
An official website of the United States government
Here’s how you know
Official websites use .gov A .gov website belongs to an official government organization in the United States.
Secure .gov websites use HTTPS A lock ( Lock A locked padlock ) or https:// means you’ve safely connected to the .gov website. Share sensitive information only on official, secure websites.


Acid rain and its environmental effects: Recent scientific advances
- More information: Publisher Index Page (via DOI)
- Open Access Version: Publisher Index Page
- Download citation as: RIS | Dublin Core
The term ‘acid rain’ refers to atmospheric deposition of acidic constituents that impact the earth as rain, snow, particulates, gases, and vapor. Acid rain was first recognized by Ducros (1845) and subsequently described by the English chemist Robert Angus Smith (Smith, 1852) whose pioneering studies linked the sources to industrial emissions and included early observations of deleterious environmental effects (Smith, 1872). Smith's work was largely forgotten until the mid-20th century when observations began to link air pollution to the deposition of atmospheric sulfate (SO 4 2− ) and other chemical constituents, first near the metal smelter at Sudbury, Ontario, Canada, and later at locations in Europe, North America, and Australia (Gorham, 1961). Our modern understanding of acid rain as an environmental problem caused largely by regional emissions of sulfur dioxide (SO 2 ) and nitrogen oxides (NO x ) stems from observations in the 1960s and early 1970s in Sweden by Svante Odén (Odén, 1976), and in North America by Gene Likens and colleagues (Likens and Bormann, 1974). These scientists and many who followed showed the link to emissions from coal-fired power plants and other industrial sources, and documented the environmental effects of acid rain such as the acidification of surface waters and toxic effects on vegetation, fish, and other biota.
Publication type | Article |
---|---|
Publication Subtype | Journal Article |
Title | Acid rain and its environmental effects: Recent scientific advances |
Series title | Atmospheric Environment |
DOI | 10.1016/j.atmosenv.2016.10.019 |
Volume | 146 |
Year Published | 2016 |
Language | English |
Publisher | Pergamon Press |
Publisher location | Oxford |
Contributing office(s) | New York Water Science Center |
Description | 4 p. |
First page | 1 |
Last page | 4 |
Google Analytic Metrics |

An official website of the United States government
The .gov means it’s official. Federal government websites often end in .gov or .mil. Before sharing sensitive information, make sure you’re on a federal government site.
The site is secure. The https:// ensures that you are connecting to the official website and that any information you provide is encrypted and transmitted securely.
- Publications
- Account settings
- My Bibliography
- Collections
- Citation manager
Save citation to file
Email citation, add to collections.
- Create a new collection
- Add to an existing collection
Add to My Bibliography
Your saved search, create a file for external citation management software, your rss feed.
- Search in PubMed
- Search in NLM Catalog
- Add to Search
Effects of acid rain on plant growth: A meta-analysis
Affiliations.
- 1 Guangdong Provincial Key Laboratory of Eco-circular Agriculture, South China Agricultural University, Guangzhou, 510642, China; Department of Ecology, College of Natural Resources and Environment, South China Agricultural University, Guangzhou, 510642, China; Guangdong Engineering Technology Research Centre of Modern Eco-agriculture and Circular Agriculture, Guangzhou, 510642, China.
- 2 Guangdong Provincial Key Laboratory of Eco-circular Agriculture, South China Agricultural University, Guangzhou, 510642, China; Department of Ecology, College of Natural Resources and Environment, South China Agricultural University, Guangzhou, 510642, China; Guangdong Engineering Technology Research Centre of Modern Eco-agriculture and Circular Agriculture, Guangzhou, 510642, China; Key Laboratory of Agro-Environment in the Tropics, Ministry of Agriculture and Rural Affairs, South China Agricultural University, Guangzhou, 510642, China; Guangdong Laboratory for Lingnan Modern Agriculture, Guangzhou, 510642, China. Electronic address: [email protected].
- 3 Guangdong Provincial Key Laboratory of Eco-circular Agriculture, South China Agricultural University, Guangzhou, 510642, China; Department of Ecology, College of Natural Resources and Environment, South China Agricultural University, Guangzhou, 510642, China; Guangdong Engineering Technology Research Centre of Modern Eco-agriculture and Circular Agriculture, Guangzhou, 510642, China; Key Laboratory of Agro-Environment in the Tropics, Ministry of Agriculture and Rural Affairs, South China Agricultural University, Guangzhou, 510642, China; Guangdong Laboratory for Lingnan Modern Agriculture, Guangzhou, 510642, China.
- PMID: 34329914
- DOI: 10.1016/j.jenvman.2021.113213
Anthropogenic driven acid gases emission has caused acid rain in many regions globally. Although efforts have been made to assess the effects of acid rain on terrestrial ecosystems, a systematic assessment of growth-related traits across plant aboveground and belowground is lacking. Hence, we performed a phylogenetically controlled meta-analysis of 755 observations from 69 independent studies to quantify the effects of acid rain on six growth-related traits of plant. We estimated the inhibitory effects of acid rain on plant growth in general and found that aboveground and belowground plant parts responded differently. The acidity of acid rain and acid rain interval had direct modulation effects on plant growth. We also found that there were interactions between acid rain pH and other acid rain characteristics (i.e., acid rain interval, mole ratio of S:N, and acid rain rate) and experimental characteristics (i.e., initial soil pH and plant exposure part), indicating that there were pH-dependent interaction patterns. Thus, an effective approach to evaluate and predict the effects of acid rain on plant growth is to fully consider the direct effects of acid rain pH and the interactions between acid rain pH and other factors.
Keywords: Acid deposition; Acidity level; Plant biomass; Plant shoots and roots; Rainfall pH; Sulfur deposition.
Copyright © 2021 Elsevier Ltd. All rights reserved.
PubMed Disclaimer
Similar articles
- Direct effect of acid rain on leaf chlorophyll content of terrestrial plants in China. Du E, Dong D, Zeng X, Sun Z, Jiang X, de Vries W. Du E, et al. Sci Total Environ. 2017 Dec 15;605-606:764-769. doi: 10.1016/j.scitotenv.2017.06.044. Epub 2017 Jul 2. Sci Total Environ. 2017. PMID: 28679120
- Comparative effects of sulfuric and nitric acid rain on litter decomposition and soil microbial community in subtropical plantation of Yangtze River Delta region. Liu X, Zhang B, Zhao W, Wang L, Xie D, Huo W, Wu Y, Zhang J. Liu X, et al. Sci Total Environ. 2017 Dec 1;601-602:669-678. doi: 10.1016/j.scitotenv.2017.05.151. Epub 2017 May 31. Sci Total Environ. 2017. PMID: 28577402
- Acid rain reduces plant-photosynthesized carbon sequestration and soil microbial network complexity. Liu Z, Chen J, Su Z, Liu Z, Li Y, Wang J, Wu L, Wei H, Zhang J. Liu Z, et al. Sci Total Environ. 2023 May 15;873:162030. doi: 10.1016/j.scitotenv.2023.162030. Epub 2023 Feb 4. Sci Total Environ. 2023. PMID: 36740064
- An overview of the direct and indirect effects of acid rain on plants: Relationships among acid rain, soil, microorganisms, and plants. Zhang Y, Li J, Tan J, Li W, Singh BP, Yang X, Bolan N, Chen X, Xu S, Bao Y, Lv D, Peng A, Zhou Y, Wang H. Zhang Y, et al. Sci Total Environ. 2023 May 15;873:162388. doi: 10.1016/j.scitotenv.2023.162388. Epub 2023 Feb 24. Sci Total Environ. 2023. PMID: 36842576 Review.
- Response and driving factors of soil enzyme activity related to acid rain: a meta-analysis. Shi Z, Zhang J, Zhang H, Wei H, Lu T, Chen X, Li H, Yang J, Liu Z. Shi Z, et al. Environ Sci Pollut Res Int. 2023 Oct;30(48):105072-105083. doi: 10.1007/s11356-023-29585-4. Epub 2023 Sep 20. Environ Sci Pollut Res Int. 2023. PMID: 37730980 Review.
- NO and H 2 S Contribute to Crop Resilience against Atmospheric Stressors. Corpas FJ. Corpas FJ. Int J Mol Sci. 2024 Mar 20;25(6):3509. doi: 10.3390/ijms25063509. Int J Mol Sci. 2024. PMID: 38542480 Free PMC article. Review.
- Effects of Simulated Acid Rain on Photosynthesis in Pinus massoniana and Cunninghamia lanceolata in Terms of Prompt Fluorescence, Delayed Fluorescence, and Modulated Reflection at 820 nm. Shu P, Gong X, Du Y, Han Y, Jin S, Wang Z, Qian P, Li X. Shu P, et al. Plants (Basel). 2024 Feb 24;13(5):622. doi: 10.3390/plants13050622. Plants (Basel). 2024. PMID: 38475467 Free PMC article.
Publication types
- Search in MeSH
LinkOut - more resources
Full text sources.
- Elsevier Science
- Citation Manager
NCBI Literature Resources
MeSH PMC Bookshelf Disclaimer
The PubMed wordmark and PubMed logo are registered trademarks of the U.S. Department of Health and Human Services (HHS). Unauthorized use of these marks is strictly prohibited.
