can be improved. However, misidentifications are an inherent part of any biodiversity dataset and can never really be completely eliminated at least because of changing taxonomic concepts and the presence of incomplete specimens. The event of DNA barcoding [ 110 ] could provide added value to accurately identify specimens (from sterile vouchers to species complexes) although bulk barcoding of large collections remains expensive and time-consuming. We must also consider the level of our taxonomic knowledge for tropical Africa. Every year, hundreds of new plant species are being described (globally, on average well over 2000/year according to data provided by IPNI, www.ipni.org ), and new taxonomic borders for species, genera, etc. are drawn. Bebber et al. [ 9 ] showed that the majority of newly published species names were based on specimens collected long before. Thus, a fair number of new species are awaiting description within one of the world’s herbaria. In the future, our advancing knowledge will further refine and improve the quality analyses such as the ones presented above.
In addition, reliability of the data can be improved by a structured collaboration continuing to work with taxonomic experts. In the case of RAINBIO, large numbers of records were indeed checked and validated by the project taxonomic experts from numerous families or geographic regions. This greatly improved the accuracy and quality of RAINBIO. Misidentification is sometimes considered as ‘background noise’ in ‘big data’ datasets. Depending on the objectives of the study this can be misleading, leading to erroneous results (e.g. when estimating total species number per country/region; conservation assessments). We advocate a ‘compile and check’ rather than a ‘compile only’ approach. Directly involving expert plant taxonomists in such large projects will greatly improve our databases and the subsequent biodiversity analysis. Second, specimens may be incorrectly georeferenced, which in turn affects biodiversity analyses [ 105 ]. However, recent tools or software packages are now available to automatically improve or correct wrong georeferencing in big datasets [ 111 , 112 ]. Checking georeferencing precision using such methods should systematically be used in order to improve the precision of these records.
Continue the botanical exploration of tropical Africa and digitization of the related specimens
No country in tropical Africa can be regarded as botanically well explored. Larger areas with no or limited data are still plentiful. Given the observed declining trends in collecting efforts (Fig. 4a ) [ 25 ], we appeal not only for additional collecting efforts, but also for increased digitization of tropical African plant collections. This will depend on the availability of major funding, mostly at governmental level. In tropical Africa, the major gaps in availability of digital specimen data are in Nigeria, the Central African Republic, South Soudan, the Republic of the Congo and Angola. We believe these to be true gaps, and therefore regions for which comparatively low numbers of specimens have been collected to date. The gap in the availability of data from the DRC should at least partly be overcome soon due to major digitization efforts at BR [ 56 ]. Here, we highlight several regions (namely PSUs) we believe would provide a significant amount of new data to our understanding of the tropical African flora in the same spirit as other studies [ 24 , 25 ].
The RAINBIO database provides the underlining data to assist in advancing phytogeographical, systematic and ecological research, and enables a wiser sustainable usage and conservation of Africa’s unique tropical plant diversity. Only when provided with sound and high quality information on biodiversity distribution, can policymakers take informed decisions about how to manage this fragile resource effectively [ 8 ]. Strangely enough, its strength is also in that it shows clear weaknesses in the amount of botanical data available for tropical Africa. To most efficiently fill the gaps and speed up the work, we should investigate how we might benefit from a much larger group of data collectors. The development of citizen science [ 113 ], i.e. public involvement in science, has proven its usefulness in a range of biodiversity-related projects (for two recent examples, see [ 114 , 115 ]). For specific plant groups, field observations made by citizen scientists backed-up with photographs and verified by specialists can add valuable distributional data especially in data-deficient areas (e.g. [ 115 ]). These ideas contrast strikingly with the observation of decreasing collecting efforts in the past two decades. In tropical Africa, the paucity of reliable data on a group of organisms as important as vascular plants cries out loud for a renewed and probably unprecedentedly massive botanical exploration of the region.
Gaston KJ. Global patterns in biodiversity. Nature. 2000;405:220–7.
Article CAS PubMed Google Scholar
Klopper RR, Gautier L, Chatelain C, Smith GF, Spichiger R. Floristics of the angiosperm flora of sub-Saharan Africa: an analysis of the African Plant Checklist and Database. Taxon. 2007;56:201–8.
Google Scholar
Linder HP. Plant diversity and endemism in sub-Saharan tropical Africa. J Biogeogr. 2001;28:169–82.
Article Google Scholar
Linder HP. The evolution of African plant diversity. Front Genet. 2014;2:38.
Watson JE, Shanahan DF, Di Marco M, Allan J, Laurance WF, Sanderson EW, et al. Catastrophic declines in wilderness areas undermine global environment targets. Curr Biol. 2016;26(21):2929–34.
McClean CJ, Lovett JC, Küper W, Hannah L, Sommer JH, Barthlott W, et al. African plant diversity and climate change. Ann Mo Bot Gard. 2005;92:139–52.
Wen J, Ickert-Bond SM, Appelhans MS, Dorr LJ, Funk VA. Collections-based systematics: opportunities and outlook for 2050. J Syst Evol. 2015;53:477–88.
Perrings C, Lovett J. Policies for biodiversity conservation: the case of sub-Saharan Africa. Int Aff. 1999;75:281–305.
Bebber DP, Carine MA, Wood JR, Wortley AH, Harris DJ, Prance GT, et al. Herbaria are a major frontier for species discovery. Proc Natl Acad Sci. 2010;107:22169–71.
Article CAS PubMed PubMed Central Google Scholar
Greve M, Lykke AM, Fagg CW, Gereau RE, Lewis GP, Marchant R, et al. Realising the potential of herbarium records for conservation biology. South Afr J Bot. 2016;105:317–23.
Index Herbariorum. A global directory of public herbaria and associated staff. New York Botanical Garden’s Virtual Herbarium. 2012. http://sweetgum.nybg.org/science/ih/ . Accessed 21 May 2015.
Graham CH, Ferrier S, Huettman F, Moritz C, Peterson AT. New developments in museum-based informatics and applications in biodiversity analysis. Trends Ecol Evol. 2004;19:497–503.
Article PubMed Google Scholar
Blagoderov V, Smith V. Bringing collections out of the dark. ZooKeys. 2012;209:1–6.
Lavoie C. Biological collections in an ever changing world: Herbaria as tools for biogeographical and environmental studies. Perspect Plant Ecol Evol Syst. 2013;15:68–76.
Kress WJ. Valuing collections. Science. 2014;346:1310.
Heerlien M, Van Leusen J, Schnörr S, De Jong-Kole S, Raes N, Van Hulsen K. The natural history production line: an industrial approach to the digitization of scientific collections. J Comput Cult Herit JOCCH. 2015;8:3.
Seregin AP. Making the Russian flora visible: fast digitisation of the Moscow University Herbarium (MW) in 2015. Taxon. 2016;65:205–7.
ter Steege H, Pitman NC, Sabatier D, Baraloto C, Salomão RP, Guevara JE, et al. Hyperdominance in the Amazonian tree flora. Science. 2013;342:1243092.
Article PubMed CAS Google Scholar
Engemann K, Sandel B, Enquist BJ, Jørgensen PM, Kraft N, Marcuse-Kubitza A, et al. Patterns and drivers of plant functional group dominance across the Western Hemisphere: a macroecological re-assessment based on a massive botanical dataset. Bot J Linn Soc. 2016;180:141–60.
ter Steege H, Vaessen RW, Cárdenas-López D, Sabatier D, Antonelli A, de Oliveira SM, et al. The discovery of the Amazonian tree flora with an updated checklist of all known tree taxa. Sci Rep. 2016;6:29549.
Article PubMed PubMed Central CAS Google Scholar
Collen B, Ram M, Zamin T, McRae L. The tropical biodiversity data gap: addressing disparity in global monitoring. Trop Conserv Sci. 2008;1:75–88.
Antonelli A, Zizka A, Silvestro D, Scharn R, Cascales-Miñana B, Bacon CD. An engine for global plant diversity: highest evolutionary turnover and emigration in the American tropics. Front Genet. 2014;6:130.
Feeley K. Are we filling the data void? An assessment of the amount and extent of plant collection records and census data available for tropical South America. PLoS One. 2015;10:e0125629.
Küper W, Sommer JH, Lovett JC, Barthlott W. Deficiency in African plant distribution data – missing pieces of the puzzle. Bot J Linn Soc. 2006;150:355–68.
Stropp J, Ladle RJ, Malhado M, Ana C, Hortal J, Gaffuri J, et al. Mapping ignorance: 300 years of collecting flowering plants in Africa. Glob Ecol Biogeogr. 2016;25:1085–96.
White F. The vegetation of Africa, a descriptive memoir to accompany the UNESCO/AETFAT/UNSO vegetation map of Africa. Paris: UNESCO; 1983.
Slik JWF, Arroyo-Rodríguez V, Aiba S-I, Alvarez-Loayza P, Alves LF, Ashton P, et al. An estimate of the number of tropical tree species. Proc Natl Acad Sci U S A. 2015;112:7472–7.
Couvreur TLP. Odd man out: why are there fewer plant species in African rain forests? Plant Syst Evol. 2015;301:1299–313.
Richards PW. Africa, the “Odd man out.”. In: Meggers BJ, Ayensu ES, Duckworth WD, editors. Tropical Forest Ecosystems in Africa and South America: A Comparative Review. Washington: Smithsonian Institution Press; 1973.
Küper W, Sommer JH, Lovett JC, Mutke J, Linder HP, Beentje HJ, et al. Africa’s hotspots of biodiversity redefined. Ann Mo Bot Gard. 2004;91:525–35.
Linder HP, Lovett J, Mutke JM, Barthlott W, Jürgens N, Rebelo T, et al. A numerical re-evaluation of the sub-Saharan phytochoria of mainland Africa. Biol Skr. 2005;55:229–52.
Linder HP, de Klerk HM, Born J, Burgess ND, Fjeldså J, Rahbek C. The partitioning of Africa: statistically defined biogeographical regions in sub-Saharan Africa. J Biogeogr. 2012;39:1189–372.
Dauby G, Zaiss R, Blach-Overgaard A, Catarino L, Damen T, Deblauwe V, et al. RAINBIO: a mega-database of tropical African vascular plants distributions. PhytoKeys. 2016;74:1–18.
Olson DM, Dinerstein E, Wikramanayake ED, Burgess ND, Powell GVN, Underwood EC, et al. Terrestrial ecoregions of the world: a new map of life on Earth. Bioscience. 2001;51:933–8.
Edler D, Guedes T, Zizka A, Rosvall M, Antonelli A. Infomap Bioregions: Interactive mapping of biogeographical regions from species distributions. Syst Biol. 2016; pii: syw087. Ahead of print.
Chiarucci A, Enright NJ, Perry GLW, Miller BP, Lamont BB. Performance of nonparametric species richness estimators in a high diversity plant community. Divers Distrib. 2003;9:283–95.
Gotelli NJ, Chao A. Measuring and estimating species richness, species diversity, and biotic similarity from sampling data. Encycl Biodivers. 2013;5:195–211.
Dauby G, Hardy OJ. Sampled-based estimation of diversity sensu stricto by transforming Hurlbert diversities into effective number of species. Ecography. 2012;35:661–72.
Droissart V, Hardy OJ, Sonké B, Dahdouh-Guebas F, Stévart T. Subsampling herbarium collections to assess geographic diversity gradients: a case study with endemic Orchidaceae and Rubiaceae in Cameroon. Biotropica. 2012;44:44–52.
Engemann K, Enquist BJ, Sandel B, Boyle B, Jørgensen PM, Morueta-Holme N, et al. Limited sampling hampers “big data” estimation of species richness in a tropical biodiversity hotspot. Ecol Evol. 2015;5:807–20.
Article PubMed PubMed Central Google Scholar
Nielsen R, Tarpy DR, Reeve HK. Estimating effective paternity number in social insects and the effective number of alleles in a population. Mol Ecol. 2003;12:3157–64.
Jost L. Entropy and diversity. Oikos. 2006;113:363–75.
Baselga A. Partitioning the turnover and nestedness components of beta diversity. Glob Ecol Biogeogr. 2010;19:134–43.
Soininen J, McDonald R, Hillebrand H. The distance decay of similarity in ecological communities. Ecography. 2007;30:3–12.
Chao A, Jost L. Coverage-based rarefaction and extrapolation: standardizing samples by completeness rather than size. Ecology. 2012;93:2533–47.
Campbell DG, Hammond HD. Floristic inventory of tropical countries: the status of plant systematics, collections, and vegetation, plus recommendations for the future. New York: New York Botanical Garden; 1989.
Dixon P. VEGAN, a package of R functions for community ecology. J Veg Sci. 2003;14:927–30.
Marcon E, Hérault B. entropart: an R package to measure and partition diversity. J Stat Softw. 2015;67:1–26.
Baselga A, Orme CDL. betapart: an R package for the study of beta diversity. Methods Ecol Evol. 2012;3:808–12.
Mayaux P, Bartholomé E, Fritz S, Belward A. A new land-cover map of Africa for the year 2000. J Biogeogr. 2004;31:861–77.
Marshall CA, Wieringa JJ, Hawthorne WD. Bioquality Hotspots in the Tropical African Flora. Curr Biol. 2016;26(23):3214–9.
Sosef MSM, Wieringa JJ, Jongkind CCH, Achoundong G, Azizet Issembé Y, Bedigian D, et al. Check-list des plantes vasculaires du Gabon/Checklist of Gabonese vascular plants. Meise: Jardin Botanique National de Belgique; 2006.
Akoègninou A, van der Burg WJ, van der Maesen LJG. Flore analytique du Bénin. Leiden: Backhuys Publishers; 2006.
ter Steege H, Haripersaud PP, Bánki OS, Schieving F. A model of botanical collectors’ behavior in the field: Never the same species twice. Am J Bot. 2011;98:31–7.
Figueiredo E, Smith GF, César J. The flora of Angola: first record of diversity and endemism. Taxon. 2009;58:233–6.
Sosef MS. Producing the Flore D’Afrique Centrale, past, present and future. Taxon. 2016;65:937–9.
Govaerts R. How many species of seed plants are there? Taxon. 2001;50:1085–90.
Cable S, Cheek M. The plants of Mt Cameroon: a conservation checklist. Kew London: Kew Publishing. Royal Botanic Gardens; 1998.
Cheek M, Onana J-M, Pollard BJ. The plants of Mount Oku and the Ijim Ridge. Cameroon: Kew Publishing, Royal Botanic Gardens Kew; 2000.
Cheek M, Pollard BJ, Darbyshire I, Onana JM, Wild C. The plants of Kupe, Mwanenguba and the Bakossi Mountains, Cameroon. A conservation checklist. The board of trustees of the Royal Botanical Gardens, Kew. Trowbridge: Cromwell Press; 2004.
Beentje H. Science comes from collaboration and communication: the Flora of Tropical East Africa as an example. Webbia. 2015;70:171–9.
Masens Da-Musa Yung B. Etude phytosociologique de la région de Kikwit (Bandundu, Rép. Dém. du Congo). Bruxelles: Université Libre de Bruxelles; 1997.
Smith GF, Roux JPK, Raven P, Figueiredo E. African herbaria support transformation on the continent. Ann Mo Bot Gard. 2011;98:272–6.
Adam JG. Flore Descriptive des Monts Nimba (Côte d’Ivoire, Guinée, Libéria). Paris: Editions du Centre National de la Recherche Scientifique; 1983.
White F. La végétation de l’Afrique: Contribution à l’étude de la flore et de la végétation de l’Afrique occidentale. 1986.
Kenfack D, Thomas DW, Chuyong G, Condit R. Rarity and abundance in a diverse African forest. Biodivers Conserv. 2007;16:2045–74.
Couvreur TLP, Sonke B, Niangadouma R, Sauquet H. Sirdavidia, an extraordinary new genus of Annonaceae from Gabon. PhytoKeys. 2015;46:1–19.
Parmentier I, Malhi Y, Senterre B, Whittaker RJ, Alonso A, Balinga MPB, et al. The odd man out? Might climate explain the lower tree alpha-diversity of African rain forests relative to Amazonian rain forests? J Ecol. 2007;95:1058–71.
Onana J-M. The vascular plants of Cameroon: A taxonomic checklist with IUCN assessments. Yaounde: National Herbarium of Cameroon; 2011.
Figueiredo E, Paiva J, Stévart T, Oliveira F, Smith GF. Annotated catalogue of the flowering plants of São Tomé and Príncipe. Bothalia. 2011;41:41–82.
Wieringa JJ, Sosef MSM. The applicability of relative floristic resemblance to evaluate the conservation value of protected areas. Plant Ecol Evol. 2011;144:242–8.
Lachenaud O, Breteler FJ. Novitates Gabonenses 76. A new Maesobotrya (Euphorbiaceae) from Crystal Mountains (Gabon and Equatorial Guinea). Adansonia. 2011;33:215–9.
Bissiengou P, Chatrou LW, Wieringa JJ, Sosef MSM. Taxonomic novelties in the genus Campylospermum (Ochnaceae). Blumea. 2013;58:1–7.
Wieringa JJ, Mackinder BA, Van Proosdij AS. Gabonius gen. nov. (Leguminosae, Caesalpinioideae, Detarieae), a distant cousin of Hymenostegia endemic to Gabon. Phytotaxa. 2013;142:15–24.
Stévart T, Biteau JP, Cawoy V, Droissart V. Taxonomy of Atlantic Central African orchids 3. A new species of Bulbophyllum Thouars (Orchidaceae) from the Monts de Cristal, Gabon. Phytotaxa. 2014;177:9.
Couvreur TLP, Niangadouma R. New species of Uvariopsis (Annonaceae) and Laccosperma (Arecaceae/Palmae) from Monts de Cristal. Gabon PhytoKeys. 2016;68:1.
Hoekstra PH, Wieringa JJ, Chatrou LW. A nonet of novel species of Monanthotaxis (Annonaceae) from around Africa. PhytoKeys. 2016;69:71.