Advertisement
The legacy from the 50 years of acid rain research, forming present and future research and monitoring of ecosystem impact
This article belongs to Ambio’s 50th Anniversary Collection. Theme: Acidification
- Perspective
- Open access
- Published: 08 December 2020
- Volume 50 , pages 273–277, ( 2021 )
Cite this article
You have full access to this open access article
- Bjørn Olav Rosseland ORCID: orcid.org/0000-0003-0739-7402 1
3646 Accesses
9 Citations
6 Altmetric
Explore all metrics
Acid rain and acidification research are indeed a multidisciplinary field. This field evolved from the first attempts to mitigate acid freshwater in the 1920s, then linking acid rain to the acidification in late 1950s, to the broad project-concepts on cause and effect from the late 1960s. Three papers from 1974, 1976 and 1988 demonstrate a broad approach and comprise scientific areas from analytical chemistry, biochemistry, limnology, ecology, physiology and genetics. Few, if any, environmental problems have led to a public awareness, political decisions and binding limitations as the story of acid rain. Acid precipitation and acidification problems still exist, but at a lower pressure, and liming has been reduced accordingly. However, the biological responses in the process of recovery are slow and delayed. The need for basic science, multidisciplinary studies, long time series of high-quality data, is a legacy from the acid rain era, and must form the platform for all future environmental projects.
Similar content being viewed by others
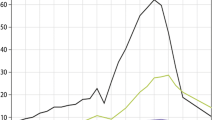
Acid rain and air pollution: 50 years of progress in environmental science and policy
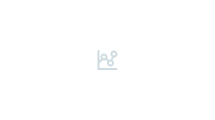
Ambio ’s legacy on monitoring, impact, and management of acid rain
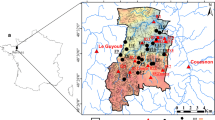
Long-term ecological observatories needed to understand ecohydrological systems in the Anthropocene: a catchment-scale case study in Brittany, France
Explore related subjects.
- Environmental Chemistry
Avoid common mistakes on your manuscript.
Three papers in Ambio , Almer et al. ( 1974 ), Schofield ( 1976 ) and Henriksen et al. ( 1988 ), represent a very important “state of the art” at their time, focusing on the huge environmental effect caused by long range transported air pollutants, called “acid rain”. In Scandinavia, acid water had been recognised as a problem for especially Atlantic salmon ( Salmo salar ) and brown trout ( Salmo trutta ) since the 1920s (Dahl 1927 ), and hatcheries had locally installed limestone-filters to improve hatching success. Researchers had first speculated that reduced fish populations were caused by fish diseases or over-fishing, until Dannevig ( 1959 ) claimed the acid precipitation to be a major factor in causing the problems. However, the focus set by Professor Svante Odén in Sweden in 1968 was unique, leading to extensive investigations, documentation of status and onset of national projects in Sweden, and later in Norway (Grennfelt et al. 2020 ). The Almer et al. ( 1974 ) paper contained high-quality chemical data from Swedish lakes, sampled in a systematic way and linked to biological data from many of the same lakes, including primary producers (algae), invertebrates and fish. Observations of increased clarity of lakes formed the hypothesis of “oligotrophication”. Increased concentrations of several trace elements like Pb and Zn were also described. This paper was extremely important for the onset of similar projects and programs in many countries suffering from acid rain. It raised the international awareness to the top political level, leading finally to the international agreements on reductions in sulphur (S), nitrogen (N), ozone, heavy metals and Persistent Organic Pollutants (POPs).
The study of a major fishkill of brown trout in the Norwegian River Tovdal during snowmelt in 1975, enabled a first understanding of the physiological responses to these episodic events of low pH and ionic dilution (Leivestad and Muniz 1976 ). This article was central in the review paper in Ambio by Schofield ( 1976 ), where he pointed to the common phenomenon of increasing numbers of barren lakes in Scandinavia, as in the eastern North America. He also concluded that the youngest life stages, egg to larvae, were the most sensitive, leading to reproduction failure and lacking year-classes. Species and strain differences in sensitivity to acid water were discussed, but because of the rapid acidification of the environment, natural selection of more tolerant strains or populations seemed not to have occurred. The reference list reflects a huge ongoing research into the field, as many papers were termed “in press” or “in preparation”.
Indeed, this was the start of an era where field data and laboratory studies were paralleled. Until 1977, the explanation for the negative biological effects of acid water was the low pH combined with low conductivity and calcium. A breakthrough came in 1977, when a report from Schofield ( 1977 ) suggested that aluminium (Al) could be THE key factor for the toxicity in acid water. Overnight, physiological groups in many countries included Al in their experiments. Another breaking news was the discovery of the importance of the chemical speciation of the Al, separating organic bound Al, from the non-organic, termed labile Al (LAl) or inorganic Al (Ali), and that the freshwater toxicity of Al was linked to LAl (Driscoll et al. 1980 ). New laboratory methods were developed to identify these species (e.g. Driscoll 1984 ).
Liming of acid waters started as a test program (1976–1981) in Sweden (Henrikson and Brodin 1995 ). Large lakes with a retention time of several years were their first target. When the Norwegian liming project started, 1978–1984, the cooperation with Sweden was strong, and has been like that ever since. The small sized lakes with heavy rainfall and retention times in months in Norway, called for other criteria and methods, including liming of large rivers (Baalsrud et al. 1985 ). The Swedish and Norwegian mitigation programs have been a great success and became the fundament for liming programs in both the USA and Canada.
In the early 1980s, there was a mass mortality of Atlantic salmon in River Vikedal (Norway) in their smolt-stage, prepared to migrate into seawater. This changed the focus from eggs and juveniles, to smolts being the most sensitive life history stage (Rosseland and Skogheim 1984 ). This meant that the water quality in rivers housing Atlantic salmon had to be protective for smolts from early spring until June, the period where smoltification and migration occurs. Eight years later, the phenomenon of “Mixing Zones” was described (Rosseland et al. 1992 ). A chemical inequilibrium zone formed downstream the mixture of an acid stream and a neutral or limed river resulted in Al polymerization and an initially extreme toxicity. Such zones could kill year-classes of smolts while migrating during spring floods. Liming all side tributaries to avoid such zones increased the cost of liming rivers.
The problems associated with acidification called for long-term monitoring, and national programs started in the beginning of the 1980s. Lakes and rivers, not influenced by liming, formed the basis for annual or periodic sampling of water and biota. ICP Waters, “the International Cooperative Program for Assessment and Monitoring of the Effects of Air Pollution on Rivers and Lakes”, started in 1984 and included water chemistry and biota.
The Norwegian 1000-lake study by Henriksen et al. ( 1988 ) was launched at a time when large databases from Norwegian lakes existed (i.e. Wright and Snekvik 1977 ), and where the scientific understanding of the relations between chemical elements and their biological effects was greatly improved, relative to the 1970s, and where politicians had agreed and started to reduce emissions. The study of Henriksen et al. ( 1988 ) included up-to-date chemistry data and a lake selection, similar to that in Almer et al. ( 1974 ) paper. Unlike lakes in Sweden and northeastern America, the Norwegian lakes had low TOC (Total Organic Carbon) and low conductivity waters with few species of fish, mainly brown trout and Arctic charr ( Salvelinus alpinus ). The lakes selected included reference lakes and covered the whole country. Besides water sampling in autumn, great effort was put into information of fish status from the same lakes, which resulted in a second paper in Ambio one year later (Henriksen et al. 1989 ). These two papers, where chemical data were directly linked to fish status, gave a basis for the application of a series of models like MAGIC (Model of Acidification of Groundwater in Catchments, Cosby et al. 1985 ) for the prediction of past and future environmental changes caused by acid rain. The monitoring program in Norway chose 100 lakes from these studies, to be followed at regular sampling intervals. Problems occurred, however, as some lakes became influenced by upstream liming or other catchment changes. True references over time are difficult to maintain.
All three papers (Almer et al 1974 ; Schofield 1976 ; Henriksen et al. 1988 ) reflect an environmental status from a time of high S and N deposition, in catchments still in the process of acidification. In the 1970s, before the international acceptance and agreement on reductions in emissions, we were not fully aware of the large time lag between change in precipitation chemistry, catchment reactions, water chemistry change and biological responses. Results from test fishing had revealed reproduction failure nearly 20 years previously to sampling (Rosseland et al. 1980 ). This illustrates that fish status cannot be reliably predicted from present lake chemistry. Prognoses of biological recovery, as depositions became reduced, had therefore to be corrected, as the recovery followed a pattern of hysteresis. Models for restoring a population of Atlantic salmon, ASRAM (“Atlantic Salmon Regional Acidification Model») Korman et al. ( 1994 ) forecasted 12–15 years of recovery, given no episodes of critical water quality for the most sensitive life stage. One critical episode could, however, delay Atlantic salmon recovery by another 10–15 years, a recovery delay also found for brown trout by the FIB-model (Raddum and Rosseland 2005 ). Recovery of fish was consistently associated with increasing pH, reduced Al, increased TOC (Keller et al. 2019 ), but also a decreased Ca concentration (Jeziorski et al. 2008 ). As Ca is a physiological important protective ion for aquatic organisms, this could slow down the biological recovery, partly explaining why fish and invertebrate populations struggled, despite “good” and improved water quality. Today’s practice with downscaling of liming in accordance with the reduced emissions will therefore depend on careful monitoring of the biological communities and their most sensitive species and life history stages for evaluation if end of liming was correct (Anderson et al. 2002 ).