Sita P, Moutsamboté JM. Catalogue des plantes vasculaires du Congo. Brazzaville: ORSTOM, Centre d’Etudes sur les Ressources Vegetales; 1988.
Lachenaud O. La flore des plantes vasculaires de la République du Congo: nouvelles données. Syst Geogr Plants. 2009;79:199–214.
Raes N, Roos MC, Slik JWF, van Loon EE, ter Steege H. Botanical richness and endemicity patterns of Borneo derived from species distribution models. Ecography. 2009;32:180–92.
Hubbell SP. Estimating the global number of tropical tree species, and Fisher’s paradox. Proc Natl Acad Sci U S A. 2015;112:7343–4.
Harris DJ, Armstrong KE, Walters GM, Wilks C, Mouandza Mbembo J-C, Niangadouma R, et al. Phytogeographical analysis and checklist of the vascular plants of Loango National Park. Gabon Plant Ecol Evol. 2012;145:242–57.
Gentry AH, Dodson C. Contribution of nontrees to species richness of a tropical rain forest. Biotropica. 1987;19(2):149–56.
Reitsma JM. Vegetation forestiere du Gabon (Forest vegetation of Gabon). Ede: Tropenbos Foundation; 1988.
Poulsen AD. The herbaceous ground flora of the Batu Apoi forest Reserve. Brunei Darussalam Trop Rainfor Res Issues. 1996;74:43–57.
Harrison SP, Prentice IC, Barboni D, Kohfeld KE, Ni J, Sutra J-P. Ecophysiological and bioclimatic foundations for a global plant functional classification. J Veg Sci. 2010;21:300–17.
Esquivel-Muelbert A, Baker TR, Dexter KG, Lewis SL, Steege H, Lopez-Gonzalez G, et al. Seasonal drought limits tree species across the Neotropics. Ecography. Ahead of print. doi: 10.1111/ecog.01904 .
Clarke GP. A new regional centre of endemism in Africa. In: Huxley CR, Lock M, Cutler DE (editors). Chorology, Taxonomy and Ecology of the Floras of Africa and Madagascar. 1998; pp. 53–65.
Burgess ND, Clarke GP. Coastal Forests of Eastern Africa. Gland: IUCN; 2000.
Timberlake J, Goyder D, Crawford F, Burrows J, Clarke GP, Luke Q, et al. Coastal dry forests in northern Mozambique. Plant Ecol Evol. 2011;144:126–37.
Bongers F, Parren MP, Traoré D. Forest climbing plants of West Africa: diversity, ecology and management. Cambridge: CAB International; 2005.
Sayer JA, Harcourt CS, Collins NM. The conservation atlas of tropical forests: Africa. London: Springer; 1992.
Book Google Scholar
Burrows J, Schmidt E, Burrows S, Lötter M. Trees and Shrubs of Mozambique. Sponsorsth ed. Cape Town: Print Matters Heritage; 2017.
Küper W, Kreft H, Nieder J, Köster N, Barthlott W. Large-scale diversity patterns of vascular epiphytes in Neotropical montane rain forests. J Biogeogr. 2004;31:1477–87.
Zhang S-B, Chen W-Y, Huang J-L, Bi Y-F, Yang X-F. Orchid species richness along elevational and environmental gradients in Yunnan, China. PLoS One. 2015;10:e0142621.
Stévart T, Geerinck D, Lejoly J. Liste des Orchidaceae de Sao-Tomé et Principe. Acta Bot Gallica. 2000;147:165–72.
Patz J, Corvalan C, Hortwitz P, Campbell-Lendrum D, Watts N, Maiero M, et al. Our planet, our health, our future. Human health and the Rio conventions: biological diversity, climate change and desertification. Geneva: World Health Organization; 2012.
Letouzey R. Etude phytogéographique du Cameroun. Theses. Toulouse: University of Toulouse; 1968.
Robyns W. Une flore du Congo Belge et du Ruanda-Urundi. Bull Jard Bot Etat Bruxelles. 1949;19:231–5.
Sosef MSM, Florence J, Wieringa JJ. Flore du Gabon, progress and inventorie. In: Jeannoda VH, Razafimandimbison SG, Block P, editors. XIXth AETFAT Congr. Madag. 25–30 April 2010 – Abstr. Meise: National Botanic Garden of Belgium; 2010. p. 465.
Aké AL. Flore de la Côte d’Ivoire: catalogue systématique, biogéographie et écologie, 1. Boissiera. 2001;57:1–396.
Aké AL. Flore de la Cote-d’Ivoire: catalogue systematique, biogeographie et ecologie. 2. Boissiera. 2002;58:1–401.
Jongkind CCH, Suter J. List of Liberian vascular plants. Advances in Botanical Knowledge of Liberia Supported by the Liberia Forest Re-assessment Project: 7-9 & appendix 1. Cambridge: Fauna Flora Int; 2004.
Yesson C, Brewer PW, Sutton T, Caithness N, Pahwa JS, Burgess M, et al. How global is the global biodiversity information facility? PLoS One. 2007;2:e1124.
Maldonado C, Molina CI, Zizka A, Persson C, Taylor CM, Albán J, et al. Estimating species diversity and distribution in the era of Big Data: to what extent can we trust public databases? Glob Ecol Biogeogr. 2015;24:973–84.
Samy G, Chavan V, Ariño AH, Otegui J, Hobern D, Sood R, et al. Content assessment of the primary biodiversity data published through GBIF network: status, challenges and potentials. Biodivers Inform. 2013;8:94–172.
Haripersaud PP. Collecting biodiversity. Utrecht: Utrecht University Repository; 2009.
Goodwin ZA, Harris DJ, Filer D, Wood JR, Scotland RW. Widespread mistaken identity in tropical plant collections. Curr Biol. 2015;25:R1066–7.
Dexter KG, Pennington TD, Cunningham CW. Using DNA to assess errors in tropical tree identifications: How often are ecologists wrong and when does it matter? Ecol Monogr. 2010;80:267–86.
Group CPW, Hollingsworth PM, Forrest LL, Spouge JL, Hajibabaei M, Ratnasingham S, et al. A DNA barcode for land plants. Proc Natl Acad Sci U S A. 2009;106:12794–7.
Töpel M, Zizka A, Calió MF, Scharn R, Silvestro D, Antonelli A. SpeciesGeoCoder: Fast Categorization of Species Occurrences for Analyses of Biodiversity, Biogeography, Ecology, and Evolution. Syst Biol. Ahead of print. doi: https://doi.org/10.1093/sysbio/syw064 .
Guo Q, Liu Y. ModEco: an integrated software package for ecological niche modeling. Ecography. 2010;33:637–42.
Silvertown J. A new dawn for citizen science. Trends Ecol Evol. 2009;24:467–71.
Amano T, Lamming JDL, Sutherland WJ. Spatial gaps in global biodiversity information and the role of citizen science. Bioscience. 2016;66:393–400.
Gardiner LM, Bachman SP. The role of citizen science in a global assessment of extinction risk in palms (Arecaceae). Bot J Linn Soc. 2016;182:543–50.
Mayaux P, Pekel J-F, Desclée B, Donnay F, Lupi A, Achard F, et al. State and evolution of the African rainforests between 1990 and 2010. Philos Trans R Soc B Biol Sci. 2013;368:20120300.
Download references
Acknowledgments
We thank Alison Specht and three anonymous reviewers for critically reading a previous version of this manuscript.
This work was co funded by the French Foundation for Research on Biodiversity (FRB) and the Provence-Alpes-Côte d’Azur region (PACA) region through the Centre for Synthesis and Analysis of Biodiversity data (CESAB) programme, as part of the RAINBIO research project.
Availability of data and materials
The RAINBIO dataset used for this study is freely available for download at http://rainbio.cesab.org where a “terms of use” section is available.
Authors’ contributions
GD, MS and TC conceived the study, all authors provided or entered data, GD undertook the analyses, all authors contributed to writing the paper. All authors read and approved the final manuscript.
Competing interests
The authors declare that they have no competing interests.
Consent for publication
Not applicable.
Ethics approval and consent to participate
Author information, authors and affiliations.
Botanic Garden Meise, Nieuwelaan 38, BE-1860, Meise, Belgium
Marc S. M. Sosef, Steven Dessein, Henry Engledow, Steven Janssens, Tariq Stévart & Piet Stoffelen
DIADE, Université Montpellier, IRD, Montpellier, France
Gilles Dauby, Vincent Deblauwe & Thomas L. P. Couvreur
Section for Ecoinformatics & Biodiversity, Department of Bioscience, Aarhus University, Ny Munkegade 114, DK-8000, Aarhus C, Denmark
Anne Blach-Overgaard & Jens-Christian Svenning
Royal Botanic Gardens, Kew, Richmond, Surrey, TW9 3AE, UK
Xander van der Burgt, John Dransfield & Barbara A. Mackinder
Centre for Ecology, Evolution and Environmental Changes (CE3C), Faculty of Sciences, University of Lisbon, Campo Grande, 1749-016, Lisbon, Portugal
Luís Catarino & Maria Cristina Duarte
Wageningen University, Biosystematics Group, Droevendaalsesteeg 1, 6708 PB, Wageningen, The Netherlands
Theo Damen & Jan J. Wieringa
Herbarium et Bibliothèque de Botanique Africaine, Université Libre de Bruxelles, Boulevard du Triomphe, B-1050, Bruxelles, Belgium
Vincent Deblauwe, Vincent Droissart, Geoffrey Fadeur, Pierre Meerts & Tariq Stévart
Laboratoire de Botanique systématique et d’Écologie, Département des Sciences Biologiques, École Normale Supérieure, Université de Yaoundé I, Yaoundé, Cameroon
Vincent Deblauwe, Bonaventure Sonké & Thomas L. P. Couvreur
Missouri Botanical Garden, Africa & Madagascar Department, P.O. Box 299, St. Louis, Missouri, 63166-0299, USA
Vincent Droissart, Roy E. Gereau & Tariq Stévart
CIBIO/InBio, Centro de Investigação em Biodiversidade e Recursos Genéticos, Universidade do Porto, Campus Agrário de Vairão, Vairão, Portugal
Rui Figueira
CEABN/InBio, Centro de Ecologia Aplicada “Professor Baeta Neves”, Instituto Superior de Agronomia, Universidade de Lisboa, Tapada da Ajuda, 1349-017, Lisboa, Portugal
Laboratoire d’Évolution biologique et Écologie, Faculté des Sciences, Université Libre de Bruxelles, Brussels, Belgium
Gilles Dauby & Olivier J. Hardy
Royal Botanic Garden Edinburgh, 20A Inverleith Row, Edinburgh, UK
David J. Harris & Barbara A. Mackinder
Naturalis Biodiversity Center, Darwinweg 2, 2333 CR, Leiden, The Netherlands
Janneke de Heij, Yannick Klomberg, Jeike L. van de Poel, Jan J. Wieringa & Thomas L. P. Couvreur
Picturae, De Droogmakerij 12, 1851LX, Heiloo, The Netherlands
Janneke de Heij
Department of Ecology, Faculty of Science, Charles University, Vinicna 7, 128 44, Prague 2, Czech Republic
Yannick Klomberg
Institut für Geobotanik und Botanischer Garten, Im Neuwerk 21, University Halle-Wittenberg, 06108, Halle (Saale), Germany
Alexandra C. Ley
Laboratoire d’Ecologie végétale et Biogéochimie, Université Libre de Bruxelles, Boulevard du Triomphe, B-1050, Bruxelles, Belgium
Pierre Meerts
Laboratoire des Sciences du Climat et de l’Environnement, LSCE/IPSL, CEA-CNRS-UVSQ, Université Paris-Saclay, F-91191, Gif-sur-Yvette, France
Pierre Sepulchre
AMAP, CNRS, INRA, IRD, Université Montpellier, Montpellier, France
Vincent Droissart & Rainer Zaiss
CESAB/FRB, Domaine du Petit Arbois, Av. Louis Philibert, Aix-en-Provence, 13100, France
Gilles Dauby
Center for Tropical Research, Institute of the Environment and Sustainability, University of California, Los Angeles, Box 951496, Los Angeles, CA, 90095, USA
Vincent Deblauwe
International Institute of Tropical Agriculture, BP 2008 (Messa), Yaounde, Cameroon
You can also search for this author in PubMed Google Scholar
Corresponding authors
Correspondence to Marc S. M. Sosef or Thomas L. P. Couvreur .
Additional information
Marc S.M. Sosef and Gilles Dauby are co-first authors.
Additional files
Additional file 1:.
Two examples of turnover rate calculation. The figure shows two examples to estimate the turnover rate used herein. For μ = 1° (meso-scale) and μ = 2° (large-scale). The focal sampling unit (SU) is highlighted in red. A circle of distance μ is drawn around the red SU. All SUs included in the circle and with record number above 100 are then selected (in grey). In white, non-selected SUs. The geographical distance between all selected SUs is then calculated based on the centroid of the convex hull around the records for each SU (not shown). The pairwise floristic similarity between all selected SUs is then computed as 1–β sim . The linear relation between the geographical distance and the floristic similarity between all comparisons is computed (line in red). The distance (in kilometres) that halves the initial floristic similarity is calculated (vertical line) and used to define the turnover rate for each SU. (PNG 917 kb)
Additional file 2:
An R script used to compute Nielsen estimator from a matrix of samples-species. (R 808 bytes)
Additional file 3:
The distribution of tropical African forests. Map showing the 0.1° sampling units selected as forest for our study based on the map of Mayaux et al. [ 116 ]. Light-green: west African forests; deep-green: central African forests; medium-green: east African forests. (PNG 136 kb)
Additional file 4:
The number of 0.5° sampling units (y-axis) containing a specified number of observations (x-axis). (PNG 12 kb)
Additional file 5:
Geographic distribution of records across tropical Africa. (a) The number of species known from a particular number of records. (b) Number of species known from a particular number of 0.5° sampling units. (c) Number of species in function of their calculated range size (convex hull). (PNG 1422 kb)
Additional file 6:
Collecting history per country. Bar plots for a selection of tropical African countries showing the number of records collected in each period of time (5-year intervals). Plots based on herbarium records. (PNG 2657 kb)
Additional file 7:
Temporal exploration of tropical Africa per decade. The figure shows the newly explored 0.5° sampling units per decade. (PNG 21 kb)
Additional file 8:
A GIF animated figure of Fig. 5 by 10-year time slices. Also available at http://rainbio.cesab.org/ . (GIF 2259 kb)
Rights and permissions
Open Access This article is distributed under the terms of the Creative Commons Attribution 4.0 International License ( http://creativecommons.org/licenses/by/4.0/ ), which permits unrestricted use, distribution, and reproduction in any medium, provided you give appropriate credit to the original author(s) and the source, provide a link to the Creative Commons license, and indicate if changes were made. The Creative Commons Public Domain Dedication waiver ( http://creativecommons.org/publicdomain/zero/1.0/ ) applies to the data made available in this article, unless otherwise stated.
Reprints and permissions
About this article
Cite this article.
Sosef, M.S.M., Dauby, G., Blach-Overgaard, A. et al. Exploring the floristic diversity of tropical Africa. BMC Biol 15 , 15 (2017). https://doi.org/10.1186/s12915-017-0356-8
Download citation
Received : 03 November 2016
Accepted : 25 January 2017
Published : 07 March 2017
DOI : https://doi.org/10.1186/s12915-017-0356-8
Share this article
Anyone you share the following link with will be able to read this content:
Sorry, a shareable link is not currently available for this article.
Provided by the Springer Nature SharedIt content-sharing initiative
- Herbarium specimens
- Digitization
- Tropical forests
- Botanical exploration
- Plant growth form
- Species richness
- Floristic patterns
BMC Biology
ISSN: 1741-7007
- General enquiries: [email protected]
Thank you for visiting nature.com. You are using a browser version with limited support for CSS. To obtain the best experience, we recommend you use a more up to date browser (or turn off compatibility mode in Internet Explorer). In the meantime, to ensure continued support, we are displaying the site without styles and JavaScript.
- View all journals
- Explore content
- About the journal
- Publish with us
- Sign up for alerts
- Open access
- Published: 01 July 2019
Impact of climate change and urban development on the flora of a southern European city: analysis of biodiversity change over a 120-year period
- Mirko Salinitro 1 ,
- Alessandro Alessandrini 2 ,
- Alessandro Zappi ORCID: orcid.org/0000-0002-0029-4403 3 &
- Annalisa Tassoni ORCID: orcid.org/0000-0002-3541-4030 1
Scientific Reports volume 9 , Article number: 9464 ( 2019 ) Cite this article
5350 Accesses
20 Citations
1 Altmetric
Metrics details
- Climate-change ecology
- Plant ecology
Ecological studies in cities are not only aimed at investigating floristic diversity, but also represent informative test cases for understanding ecological system dynamics and responses to urban and climate changes since cities represent microcosms of environmental changes happening globally. The city of Bologna was selected as a case study since two specific and complete studies have been carried out in a 120-years timespan, one in 1894 and one in 2018. Since 1894, a large increase occurred in the number of taxa (families from 41 to 101, species from 176 to 477) and alien species (from 22 to 144), with a 65% total species turnover. The comparison of species life forms pointed out a noticeable recent expansion of phanerophytes and geophytes at the expense of therophytes and hemicryptophytes. The correlation between urbanistic features and plant richness indicated that the main factor affecting plant richness is the presence of green spaces (parks, tree lines, flowerbeds, etc.). Analysis of variation in Ellenberg’s indicator values over the last 120 years evidenced a shift toward shade-tolerant species, mainly connected to the increased presence of parks and trees within the city. Climate change and the presence of artificially irrigated areas within the city has led to an increase in both hygrophilous and drought-resistant species. In particular, the temperature index showed a significantly higher amount of macrothermal species in accordance with a warmer climate and the urban heat island effect.
Similar content being viewed by others
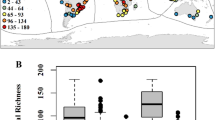
The landscape ecological view of vertebrate species richness in urban areas across biogeographic realms
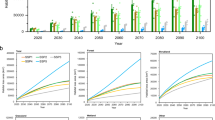
Global impacts of future urban expansion on terrestrial vertebrate diversity

Spatial patterns of vascular plant species richness in Poland - a data set
Introduction.
The world’s population is experiencing a dramatic shift from rural to urban living. Whereas in 1900 only 10% of people were residing in cities, this number now exceeds 54% and is expected to increase to 66% by 2050 1 . The development of urban environments is one of the principal causes of land use change worldwide 2 and believed to be the major cause of biodiversity alteration. Although floristic diversity 3 in urban areas is generally poorly documented, inside cities where comprehensive surveys have been undertaken plant species biodiversity is often remarkably high 2 . General data indicate that urbanization reduces native species diversity at a regional scale, while, on the contrary, total plant species richness often increases in the city centres in comparison to wildlands, due to the highly heterogeneous patchwork of urban habitats coupled with the human introduction of exotic species 4 . In addition, in some semi-natural environments within cities, endangered native plant species show consistent populations 5 , 6 making urban habitats preferential sites for their conservation.
It is argued that with the increase of global travel, which has led to a greater circulation of plant species and the spread from gardens of introduced alien plants 7 , 8 , 9 , 10 , there has been an enhancement of species richness counterbalancing or even exceeding the potential local extinction of native species 11 , 12 . This high number of non-native species within cities may actually improve ecosystem services (e.g. air quality, pollinator insect diversity) 13 , 14 and it is expected that the establishment of garden ornamental plants will keep increasing in the future 15 .
Ecological studies in cities are not only aimed at investigating floristic diversity and connected ecosystem features; since cities represent microcosms of environmental changes happening globally, they can serve as informative test cases for understanding ecological system dynamics and responses to climate and land changes 4 . Several previously published historical floristic/ecological studies considered comparisons over long periods of time (Table 1 ). These studies are of great historical and botanical value as they reflect the situation preceding most anthropogenic interventions, such as industrialization and increasing household density. In fact, original site conditions in urban habitats have changed tremendously and present living conditions for plants are completely different from those occurring during the last century 16 . Past information, climate records and urban structure of the study sites make it possible to evaluate the influence exerted by humans on local floras 17 .
In this context, the city of Bologna can be considered a perfect case study for the assessment of long-term floristic changes since two floristic surveys were carried out with a gap of more than 120 years. The first study dates back to 1894 18 , when the city still had its medieval arrangement, and aimed at describing the species occurring in the city and their habitats, while the second one was carried out in 2018 19 in the same sampling area in order to create an updated checklist of urban plant diversity. Throughout the paper, we will refer to the historical flora of 1894 as “Gabelli, 1894” and to the modern flora of 2018 as “Salinitro et al ., 2018”.
Taking into consideration both available datasets, the present report aims at: (i) describing the changes in species richness and composition that occurred over a 120-year time period; (ii) analysing the current presence of alien species compared to the past and their role in overall floristic richness of the city; (iii) evaluating changes in species life forms and their ecological requirements using Ellenberg indicator values (EIVs); (iv) correlating urban flora with climate records and urban changes.
The city of Bologna is located in the Po valley (Northern Italy), close to the Apennine mountains (latitude of 44°30′27″N; longitude 11°21′05″E), with an average altitude of 54 m a.s.l. ( http://www.comune.bologna.it/media/files/f2_relazionegeologica.pdf ).
The urban soil below the city of Bologna is an assemblage of river sediments (sand and clay) and anthropic deposits 20 . In the area corresponding to the most ancient part of the city founded by Romans, superficial layers are constituted by anthropic rubble, while clay and anthropic deposits are alternated in later urbanized areas like the medieval part ( http://ambiente.regione.emilia-romagna.it ). Bologna is crossed by two natural streams and numerous artificial canals deriving water from the adjacent Reno and Savena rivers. This dense water system was buried underground in the early 1900s, resulting in the almost complete absence of water inside the city. At present only a few hundred meters of the Reno canal remain unburied, representing the only residual humid habitat inside the city centre.
Climatic changes in the last decades
A study conducted in 2013 by ARPAE Emilia-Romagna ( http://storage.provincia.re.it ) revealed a clear trend of regional climate change that also involved the city of Bologna. The study reported measured temperatures and precipitations in the period 1961–2012, pointing out that since the 1990s an average increase of about 2 °C during the summer period occurred. This rise in temperature led to a failure of the hydro-climatic balance, so that the precipitation-evapotranspiration ratio has been negative since 1985 for most of the years. Since the 1970s, the presence of the urban heat island effect (U.H.I.) in the city of Bologna was also assessed, showing a general increase of 2.7 °C of winter temperatures in the city centre compared to the countryside 21 .
Urban changes in the study area during the last 120 years
At the end of the 19th century, Bologna still looked like a medieval city (Fig. 1A ) with a complete circle of walls (built around the XIV century), narrow roads mainly made of packed-earth and very low vehicular traffic. Public urban parks were almost completely absent, while large agricultural areas (nowadays completely disappeared) were present within the historical city centre located inside the walls. Bare brick walls were common while roads were mainly unpaved or cobblestone-covered, offering the perfect conditions for plant settling. Natural local materials were used, like wood and gypsum in buildings and pebbles or trachyte slabs in main streets. In this urban context, the first complete study on the urban flora of Bologna was carried out by Lucio Gabelli in 1894 18 . At the beginning of the 20th century, however, the city was completely renovated due to the large industrial expansion and the increase of the urban population. The ancient XIV century walls were almost completely demolished together with the moat and embankment surrounding them. All the main streets of the city were enlarged, rectified and paved, and the agricultural areas within the city centre were replaced by new buildings. Local materials were replaced and at present, streets are covered with asphalt, trachyte plates and porphyry cubes. Gypsum and wood lost importance as building materials, while brick walls were mostly plastered. Following the Second World War, the city experienced a great expansion outside its historical core, eliminating all the rural areas still present close to the city. To counterbalance the lack of green areas, tree lines were planted and small parks were created within the city centre ( http://dru.iperbole.bologna.it/normativa/prg-1985 ; http://www.rapu.it/ricerca/scheda_piano.php?id_piano=138 ; http://ambiente.regione.emilia-romagna.it ).
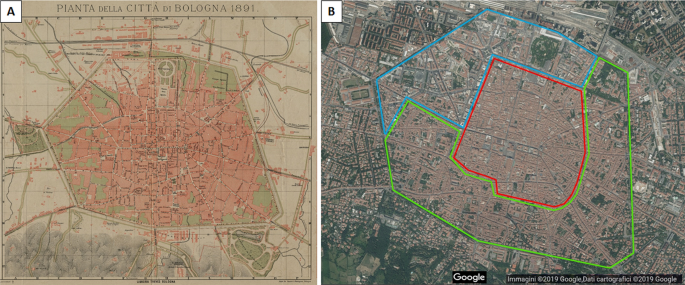
Study area corresponding to the historical centre of the city of Bologna. ( A ) Map of 1891 (from Biblioteca Archiginnasio Bologna, BCABO, GDS, Raccolta piante e vedute della città di Bologna, Cartella 8, n.79, ID 143); ( B ) 2018 satellite image downloaded from Google Maps ( www.google.com/maps ) and graphically elaborated with the ArcMap programme (version 10.1, ESRI, www.esri.com ) by M. Salinitro on the basis of the dataset “Salinitro et al . 2018” (Supplementary Table S1 ). HBA, historical building area (blue); NBA, new building area (green); OCC, old city centre (red).
Nowadays, Bologna’s historical city centre corresponds to the part of the city formerly surrounded by the ancient city walls of the XIV century. In this area, three zones with different building construction types are recognizable (Fig. 1B ). The new building area (NBA) was created after the Second World War and consists of tall buildings and wide streets with scarce vegetation mostly confined to public parks. The old city centre (OCC), located in the central part of the city, inherited part of the roman and medieval structures. It consists of narrow streets (except those widened at the beginning of the 1900s) with 3–4-storey buildings and lacks public green areas. Finally, in the southern and eastern part of the city, there is an area with historical buildings (HBA), ranging from XVIII century palaces to modern houses largely endowed with internal courtyards (Fig. 1B ). This area, though poor in public parks, is quite green with generally wide streets.
Data sources
The study area taken into consideration in the present paper corresponds to Bologna’s historical city centre identified as the part of the city formerly surrounded by the walls built in the XIV century. This particularly restricted study area was selected in order to allow the comparison of the data acquired in “Salinitro et al .” 19 , that aimed at a comprehensive survey of total plant richness, with those of “Gabelli” 18 , a study meant to describe the species occurring in the city and their habitats. Making a comparison between Bologna’s current flora and the one present over 120 years ago is certainly not an easy task, in particular due to the different methodologies followed for data collection and the changes of plant scientific names that occurred over the years. In fact, in “Salinitro et al .” 19 , data were generated from direct observations based on a network of transects that uniformly covered the study area. In this way it was possible to link every observation to a specific locality, allowing for a spatial analysis of the urban flora. The data described in “Gabelli” 18 were instead a mix of direct observations, done by the author himself, and reports by two other previous authors, without following a scientific sampling methodology. Species localities in the ancient study were rarely recorded (i.e. with the name of a street or an ancient city door), instead most species were described as common in certain habitats (i.e. along the roads, in paved areas, in gardens, etc.) or defined as widespread in the whole city. Plant names cited in the ancient study were often outdated (not used anymore in modern plant systematics) and, even though common names were not reported in “Gabelli” 18 , the linkage between ancient synonym and modern name was always possible.
Finally, data collection based on reports in “Gabelli” 18 has caused an inevitable bias in the estimation of species abundance. In fact, most of the old reports focused on unusual or rare species in the urban area, leading to an enrichment in rare species and an underestimation or lack of some common species, as in the case of Plantago lanceolata L., Sagina apetala Ard. and Solanum nigrum L., which were not recorded but certainly already present.
Despite the limits listed above, the old floristic study constitutes an important and unique source of data on the urban flora of Bologna at the end of the 19 th century, from which it is possible to draw relevant conclusions on the influence that climate change, urban planning and floricultural trends have had over the last 120 years on Bologna’s urban flora biodiversity.
Data processing
To compare the current flora to that of 1894, and to correlate them with climate and urban changes, Ellenberg indicator values (EIVs) 22 were applied to the Italian flora 23 and to exotic species 24 . As EIVs for neophytes were not available in the literature, the indices were calculated by comparison with known species growing in the same habitat following the method of Domina et al . 24 . To ascertain the difference between the modern and ancient flora, the Sørensen similarity index was calculated 25 .
A standardization of the two datasets with respect to botanical names and geographical localities was performed. All the historical plant names were linked to modern ones using the PlantList website ( www.theplantlist.org ) which provided a complete list of synonyms associated with each species. No ambiguities were evidenced in correlating old and current plant names. When possible, the city’s historical plant distribution was inferred by linking the names of the old toponyms to modern ones. Species were considered present in specific ancient habitats (i.e, road sides, gardens, etc.) when they were also detected in the corresponding modern habitat. Plants defined as common or widespread in the 1894 city were also taken into consideration for the whole modern city area. Despite all efforts to reconstruct the spatial distribution of the ancient flora, the lack of detailed information in the “Gabelli, 1894” dataset did not allow a spatial comparison with the “Salinitro et al ., 2018” dataset.
To determine how species richness in different building areas was influenced by paving materials, and which materials mainly characterized each type of building area, a non-parametric multi-dimensional scaling (NMDS) was performed (software R , package Vegan 26 ). Manhattan distance was used for computation, convergence was reached after 100 iterations and the stress of the optimal solutions was 0.0397. To analyse the distribution of species according to their presence or absence in different urban building areas, a PCA analysis was performed. The G-test (software R , package DescTools 27 ) was used to evaluate significant differences between the two datasets with regard to EVIs, life forms, main botanical families and chorotypes. Graphic elaborations were carried out using Microsoft Excel and R software (R Core Team, Vienna, Austria).
The whole set of raw data is available in Supplementary Table S1 .
General floristic trends
When comparing floristic data in”Gabelli, 1894” with those in “Salinitro et al . 2018” 18 , 19 , a big increase in the number of recorded taxa could be detected. Recorded botanical families increased from 41 to 101, genera from 138 to 306 and species from 176 to 477 (Fig. 2B ). In 120 years, the number of alien species has more than doubled, from less than 12.5% to the current 30.1% (i.e. from 22 to 144 species of the respective total amounts) (Fig. 2A ). A large turnover in species was detected, with only 113 species still in common between the two floras; the Sørensen similarity index value was 0.35, indicating a mean 65% of species turnover in 120 years. Over this time period some of the most represented botanical families (Fig. 2C ) have changed. Apart from Asteraceae and Poaceae species that resulted the most abundant in both floras, those of the Fabaceae and Lamiaceae families decreased. In contrast, the Asparagaceae family, not recorded in the old study, entered in the top ten, while the Rosaceae family underwent a significant increase of 68%. The species newly introduced in Bologna’s contemporary flora 19 (mostly alien ornamental) belong to different botanical families, with the result that 30% of the current botanical families are represented by only one species (data not shown). A comparison between the life forms (Fig. 2D ) of the ancient and present floras pointed out a noticeable increase in the incidence of phanerophytes (from 6 to 25%) and geophytes (from 4 to 12%). Therophytes and hemicryptophytes decreased (from 49 to 32% and from 36 to 26%, respectively) while no change was found for chamaephytes (around 5%). In addition, the chorological spectra deeply changed between the ancient and the modern floras (Fig. 3 ), evidencing a doubling of naturalised (from 13.6 to 28.9%) and stenomediterranean species (from the 3.4 to the 6.5%). As a consequence, all the other classes experienced a decrease in comparison with “Gabelli, 1894”, in particular the eurasiatic species (from 10.8 to 5.9%).
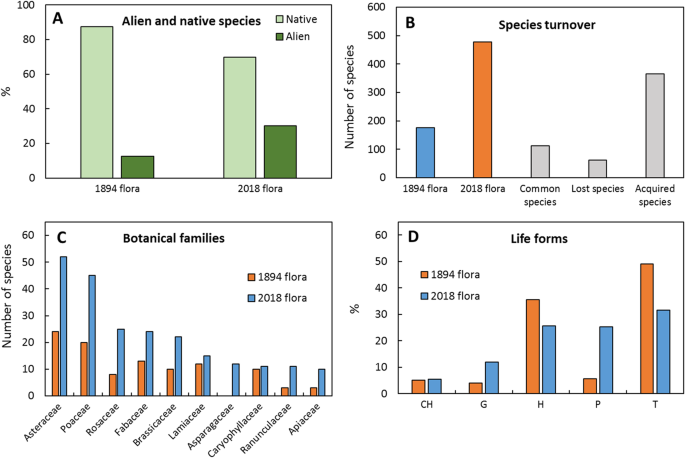
Comparison of floristic data reported in the two reference studies, Gabelli 18 and Salinitro et al . 19 . ( A ) Percentage of alien and native species. ( B ) Total species and number of lost, acquired and common species. ( C ) Most abundant botanical families, the statistical difference in the distribution of families was tested by G-test ( p < 0.01). ( D ) Life forms: CH = chamaephytes; G = geophytes; H = hemicryptophytes; P = phanerophytes; T = therophytes. The statistical difference in the distribution of families was determined using the G-test ( p < 0.01).
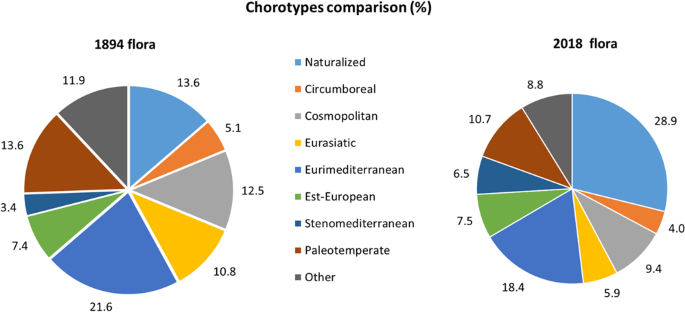
Comparison of chorotypes between “Gabelli, 1894” 18 and “Salinitro et al ., 2018” 19 . Some chorotypes have been grouped into a single category, such as Naturalised (Naturalised + Cultivated), Est-European (Eurosiberian + Eurocaucasian), and Cosmopolitan (Cosmopolitan + Subcosmopolitan). The statistical difference in the distribution of chorotypes was tested by G-test ( p < 0.01).
Changes in ecological requirements: Ellenberg indicator values analysis
When comparing Ellenberg indicator values (EIVs), associated to the two datasets several interesting differences were revealed. The comparison was performed by taking into consideration light (L), moisture (F), temperature (T), reaction (R), continentality (K) and nutrients (N) EIVs, while the salinity (S) index was not included in the analysis since most of the species recorded in both studies were from non-saline soils. Differences between the “Gabelli, 1894” and “Salinitro et al ., 2018” floras were detected analysing the two whole datasets and lost and acquired species of the modern flora with respect to the old one were highlighted (Fig. 4 ).
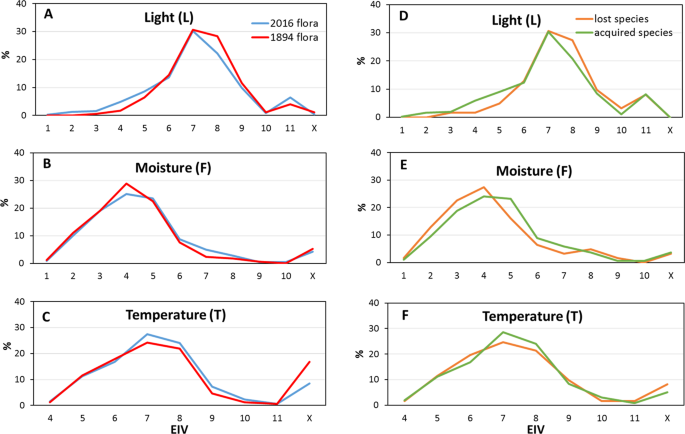
Ellenberg indicator values for “Gabelli, 1894” 18 and “Salinitro et al ., 2018” 19 and for acquired and lost species of the modern and 1894 floras. ( A , D ) light (L) index; ( B , E ) moisture ( F ) index; ( C , F ) temperature (T) index. The statistical difference was tested by G-test ( p < 0.05).
K, R, N indexes did not show significant changes ( p = 0.456, p = 0.345, p = 0.746 respectively) between the two datasets and, therefore, they were not included in Fig. 4 . The complete set of data is available in Supplementary Table S1 . The light (L) index pattern of the two whole floristic datasets (Fig. 4A ) indicates that shade-tolerant and intermediate species (categories 1–5) experienced an increase between 1 to 3%, moderate heliophilous species (categories 8 and 9) showed a strong decrease (respectively, 6 and 2%) while highly heliophilous species (category 11) experienced an increase of 2.4% ( p = 0.053). These general changes were even more emphasized when lost and acquired species (of the modern flora) were compared, with exception of category 11 ( p = 0.032) (Fig. 4D ). The moisture (F) index (Fig. 4B ) showed no significant changes in drought-resistant species (categories 1 to 3), while a 3.7% decrease was observed in category 4. Intermediate categories (6–8) increased by between 4.7 and 3.5%, while no significant changes were observed for hygrophilous species (categories 9–10) and moisture adaptable species (category X). Overall, the changes in F index were associated with a p value of 0.081. On the other end, when the moisture indexes of lost and acquired species of the present flora were compared (Fig. 4F ), a 4% decrease of drought-resistant species (categories 2–4), and an enrichment (2.7 to 7%) of intermediate tolerant species (categories 5 to 7) were found ( p = 0.029). The temperature (T) index in both studies starts from category 4 as no plants tolerant to very low temperatures (e.g. alpine or sub-alpine species) were or are actually present in the Bologna study area. The analysis of T indexes (Fig. 4C ) indicates that microthermal and mesothermal species (categories 4 to 6) did not change in 120 years. On the contrary, macrothermal species increased by 3% (categories 7 to 10) at the expense of plants adapted to a wide range of temperature. In fact, the number of species belonging to category X halved in 120 years (from 16.8 to 8.5%) ( p = 0.033). A similar pattern was found when comparing lost and acquired species (Fig. 4E ) (p = 0.024).
Species diversity in relation to urban structure
During the present study, particular attention was given to differences in the city structure and the distribution of different building materials. Building materials (especially paving materials) are in fact primary selective factors for plant colonisation.
Large part of the current floral richness (477 plant species) of Bologna’s historical city centre 19 is ascribable to green areas, which alone contain 418 species (Fig. 5A ). Among the three different building areas identified (Fig. 1B ), new building area (NBA), old city centre (OCC), and historical building area (HBA), several differences were observed regarding the presence of green spaces and the type of flooring used that affect floristic richness and composition. The presence of green spaces adjacent to streets, such as gardens, flowerbeds or tree-lines, was quite different: 40.6% in NBA, 36.2% in HBA and only 12.7% in OCC. The most widespread paving for HBA and NBA was asphalt, while trachyte plates were more abundant in OCC. The presence of porphyry cubes was almost constant among the three building areas, while cobblestone paving was scarcely represented (Fig. 5B ). The influence of paving materials on total species richness was shown in Fig. 6A . Transects richest in species were primarily characterized by the presence of green spaces and asphalt, while, on the contrary, those showing porphyry cubes and trachyte slabs were the poorest. Accordingly, decreasing values of species richness were detected for HBA, NBA and OCC containing on the whole 244, 226 and 132 species, respectively (Fig. 5A ). Differences in structure and materials present in each type of building area not only affected species richness but also led to the differentiation into three different sub-floras (Fig. 6B ). Eight species were exclusive to OCC, among which roof plants, like Polypodium interjectum Shivas and Sedum dasyphyllum L.; 58 species were only found in HBA, among which many ornamental garden plants, such as Alcea rosea L., Buxus sempervirens L. and Elaeagnus pungens Thunb.; 47 species grew exclusively in NBA, among which some exotic species of sunny places, like Dysphania pumilio (R. Br.) Mosyakin & Clemants, Delosperma cooperi (Hook.f.) L. Bolus and Lepidium virginicum L.; and 159 species were only detected in green areas, among them many rare native species of shady habitats, like Scilla bifolia L., Silene flos-cuculi L. and Vinca minor L. A large number of species (39.7%) were shared between two or more building areas, such as Mahonia japonica (Thumb.) DC., Papaver rhoeas L. in NBA and HBA and Ailanthus altissima (Mill.) Swingle, Plantago major L., and Stellaria media L. (Vill.) present in all three building areas.
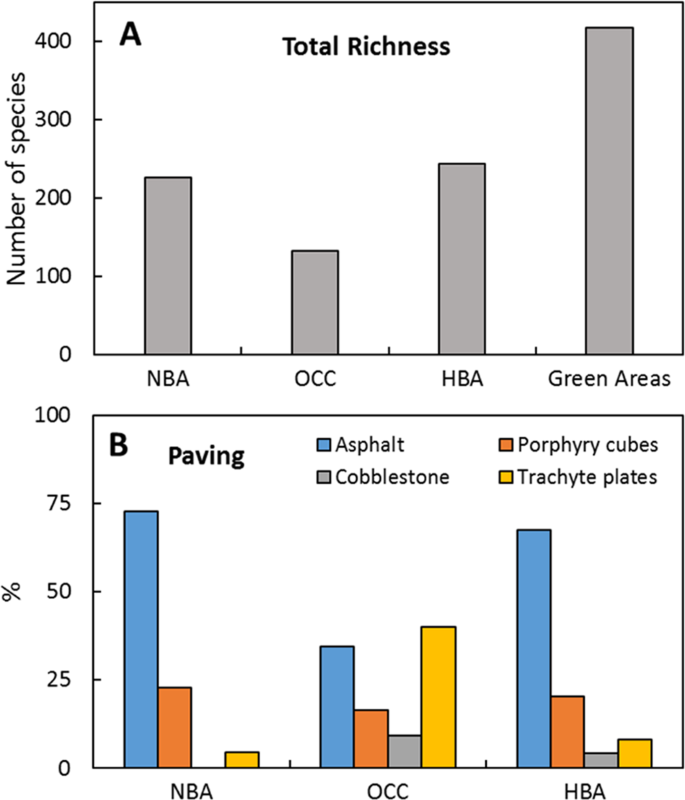
Floristic richness variation in the different building areas. ( A ) Total species richness in different city centre areas. HBA, historical building area; NBA, new building area; OCC, old city centre. ( B ) Type of paving coverage in the different urban areas.