More developed genetic and physiological methods and chemical speciation tools have enabled a deeper understanding of species-, strain- and life history stage sensitivities. The extreme sensitivity towards Al for Atlantic salmon smolts prior to sea migration (Kroglund et al. 2008 ) is caused by the formation of the “supersensitive seawater isoform” of Na–K–ATPase (Nilsen et al. 2010 ), the major ion-regulatory enzymes in gills. Experiences from Al toxicity studies are now a model for testing of other metals, radioactive substances and organic substances in fresh or marine waters.
The scientific era of acidification, starting in the early 1970s and represented by the three papers I reflect on here, forms the basis of many environmental programs until today. Long time series of data (chemistry or biota) based on international protocols, and permanent sites with minimum disturbances, are mandatory for any modelling and forecasting of environmental or climate change. Huge international research programs and manipulation studies, many partly financed by the EU, have studied catchment responses to increased or decreased occurrence of, e.g. S, N, TOC and CO 2 , as well as changing temperature. The development of techniques of detoxifying acid water through lime, silica lye, use of seawater, etc., have been an extremely important factor for the success of Atlantic salmon smolt production worldwide. In 2017, 15% of the wild Atlantic salmon caught by anglers in Norway was in limed rivers.
Data from one of the longest running chemical and biological data series (Lake Saudlandsvatn, Hesthagen et al. 2011 , Fig. 1 ) were used to illustrate positive changes in European environments (Maas and Grennfelt 2016 ) and demonstrated a process of recovery from acidification in biota. However, the figure does not show the lack of older age classes in brown trout, dying after spawning, as a result of still marginal chemical conditions for this sensitive life history stage.
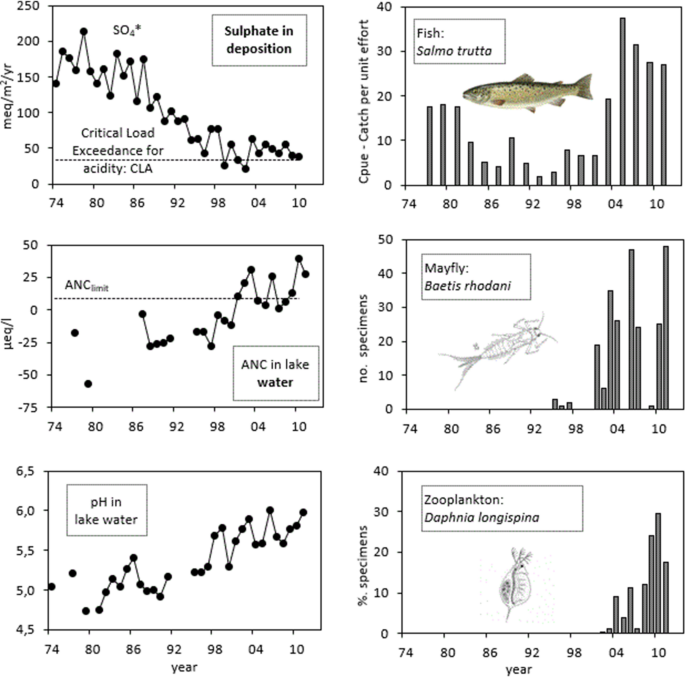
modified from Hesthagen et al. ( 2011 ), was used in the report by Maas and Grennfelt ( 2016 ) to illustrate recovery from acidification at Lake Saudlandsvatn, Southern Norway. As sulphur deposition has decreased, so the acid neutralising capacity (ANC) and pH of the lake water have increased, and the populations of the three sensitive species have begun the process of recovery. The figure looks very promising, also for brown trout, but the increase in catch from 1994 and onwards was mainly by young fish. Lacking adult post-spawners indicates marginal chemical conditions to a sensitive life history stage
This figure,
In 2019, a “new” 1000-lake study was performed in Norway. The legacy from the acidification research will forever be a “lesson to be learnt” for future environmental science programs, and reference for modelling of our future environment.
Almer, B., W. Dickson, C. Ekström, E. Hörnström, and U. Miller. 1974. Effects of acidification on Swedish lakes. Ambio 3: 30–36.
Google Scholar
Anderson, B.A., H. Borg, F. Edberg, and H. Hultberg. 2002. Återförsurning av sjöar. Observerade och förväntade biologiska och kemiska effekter . Naturvärdsverket Rapport 5249 2002. ISBN 91-620-5249-7.pdf.
Baalsrud, K., A. Hindar, M. Johannessen, and D. Matzow, eds. 1985. Lime treatment of acid water . Final technical report of the Norwegian liming project. ISBN 82-90404-30-1.
Cosby, B.J., R.F. Wright, G.M. Hornberger, and J.N. Galloway. 1985. Modelling the effects of acid deposition: Estimation of long term water quality responses in a small forested catchment. Water Resources Research 21: 1591–1601.
Article CAS Google Scholar
Dahl, K. 1927. The effect of acid water on trout fry. Salmon and Trout Magazine 46: 35–43.
Dannevig, A. 1959. Influence from precipitation on the acidity of freshwater and fish populations. Jeger og Fisker 3: 116–118 (in Norwegian).
Driscoll, C.T., J.P. Baker, J.J. Bisogni Jr., and C.L. Scofield. 1980. Effects of aluminium speciation on fish in dilute acidified waters. Nature 284: 161–164.
Driscoll, C.T. 1984. A procedure for the fractionation of aqueous aluminium in dilute acidic waters. International Journal of Environmental Analytical Chemistry 16: 267–283.
Grennfelt, P., A. Engleryd, M. Forsius, Ø. Hov, H. Rohde, and E. Cowling. 2020. Acid rain and air pollution: 50 Years of progress in environmental science and policy. Ambio 49: 849–864. https://doi.org/10.1007/s13280-019-01244-4 .
Henriksen, A., L. Lien, T. Traaen, I. Sevaldrud, and D.F. Brakke. 1988. Lake acidification in Norway—Present and predicted chemical status. Ambio 17: 259–266.
CAS Google Scholar
Henriksen, A., L. Lien, B.O. Rosseland, T. Traaen, and I. Sevaldrud. 1989. Lake acidification in Norway—Present and predicted fish status. Ambio 18: 314–321.
Henrikson, L., and Y.W. Brodin, eds. 1995. Liming of acidification surface waters. A Swedish synthesis , 457. Berlin: Springer. ISBN-13:978-3-642-79311-0.
Hesthagen, T., A. Fjellheim, A.K. Schartau, R.F. Wright, R. Saksgård, and B.O. Rosseland. 2011. Chemical and biological recovery of Lake Saudlandsvatn, a highly acidified lake in southernmost Norway, in response to decreased acid deposition. Science of the Total Environment 409: 2908–2916.
Jeziorski, A., N.D. Yan, A.M. Paterson, A.M. DeSellas, M.A. Turner, D.S. Jeffries, B. Keller, R.C. Weeber, et al. 2008. The widespread treat of calcium decline in fresh waters. Science 322: 1374–1377. https://doi.org/10.1126/science.1164949 .
Keller, W., J. Heneberry, and B.A. Edwards. 2019. Recovery of acidified Sudbury, Ontario, Canada, lakes: A multi-decade synthesis and update. Environmental Reviews 27 (1): 1–16. https://doi.org/10.1139/er-2018-0018 .
Article Google Scholar
Korman, J., D.R. Marmorek, G.L. Lacroix, P.G. Amiro, J.A. Ritter, W.D. Watt, R.E. Cutting, and D.C.E. Robinson. 1994. Development and evaluation of a biological model to assess regional-scale effects of acidification on Atlantic salmon ( Salmo salar ). Canadian Journal of Fisheries and Aquatic Sciences 51: 662–680.
Kroglund, F., B.O. Rosseland, H.-C. Teien, B. Salbu, T. Kristensen, and B. Finstad. 2008. Water quality limits for Atlantic salmon ( Salmo salar L.) exposed to short term reductions in pH and increased aluminium simulating episodes. Hydrology and Earth System Science 12: 491–507.
Leivestad, H., and I.P. Muniz. 1976. Fish kill at low pH in a Norwegian river. Nature 259: 391–392.
Maas, R., and P. Grennfelt, eds. 2016. Towards Cleaner Air. Scientific Assessment Report 2016 . Oslo: EMEP Steering Body and Working Group on Effects of the Convention on Long-Range Transboundary Air Pollution. https://www.unece.org/fileadmin/DAM/env/lrtap/ExecutiveBody/35th_session/CLRTAP_Scientific_Assessment_Report_-_Final_20-5-2016.pdf .
Nilsen, T.O., L.O.E. Ebbeson, O.G. Kverneland, F. Kroglund, B. Finstad, and S.O. Stefansson. 2010. Effects of acid water and aluminium exposure on gill Na + , K + –ATPase α-subunit isoforms, enzyme activity, physiology and return rates in Atlantic salmon (S almo salar L.). Aquatic Toxicology 97: 250–259. https://doi.org/10.1016/j.aquatox.2009.12.001 .
Raddum, G., and B.O. Rosseland. 2005. Chapter 9: Dynamic biological response models—An overview. In Proceedings of the 20th meeting of the ICP Waters Programme Task Force , Falun, Sweden, October 18–20, 2004. NIVA Report 5018-2005. ICP Waters report 80/2005, eds. H. deWitt and B.L. Skjelkvåle, 45–54.
Rosseland, B.O., I. Sevaldrud, D. Svalastog, and I.P. Muniz. 1980. Studies on freshwater fish populations—Effects of acidification on reproduction, population structure, growth and food selection. In Ecological impact of acid precipitation . SNSF-project FA 105/80, eds. A. Tollan, and D. Drabløs, 336–337.
Rosseland, B.O., and O.K. Skogheim. 1984. A comparative study on salmonid fish species in acid aluminium-rich water. II. Physiological stress and mortality of one and two year old fish. Report of Institute of Freshwater Research Drottningholm 61: 186–194.
Rosseland, B.O., I. Blakar, A. Bulger, F. Kroglund, A. Kvellestad, E. Lydersen, D.H. Oughton, B. Salbu, et al. 1992. The mixing zone between limed and acidic river waters: Complex aluminium chemistry and extreme toxicity for Salmonids. Environmental Pollution 78: 3–8.
Schofield, C.L. 1976. Effects on fish. Ambio 5: 228–230.
Schofield, C.L. 1977. Acid snow-melt effects on water quality and fish survival in the Adirondack Mountains of New York State . Res. Proj. Tech. Comp. Rep. Project A-072-NY. Ithaca: Cornell University.
Wright, R.F., and E. Snekvik. 1977. Chemistry and fish populations in 700 lakes in southernmost Norway . SNSF-project, TN 17/77.
Download references
Acknowledgements
I wish to thank Dr. Bo Söderström and Dr. Lars Tranvik for the invitation to contribute to this very important Ambio anniversary collection on “Acid Rain”. As a member of the “International Acid Rain Family” for 45 years, it has been a privilege to work with so many great scientists and students in many of the affected countries. This interdisciplinary field, with open national and international cooperation, funded mainly by Governments, Research Councils and Universities, sets an example for how to cope with today’s and future environmental challenges. A special thanks goes to Dr. Lars Tranvik, Dr. John P. Smol and an anonymous referee, for greatly improving the manuscript.
Open Access funding provided by Norwegian University of Life Sciences.
Author information
Authors and affiliations.
Faculty of Environmental Sciences and Natural Resource Management (MINA), Norwegian University of Life Sciences (NMBU), Sørhellinga, Postboks 5003 NMBU, 1432, Ås, Norway
Bjørn Olav Rosseland
You can also search for this author in PubMed Google Scholar
Corresponding author
Correspondence to Bjørn Olav Rosseland .
Additional information
Publisher's note.
Springer Nature remains neutral with regard to jurisdictional claims in published maps and institutional affiliations.
Rights and permissions
Open Access This article is licensed under a Creative Commons Attribution 4.0 International License, which permits use, sharing, adaptation, distribution and reproduction in any medium or format, as long as you give appropriate credit to the original author(s) and the source, provide a link to the Creative Commons licence, and indicate if changes were made. The images or other third party material in this article are included in the article's Creative Commons licence, unless indicated otherwise in a credit line to the material. If material is not included in the article's Creative Commons licence and your intended use is not permitted by statutory regulation or exceeds the permitted use, you will need to obtain permission directly from the copyright holder. To view a copy of this licence, visit http://creativecommons.org/licenses/by/4.0/ .
Reprints and permissions
About this article
Rosseland, B.O. The legacy from the 50 years of acid rain research, forming present and future research and monitoring of ecosystem impact. Ambio 50 , 273–277 (2021). https://doi.org/10.1007/s13280-020-01408-7
Download citation
Received : 08 July 2020
Revised : 01 October 2020
Accepted : 05 October 2020
Published : 08 December 2020
Issue Date : February 2021
DOI : https://doi.org/10.1007/s13280-020-01408-7
Share this article
Anyone you share the following link with will be able to read this content:
Sorry, a shareable link is not currently available for this article.
Provided by the Springer Nature SharedIt content-sharing initiative
- Acidification
- Find a journal
- Publish with us
- Track your research
Acid Rain: Does it Contribute to Forest Decline?
PDF Version Also Available for Download.
Description
This minibrief describes the major hypothesis explaining why acid rain may be contributing to forest decline, along with the major arguments against this hypothesis. For additional information on acid rain and current legislation for pollutant emissions controls, see IB83016 -- Acid Rain: Current Issues, and IB83005 -- Clean Air Act: An Overview.
Physical Description
Creation information.
Backiel, Adela January 24, 1985.
This report is part of the collection entitled: Congressional Research Service Reports and was provided by the UNT Libraries Government Documents Department to the UNT Digital Library , a digital repository hosted by the UNT Libraries . It has been viewed 592 times. More information about this report can be viewed below.
People and organizations associated with either the creation of this report or its content.
- Backiel, Adela Environment and Natural Resources Policy Division
- Library of Congress. Congressional Research Service. Place of Publication: Washington D.C.
Provided By
Unt libraries government documents department.
Serving as both a federal and a state depository library, the UNT Libraries Government Documents Department maintains millions of items in a variety of formats. The department is a member of the FDLP Content Partnerships Program and an Affiliated Archive of the National Archives.
Descriptive information to help identify this report. Follow the links below to find similar items on the Digital Library.
Legislative Indexing Vocabulary
- Air pollution
- Air pollution control
- Environmental policy
- Environmental protection
- Environmental research
- Forest ecology
- Natural resources
Unique identifying numbers for this report in the Digital Library or other systems.
- CRS Report Number : MB84204 | Check for Other Editions
- Accession or Local Control No : MB84204 1985-01-24
- Archival Resource Key : ark:/67531/metacrs9048
Collections
This report is part of the following collection of related materials.
Congressional Research Service Reports
The Congressional Research Service (CRS) is the public policy research arm of Congress. This legislative branch agency works exclusively for Members of Congress, their committees and their staff. This collection includes CRS reports from the mid-1960's through 2018—covering a variety of topics from agriculture to foreign policy to welfare.
What responsibilities do I have when using this report?
Digital Files
- 6 image files available in multiple sizes
- 1 file (.pdf)
- Metadata API: descriptive and downloadable metadata available in other formats
Dates and time periods associated with this report.
Creation Date
- January 24, 1985
Coverage Date
Added to the unt digital library.
- Aug. 10, 2006, 2:36 p.m.
Description Last Updated
- April 5, 2020, 2 p.m.
Usage Statistics
When was this report last used?
Interact With This Report
Here are some suggestions for what to do next.
Search Inside
- or search this site for other reports
Start Reading
- All Formats
Citations, Rights, Re-Use
- Citing this Report
- Responsibilities of Use
- Licensing and Permissions
- Linking and Embedding
- Copies and Reproductions
International Image Interoperability Framework

We support the IIIF Presentation API
Print / Share
Links for robots.
Helpful links in machine-readable formats.
Archival Resource Key (ARK)
- ERC Record: /ark:/67531/metacrs9048/?
- Persistence Statement: /ark:/67531/metacrs9048/??
International Image Interoperability Framework (IIIF)
- IIIF Manifest: /ark:/67531/metacrs9048/manifest/
Metadata Formats
- UNTL Format: /ark:/67531/metacrs9048/metadata.untl.xml
- DC RDF: /ark:/67531/metacrs9048/metadata.dc.rdf
- DC XML: /ark:/67531/metacrs9048/metadata.dc.xml
- OAI_DC : /oai/?verb=GetRecord&metadataPrefix=oai_dc&identifier=info:ark/67531/metacrs9048
- METS : /ark:/67531/metacrs9048/metadata.mets.xml
- OpenSearch Document: /ark:/67531/metacrs9048/opensearch.xml
- Thumbnail: /ark:/67531/metacrs9048/thumbnail/
- Small Image: /ark:/67531/metacrs9048/small/
- In-text: /ark:/67531/metacrs9048/urls.txt
- Usage Stats: /stats/stats.json?ark=ark:/67531/metacrs9048
Backiel, Adela. Acid Rain: Does it Contribute to Forest Decline? , report , January 24, 1985; Washington D.C. . ( https://digital.library.unt.edu/ark:/67531/metacrs9048/ : accessed August 22, 2024 ), University of North Texas Libraries, UNT Digital Library, https://digital.library.unt.edu ; crediting UNT Libraries Government Documents Department .

An official website of the United States government
The .gov means it’s official. Federal government websites often end in .gov or .mil. Before sharing sensitive information, make sure you’re on a federal government site.
The site is secure. The https:// ensures that you are connecting to the official website and that any information you provide is encrypted and transmitted securely.
- Publications
- Account settings
Preview improvements coming to the PMC website in October 2024. Learn More or Try it out now .
- Advanced Search
- Journal List
- v.50(2); 2021 Feb

The legacy from the 50 years of acid rain research, forming present and future research and monitoring of ecosystem impact
Bjørn olav rosseland.
Faculty of Environmental Sciences and Natural Resource Management (MINA), Norwegian University of Life Sciences (NMBU), Sørhellinga, Postboks 5003 NMBU, 1432 Ås, Norway
Acid rain and acidification research are indeed a multidisciplinary field. This field evolved from the first attempts to mitigate acid freshwater in the 1920s, then linking acid rain to the acidification in late 1950s, to the broad project-concepts on cause and effect from the late 1960s. Three papers from 1974, 1976 and 1988 demonstrate a broad approach and comprise scientific areas from analytical chemistry, biochemistry, limnology, ecology, physiology and genetics. Few, if any, environmental problems have led to a public awareness, political decisions and binding limitations as the story of acid rain. Acid precipitation and acidification problems still exist, but at a lower pressure, and liming has been reduced accordingly. However, the biological responses in the process of recovery are slow and delayed. The need for basic science, multidisciplinary studies, long time series of high-quality data, is a legacy from the acid rain era, and must form the platform for all future environmental projects.