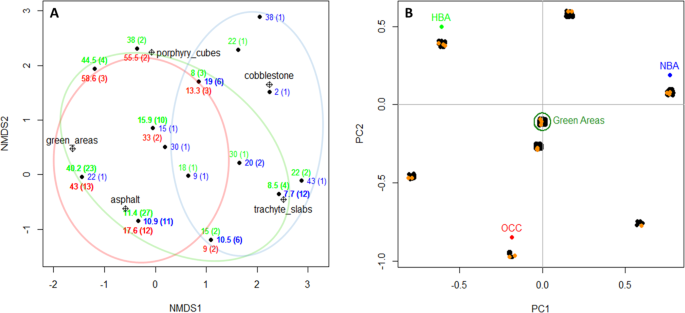
Species richness in Bologna’s different urban areas and correlation with paving materials. ( A ) NMDS analysis showing the distribution of transects (black dots) according to the presence of different paving materials (empty crossed-squares). Black dots represent several overlapped transects with similar features. Numbers represent the average richness and (in brackets) the amount of transects. Blu colour, HBA; green, NBA; red, OCC. ( B ) PCA analysis showing the grouping of species according to their presence in the different building areas. Several species are overlapped in each group of black dots, each dot represents a single species. Dark green circle: species growing exclusively in green spaces. Orange dots represent typical species of each habitat (see text for details). PC2 vs PC3 was used to allow better species grouping. In all the figures, HBA: historical building area, NBA: new building area, OCC: old city centre.
Comparison between the 1894 and 2018 floristic data 18 , 19 clearly demonstrated the impact of climatic and urban changes on floristic diversity and assemblage in Bologna’s historical city centre.
In agreement with several published reports on other cities 11 , 28 , 29 worldwide, the present results point out that the total number of species increased dramatically over a 120 years timespan, in particular, the number of alien species which doubled (from 12.5 to 30.1% of total species) (Fig. 2A ). The species number increase seems to be a common trend in many urban areas 17 , 28 , 30 , 31 , 32 , nonetheless the large number of species detected in “Salinitro et al . 2018” could also be partly due to an underestimation of total species richness in “Gabelli. 1894”. However, the recorded amount of alien species was in line with European and world averages, e.g. 25.2% 28 and 28% 12 . A similar increase was also found when higher taxa levels (genera and families) were analysed and, in general, the high diversity in cities could be ascribed to the large variety of urban microhabitats and, above all, to the human voluntary and non-voluntary introduction of species for ornamental purposes 7 , 8 , 33 , 34 . In fact, the cultivation of ornamental plants for leisure and decoration represents the primary factor contributing to the increase of neophytes 9 , 15 . Asteraceae and Poaceae were found to be the most widespread botanical families in Bologna’s current flora, similarly to many other urban areas in the world 35 . The increase of Asparagaceae , Rosaceae and Oleaceae could be mainly ascribed to the naturalisation of cultivated species. Interestingly, 65% of recently lost species are represented by indigenous ones, as previously detected also in other cities such as Zürich 29 (about 60%). As a confirmation of previous considerations, the Sørensen similarity index, indicating the species turnover and calculated between Bologna’s ancient and modern flora, is significantly lower (0.35) compared to other cities like Zürich, Brussels and Plzen 16 , 17 , 29 (on average 0.80). This large species turnover detected in Bologna over 120 years may reflect the urbanistic changes the city centre underwent from the beginning of the 20 th century, like the disappearance of cultivated fields and medieval walls, which dramatically reduced the presence of green areas in the city 36 . Therefore, the huge floristic turnover connected to urban modification regarded in particular the disappearance of crop species 36 . “Gabelli, 1894” 18 reported fruit-bearing trees, cereals and common weeds as widely naturalised inside the city centre. In Bologna’s current flora, field species, like Legousia speculum-veneris (L.) Chaix, Echium vulgare L. and Matricaria chamomilla L., and cultivated crops like Zea mays L., are no longer present in the urban area due to the intense urbanization process that occurred over the last century and changed the shape of the city (Fig. 1 ). A similar reduction in field species has previously been observed in other cities like, e.g., Brussels 16 . On the other hand, because of the choice of a restricted and highly urbanized study area for both reference studies, 18 , 19 stochastic processes connected to extreme anthropogenic influences are predominant in urban environments 37 .
When taking into consideration life form variations, the current increase in phanerophytes (Fig. 3 ) seems to be a common trait connected with the increase of naturalised species in urban floras 17 , 38 . Similarly, the growing amount of woody species (mainly ornamental plants) has been hypothesized to be in relation with the naturalisation of cultivated species 7 , 8 , 17 , 38 . Due to the strong increase of woody plants, the total percentage of therophytes and hemicryptophytes decreased (Fig. 3 ). Nonetheless, the current flora of Bologna is still dominated by therophytes and hemicryptophytes, while most of the newly introduced phanerophytes occur only temporarily, without forming persisting populations, as was also pointed out by Chocholousková et al . 17 with regard to the flora of the city of Plzen. The therophytes/hemicryptophytes ratio also decreased in the period from 1894 (1.36) to 2018 (1.23) indirectly confirming climatic data that evidence an increase of summer drought over the years ( http://storage.provincia.re.it ) which generally favoured the growth of annual plants. Moreover, the increase of geophytes species (Fig. 3 ) (from 4 to 12%) could be explained by the recent naturalisation of some ornamental species (e.g. Iris germanica L.), even if an underestimation of this type of plants in the past study could be hypothesized, as some common certainly present native species, like Allium vineale L., were not recorded. Similar results were reported for several other European cities 39 , 40 . However, the most glaring sign of the adaptation of the current flora to a warmer and drier climate is the increase in stenomediterranean species with respect to the past century. In fact, as pointed out by Hruška 41 and Interdonato et al . 42 , a high ratio of stenomediterranean/eurimediterranean species is typical of cities in southern Italy, which are usually on average much warmer than the city of Bologna located in northern Italy. Therefore, these cities may have started to host plants coming from warmer world regions 37 .
The comparison of EIVs (Fig. 4 ) pointed out the ongoing adaptation of the flora to the changes that occurred in the city over the last century. In particular, an expansion of all shade-tolerant categories at the expense of heliophilous ones was observed (Fig. 4A,D ). Analogous findings were reported for the cities of Plzen, Brussels, Zurich and Halle using data collected over a similar timespan 16 , 17 , 29 , 36 . The observed changes could be correlated to urbanistic and structural modifications of the city, which include a general enlargement of the main avenues and planting of trees in parks and streets 36 . The currently present tree lines can, therefore, be considered responsible for the settling of many understory and sciophilous species 29 , 36 , like Hedera helix L. and Trachycarpus fortunei (Hook.) H. Wendl. Pteridophytes also largely contributed to the shade-tolerant plant category (light index categories 1 to 5) while they were poorly represented in the historical flora list, being probably underestimated. Moisture index changes (Fig. 4B,E ) mainly reflected the anthropic changes of the city, like the widespread use of irrigation systems inside Bologna’s historical centre. This practice inevitably overshadows the effects of climate changes on floral biodiversity, as long as the soil moisture content is artificially modified. F index data collected for Bologna’s city centre showed an increased presence of hygrophilous plants (categories 6 to 8, Fig. 4B ) analogously to what reported for several other European cities 43 like Plzen, Halle and Brussels 16 , 17 , 36 . This trend was confirmed by EIVs analysed for lost and acquired species (Fig. 4E ) with a net gain of hygrophilous species in the 2018 flora. As previously demonstrated for other cities, for example Boston 44 , irrigated areas turn out to be a shelter for species that otherwise would be no longer present in the area, such as hygrophilous species like Epilobium montanum L., Equisetum arvense L. and Eclipta prostrata (L.) L., which were recently found in Bologna. However, it is not possible to exclude the presence of such species from the 1894 flora. In fact, at that time the city centre was characterized by a wide extension of channels that probably hosted a large number of plants, including many hygrophilous species, which were not recorded by Gabelli as these channels were not investigated.
Analysing the temperature EIV (Fig. 4C,F ), an increase in mesothermal and macrothermal (categories 7 to 10) species and a concomitant decrease of tolerant species (category X), was evidenced. A similar trend has been found in most of the cities previously investigated, in agreement with a global temperature increase (more evident in urban environments) in the last decades 16 , 29 , 40 . Key players of these biodiversity changes are many Mediterranean (e.g. Pistacia therebinthus L. and Cupressus sempervirens L.) and alien thermophilic species (e.g. D . cooperi (Hook.f.) L.Bolus and Chlorophytum comosum (Thunb.) Jacques), which in recent years have started to survive the winter given the milder temperatures. The tropicalization of the flora is, in fact, supported by the U.H.I.’s in many European cities 33 , 45 which, in particular in the Bologna city area, resulted in an increase of winter low temperatures of 2.7 °C compared to the past 21 . Moreover, the strong decrease of species with a wider tolerance (category X) was confirmed by a shift toward extreme temperatures, especially in summer. In the present data, the maximum plant species richness was detected in green areas (e.g. parks, flowerbeds or tree lines) (Figs 5A and 6A ), as confirmed also by similar reports for the cities of Rome 46 and Zürich 29 with in the latter case the richest area sampled being the one containing the botanical garden.
The positive correlation between species richness and green areas was further confirmed by the progressive impoverishment of urban biodiversity going from HBA to NBA to OCC, in accordance with the decreasing presence of green areas. Similar patterns were reported for the city of Rome 46 , where the historical centre (approximately corresponding to Bologna’s OCC) and new 1950–1980 building developments (similar to NBA) showed the poorest levels of plant diversity. Our findings also support the thesis that paving materials differently contributed to enhance or decrease street floristic richness (Fig. 6A ), suggesting that building materials could have played an important role in characterizing current urban flora. Furthermore, previous results on Bologna 19 showed a negative correlation between floristic diversity and street width (generally higher in NBA and HBA with respect to OCC) suggesting that the larger modern streets have played an important role in increasing plant richness coupled with the creation of new green spaces.
Conclusions
The analysed data demonstrated that over 120 years the floristic diversity of Bologna’s historical city centre has deeply changed as a result of modifications in urban architecture, warmer climate conditions and the increasing anthropogenic pressure on the urban environment. In general, climate and urban changes did not lead to a loss of floristic diversity, but rather to a loss of “typicality”, with an almost tripled amount of alien species (mostly introduced ornamental plants 7 , 8 ) and the disappearance of native and crop species, resulting in a homogenisation of the flora 5 , 47 . Nevertheless, the addition of tree lines along the main streets, the creation of small parks and the increase of irrigated areas around the historical city centre have made the urban landscape more heterogeneous than in the past, creating opportunities for the growth of a wider range of species. This factor coupled with the massive introduction of alien species are believed to be the cause of the general increase of biodiversity over the past 120 years.
Data Availability
All data generated or analyzed during this study are included in the published article and in Supplementary Table S1 .
United Nation Population Division. World Urbanization Prospects: The 2014 Revision . New York, USA (United Nations Publications, 2015).
Miller, J. R. & Hobbs, R. J. Conservation where people live and work. Conserv Biol 16 , 330–337 (2002).
Article Google Scholar
Niemelä, J. Ecology and urban planning. Biodivers Conserv 8 , 119–131 (1999).
Grimm, N. B. et al . Global change and the ecology of cities. Science 319 , 756–760 (2008).
Article ADS CAS Google Scholar
Kühn, I., Brandl, R. & Klotz, S. The flora of German cities is naturally species rich. Evol Ecol Res 6 , 749–764 (2004).
Google Scholar
Ives, C. D. et al . Cities are hotspots for threatened species. Global Ecol Biogeogr 25 , 117–126 (2016).
van Kleunen, M. et al . The changing role of ornamental horticulture in alien plant invasions. Biol Rev Camb Philos Soc 93 , 1421–1437 (2018).
Muller, N. & Sukopp, H. Influence of different landscape design styles on plant invasions in Central Europe. Landsc Ecol Eng 12 , 151–169 (2016).
Kühn, I. & Klotz, S. Floristischer Status und gebietsfremde Arten. Schr.reihe Veg.kd. 38 , 47–56 (2002).
Pyšek, P. et al . A global assessment of invasive plant impacts on resident species, communities and ecosystems: the interaction of impact measures, invading species’ traits and environment. Global Change Biol 18 , 1725–1737 (2012).
Article ADS Google Scholar
Tait, C. J., Daniels, C. B. & Hill, R. S. Changes in species assemblages within the Adelaide Metropolitan Area, Australia, 1836–2002. Ecol Appl 15 , 346–359 (2005).
Aronson, M. F. J. et al . A global analysis of the impacts of urbanization on bird and plant diversity reveals key anthropogenic drivers. P Roy Soc B-Biol Sci 281 , 20133330 (2014).
Kowarik, I. Novel urban ecosystems, biodiversity, and conservation. Environ Pollut 159 , 1974–1983 (2011).
Article CAS Google Scholar
Hansen, A. J. & DeFries, R. Ecological mechanisms linking protected areas to surrounding lands. Ecol Appl 17 , 974–988 (2007).
Gregor, T., Bönsel, D., Starke-Ottich, I. & Zizka, G. Drivers of floristic change in large cities - A case study of Frankfurt/Main (Germany). Landscape Urban Plan 104 , 230–237 (2012).
Godefroid, S. Temporal analysis of the Brussels flora as indicator for changing environmental quality. Landscape Urban Plan 52 , 203–224 (2001).
Chocholouskova, Z. & Pyšek, P. Changes in composition and structure of urban flora over 120 years: a case study of the city of Plzen. Flora 198 , 366–376 (2003).
Gabelli, L. Notizie sulla vegetazione ruderale della città di Bologna. Malpighia 8 , 41–68 (1894).
Salinitro, M., Alessandrini, A., Zappi, A., Melucci, D. & Tassoni, A. Floristic diversity in different urban ecological niches of a southern European city. Sci Rep 8 , 15110, https://doi.org/10.11038/s41598-15018-33346-15116 (2018).
Article ADS PubMed Google Scholar
Vai, G. B. & Martini, I. P. Anatomy of an Orogen: The Apennines and Adjacent Mediterranean Basins Dordrecht (Springer Science + Business Media, 2001).
Zauli Sajani, S., Tibaldi, S., Scotto, F. & Lauriola, P. Bioclimatic characterisation of an urban area: a case study in Bologna (Italy). Int J Biometereol 52 , 779–785 (2008).
Ellenberg, H. Zeigerwerte der Gefäβflanzen Mitteleuropas (Indicator values of vascular plants in Central. Europe). Scr Geobot 9 , 1–122 (1979).
Pignatti, S., Menegoni, P. & Pietrosanti, S. Biondicazione attraverso le piante vascolari. Valori di indicazione secondo Ellenberg (Zeigerwerte) per le specie della Flora d’Italia. Braun-Blanquetia 39 , 1–97 (2005).
Domina, G., Galasso, G., Bartolucci, F. & Guarino, R. Ellenberg Indicator Values for the vascular flora alien to Italy. Fl Medit 28 , 53–61 (2018).
Sørensen, T. J. Method of establishing groups of equal amplitude in plant sociology based on simi- larity of species content , and its application to anal- yses of the vegetation onl Daniish commons . Vol. 4 Copenhaghen, Danmark (Kommission Hos Ejnar Munksgaard, 1948).
Oksanen, J. et al . Vegan: Community Ecology Package. R package version 2.4–3, https://CRAN.R-project.org/package=vegan , (2017).
Signorell, A. et al . Package DescTools, Tools for descriptive statistics. R package version 0.99.28.(2019).
Pyšek, P. Factors affecting the diversity of flora and vegetation in Central-European settlements. Vegetatio 106 , 89–100 (1993).
Landolt, E. Some results of a floristic inventory within the city of Zürich. Preslia 72 , 441–455 (2000).
Pyšek, P. Alien and native species in Central European urban floras: a quantitative comparison. J Biogeogr 25 , 155–163 (1998).
Zhao, J. J. et al . Plant species composition in green spaces within the built-up areas of Beijing, China. Plant Ecol 209 , 189–204 (2010).
Clemants, S. E. & Moore, G. Patterns of species richness in eight northeastern United States cities. Urban Habitats 1 , 4–16 (2003).
Sukopp, H. & Werner, P. Urban environment and vegetation, in Man’s impact on vegetation (eds Holzner, W., Werger, M. J. A. & Ikusima, I.), 247–260, The Hague, Netherlands (Dr W. Junk Publisher, 1983).
Sukopp, H. & Wurzel, A. The effects of climate change on the vegetation of Central European cities. Urban Habitats 1 , 66–86 (2003).
Pyšek, P. et al . Naturalized alien flora of the world: species diversity, taxonomic and phylogenetic patterns, geographic distribution and global hotspots of plant invasion. Preslia 89 , 203–274 (2017).
Knapp, S., Kuhn, I., Stolle, J. & Klotz, S. Changes in the functional composition of a Central European urban flora over three centuries. Perspect Plant Ecol 12 , 235–244 (2010).
Sukopp, H. On the early history of urban ecology in Europe. Preslia 74 , 373–393 (2002).
Sal’nikov, A. L. & Pilipenko, V. N. Anthropogenic transformation of flora in the city of Astrakhan and its environs over the past 100 years. Rus J Ecol 36 , 383–390 (2005).
Hruška, K. A comparative analysis of the urban flora in Italy. Braun-Blanquetia 3 , 45–50 (1989).
Stešević, D., Caković, D. & Jovanović, S. The urban flora of Podgorica (Montenegro, SE Europe): Annotated checklist, distribution atlas, habitat and life-forms, taxonomic, phytogeographical and ecological analysis. Ecologia Montenegrina Suppl. 1 , 1–171 (2014).
Hruška, K. Research on plant strategies in urban areas, in Applied Vegetation Ecology (eds Song,Y., Dierschke, H. & Wang, X.), 360–363, Shangai, China (Est China Norm. Univsity Press, 1994).
Interdonato, M., Hruška, K. & Villari, R. Research on the urban flora of Messina. Annali di Botanica 3 , 105–116 (2003).
Wittig, R. Siedlungsvegetation . Stuggard, Germany (Eugen Ulmer, 2002).
Drayton, B. & Primack, R. B. Plant species lost in an isolated conservation area in Metropolitan Boston from 1894 to 1993. Conserv Biol 10 , 30-39 (1996).
Gilbert, O. H. Ecology of urban habitats . London, UK (Chapman & Hall, 1989).
Celesti-Grapow, L., Pysek, P., Jarosik, V. & Blasi, C. Determinants of native and alien species richness in the urban flora of Rome. Divers Distrib 12 , 490–501 (2006).
Wittig, R. & Becker, U. The spontaneous flora around street trees in cities-A striking example for the worldwide homogenization of the flora of urban habitats. Flora 205 , 704–709 (2010).
Klotz, S. & Gutte, P. Biologisch- ökologische Daten zur Flora von Leipzig – ein Vergleich. Acta Acad Sci 1 , 94–97 (1992).
Kent, M., Stevens, R. A. & Zhang, L. Urban plant ecology patterns and processes: a case study of the flora of the City of Plymouth, Devon, U.K. J Biogeogr 26 , 1281–1298 (2001).
Lososová, Z. & Simonová, D. Changes during the 20th century in species composition of synanthropic vegetation in Moravia (Czech Republic). Preslia 80 , 291–305 (2008).
Klotz, S. Floristische und vegetationskundliche Untersuchungen in Stadten der DDR. Düsseldorf. Geobotanisches Kolloquium 4 (1987).
Download references
Acknowledgements
We wish to thank Dr. Marianne Louise van Buuren for the editing of the English language.
Author information
Authors and affiliations.
Department of Biological Geological and Environmental Sciences, University of Bologna, Via Irnerio 42, 40126, Bologna, Italy
Mirko Salinitro & Annalisa Tassoni
Institute for Cultural Heritage, Via Galliera 21, 40121, Bologna, Italy
Alessandro Alessandrini
Department of Chemistry “Giacomo Ciamician”, University of Bologna, Via Selmi 2, 40126, Bologna, Italy
Alessandro Zappi
You can also search for this author in PubMed Google Scholar
Contributions
M.S. analysed data and contributed to the writing of the manuscript; A.A. contributed to the coordination of the study; A.Z. contributed to data statistical analysis; A.T. coordinated the study and contributed to the writing of the manuscript. All authors revised the manuscript.
Corresponding author
Correspondence to Annalisa Tassoni .
Ethics declarations
Competing interests.
The authors declare no competing interests.
Additional information
Publisher’s note: Springer Nature remains neutral with regard to jurisdictional claims in published maps and institutional affiliations.
Supplementary information
Supplementary dataset, rights and permissions.
Open Access This article is licensed under a Creative Commons Attribution 4.0 International License, which permits use, sharing, adaptation, distribution and reproduction in any medium or format, as long as you give appropriate credit to the original author(s) and the source, provide a link to the Creative Commons license, and indicate if changes were made. The images or other third party material in this article are included in the article’s Creative Commons license, unless indicated otherwise in a credit line to the material. If material is not included in the article’s Creative Commons license and your intended use is not permitted by statutory regulation or exceeds the permitted use, you will need to obtain permission directly from the copyright holder. To view a copy of this license, visit http://creativecommons.org/licenses/by/4.0/ .
Reprints and permissions
About this article
Cite this article.
Salinitro, M., Alessandrini, A., Zappi, A. et al. Impact of climate change and urban development on the flora of a southern European city: analysis of biodiversity change over a 120-year period. Sci Rep 9 , 9464 (2019). https://doi.org/10.1038/s41598-019-46005-1
Download citation
Received : 02 January 2019
Accepted : 18 June 2019
Published : 01 July 2019
DOI : https://doi.org/10.1038/s41598-019-46005-1
Share this article
Anyone you share the following link with will be able to read this content:
Sorry, a shareable link is not currently available for this article.
Provided by the Springer Nature SharedIt content-sharing initiative
This article is cited by
Floristic changes of vascular flora in the city of rome through grid-cell census over 23 years.
- Carlo Fratarcangeli
- Giuliano Fanelli
- Alessandro Travaglini
Urban Ecosystems (2022)
Functional diversity changes in native and alien urban flora over three centuries
- Marija Milanović
- Ingolf Kühn
- Sonja Knapp
Biological Invasions (2021)
By submitting a comment you agree to abide by our Terms and Community Guidelines . If you find something abusive or that does not comply with our terms or guidelines please flag it as inappropriate.
Quick links
- Explore articles by subject
- Guide to authors
- Editorial policies
Sign up for the Nature Briefing newsletter — what matters in science, free to your inbox daily.