Three papers in Ambio , Almer et al. ( 1974 ), Schofield ( 1976 ) and Henriksen et al. ( 1988 ), represent a very important “state of the art” at their time, focusing on the huge environmental effect caused by long range transported air pollutants, called “acid rain”. In Scandinavia, acid water had been recognised as a problem for especially Atlantic salmon ( Salmo salar ) and brown trout ( Salmo trutta ) since the 1920s (Dahl 1927 ), and hatcheries had locally installed limestone-filters to improve hatching success. Researchers had first speculated that reduced fish populations were caused by fish diseases or over-fishing, until Dannevig ( 1959 ) claimed the acid precipitation to be a major factor in causing the problems. However, the focus set by Professor Svante Odén in Sweden in 1968 was unique, leading to extensive investigations, documentation of status and onset of national projects in Sweden, and later in Norway (Grennfelt et al. 2020 ). The Almer et al. ( 1974 ) paper contained high-quality chemical data from Swedish lakes, sampled in a systematic way and linked to biological data from many of the same lakes, including primary producers (algae), invertebrates and fish. Observations of increased clarity of lakes formed the hypothesis of “oligotrophication”. Increased concentrations of several trace elements like Pb and Zn were also described. This paper was extremely important for the onset of similar projects and programs in many countries suffering from acid rain. It raised the international awareness to the top political level, leading finally to the international agreements on reductions in sulphur (S), nitrogen (N), ozone, heavy metals and Persistent Organic Pollutants (POPs).
The study of a major fishkill of brown trout in the Norwegian River Tovdal during snowmelt in 1975, enabled a first understanding of the physiological responses to these episodic events of low pH and ionic dilution (Leivestad and Muniz 1976 ). This article was central in the review paper in Ambio by Schofield ( 1976 ), where he pointed to the common phenomenon of increasing numbers of barren lakes in Scandinavia, as in the eastern North America. He also concluded that the youngest life stages, egg to larvae, were the most sensitive, leading to reproduction failure and lacking year-classes. Species and strain differences in sensitivity to acid water were discussed, but because of the rapid acidification of the environment, natural selection of more tolerant strains or populations seemed not to have occurred. The reference list reflects a huge ongoing research into the field, as many papers were termed “in press” or “in preparation”.
Indeed, this was the start of an era where field data and laboratory studies were paralleled. Until 1977, the explanation for the negative biological effects of acid water was the low pH combined with low conductivity and calcium. A breakthrough came in 1977, when a report from Schofield ( 1977 ) suggested that aluminium (Al) could be THE key factor for the toxicity in acid water. Overnight, physiological groups in many countries included Al in their experiments. Another breaking news was the discovery of the importance of the chemical speciation of the Al, separating organic bound Al, from the non-organic, termed labile Al (LAl) or inorganic Al (Ali), and that the freshwater toxicity of Al was linked to LAl (Driscoll et al. 1980 ). New laboratory methods were developed to identify these species (e.g. Driscoll 1984 ).
Liming of acid waters started as a test program (1976–1981) in Sweden (Henrikson and Brodin 1995 ). Large lakes with a retention time of several years were their first target. When the Norwegian liming project started, 1978–1984, the cooperation with Sweden was strong, and has been like that ever since. The small sized lakes with heavy rainfall and retention times in months in Norway, called for other criteria and methods, including liming of large rivers (Baalsrud et al. 1985 ). The Swedish and Norwegian mitigation programs have been a great success and became the fundament for liming programs in both the USA and Canada.
In the early 1980s, there was a mass mortality of Atlantic salmon in River Vikedal (Norway) in their smolt-stage, prepared to migrate into seawater. This changed the focus from eggs and juveniles, to smolts being the most sensitive life history stage (Rosseland and Skogheim 1984 ). This meant that the water quality in rivers housing Atlantic salmon had to be protective for smolts from early spring until June, the period where smoltification and migration occurs. Eight years later, the phenomenon of “Mixing Zones” was described (Rosseland et al. 1992 ). A chemical inequilibrium zone formed downstream the mixture of an acid stream and a neutral or limed river resulted in Al polymerization and an initially extreme toxicity. Such zones could kill year-classes of smolts while migrating during spring floods. Liming all side tributaries to avoid such zones increased the cost of liming rivers.
The problems associated with acidification called for long-term monitoring, and national programs started in the beginning of the 1980s. Lakes and rivers, not influenced by liming, formed the basis for annual or periodic sampling of water and biota. ICP Waters, “the International Cooperative Program for Assessment and Monitoring of the Effects of Air Pollution on Rivers and Lakes”, started in 1984 and included water chemistry and biota.
The Norwegian 1000-lake study by Henriksen et al. ( 1988 ) was launched at a time when large databases from Norwegian lakes existed (i.e. Wright and Snekvik 1977 ), and where the scientific understanding of the relations between chemical elements and their biological effects was greatly improved, relative to the 1970s, and where politicians had agreed and started to reduce emissions. The study of Henriksen et al. ( 1988 ) included up-to-date chemistry data and a lake selection, similar to that in Almer et al. ( 1974 ) paper. Unlike lakes in Sweden and northeastern America, the Norwegian lakes had low TOC (Total Organic Carbon) and low conductivity waters with few species of fish, mainly brown trout and Arctic charr ( Salvelinus alpinus ). The lakes selected included reference lakes and covered the whole country. Besides water sampling in autumn, great effort was put into information of fish status from the same lakes, which resulted in a second paper in Ambio one year later (Henriksen et al. 1989 ). These two papers, where chemical data were directly linked to fish status, gave a basis for the application of a series of models like MAGIC (Model of Acidification of Groundwater in Catchments, Cosby et al. 1985 ) for the prediction of past and future environmental changes caused by acid rain. The monitoring program in Norway chose 100 lakes from these studies, to be followed at regular sampling intervals. Problems occurred, however, as some lakes became influenced by upstream liming or other catchment changes. True references over time are difficult to maintain.
All three papers (Almer et al 1974 ; Schofield 1976 ; Henriksen et al. 1988 ) reflect an environmental status from a time of high S and N deposition, in catchments still in the process of acidification. In the 1970s, before the international acceptance and agreement on reductions in emissions, we were not fully aware of the large time lag between change in precipitation chemistry, catchment reactions, water chemistry change and biological responses. Results from test fishing had revealed reproduction failure nearly 20 years previously to sampling (Rosseland et al. 1980 ). This illustrates that fish status cannot be reliably predicted from present lake chemistry. Prognoses of biological recovery, as depositions became reduced, had therefore to be corrected, as the recovery followed a pattern of hysteresis. Models for restoring a population of Atlantic salmon, ASRAM (“Atlantic Salmon Regional Acidification Model») Korman et al. ( 1994 ) forecasted 12–15 years of recovery, given no episodes of critical water quality for the most sensitive life stage. One critical episode could, however, delay Atlantic salmon recovery by another 10–15 years, a recovery delay also found for brown trout by the FIB-model (Raddum and Rosseland 2005 ). Recovery of fish was consistently associated with increasing pH, reduced Al, increased TOC (Keller et al. 2019 ), but also a decreased Ca concentration (Jeziorski et al. 2008 ). As Ca is a physiological important protective ion for aquatic organisms, this could slow down the biological recovery, partly explaining why fish and invertebrate populations struggled, despite “good” and improved water quality. Today’s practice with downscaling of liming in accordance with the reduced emissions will therefore depend on careful monitoring of the biological communities and their most sensitive species and life history stages for evaluation if end of liming was correct (Anderson et al. 2002 ).
More developed genetic and physiological methods and chemical speciation tools have enabled a deeper understanding of species-, strain- and life history stage sensitivities. The extreme sensitivity towards Al for Atlantic salmon smolts prior to sea migration (Kroglund et al. 2008 ) is caused by the formation of the “supersensitive seawater isoform” of Na–K–ATPase (Nilsen et al. 2010 ), the major ion-regulatory enzymes in gills. Experiences from Al toxicity studies are now a model for testing of other metals, radioactive substances and organic substances in fresh or marine waters.
The scientific era of acidification, starting in the early 1970s and represented by the three papers I reflect on here, forms the basis of many environmental programs until today. Long time series of data (chemistry or biota) based on international protocols, and permanent sites with minimum disturbances, are mandatory for any modelling and forecasting of environmental or climate change. Huge international research programs and manipulation studies, many partly financed by the EU, have studied catchment responses to increased or decreased occurrence of, e.g. S, N, TOC and CO 2 , as well as changing temperature. The development of techniques of detoxifying acid water through lime, silica lye, use of seawater, etc., have been an extremely important factor for the success of Atlantic salmon smolt production worldwide. In 2017, 15% of the wild Atlantic salmon caught by anglers in Norway was in limed rivers.
Data from one of the longest running chemical and biological data series (Lake Saudlandsvatn, Hesthagen et al. 2011 , Fig. 1 ) were used to illustrate positive changes in European environments (Maas and Grennfelt 2016 ) and demonstrated a process of recovery from acidification in biota. However, the figure does not show the lack of older age classes in brown trout, dying after spawning, as a result of still marginal chemical conditions for this sensitive life history stage.

This figure,
modified from Hesthagen et al. ( 2011 ), was used in the report by Maas and Grennfelt ( 2016 ) to illustrate recovery from acidification at Lake Saudlandsvatn, Southern Norway. As sulphur deposition has decreased, so the acid neutralising capacity (ANC) and pH of the lake water have increased, and the populations of the three sensitive species have begun the process of recovery. The figure looks very promising, also for brown trout, but the increase in catch from 1994 and onwards was mainly by young fish. Lacking adult post-spawners indicates marginal chemical conditions to a sensitive life history stage
In 2019, a “new” 1000-lake study was performed in Norway. The legacy from the acidification research will forever be a “lesson to be learnt” for future environmental science programs, and reference for modelling of our future environment.
Acknowledgements
I wish to thank Dr. Bo Söderström and Dr. Lars Tranvik for the invitation to contribute to this very important Ambio anniversary collection on “Acid Rain”. As a member of the “International Acid Rain Family” for 45 years, it has been a privilege to work with so many great scientists and students in many of the affected countries. This interdisciplinary field, with open national and international cooperation, funded mainly by Governments, Research Councils and Universities, sets an example for how to cope with today’s and future environmental challenges. A special thanks goes to Dr. Lars Tranvik, Dr. John P. Smol and an anonymous referee, for greatly improving the manuscript.
Dr. Philos, is a Professor Emeritus in Ecotoxicology at the Norwegian University of Life Sciences. As a Fish Physiologist, he studied the toxicity as well as mitigation of acid water towards fish from 1975. He has documented contamination of trace metals, POPs, and radioactivity in natural fish populations from five continents. He has since early 1980s taught water quality, ecotoxicology and fish welfare in aquaculture in many countries and held professor positions at three universities, including Canada.
Open Access funding provided by Norwegian University of Life Sciences.
Publisher's Note
Springer Nature remains neutral with regard to jurisdictional claims in published maps and institutional affiliations.
- Almer B, Dickson W, Ekström C, Hörnström E, Miller U. Effects of acidification on Swedish lakes. Ambio. 1974; 3 :30–36. [ Google Scholar ]
- Anderson, B.A., H. Borg, F. Edberg, and H. Hultberg. 2002. Återförsurning av sjöar. Observerade och förväntade biologiska och kemiska effekter . Naturvärdsverket Rapport 5249 2002. ISBN 91-620-5249-7.pdf.
- Baalsrud, K., A. Hindar, M. Johannessen, and D. Matzow, eds. 1985. Lime treatment of acid water . Final technical report of the Norwegian liming project. ISBN 82-90404-30-1.
- Cosby BJ, Wright RF, Hornberger GM, Galloway JN. Modelling the effects of acid deposition: Estimation of long term water quality responses in a small forested catchment. Water Resources Research. 1985; 21 :1591–1601. doi: 10.1029/WR021i011p01591. [ CrossRef ] [ Google Scholar ]
- Dahl K. The effect of acid water on trout fry. Salmon and Trout Magazine. 1927; 46 :35–43. [ Google Scholar ]
- Dannevig A. Influence from precipitation on the acidity of freshwater and fish populations. Jeger og Fisker. 1959; 3 :116–118. [ Google Scholar ]
- Driscoll CT, Baker JP, Bisogni JJ, Jr, Scofield CL. Effects of aluminium speciation on fish in dilute acidified waters. Nature. 1980; 284 :161–164. doi: 10.1038/284161a0. [ CrossRef ] [ Google Scholar ]
- Driscoll CT. A procedure for the fractionation of aqueous aluminium in dilute acidic waters. International Journal of Environmental Analytical Chemistry. 1984; 16 :267–283. doi: 10.1080/03067318408076957. [ CrossRef ] [ Google Scholar ]
- Grennfelt P, Engleryd A, Forsius M, Hov Ø, Rohde H, Cowling E. Acid rain and air pollution: 50 Years of progress in environmental science and policy. Ambio. 2020; 49 :849–864. doi: 10.1007/s13280-019-01244-4. [ PMC free article ] [ PubMed ] [ CrossRef ] [ Google Scholar ]
- Henriksen A, Lien L, Traaen T, Sevaldrud I, Brakke DF. Lake acidification in Norway—Present and predicted chemical status. Ambio. 1988; 17 :259–266. [ Google Scholar ]
- Henriksen A, Lien L, Rosseland BO, Traaen T, Sevaldrud I. Lake acidification in Norway—Present and predicted fish status. Ambio. 1989; 18 :314–321. [ Google Scholar ]
- Henrikson, L., and Y.W. Brodin, eds. 1995. Liming of acidification surface waters. A Swedish synthesis , 457. Berlin: Springer. ISBN-13:978-3-642-79311-0.
- Hesthagen T, Fjellheim A, Schartau AK, Wright RF, Saksgård R, Rosseland BO. Chemical and biological recovery of Lake Saudlandsvatn, a highly acidified lake in southernmost Norway, in response to decreased acid deposition. Science of the Total Environment. 2011; 409 :2908–2916. doi: 10.1016/j.scitotenv.2011.04.026. [ PubMed ] [ CrossRef ] [ Google Scholar ]
- Jeziorski A, Yan ND, Paterson AM, DeSellas AM, Turner MA, Jeffries DS, Keller B, Weeber RC, et al. The widespread treat of calcium decline in fresh waters. Science. 2008; 322 :1374–1377. doi: 10.1126/science.1164949. [ PubMed ] [ CrossRef ] [ Google Scholar ]
- Keller W, Heneberry J, Edwards BA. Recovery of acidified Sudbury, Ontario, Canada, lakes: A multi-decade synthesis and update. Environmental Reviews. 2019; 27 (1):1–16. doi: 10.1139/er-2018-0018. [ CrossRef ] [ Google Scholar ]
- Korman J, Marmorek DR, Lacroix GL, Amiro PG, Ritter JA, Watt WD, Cutting RE, Robinson DCE. Development and evaluation of a biological model to assess regional-scale effects of acidification on Atlantic salmon ( Salmo salar ) Canadian Journal of Fisheries and Aquatic Sciences. 1994; 51 :662–680. doi: 10.1139/f94-067. [ CrossRef ] [ Google Scholar ]
- Kroglund F, Rosseland BO, Teien H-C, Salbu B, Kristensen T, Finstad B. Water quality limits for Atlantic salmon ( Salmo salar L.) exposed to short term reductions in pH and increased aluminium simulating episodes. Hydrology and Earth System Science. 2008; 12 :491–507. doi: 10.5194/hess-12-491-2008. [ CrossRef ] [ Google Scholar ]
- Leivestad H, Muniz IP. Fish kill at low pH in a Norwegian river. Nature. 1976; 259 :391–392. doi: 10.1038/259391a0. [ PubMed ] [ CrossRef ] [ Google Scholar ]
- Maas, R., and P. Grennfelt, eds. 2016. Towards Cleaner Air. Scientific Assessment Report 2016 . Oslo: EMEP Steering Body and Working Group on Effects of the Convention on Long-Range Transboundary Air Pollution. https://www.unece.org/fileadmin/DAM/env/lrtap/ExecutiveBody/35th_session/CLRTAP_Scientific_Assessment_Report_-_Final_20-5-2016.pdf .
- Nilsen TO, Ebbeson LOE, Kverneland OG, Kroglund F, Finstad B, Stefansson SO. Effects of acid water and aluminium exposure on gill Na + , K + –ATPase α-subunit isoforms, enzyme activity, physiology and return rates in Atlantic salmon (S almo salar L.) Aquatic Toxicology. 2010; 97 :250–259. doi: 10.1016/j.aquatox.2009.12.001. [ PubMed ] [ CrossRef ] [ Google Scholar ]
- Raddum, G., and B.O. Rosseland. 2005. Chapter 9: Dynamic biological response models—An overview. In Proceedings of the 20th meeting of the ICP Waters Programme Task Force , Falun, Sweden, October 18–20, 2004. NIVA Report 5018-2005. ICP Waters report 80/2005, eds. H. deWitt and B.L. Skjelkvåle, 45–54.
- Rosseland, B.O., I. Sevaldrud, D. Svalastog, and I.P. Muniz. 1980. Studies on freshwater fish populations—Effects of acidification on reproduction, population structure, growth and food selection. In Ecological impact of acid precipitation . SNSF-project FA 105/80, eds. A. Tollan, and D. Drabløs, 336–337.
- Rosseland BO, Skogheim OK. A comparative study on salmonid fish species in acid aluminium-rich water. II. Physiological stress and mortality of one and two year old fish. Report of Institute of Freshwater Research Drottningholm. 1984; 61 :186–194. [ Google Scholar ]
- Rosseland BO, Blakar I, Bulger A, Kroglund F, Kvellestad A, Lydersen E, Oughton DH, Salbu B, et al. The mixing zone between limed and acidic river waters: Complex aluminium chemistry and extreme toxicity for Salmonids. Environmental Pollution. 1992; 78 :3–8. doi: 10.1016/0269-7491(92)90003-S. [ PubMed ] [ CrossRef ] [ Google Scholar ]
- Schofield CL. Effects on fish. Ambio. 1976; 5 :228–230. [ Google Scholar ]
- Schofield, C.L. 1977. Acid snow-melt effects on water quality and fish survival in the Adirondack Mountains of New York State . Res. Proj. Tech. Comp. Rep. Project A-072-NY. Ithaca: Cornell University.
- Wright, R.F., and E. Snekvik. 1977. Chemistry and fish populations in 700 lakes in southernmost Norway . SNSF-project, TN 17/77.