An official website of the United States government
The .gov means it’s official. Federal government websites often end in .gov or .mil. Before sharing sensitive information, make sure you’re on a federal government site.
The site is secure. The https:// ensures that you are connecting to the official website and that any information you provide is encrypted and transmitted securely.
- Publications
- Account settings
Preview improvements coming to the PMC website in October 2024. Learn More or Try it out now .
- Advanced Search
- Journal List
- ScientificWorldJournal
- v.2014; 2014

Floristic Diversity and Distribution Pattern of Plant Communities along Altitudinal Gradient in Sangla Valley, Northwest Himalaya
Pankaj sharma.
1 National Bureau of Plant Genetic Resources, Regional Station, Phagli, Shimla 171 004, India
S. S. Randhawa
2 State Council for Science, Technology and Environment, Shimla 171 009, India
Rajesh Kumar
3 School of Basic Sciences and Research, Sharda University, Knowledge Park III, Greater Noida, Gautam Budh Nagar 201306, India
Himalayas are globally important biodiversity hotspots and are facing rapid loss in floristic diversity and changing pattern of vegetation due to various biotic and abiotic factors. This has necessitated the qualitative and quantitative assessment of vegetation here. The present study was conducted in Sangla Valley of northwest Himalaya aiming to assess the structure of vegetation and its trend in the valley along the altitudinal gradient. In the forest and alpine zones of the valley, 15 communities were recorded. Study revealed 320 species belonging to 199 genera and 75 families. Asteraceae, Rosaceae, Apiaceae, and Ranunculaceae were dominant. Among genera, Artemisia followed by Polygonum , Saussurea , Berberis , and Thalictrum were dominant. Tree and shrub's density ranged from 205 to 600 and from 105 to 1030 individual per hectare, respectively, whereas herbs ranged from 22.08 to 78.95 individual/m 2 . Nearly 182 species were native to the Himalaya. Maximum altitudinal distribution of few selected climate sensitive species was found to be highest in northeast and north aspects. This study gives an insight into the floristic diversity and community structure of the fragile Sangla Valley which was hitherto not available.
1. Introduction
Himalayas comprised of earth's most multifaceted and diverse montane ecosystems, characterized by a harsh climate, a strong degree of seasonality, and a high diversity of both plant communities and species [ 1 , 2 ]. These are geodynamic young mountains and have been recognized as one of the globally important biodiversity hotspots [ 3 , 4 ]. In these mountains presence of often sharp environmental gradients due to rapid geoclimatic variations generate diverse vegetation and community types having high plant species diversity [ 5 ]. Here a wide range of altitude, rainfall, climate, geological conditions, river systems, and topography have given rise to an immense diversity of ecosystems and ultimately to immense biological diversity.
The structure, composition, and vegetative functions are most significant ecological attributes of a particular ecosystem, which show variations in response to environmental as well as anthropogenic variables [ 6 – 8 ]. Major threats to ecosystems and biodiversity are habitat loss and fragmentation, overexploitation, pollution, invasions of alien species, and global climate change [ 9 ] with disruption of community structure.
The vegetation distribution pattern, communities, and population dynamics in high altitude arid areas of the fragile Himalaya have seldom given the due attention by researchers and are hence poorly understood. Furthermore, altitude and aspect are the major topographic factors that control the distribution patterns of vegetation in mountain areas. These factors determine the microclimate and thus the distribution of vegetation in the mountain areas [ 10 ]. The anthropogenic pressures, heavy grazing, and the natural calamities have led to degradation of natural habitats of many species. Such practices are discouraging the moisture loving native species and promoting the hardy nonnative exotic species having little value for the local ecosystem [ 11 ].
The rapid loss in floristic diversity and changing pattern of vegetation due to various biotic and abiotic factors have necessitated the qualitative and quantitative assessment of vegetation. However numbers of studies on community dynamics and phytogeographic affinities have been conducted qualitatively [ 12 – 17 ] as well as quantitatively [ 5 , 18 – 24 ] and in northwest Himalaya [ 4 , 6 , 25 – 27 ] in particular as well. Nevertheless, a very few studies incorporating composition, structural and functional diversity, and nativity of the biodiversity have been carried out in northwest Himalaya [ 28 – 31 ]. But not many studies that give detailed account of floristic diversity of Kinnaur [ 5 ], which forms a typical geographical entity of Himachal Pradesh and Sangla Valley in particular, are available till now. Therefore, this work is emphasized to study floristic composition; to assess the community structure of the vegetation by different phytosociological methods and phytogeographic affinities of the species; and to study the vegetation pattern in the different aspects of the SV along an altitudinal gradient.
2. Materials and Methods
2.1. physiographic features of the study area.
The study area is commonly known as Sangla Valley (hereafter, SV) and situated at 31°31′– 36′N and 77°20′−27′E along the Baspa River that flows through the middle of valley ( Figure 1 ). The valley is oriented from southeast to northwest directions. This is one of the most ecologically fragile biogeographical zones [ 32 ] and inhabited by indigenous tribal communities having Mongolian features and Buddhist religion and culture. It is surrounded by high mountains with elevation ranging from 1800 to 5480 m above mean sea level. The upper ranges of the valley are highly glaciered and receive most of its water through dry precipitation (snow) in winters from November to April. The vegetation of the valley is temperate; subalpine and alpine types and forests are dominated by Pinus wallichiana , Betula utilis , Abies pindrow , and Cedrus deodara communities. The livelihood of communities is based on agrihorticultural activities, which are generally performed after snow melt in April to October. The communities have close affinity with plant resources not only to meet their basic requirements like food, fodder, fuel, health, and shelter but also to perform several religious and cultural rituals. Though the environment of the valley is very close to nature, several anthropogenic activities have successfully altered the natural and traditional agroecosystem of the valley. Several new climatic events such as increasing frequency of rains in July-August (150–200 mm in 1980s to 465 mm in 2012), rising temperature, frost, and fog are being witnessed more frequently than two decades ago.