Thank you for visiting nature.com. You are using a browser version with limited support for CSS. To obtain the best experience, we recommend you use a more up to date browser (or turn off compatibility mode in Internet Explorer). In the meantime, to ensure continued support, we are displaying the site without styles and JavaScript.
- View all journals
- Explore content
- About the journal
- Publish with us
- Sign up for alerts
- Open access
- Published: 12 February 2024
Impact of simulated acid rain on chemical properties of Nyalau series soil and its leachate
- Mohamad Hilmi Ibrahim 1 ,
- Susilawati Kasim 2 ,
- Osumanu Haruna Ahmed 3 ,
- Mohd. Rashid Mohd. Rakib 4 ,
- Nur Aainaa Hasbullah 4 &
- Md. Tariqul Islam Shajib 5 nAff6
Scientific Reports volume 14 , Article number: 3534 ( 2024 ) Cite this article
1570 Accesses
1 Citations
Metrics details
- Environmental sciences
Greenhouse gases can cause acid rain, which in turn degrades soil chemical properties. This research was conducted to determine the effects of simulated acid rain (SAR) on the chemical properties of Nyalau series ( Typic paleudults ). A 45-day laboratory leaching and incubation study (control conditions) was conducted following standard procedures include preparing simulated acid rain with specific pH levels, followed by experimental design/plan and systematically analyzing both soil and leachate for chemical changes over the 45-day period. Six treatments five of which were SAR (pH 3.5, 4.0, 4.5, 5.0, and 5.5) and one control referred to as natural rainwater (pH 6.0) were evaluated. From the study, the SAR had significant effects on the chemical properties of the soil and its leachate. The pH of 3.5 of SAR treatments decreased soil pH, K + , and fertility index. In contrast, the contents of Mg 2+ , Na + , SO 4 2− , NO 3 − , and acidity were higher at the lower SAR pH. Furthermore, K + and Mg 2+ in the leachate significantly increased with increasing acidity of the SAR. The changes in Ca 2+ and NH 4 + between the soil and its leachate were positively correlated (r = 0.84 and 0.86), whereas the changes in NO 3 − negatively correlated (r = − 0.82). The novelty of these results lies in the discovery of significant alterations in soil chemistry due to simulated acid rain (SAR), particularly impacting soil fertility and nutrient availability, with notable positive and negative correlations among specific ions where prolonged exposure to acid rain could negatively affect the moderately tolerant to acidic and nutrient-poor soils. Acid rain can negatively affect soil fertility and the general soils ecosystem functions. Long-term field studies are required to consolidate the findings of this present study in order to reveal the sustained impact of SAR on tropical forest ecosystems, particularly concerning soil health, plant tolerance, and potential shifts in biodiversity and ecological balance.
Similar content being viewed by others
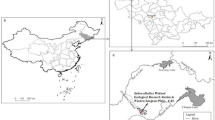
The effects of soil freeze–thaw processes on water and salt migrations in the western Songnen Plain, China
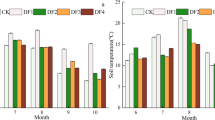

Effects of rainfall amount and frequencies on soil net nitrogen mineralization in Gahai wet meadow in the Qinghai-Tibetan Plateau
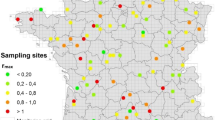
Management of soil pH promotes nitrous oxide reduction and thus mitigates soil emissions of this greenhouse gas
Introduction.
Acid deposition poses several threats to ecosystems by affecting plant health, diversity and structure, including processes and functions in the ecosystem 1 , 2 . Acid deposition is defined as accumulation of undesired chemical compounds in the atmosphere at toxic concentrations 3 . Acid deposits are materials (solids, liquids and gases) occurring in excess quantities from the average amount and present at the lowest layer of the atmosphere 4 . Acid deposition in the atmosphere can be attributed to diverse chemical compounds originating from fossil fuel combustion, agriculture, mining, and manufacturing activities. Acid deposition is a global threat that has been shown to result in various environmental and human health hazards such as depleting essential nutrients and increasing toxic metals, which can lead to reduced plant growth and biodiversity 5 , 6 , 7 .
Acid deposits refer to rain, snow, fog, particulates, and gases, whereas acid rain only refers to rainwater at pH below 5.6 8 , 9 . Acid rain mainly consists of sulfur dioxide (SO 2 ) and nitrogen oxides (NOx) forming acidic compounds, whereas other greenhouse gases like Cl − and CO 2 , linked to climate change and global warming. These gases undergo complex chemical reactions in the atmosphere after which they fall to the earth’s surface as wet or dry deposition 10 . According to Zhang et al. 11 , acid rain with a pH of 5.6 is deemed normal as atmospheric CO 2 at a pressure of 101 kPa and temperature of 20 °C lowers rainwater pH from 7 to 5.6. This normalcy shifts when gases like N 2 O and SO 2 contribute to a further decrease in pH below 5.6 due to increasing hydrogen ion concentrations.
Soil fertility and soil physico-chemical properties such as soil nutrients for plant growth and production, are commonly affected by prolonged exposure to acid deposition 12 , 13 . Several scientific reports have demonstrated that acid deposition may disrupt nutrient cycling in soil habitats, particularly by deteriorating soil physico-chemical properties, especially its fertility 14 , 15 , 16 , 17 . For example, soil nutrient leaching in White Mountain National Forest in the Central New Hampshire, US, resulted from acid deposition 18 . In addition, other studies on the impact of acid deposition on ecosystems have revealed that this phenomenon affects species richness and diversity 19 , 20 and hydrological cycle, including water quality 21 .
More than that, this acidic precipitation lowers the soil pH, a process termed soil acidification. Research by Yang et al. 22 shows that acidification leads to nutrient leaching, particularly of calcium and magnesium, while increasing the solubility and toxicity of metals like aluminum and lead. This results in reduced soil fertility and damage to plant root systems, adversely affecting plant growth and crop yields, as noted by Dai et al. 23 . Furthermore, soil acidification disrupts microbial communities, impacting critical processes like decomposition and nutrient cycling 24 .
Soil leaching is defined as the movement of nutrients from the upper soil profile to its lower depths 25 . Leaching typically causes soil pH to decrease with decreasing base cations concentrations. When acid deposition occurs, there is an increase in the solubility of heavy metals and Al mobilization in soils 26 . To this effect, accumulation of H + ions reduces soil pH while increasing the solubility of heavy metals and Al mobilization. The leaching of macronutrients occurs due to the replacement of H + ions by acid rain, which increases soil acidity to levels that compromise fertility 27 . This phenomenon of soil acidification is not just theoretical; it has been observed on a large scale, for instance, in Southern China, where soil acidification was documented after 20 years of continuous exposure to acid rain 28 , 29 .
The mineral acid soils in Sarawak, Malaysia belong to four major series, namely Bekenu, Nyalau, Merit, and Stom series 30 . Nyalau series are the soils contaminated with eroded material from upslope areas with high content of sesquoxides 31 . According to Tan et al. 32 , Nyalau series belongs to Typic paleudults , therefore it is classified as acidic soils, with pH between 4.3 and 4.8 and CEC values below 24 cmol kg −1 . The textural class of these soils is sandy clay loam with brownish yellow to yellow colouration. In Malaysia, the cumulative acid loading from the atmosphere to terrestrial ecosystems has been on the increase since 2010–2019 33 . As a result, SO 2 and N 2 O composition in some states in Malaysia are 0.66 and 0.17 ppm, respectively 34 , while the pH of rainwater in selected industrial areas in Malaysia have reached 4.32 35 . EANET 36 reported the annual rainwater pH at Petaling Jaya, Tanah Rata, Danum Valley, and Kuching, Malaysia as 4.15, 5.01, 5.21, and 5.43, respectively.
According to Department of Environment of Malaysia (unpublished data), the total SO 2 emission in Malaysia was 0.25 ppm in 2020. Although this value is less than those of other countries, precautions should be taken to manage this occurrence to prevent it from increasing in severity. Although there are studies on simulated acid rain on soils in other areas 26 , 27 , 37 , 38 , there is dearth of information on the effect of SAR in Nyalau soils and its leachate. This study is important because the Nyalau series is not widely known. The Nyalau series, a tropical soil, is unique for its high sand content, strong acidity, and poor nutrient retention, making it challenging for agriculture but crucial for soil studies. Its characteristics and study are valuable for soil science and geology and contribute to our understanding of soil composition and geological history in certain regions facing the problem of acid rain.
This study embodies three objectives that significantly centre on the effects of simulated acid rain on chemistry and properties of Nyalau series ( Typic paleudults ) soil and its leachate. Firstly, the objective of the study is to identify the possibility of significant differences in soil fertility index and soil evaluation factor of Nyalau series soils when exposed to SAR. Secondly, the objective of the study seeks to ascertain the possibility of significant differences in the chemical properties of Nyalau series soils and its leachate when exposed to SAR. Finally, the study strives to examine the correlation and cluster between soil and leachate chemical properties across SAR pH. Soil fertility index and soil evaluation factor were used as key indicators to determine the effects of SAR on the fertility of Nyalau series.
Materials and methods
Soil collection, preparation and analysis.