Map of the study area.
2.2. Sampling Plot and Estimation Design
Representative plots of 50 × 50 m were selected in different aspects and habitats. 10 quadrats of 10 × 10 m for trees, 20 quadrats of 5 × 5 m for shrubs, and 20 quadrats of 1 × 1 m for herbs were randomly laid within the plot. Plots were selected based on different topographical features such as habitat types, altitude, aspects, slope, and different vegetation types ( Table 1 ). The habitats were identified based on the physical characters and dominance of the vegetation. The plots facing high anthropogenic pressure were considered as degraded habitats and sites having closed canopy with high percent of humus and moisture were considered as moist habitat whereas those of low percent of the same were considered as dry habitat. The site having >50% boulders of the ground cover were considered as bouldery habitat. Geographical coordinates of the sites were taken with the help of Global Positioning System (GPS). Slope was measured with the help of Abney's level.
Physical Characteristics of the plots assessed in Sangla Valley.
S. No. | Altitude (m) | Habitat | Slope | Aspect | Latitude | Longitude |
---|---|---|---|---|---|---|
1 | 1950 | Degraded | 35° | S | 31° 28.040 N | 78° 11.209 E |
2 | 2000 | Dry | 60° | NW | 31° 28.853 N | 78° 10.892 E |
3 | 2100 | Dry | 60° | SW | 31° 28.823 N | 78° 10.962 E |
4 | 2250 | Dry | 50° | S | 31° 28.045 N | 78° 11.111 E |
5 | 2550 | Shady Moist | 20° | N | 31° 25.025 N | 78° 16.103 E |
6 | 2625 | Riverine | 50° | NE | 31° 24.854 N | 78° 16.828 E |
7 | 2675 | Bouldery | 40° | N | 31° 25.061 N | 78° 16.368 E |
8 | 2690 | Dry | 15° | NE | 31° 24.913 N | 78° 16.085 E |
9 | 2750 | Shady Moist | 10° | SW | 31° 25.758 N | 78° 16.746 E |
10 | 2770 | Shady Moist | 45° | NE | 31° 24.342 N | 78° 18.038 E |
11 | 3120 | Bouldery | 20° | NE | 31° 23.039 N | 78° 21.614 E |
12 | 3185 | Dry | 30° | SW | 31° 23.631 N | 78° 21.370 E |
13 | 3250 | Dry | 45° | S | 31° 24.342 N | 78° 18.038 E |
14 | 3320 | Dry | 35° | SW | 31° 21.286 N | 78° 24.438 E |
15 | 3340 | Shady Moist | 55° | SW | 31° 23.840 N | 78° 21.340 E |
16 | 3350 | Rocky | 60° | S | 31° 21.756 N | 78° 24.029 E |
17 | 3385 | Dry | 45° | N | 31° 20.992 N | 78° 26.287 E |
18 | 3399 | Dry | 40° | N | 31° 23.640 N | 78° 21.558 E |
19 | 3400 | Shady Moist | 40° | N | 31° 20.955 N | 78° 26.135 E |
20 | 3420 | Shady Moist | 40° | NW | 31° 23.209 N | 78° 25.577 E |
21 | 3450 | Bouldery | 50° | W | 31° 23.881 N | 78° 21.441 E |
22 | 3480 | Shady Moist | 50° | NW | 31° 20.886 N | 78° 26.179 E |
23 | 3500 | Shady Moist | 40° | NW | 31° 23.959 N | 78° 21.554 E |
24 | 3501 | Moraine | 45° | SE | 31° 20.967 N | 78° 27.303 E |
25 | 3516 | Rocky | 40° | SE | 31° 21.002 N | 78° 27.381 E |
26 | 3527 | Moraine | 45° | SE | 31° 21.002 N | 78° 27.374 E |
27 | 3650 | Shady Moist | 40° | N | 31° 20.636 N | 78° 26.224 E |
28 | 3700 | Shady Moist | 40° | N | 31° 20.515 N | 78° 26.267 E |
29 | 3770 | Shady Moist | 35° | NW | 31° 20.389 N | 78° 26.334 E |
30 | 3850 | Shady Moist | 30° | NE | 31° 20.167 N | 78° 26.411 E |
31 | 4129 | Alpine meadow | 45° | NE | 31° 19.310 N | 78° 26.151 E |
32 | 4205 | Alpine meadow | 25° | N | 31° 19.242 N | 78° 26.049 E |
33 | 4330 | Moraine | 30° | NE | 31° 18.831 N | 78° 25.888 E |
34 | 4500 | Alpine meadow | 20° | NE | 31° 18.347 N | 78° 25.648 E |
Sites were selected in each and every aspect between 1950 and 4500 m for the field study and analysis of floristic diversity. In various representative ecoclimatic zones of SV, 34 plots were sampled.
2.3. Data Analysis
The SV, which falls under cold arid zone of Himachal Pradesh, is diverse and rich in species. Communities were identified based on the importance value index and calculated as the sum of relative frequency, relative density, and relative basal area/relative abundance. For diversity index, Shannon-Wiener information index [ 33 ] was used. Species richness was considered as the total number of species in a growth form. For the collection and analysis of data standard ecological methods [ 20 , 24 , 34 – 39 ] were followed. Vegetation was analytically computed following [ 39 , 40 ]. The taxa of Himalayan origin were considered as native and all others as nonnative. During qualitative assessment, rapid surveys and samplings were done in each season and taxa identified on spot and with the help of floras and research papers [ 12 – 14 , 40 – 43 ].
3. Results and Discussion
Plots accessed were 34 in number and a total 15 communities (9 tree, 5 shrub, and 1 herb communities) were delineated based on importance value index for the tree communities and relative density for the shrub and herb communities.
3.1. Vegetational Analysis
The different habitats covered during the study were shady moist, rocky, bouldery, dry, alpine meadow, riverine, moraines, and so forth. Among these, shady moist (12) followed by dry habitats (9) represented maximum sites. Because of unique topography and different altitudinal zones of western Himalaya, vegetation varies from aspect to aspect. The sites were selected in every accessible habitat and aspect along an altitudinal gradient. North and northeastern aspects represented maximum sites (8 each) followed by southwest and northwest (5 each) ( Table 1 ).
Within the communities so delineated, we recorded 320 species of plants belonging to 199 genera and 75 families. Dominant group reported was angiosperms, (68 families, 190 genera, and 302 species); gymnosperms represented by 4 families, 7 genera, and 13 species and pteridophytes by 4 families, 3 genera, and 5 species. They were distributed in different life forms, that is, trees (29 spp.), shrubs (43 spp.), and herbs (248 spp.) ( Table 2 ).
Taxonomic account of floristic diversity.
Taxonomic group | Families | Genera | Species | Herbs | Shrubs | Trees |
---|---|---|---|---|---|---|
Angiosperms | 68 | 190 | 302 | 243 | 39 | 20 |
Gymnosperms | 4 | 7 | 13 | — | 4 | 9 |
Pteridophytes | 3 | 3 | 5 | 5 | — | — |
Total |
Among the angiosperm families, Asteraceae (49 spp.); Rosaceae (21 spp.); Apiaceae (20 spp.); and Ranunculaceae (18 spp.) were dominant. Among genera, Artemisia and Polygonum (7 spp.); Saussurea (6 spp.); Berberis and Thalictrum (5 spp.), and Geranium , Juniperus , Nepeta , Potentilla , Poa , Rosa , and Salix (4 spp. each) were dominant genera. Twenty-eight (28) families were monotypic and represented only by one species. The occurrence of 320 species in the quantified area indicates that its environmental conditions, particularly shady moist and forest habitats, are suitable for the growth and development of species. Amongst the communities, P. wallichiana community represented maximum sites (6 sites), followed by B. utilis (5 sites); B. utilis - P. wallichiana mixed (4 sites); C. deodara and P. gerardiana (3 sites, each); R. anthopogon , H. salicifolia , and R. anthopogon-S. caliculata mixed (2 sites, each), and the rest of the communities were represented by one site only. Dominance of the Asteraceae in SV is also validated by floras of Lahaul-Spiti, Himachal Pradesh, in high altitude regions of western Himalaya [ 13 , 42 ]. The affinity of vegetation towards the flora of the Lahaul-Spiti Valley and Bhaba Valley [ 5 , 42 ] is apparent by the presence of similar dominant families. Moreover, the major part of the valley is covered with snow throughout the year. Presence of number of herbaceous families (namely, Apiaceae, Brassicaceae, Ranunculaceae, Rosaceae, Polygonaceae, and Scrophulariaceae) is attributed to the temperate and alpine nature of the area. Lesser Pteridophytes in the area may be attributed to the more exposed arid nature of the valley with low broad leaf forest cover and moisture. Nonetheless, as a whole, the high diversity and richness of the species in the SV indicate the high conservation value of the area. Occurrences of 320 species in the 15 identified communities of 34 quantified plots validate this.
3.2. Communities: Composition and Structure
Total tree density ranged from 205 to 600 no./ha (number per hectare) and total basal area from 8.70 to 42.41 m 2 /ha. Shrubs and herbs densities ranged from 105.0 to 1030.00 no./ha and from 22.08 to 48.73 no./m 2 , respectively. Shrub density is maximum in C. deodara-P. smithiana mixed community and herbs density is maximum in Poa alpina-Agrostis stolonifera-Bistorta affinis-Aconitum violaceum community. Among five major shrub communities, Spiraea canescens-Lonicera hypoleuca mixed community has highest shrub and herb density, that is, 540.00 no./ha and 48.73 no./m 2 , respectively ( Table 3 ). Tree density range is comparable to the other Himalayan studies [ 40 , 44 ] and European temperate forests [ 45 ]. Similarly, shrub and herb's density ranges (105–1030 no./ha and 22.08–78.95 no./m 2 , resp.) are in compliance with the earlier studies of the Himalayan regions [ 46 ]. However the lower range of shrub densities in R. anthopogon communities (105 no./ha and 230 no./ha) in the valley is due to the presence of its scanty patches near the subalpine areas and often the rugged and arid and moraine topography.
Identified communities showing TBA, species richness, densities, and diversity in Sangla Valley.
Communities | SR | TBA (m /ha) | Species richness | Density | Species diversity index ( ′) | ||||
---|---|---|---|---|---|---|---|---|---|
Trees (no./ha) | Shrubs (no./ha) | Herbs (no./m ) | Trees | Shrubs | Herbs | ||||
Trees | |||||||||
BU | 5 | 11.17 | 47 | 480 | 330 | 35.16 | 0.73 | 2.12 | 3.17 |
CD | 3 | 42.41 | 38 | 403.33 | 673.33 | 33.44 | 0.57 | 1.68 | 3.62 |
QF | 1 | 14.17 | 19 | 340 | 290 | 22.08 | 0 | 0.4 | 2.49 |
PG | 3 | 12.09 | 47 | 490 | 606.29 | 35.20 | 0.82 | 1.16 | 3.11 |
PW | 6 | 8.702 | 96 | 205 | 625.83 | 36.34 | 0 | 2.38 | 4.01 |
BU-AP | 1 | 14.16 | 35 | 440 | 770 | 25.03 | 1.02 | 1.52 | 3.16 |
BU-PW | 4 | 12.28 | 80 | 420 | 327.5 | 40.36 | 0.93 | 2.22 | 3.69 |
CD-PS | 1 | 17.98 | 41 | 600 | 1030 | 42.40 | 1.28 | 2.07 | 2.98 |
CD-PW | 1 | 21.99 | 29 | 400 | 420 | 38.20 | 0.69 | 0.95 | 3.07 |
Shrubs | |||||||||
HS | 2 | — | 41 | — | 370 | 45.39 | — | 1.63 | 3.21 |
RA | 2 | — | 40 | — | 105 | 32.56 | — | 0.96 | 3.45 |
RA-SC | 2 | — | 52 | — | 230 | 34.80 | — | 1.15 | 3.55 |
SCa-CB-BJ | 1 | — | 34 | — | 540 | 48.73 | — | 1.53 | 2.87 |
SCa-LH | 1 | — | 33 | — | 630 | 27.70 | — | 1.43 | 3.1 |
Herbs | |||||||||
PA-AS-BA-AV | 1 | — | 34 | — | — | 78.95 | — | — | 2.91 |
SR = site represented, TBA: total basal area, Ind = individual, BU = Betula utilis , CD = Cedrus deodara , QF = Quercus floribunda , PG = Pinus gerardiana , PW = Pinus wallichiana , BU-AP = Betula utilis-Abies pindrow mixed, BU-PW = Betula utilis-Pinus wallichiana mixed, CD-PS = Cedrus deodara-Picea smithiana , CD-PW = Cedrus deodara-Pinus wallichiana mixed, HS = Hippophae salicifolia , RA = Rhododendron anthopogon , RA-SC = Rhododendron anthopogon-Salix caliculata mixed, SCa-CB-BL = Spiraea canescens-Cotoneaster bacillaris-Berberis jaeschkeana mixed, SCa-LH = Spiraea canescens-Lonicera hypoleuca mixed, and PA-AS-BA-AV = Poa alpina-Agrostis stolonifera-Bistorta affinis-Aconitum violaceum .
3.3. Species Richness and Diversity Index (H′)
Species richness in identified communities ranged from 19 to 96. Among the communities, it was highest in P. wallichiana (96 spp.), followed by B. utilis-P. wallichiana mixed (80 spp.), R. anthopogon-S. caliculata mixed (52 spp.), and P. gerardiana (47 spp.) communities. Species diversity index for trees is maximum (1.28) for C. deodara-P. smithiana mixed community and minimum (0.0) for Q. floribunda and P. wallichiana communities; among shrubs it is maximum (2.38) for P. wallichiana community and minimum (0.40) for Q. floribunda community and for herbs it is maximum (4.01) for P. wallichiana community and minimum (2.49) for Q. floribunda community ( Table 3 ).
The species richness (19–96) in the communities was higher than the earlier reported values [ 47 , 48 ] but comparable to the [ 24 , 49 ] from high altitude areas of Himalaya. The high richness of trees and shrubs may be due to diverse habitats and suitable edaphic and climatic factors supporting growth and survival of the species. The diversity index for trees (0.0–1.28) is within the reported value from the other Himalayan areas [ 40 , 47 , 49 , 50 ]. In P. gerardiana community, it is the only tree species which is present, so its diversity index value is zero. The diversity of shrubs (0.40–2.38) is comparable to the previous records from the higher Himalaya and also from the lower parts (0.51–1.33) [ 46 , 48 , 50 ] and (0.74–3.14) reported by [ 40 , 51 ] for subtropical and temperate forests. However for herbs diversity (2.49–4.01) it was higher than earlier records. The value of the total basal area was found to be maximum in C. deodara and C. deodara-P. wallichiana mixed communities (42.41 and 21.99 m 2 /ha, resp.) and average basal area is 17.23 m 2 /ha which is very low as compared to the other parts of the Himalaya where it is above 70 m 2 /ha [ 52 – 54 ]. This may be due to unscrupulous tree felling and logging pressure on the forest resources in addition to the other natural causes like heavy erratic rainfalls which leads to the massive landslides in the region.
3.4. Species Dominance in the Identified Communities
Among the various communities identified in SV, we figured out the maximum values of dominant species as 74.5% ( B. utilis ) and 19.9% ( P. wallichiana ) in B. utilis community; 80.2% ( C. deodara ) and 17.4% ( P. wallichiana ) in C. deodara community; 100% ( Q. floribunda ) in Q. floribunda community as it was the only tree species present in the community; 57.14% ( Pinus gerardiana ) and 38.8%( Quercus floribunda ) in Pinus gerardiana community; 90.9% ( P. wallichiana ) and 8.1% ( C. deodara ) in P. wallichiana community; 43.1% ( B. utilis ) and 40.9% ( A. pindrow ) in B. utilis - A. pindrow mixed community; 62.5% ( B. utilis ) and 29.8% ( P. wallichiana )in B. utilis-P. wallichiana mixed community; 38.3% ( C. deodara ) and 31.6% ( Picea smithiana ) in C. deodara-P. smithiana mixed community; and 52.5% ( C. deodara ) and 42.5% ( P. wallichiana ) in C. deodara-P. wallichiana mixed community ( Table 4 ).
Relative densities of dominant species in various communities.
Communities | Dominant elements | ||
---|---|---|---|
Trees | Shrubs | Herbs | |
D. Don | D. Don (74.5%), A. B. Jacks. (19.9%), and (Royle ex D. Don) Royle (5.0%) | (Wall.) D. Don (28.5%), D. Don (17.6%), and Wall. ex Royle (9.7%) | (L.) All. (11.4%), D. Don ex Sweet (5.7%), (Hook. f.) Lindl. ex Lacaita (5.6%), and (D. Don) H. Hara (5.4%) |
(Roxb. ex Lamb.) G. Don | (Roxb. ex Lamb.) G. Don (80.2%), A. B. Jacks. (17.4%), and (Royle ex D. Don) Royle (2.5%) | (Wall. ex Benth.) H. Hara (31.3%), (29.8%), and Wall. ex Lindl. (7.5%) | Wall. (11.1%) (7.4%), and (Royle ex Benth.) Benth. (7.4%) |
Lindl. ex A. Camus | Lindl. ex A. Camus (100%) | (Wall. ex Benth.) H. Hara (80.1%), (Lindl.) Rehder (9.1%) | L. (14.3%) (L.) Mosyakin & Clemants (10.9%), and L. (9.5%) |
Wall. ex D. Don | Wall. ex D. Don (57.14%), Lindl. ex A. Camus (38.8%), and L. (4.1%) | (Wall. ex Benth.) H. Hara (55.9%), DC. (18.9%), and L. (13.2%) | (S. F. Blake) Pruski & G. Sancho (9.4%), L. (6.8%), and L. (5.4%) |
A. B. Jacks. | A. B. Jacks. (90.9%), (Roxb. ex Lamb.) G. Don (8.1%) | Lindl. (17.4%), C. K. Schneid. (14.4%), and Wall. ex Lindl. (12.8%) | L. (6.1%), Wall. (3.6%), and L. (3.3%) |
D. Don Royle ex D. Don) Royle mixed | D. Don (43.1%), (Royle ex D. Don) Royle (40.9%),and A. B. Jacks. (15.9%) | Wall. ex Lindl. (32.5%), Lindl. (22.0%), and C. K. Schneid. (11.7%) | ( ) (Hook. f.) Lindl. ex Lacaita (18.2%), Benth., and L. (4.6% each) |
D. Don A. B. Jacks. mixed | D. Don (62.5%), A. B. Jacks. (29.8%), and (Royle ex D. Don) Royle (4.3%) | (Wall.) D. Don (33.3%), D. Don (10.0%), and D. Don (7.6%) | (L.) (6.8%), Royle (4.5%), and (Wulfen) Rchb. (3.5%) |
(Roxb. ex Lamb.) G. Don- (Wall.) Boiss. mixed | (Roxb. ex Lamb.) G. Don (38.3%), (Wall.) Boiss. (31.6%), A. B. Jacks. (20.0%), and L. (10.3) | Sm. (15.5%), DC. (14.6%), and DC. (13.6%) | (Hook. f.) Lindl. ex Lacaita (16.1%), L. (5.2%), Wall., and Wall. & DC. (3.8% each) |
(Roxb. ex Lamb.) G. Don A. B. Jacks. mixed | (Roxb. ex Lamb.) G. Don (52.5%), A. B. Jacks. (42.5%), and (Wall.) Boiss. (5.0%) | C. K. Schneid. (59.52%), D. Don (21.4%), Wall. ex Royle (19.0) | (Hook. f.) Lindl. ex Lacaita (8.2%), Benth. (6.3%), and L. (5.5%) |
D. Don | — | D. Don (58%), (Lindl.) Rehder (13.2%), and (Wall. ex Benth.) H. Hara (9.4%) | (Hook. f.) Lindl. ex Lacaita (15.6%), L. (8.3%) L. (6.0%), and (Royle ex Benth.) Benth. (4.5%) |
D. Don | — | D. Don (57.2%), L. (28.6%), and Wall. ex Lindl. (14.3%) | Royle (7.2%), (D. Don) S. H. Fu (5.7%), (D. Don) Greene (5.3%) (Royle) Hook. f. (4.8%), and Hook. f. (4.2%) |
D. Don Hook. f. ex Andersson mixed | — | D. Don (45.65%), Hook. f. ex Andersson (36.9%), and D. Don (8.7%) | (Hook. f.) Lindl. ex Lacaita (19.5%), (D. Don) Greene (10.7%), (Sims) Sims ex C. B. Clarke (6.7%), L. (6.2%), Wall. (5.7%), and (L.) Hill (5.3%) |
D. Don Wall. ex Lindl.- C. K. Schneid. mixed | — | D. Don (27.0%), Wall. ex Lindl. (11.5%), and C. K. Schneid. (8.2%) | (Benth.) Benth. (17.2%), (Hook. f.) Lindl. ex Lacaita (15.4%), L. (7.7%), L. (7.4%), and Hook. f. (3.5%) |
D. Don Decne. Mixed | — | D. Don. (44.4%), Decne (19.0%), and C. K. Schneid. (11.1%) | Thunb. (10.5%), Roxb. (9.6%), (D. Don) H. Hara (7.2%), and (Hook. f.) Lindl. ex Lacaita (6.3%) |
L.- L.- (D. Don) Greene Jacquem. ex Stapf mixed | — | — | L. (25.33%), L. (13.2%), (D. Don) Greene (4.6%), Jacquem. ex Stapf (4.6%), and C. B. Clarke (4.3%) |
Among shrubs maximum density percentages were of the species Berberis aristata , Berberis jaeschkeana , Cassiope fastigiata , Cotoneaster bacillaris , Cotoneaster microphyllus , Desmodium elegans , Hippophae salicifolia , Lonicera hypoleuca , Rhododendron campanulatum , Rabdosia rugosa , Rhododendron anthopogon , Rubus ellipticus , Salix calyculata , Sorbaria tomentosa , Spiraea canescens , and so forth. Rabdosia rugosa (80.1%) and Cotoneaster bacillaris (7.5%) were having maximum and minimum density percentages in Quercus floribunda and Cedrus deodara communities, respectively. Among herbs assessed Aconogonum molle , Agrostis stolonifera , Bistorta affinis , Bromus japonicas , Cannabis sativa , Conyza sumatrensis , Cynoglossum wallichii , Delphinium cashmerianum , Euphrasia officinalis , Fragaria nubicola , Impatiens thomsonii , Morina longifolia , Nepeta erecta , Origanum vulgare , Persicaria vivipara , Poa alpina , Polygonatum verticillatum , Thalictrum cultratum , Trifolium pratense , and so forth were in abundance, hence showing comparatively more densities. Herbs having maximum density recorded were Poa alpina (25.3%) followed by Cynoglossum wallichii (23.4%) and Fragaria nubicola (19.5%) in Poa alpina-Agrostis stolonifera-Bistorta affinis-Aconitum violaceum mixed, B. utilis-A. pindrow mixed, and Rhododendron anthopogon-Salix calyculata mixed communities, respectively ( Table 4 ).
Tree, shrub, and herb percentage covers within the communities showed a typical composition of the Himalayan region wherein shrubs Rabdosia rugosa and Cotoneaster bacillaris were having maximum and minimum density percentages, respectively, and herbs Poa alpina, Cynoglossum wallichii ,and Fragaria nubicola were abundant. Dominance of these species might be due to their high adaptability in addition to their good capability to proliferate in the extreme climatic conditions of this part of western Himalaya.
3.5. Phytogeographic Affinities
As a whole in all the communities, 182 species were native to the Himalayan region and the remaining were nonnatives as they are from different biogeographic domains of the world. The trend of nativity of plants occurring in SV was as follows: European/Oriental region (28) > Asia (25) > European region (16) > Temperate region (13) > Indian region (10) > India/Oriental region (8) > America (7) > European/Oriental/African and Temperate, Arctic (6 each) > Cosmopolitan (5) > Australian (4) > Amphigean (3) > Arctic, European/Oriental/American and Oriental (2 each) and European/African (1) ( Figure 2 ).

Species showing their biogeographic realms. Afr = Africa; Amer = America; Amphig = Amphigean; Arct = Arctic; As = Asia; Aust = Australia; Cosmo = Cosmopolitan; Euro = Europe; Himal = Himalaya; Ind = India; Orient = Oriental; and Temp = Temperate.
Natives are the species which evolved naturally in a particular region before their human introduction. To prioritize a species or habitat of the region for conservation, status of a species as to whether it is native or introduced in a given area is required. Species invasions beyond their native range constitute a global driver of change as nonnative species threaten biodiversity and change ecosystem functioning [ 55 ]. Like in other parts of the Himachal Himalaya [ 29 , 30 ] in SV also the percentage of native species increased with the altitude. There is a strong evidence from a scatter diagram that a positive linear relationship exists between the native species richness and altitude ( r = 0.83, P < 0.01, n = 34) ( Figure 3 ). The high percentage of the native species at higher elevations may be due to low anthropogenic pressure and severe climatic conditions compared to the lower elevations where high anthropogenic pressure and mild climatic conditions support the speciation of the nonnative species [ 30 ]. Regular monitoring of the habitats and populations of the native species facing high anthropogenic pressure even in higher altitude is essentially required, so that the adequate planning for their conservation and management could be done in time.

Native species along the altitude gradient.
3.6. Altitudinal and Aspectwise Distribution of Species
Altitude and aspect are the most important determinants of vegetation distribution due to their direct impact on microclimate of the habitat [ 56 , 57 ]. The Himalayan region has typical topography and environment where biodiversity varies from aspect to aspect and habitats of the communities [ 58 ].
Maximum altitudinal distribution of few selected climate sensitive species, namely, Bistorta affinis , Fragaria nubicola , Geranium pratense , Pleurospermum candollei , Podophyllum hexandrum , Rhodiola heterodonta , Saussurea obvallata , Saxifraga sibrica , and Sedum ewersii , was studied in the valley. It was found to be highest in northeast followed by north, south, and southeast aspects ( Figure 4 ). Species like Bistorta affinis reaching up to 4510 m and 3890 m in northeast and north aspects, respectively, are restricted to 3580 m and 3429 m in south and southeast aspects, resepectively. Similar trend was seen with all other climate sesitive species in the region.

Distribution range of species along altitudinal gradient in different aspects.
P. wallichiana showed the broadest range from 2100 to 3500 m and almost reaching the tree line along with B. utilis .
In this valley, northern and northeastern slopes have lower temperatures and higher soil and air moisture contents as compared to southern and other slopes at the same altitude due to less solar exposure and higher moisture content and evapotranspiration which is akin to the other Himalayan areas [ 59 , 60 ]. In northern and northeastern slopes B. utilis, A. pindrow , and P. wallichiana were recorded at the altitude as low as 2200 m, whereas on the south and southeastern aspects their altitudinal range started from 2300 m.
3.7. Final Considerations
In northwestern Himalaya the high mountain plant communities support a rich biodiversity in terms of ecological indicator species and natives. They need a proper management against harsh climate and anthropogenic pressure for continued future sustainability. Regular monitoring using random sampling by quadrat method is suggested to understand the dynamics of the habitats and communities and accordingly plan for their management. The climate sensitive species are required to be regularly monitored for their phenological attributes so that the baseline data can be generated for future changes in the area. The information generated on these lines will provide a better insight about the present status of floristic diversity and help in developing adequate strategies and action plan for the management of such biodiversity-rich areas. The state and central government agencies are suggested to encourage the native species so that the ambient regional ecosystems are protected for the posterity. Further, for in situ conservation of the economically and ecologically important species, regular monitoring of the sites and complete protection of the habitats is suggested. In addition, seed germination protocols developed may be used for mass multiplication of the species and seedlings should be transplanted in comparable habitats so that viable population of the species can be maintained. Finally a pragmatic and ameliorative conservational approach which was hitherto absent in this part of the Himalaya needs to be implemented.
Acknowledgments
Thanks are due to Department of Science and Technology, Government of India, for providing funds to conduct the study. The authors also thank and appreciate local communities for their cooperation and patience in providing the information and hospitality while undertaking surveys.
Conflict of Interests
The authors declare that there is no conflict of interests regarding the publication of this paper.
A bibliometric analysis of research on fish and floristic diversity: Trends and themes
- August 2024
- Journal of Fisheries 12(2):122301
- 12(2):122301
- CC BY-NC-SA 4.0
- This person is not on ResearchGate, or hasn't claimed this research yet.
Discover the world's research
- 25+ million members
- 160+ million publication pages
- 2.3+ billion citations

- Anup R. Joshi
- Nathan R. Hahn
- Andrew Zolli
- Nat. Ecol. Evol.

- David Tickner
- Steve Carpenter

- Katherine Abernathy

- Dongmei Luo
- Chenchen Jia

- Charles A. Wainright

- James J. Elser

- Recruit researchers
- Join for free
- Login Email Tip: Most researchers use their institutional email address as their ResearchGate login Password Forgot password? Keep me logged in Log in or Continue with Google Welcome back! Please log in. Email · Hint Tip: Most researchers use their institutional email address as their ResearchGate login Password Forgot password? Keep me logged in Log in or Continue with Google No account? Sign up
Floristic Diversity of India: An Overview
- First Online: 27 February 2020
Cite this chapter
- Paramjit Singh 4
Part of the book series: Topics in Biodiversity and Conservation ((TOBC,volume 18))
694 Accesses
8 Citations
The present chapter gives a synthesis of the floristic diversity of India, dwelling briefly on its ecosystem diversity, vegetation pattern, species richness in various taxonomic groups traditionally dealt with as plants (angiosperms, gymnosperms, pteridophytes, bryophytes, algae, fungi and lichens), endemism and primitive angiosperms and phytogeographical analysis of the flora. It is estimated that out of 49,003 species of plants forming the evident vegetal cover, angiosperms comprise ca. 18,532 species, representing ca. 10% of all known flowering plants of the world. The largest angiosperm family in the number of species is Leguminosae (with 1421 spp.), followed by Poaceae (1291spp.), Orchidaceae (1251 spp.), Asteraceae (1120 spp.) and Rubiaceae (679 spp.). The largest genus is Impatiens (with 279 spp.), followed by Carex (160 spp.), Pedicularis (145 spp.), Bulbophyllum and Primula (135 spp. each). Existing estimate pertaining to the political boundaries of present-day India is that about 4300 (23.20%) of 18,532 flowering plant species are endemic to this country.
This is a preview of subscription content, log in via an institution to check access.
Access this chapter
Subscribe and save.
- Get 10 units per month
- Download Article/Chapter or eBook
- 1 Unit = 1 Article or 1 Chapter
- Cancel anytime
- Available as PDF
- Read on any device
- Instant download
- Own it forever
- Available as EPUB and PDF
- Compact, lightweight edition
- Dispatched in 3 to 5 business days
- Free shipping worldwide - see info
- Durable hardcover edition
Tax calculation will be finalised at checkout
Purchases are for personal use only
Institutional subscriptions
Similar content being viewed by others
Status, Issues and Challenges of Biodiversity: Higher Plants
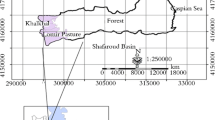
Study of plant diversity and floristics in the westernmost Hyrcanian forests
Flora of the Pantanal
Ahmedullah M (2000) Endemism in the Indian Flora. In: Singh NP, Singh DK, Hajra PK, Sharma BD (eds) Flora of India, Introductory Volume 1. Part 2. Botanical Survey of India, Calcutta, pp 246–265
Google Scholar
APG IV (2016) An update of the Angiosperm Phylogeny Group classification for the orders and families of flowering plants: APG IV. Bot J Linn Soc 181:1–20
Article Google Scholar
Arora RK (1960) The botany of Coorg forests. Proc Natl Acad Sci 50(B):289–305
Arora RK (1964) Phytogeographic notes on the humid tropics flora of India - World distribution and analysis of the woody dicotyledonous flora of Western Ghats and Assam. J Indian Bot Soc 43:220–228
Balakrishnan NP (1977) Floristic studies in Andaman & Nicobar Island. Bull Bot Surv India 19:127–137
Balakrishnan NP (1988) Andaman Islands – vegetation and floristics. In: Saldanha CJ (ed) Andaman, Nicobar and Lakshadweep – an environmental impact assessment. Oxford & IBH Publishing Co, New Delhi, pp 55–68
Balakrishnan NP (1996) Phytogeographic division: general considerations. In Hajra PK et al (ed) Flora of India (Intro) 1:197–204
Brummit RK (1992) Vascular plant families and genera. Royal Botanic Gardens, Kew
Burkill IH (1924–1925) The botany of Abor expeditions. Rec Bot Surv India 10(1):1–154. 1924 & 10(2):155–420. 1925. T.t.1–10. 1925
Champion HG, Seth SK (1968) A revised survey of the forest types of India. Manager of Publications, New Delhi
Chatterjee D (1940) Studies on the endemic flora of India and Burma. J Asiat Soc Bengal 5:19–67
Chatterjee D (1962) Floristic patterns on Indian vegetation. Proc Summer School Bot Darjeeling 1960:32–42
Clarke CB (1898) Sub-subareas of British Empire, illustrated by the detailed distribution of Cyperaceae in that empire. J Linn Soc Lond 34:1–146
Conservation International fact sheets (2005) Biodiversity hotspots. www.conservation.org
Dash SS, Singh P (2018) Flora of Kurung Kumey District, Arunachal Pradesh, India. Botanical Survey of India, Kolkata
Diels L (1918) Pflanzengeographie, 2 Aufl, Berlin
Engler A (1882) Versnch einer Entwichlungsgeschichite der Planzenwelt, insbesondere der Florenebiete, seit der Tertiarperiode. I–II, Leipzig
Engler A (1924) Ubersicht uber die Florenrciche and Florengebiete der Erde. In: Engler A, Gilg (eds) Syllabus der Pflanzenfamilien . Aufl. Berlin, pp 9–10
FSI 2011. Forest Survey of India, Dehradun. http://www. fsi.org.in /sfr_ 2011/htm
Gadgil M, Meher-Homji VM (1990) Ecological Diversity. In: Daniel JC (ed) Developing countries: problems and prospects. Bombay Natural History Society, Oxford University Press, Delhi, pp 175–198
Good R (1947) The geography of the flowering plants, 1st edn. Longman, London
Good R (1974) The geography of the flowering plants, 4th edn. Longman, London
Gupta P (2012a) Algae of India- a checklist of Cyanoprokaryota (Cyanophyceae), vol 1. Botanical Survey of India, Kolkata
Gupta RK (2012b) Algae of India: Chlorophyceae, Xanthophyceae, Chrysophyceae and Euglenophyceae – a checklist, vol 2. Botanical Survey of India, Kolkata
Hajra PK (1982) A contribution to the botany of Nanda Devi National Park . Botanical Survey of India, Calcutta
Hajra PK, Rao RR (1990) Distribution of vegetation types in northwest Himalaya with brief remarks on phytogeography and floral resource conservation. Proc Indian Acad Sci 100:263–277
Hajra PK, Shukla U (1982) Dudhwa National Park. Botanical Survey of India, Calcutta
Hooker JD 1904 (1907) A sketch of the flora of British India. Routledge, London
Irwin JS, Narasimhan D (2011) Endemic genera of angiosperms in India: a review. Rheeda 21(1):87–105
Jain SK (1982) Botany of the Eastern Himalayas. In: Pailiwal GS (ed) Vegetation wealth of Himalayas, pp 201–217
Jain SK, Sastry ARK (1978) Plant resources of the Himalayas. In: Proceedings of the national seminar on research development of environmental of Himalayan, pp 98–107. Department of Science & Technology, New Delhi
Mabberley DJ (2008) Mabberley's Plant-book: A Portable Dictionary of Plants, Their Classifications and Uses. Cambridge University Press, London
Mani MS (1974) Biogeographical evolution in India: ecology and biogeography in India , Mani MS (ed), Netherlands
Meher-Homji VM (1965) On the Sudan-Deccanian floral element J . Bombay Nat Hist Soc 62(1):15–18
Meher-Homji VM (1973) Phytogeography of the Indian subcontinent. Progr Plant Ecol 1:9–88
Melville R (1973) Continental drift and the distribution of Island floras of the Indian Ocean. J Marine Biol Assos India 15(1):236–241
Mitra S, Mukherjee SK (2007) Reassessment and diversity of endemic angiospermic genera of India. J Econ Taxon Bot 31:163–176
Mittermeier RA, Gil PR, Hoffmann M, Pilgrim J, Brooks T, Mittermeier CG, Lamoreux J, da Fonseca GAB (2004) Hotspots revisited: Earth’s biologically richest and most endangered terrestrial ecoregions. CEMEX, Mexico
Myers N (1990) The biodiversity challenge: Expanded hot-spots analysis. Environmentalist 10:243–256
Article CAS PubMed Google Scholar
Myers N, Mittermier RA, Mittermier CG, da Fonseca GAB, Kent J (2000) Biodiversity hotspots for conservation priorities. Nature 403:853–858
Nair NC, Daniel P (1986) The floristic diversity of the Western Ghats and its conservation. Proc Indian Acad Sci (Anim & Pl Sci), Suppl:127–163
Nair NC, Vajravelu E, Bhargavan P (1980) Preliminary Report on the Botany of Silent Valley (Mimeogr.). BSI, Coimbatore
Nayar MP (1980) Endemic flora of Peninsular India and its significance. Bull Bot Surv India 22:12–33
Nayar MP (1996) Hot Spots of Endemic Plants of India, Nepal and Bhutan. Tropical Botanic Garden and Research Institute, Thiruvananthapuram
Polhill RM, Raven PH (eds) (1981) Advances in legume systematics. Royal Botanic Gardens, Kew
Rao AS (1974) The vegetation and phytogeography of Assam – Burma. In: Mani MS (ed) Ecology & biogeopgraphy of India. W. Junk Publishers, The Hague, pp 204–246
Chapter Google Scholar
Rao RR, Murti SK (1990) Northeast India. A major centre for plant diversity in India. Indian J For 13:214–222
Rau MA (1974) Vegetation and phytogeography of the Himalayas. In: Mani MS (ed) Ecology & biogeography of India. W. Junk Publishers, The Hague, pp 247–280
Renovize SA (1979) The origin of Indian Ocean Island floras. In: Bramwell D (ed) Plants and Islands. Academic, London, pp 107–129
Rodgers WA, Panwar HS (1990) A biogeographical classification for conservation planning. Wildlife Institute of India, Dehradun
Rodgers WA, Panwar HS, Mathur VB (2000) Biogeographical classification of India. In: Wildlife protected area network in India: a review (executive summary). Wildlife Institute of India, Dehradun. 49pp
Sagareiya KP (1969) Forests and forestry. National Book Trust, New Delhi
Sahni KC (1969) A contribution to the flora of Kameng and Subansiri district, NEFA. Indian Forester 95(5):330–352
Sanjappa M (1991) Legumes of India. Bishen Singh Mahendra Pal Singh, Dehradun
Sanjappa M (2001) Leguminosae. In: Singh NP, Singh DK (eds) Floristic diversity and conservation strategies in India. Botanical Survey of India, Howrah, pp 1847–1902
Sarkar AK (1995) Endemic genera of angiosperms and their species in India. In Gupta SK (ed) Higher plants of Indian subcontinent, vol 1 . Indian J Forest Addit Ser IV . Dehradun, pp 235–257
Schouw JF (1823) Gundzuge Einer Allgemeinen Pflanzengeographie , Berlin
Sharma JR (2012) Aphyllophorales of Himalaya. Botanical Survey of India, Kolkata
Singh P, Dash SS (eds) (2018) Plant discoveries 2017. Botanical Survey of India, Kolkata
Singh KP, Sinha GP (2010) Indian lichens: an annotated checklist. Botanical Survey of India, Kolkata
Singh, P, K. Karthigeyan, P. Lakshminarasimhan & S.S. Dash 2015. Endemic vascular plants of India. Botanical Survey of India, Kolkata
Smith WW, Cave GH (1911) The vegetation of Zemu & Llonakh valleys of Sikkim. Rec Bot Sur India 4:141.157
Subramanyam K, Nayar MP (1974) Vegetation and phytogeography of Western Ghats. In: Mani MS (ed) Ecology & biogeography of India, pp 178–196
Takhtajan A (1969) Flowering plants: origin and dispersal. Oliver and Boyd, Edinburgh [Translated from Russian by C Jeffrey]
Takhtajan A (1986) Floristic regions of the world. University of California Press, Berkeley
Thothathri K, Banerjee SP, Mukherjee PK, Hajra PK, Pal GD (1975) Botanical studies of the joint scientific expedition to Great Nicobar Islands. Bull Bot Surv India 15:235–265
Turrill WB (1953) Pioneer plant geography. M. Nijhoff, The Hague
Turrill WB (1959) Plant geography. In: Rollins RC, Taylor G (eds) Vistas in botany, vol 2. Pergamon Press, London, pp 172–228
Download references
Acknowledgements
A review paper encompassing such an extensive canvas of literature could not have been possible without help from within Botanical Survey of India and various universities and institutes. I thank the staff of Botanical Survey of India, particularly different subject experts, for helping in collating the information on different groups of plants.
Author information
Authors and affiliations.
Botanical Survey of India, Kolkata, India
Paramjit Singh
You can also search for this author in PubMed Google Scholar
Editor information
Editors and affiliations.
Department of Botany, University of Kashmir, Srinagar, Jammu and Kashmir, India
Ghulam Hassan Dar
Centre for Biodiversity and Taxonomy, Department of Botany, University of Kashmir, Srinagar, Jammu and Kashmir, India
Anzar A. Khuroo
Rights and permissions
Reprints and permissions
Copyright information
© 2020 Springer Nature Singapore Pte Ltd.
About this chapter
Singh, P. (2020). Floristic Diversity of India: An Overview. In: Dar, G., Khuroo, A. (eds) Biodiversity of the Himalaya: Jammu and Kashmir State . Topics in Biodiversity and Conservation, vol 18. Springer, Singapore. https://doi.org/10.1007/978-981-32-9174-4_3
Download citation
DOI : https://doi.org/10.1007/978-981-32-9174-4_3
Published : 27 February 2020
Publisher Name : Springer, Singapore
Print ISBN : 978-981-32-9173-7
Online ISBN : 978-981-32-9174-4
eBook Packages : Biomedical and Life Sciences Biomedical and Life Sciences (R0)
Share this chapter
Anyone you share the following link with will be able to read this content:
Sorry, a shareable link is not currently available for this article.
Provided by the Springer Nature SharedIt content-sharing initiative
- Publish with us
Policies and ethics
- Find a journal
- Track your research

IMAGES
VIDEO
COMMENTS
global diversity hotspots (Stebbins and Major 1965, Cole 1979, Huston 1994, Cowling et al. 1996, Myers et al. 2000, Dirzo and Raven 2003). The California Floristic Province contains over 50 endemic genera of vascular plants, while by comparison the Rocky Mountain Floristic Province of North America contains only 5 endemic genera (Flora of
Human-driven biotic homogenization and the erosion of biological diversity are widely reported in the scientific literature 1,2, as articulated by the Intergovernmental Science-Policy Platform on ...
Introduction. Floristic diversity is an important element of ecosystems (Hua et al., 2022).This diversity includes the plant species diversity of a particular area representing the local flora of a given area (Qian et al., 2021).Taxonomy is concerned with their identification and classification of species (Barkley et al., 2004).Floristic and taxonomic studies provide efficient information ...
Studying the floristic diversity of a certain forest is a basic aspect of the design and management of forest vegetation; and consequently this study focused on the plant diversity and community analysis of the Sele-Nono forest. For the current study, plants were sampled from 90 plots using a stratified random sampling technique along the established strata of the study forest.
Measures that include phylogenetic diversity can provide a foundation for assessing hypotheses about ecological processes driving the patterns we observe. In this dissertation, I explore how incorporating the evolutionary history of coexisting species can help to disentangle patterns of diversity at macro-ecological scales.
Master Thesis Date of Defense: November 19, 2013 Committee Members: David A Mortensen, Thesis Advisor/Co-Advisor Keywords: pollinators wild bees ... Therefore, I documented floristic diversity in orchard, forest, and forest edge habitats in an apple (Malus domestica Borkh.) production region in south-central Pennsylvania, USA. I tested the ...
The floristic diversity of tropical Africa Botanical records across tropical Africa are well distributed but highly heterogeneous (Fig. 1a ). Continental or regional scale biodiversity data are typically distributed in an uneven and patchy way [ 20 , 24 , 40 ], related to differences in accessibility (presence of roads or rivers), focus of ...
Comparison between the 1894 and 2018 floristic data 18,19 clearly demonstrated the impact of climatic and urban changes on floristic diversity and assemblage in Bologna's historical city centre.
Himalayas Pakistan, PhD thesis Department of . Biological Sciences/ Quaid-I- University, Islama bad. ... The floristic diversity revealed 228 plant species belonged to 184 genera and 72 families.
Floristic Composition and Diversity The vascular plant species composition, abundance, and diversity of the study districts of the remnant vegetation in Arsi-Bale Massifs were documented. There were 382 species of vascular plants belonging to 223 genera and 92 families (Table 1 ) within the altitudinal ranges between 2,318 and 3,882 m.a.s.l.
These environmental conditions provide considerable scope for diversity in the floristic composition, adaptive characteristics displayed by the plants, patterns of groupings of plant species and the structural features of the plant communities. ... Ph.D. Thesis, Univ. of Western Aust., Nedlands. Google Scholar Churchill, D. M., 1968. The ...
The observations, data and deductions described in this thesis were collected from the Soutpansberg and surrounding areas over a period of over 20 years. This thesis aims to explain the underlying mechanisms driving the processes in the Soutpansberg that have brought forth its immense floristic diversity.
1. Introduction. Ethiopia with its vast geographical diversity and due to its high edaphic, climatic, topography, and altitudinal variations has a diversified biota (Yirdaw, Citation 2001).Although there is controversy over the precise figures of the former forest cover in Ethiopia, historical sources indicate that some 35-40% of the land area might have been once covered with forests (EFAP ...
A Review on Structure, Floristic Diversity and Functions of Homegardens. March 2023. DOI: 10.1007/978-981-99-0945-2_16. In book: Conservation of Biodiversity in the North Eastern States of India ...
Floristic inventory and diversity assessments are nece ssary to understand the present diversity status and. conservation of forest biodiversity. Although, inventory and diversity studies are ...
The present study was carried out to assess the floristic diversity and community characteristics in the forest and alpine zone of Gulmarg Wildlife Sanctuary (GWLS), Kashmir Himalaya. A total of 123 sites were selected along an elevational gradient (2300-4200 m a.m.s.l) in each and every accessible aspect and habitats from July 2018 to June 2022.
Certified that this thesis entitled "Floristic diversity,' autecology and competitive behavior of weed flora in wetland rice ecosystem" is a record of research work done independently by Sajith Babu. D. under my guidance and supervision and that it has not previously formed the basis for the award of any
Sharma A (2008) Studies on floristic diversity and prioritization of communities for conservation in Hirb and Shoja catchments, district Kullu of Himachal Pradesh, North Western Himalaya. (PhD Thesis). Dept. of Botany, GB Pant Institute of Himalayan Environment and Development, Himachal Unit, Kullu, Himachal Pradesh, India. pp 156-162.
The rapid loss in floristic diversity and changing pattern of vegetation due to various biotic and abiotic factors have necessitated the qualitative and quantitative assessment of vegetation. However numbers of studies on community dynamics and phytogeographic affinities have been conducted qualitatively [ 12 - 17 ] as well as quantitatively ...
This study assesses the effects of human disturbance on: (1) floristic composition, diversity, and plant community types; and, (2) the regeneration potential of woody species in the Debrelibanos Monastery forest patch in the North Showa zone of Oromia National Regional State, Ethiopia. Fifty sample quadrats were placed on parallel transect lines along altitudes in the forest using a systematic ...
The area lies at the latitude of 9.9183°N and a longitude of 76.4042°E. There is an average annual temperature of 33°C. The rainfall is significantly in most months of the year and has an average rainfall of 248 mm. The area is dry for 282 days a year with an average humidity of 42% and an UV index of 7.
Research on the relationship between fish and floristic diversity has been limited by narrow scopes, inconsistent methodologies, and geographic biases, resulting in fragmented insights.
Thesis on Floristic Diversity - Free download as PDF File (.pdf), Text File (.txt) or read online for free. The document discusses the challenges of writing a thesis on the complex topic of floristic diversity. It then introduces HelpWriting.net as a service that can assist students with their thesis projects by providing expert writing assistance.
The present chapter gives a synthesis of the floristic diversity of India, dwelling briefly on its ecosystem diversity, vegetation pattern, species richness in various taxonomic groups traditionally dealt with as plants (angiosperms, gymnosperms, pteridophytes, bryophytes, algae, fungi and lichens), endemism and primitive angiosperms and phytogeographical analysis of the flora.