The topsoil (0–20 cm depth) of Nyalau series from the undisturbed/minimal human intervention or alteration agricultural field, Universiti Putra Malaysia, Bintulu Campus, Sarawak, (03° 12.721′ N, 113° 4.477′ E) was collected from 10 points apart then bulked together using a spade until approximately 50 kg of soil (Fig. 1 ). The soil was collected in transparent plastic bags and transported to the laboratory, where it was air-dried in room temperature for a few days to a week and sieved to pass a 2 mm mesh. The initial chemical properties of the soil samples were determined using standard procedures as adopted from Tan 39 , for pH, Allen et al. 40 for CEC, K + , Ca 2+ , Na + , Mg 2+ and P, Keeney and Nelson method 41 for NO 3 − and NH 4 + , Rowell 42 , for acidity, Al 3+ , and H + and Cheftetz et al. 43 for soil organic matter and total organic carbon (Table 1 ).
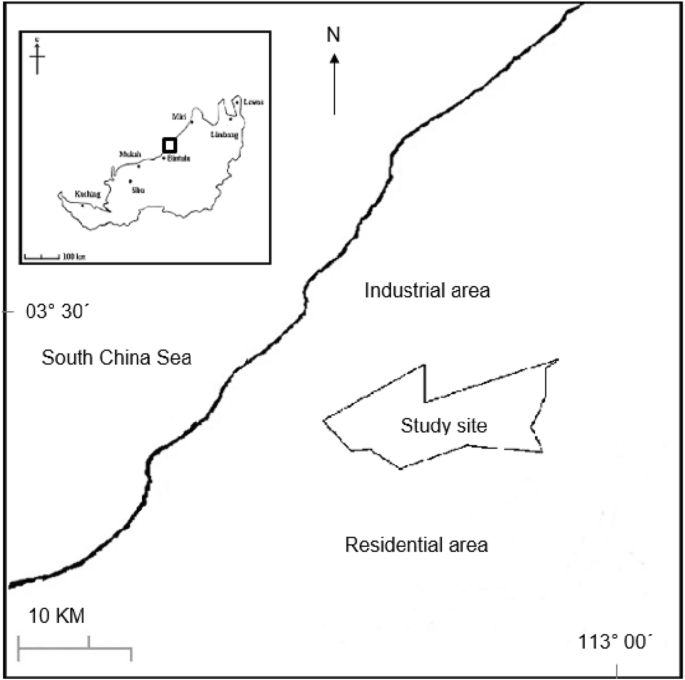
Location of the soil sampling sites in Universiti Putra Malaysia, Bintulu, Sarawak. Sampling were conducted ramdomly from several points in study sites.
Leaching experiment design and setup
The experiment was conducted using 18 polyethylene soil columns having 16 cm diameter and 28 cm depth and fitted with 26 holes (3 mm in diameter) at the bottom. The holes evenly distributed in a uniform circular pattern for optimal drainage. Analytical grade tissue paper was placed at the bottom of the column (to prevent soil loss) after which the column was filled with 270 g soil. Soil bulk density 44 at the the undisturbed agricultural field site was first quantified, and the value was used to estimate the quantity of soil (i.e. soil without water content) to be used/ correspond with soil compaction in each column. This resulted in each empty soil column being filled with 270 g of air-dried soil, to simulate the natural condition of the Nyalau soil at the study sites. A tray was placed underneath each soil column to collect leachate.
Treatment preparation and application
The soil in the columns were exposed to SAR by applying water with pH of 3.5, 4.0, 4.5, 5.0, 5.5, and 6.0.The pH 6.0 served as natural rainwater (control treatment). The selected SAR pH values of 3.5, 4.0, 5.0 and 5.5 were chosen to represent a range of acid deposition scenarios, from extreme to more moderate conditions enabling the study of soil responses under different environmental stress levels. A pH of 3.5 represents the worst-case scenario for acid rain worldwide and indicates the most severe environmental impacts. The other values, 4.0, 5.0 and 5.5, serve as projections ranging from extreme acidity to normal rainwater conditions. This range provides a comprehensive understanding of how different acidity levels can affect ecosystems, making the study relevant to real-world scenarios.
Water with varying pH levels was prepared by adding 0.1 molar H 2 SO 4 and HNO 3 in a 3:2 volume-to-volume ratio to distilled water, after which the pH was adjusted to the desired level 45 . The chemical properties of the SAR are presented in Table 2 . Each treatment had three replications; thus, the total experimental units were 18. The experimental units were arranged in a completely randomized design (CRD) with aset up of 6 m × 4 m room having a 76% relative humidity and a temperature of 21 °C. Approximately 318 mL of SAR were applied to each soil column and this volume was based on the field capacity of the soil using a drip system operating at a flow rate of 2.71 mL s −1 . The soil in the leaching columns were exposed to the SAR once every three days for 45 days (15 applications in total) at 8 pm. SAR application interval was based on average monthly/yearly rainfall events in Bintulu (MMD, Unpublished data), Sarawak, Malaysia. At the end of the experiment, the soil and its leachate were collected for chemical analysis.
Analysis of selected chemical properties of Nyalau series
After the incubation experiment, the soil samples in the columns were collected, air-dried, and sieved to pass through a 2 mm sieve for chemical analysis. The soil pH was measured in distilled water at a soil/water ratio of 1:2.5 39 . The CEC in mg/kg of the soil was determined using 1 M ammonium acetate buffered at pH 7. Exchangeable base cations were extracted using 100 mL of 1 M ammonium acetate buffered at pH 7, after which the filtrates were analyzed to determine the concentrations of exchangeable K, Ca, Na, and Mg using Flame Atomic Absorption Spectrometery (AAS) (iCE 300, Thermo Fisher Scientific®, NSW, Australia). The concentration of available P in the soil filtrate was determined using a UV–VIS spectrophotometer (UV-1800, Shimadzu, Kyoto, Japan) operated at 820 nm wavelength after extracting the soils using Bray’s solution (0.03 N of ammonium fluoride, NH 4 Fl in 0.025 N of HCI) 40 .
Soil available NO 3 − and NH 4 + were determined using Keeney and Nelson method 41 followed by steam distillation 40 . Soil acidity, Al 3+ , and H + were determined using the titration method 42 . The soil available sulfate was extracted using 0.5 M of NaHCO 3 , after which the extract was analyzed using ion chromatograph IC-MS (AI300, PerkinElmer Inc., USA). The loss-on-ignition (LOI) method was used to determine soil organic matter and total organic carbon 43 . A 5 g of oven-dried sample (dried at 6 °C for 24 h) was weighed into a porcelain dish, placed in a muffle furnace, and heated at 300 °C for 1 h to determine soil organic matter content.
The Soil Fertility Index (SFI; Eq. 1 ) and Soil Evaluation Factor (SEF; Eq. 2 ) of Nyalau series were calculated using the formulas of Moran et al. 46 and Lu et al. 47 , respectively.
Analysis of selected chemical properties of leachate
The leachate pH was measured using a pH meter (S220, Thermo Fisher Scientific®, USA) whereas electric conductivity (EC), salinity, and total dissolved solids were determined using EC meter (S70, Mettler Toledo Co., USA). Exchangeable cations were determined using AAS (AA5000, PerkinElmer Inc., USA) whereas nitrite (NO 2− ), phosphate (PO 4 3− ), nitrate (NO 3 − ), and ammonium (NH 4 + ) were measured using UV-spectrophotometer (DR 2010, Hach©, USA).
Statistical analysis
One-way analysis of variance (ANOVA) was used to detect between-treatment before after which treatment means were compare dusing Duncan’s New Multiple Range Test (post-hoc analysis) at p ≤ 0.05. Pearson’s correlation analysis was conducted to determine the relationship between the chemical properties of the soil and its leachate. In addition, Pearson’s correlation analysis was performed to analyze the response of soil and leachate variables across the pH of SARtreatment. The statistical analysis was performed using SAS version 9.4 48 .
Hierarchical cluster analysis (CA) was performed to find out similar groups of soil properties depending of origin (one soil type) and concentration. The CA was performed on the various chemical properties simulated acid rain and leachate, using a distance cluster between 15 and 20 49 , 50 . A distance criterion between two variables express how closely correlate within the group. Two cluster analyses by means of hierarchical dendrograms were performed by using SPSS 28.0 (IBM SPSS Statistics, USA) applied to the SAR and soil leachate. All these analysis collectively allowed for interpreting how SAR treatments affected soil and leachate composition, guiding conclusions on acid rain's impact.
Effects of simulated acid rain treatments on soil properties
Soil pH, K + , SFI and SEF significantly decreased with increasing acidity of SAR. As example, significant decrease in soil pH and SFI (2.21% reduction) were recorded when the soil was exposed to SAR with pH 4.0 and pH 3.5. Potassium ions in the soil decreased from 0.037 to 0.019 mg kg −1 (48.64% reduction). Contrastingly, Mg 2+ , Na + , SO 4 2− , NO 3 − , and soil acidity significantly increased with increasing acidity of the SAR. Relative to control (natural rainwater) the soil which was exposed to SAR with a pH of 3.5 increased Mg 2+ , Na + , SO 4 2− , NO 3 − , and acidity by 193.33%, 101.30%, 46.2%, 18.65% and 22.02%, respectively. Furthermore, significant reduction was observed in the level of Al 3+ , H + , and Zn 2+ in soils exposed to SAR with pH 5.0. However, the K + , Ca 2+ and Zn 2+ cations decreased with increasing acidity of SAR (pH 4.0 and below). Similarly, available P in the soil significantly reduced from 1.62 mg kg −1 at SAR of pH 6.0 to 1.43 mg kg −1 at pH of 4.5, whereas SAR with pH 3.5 recorded an available P value of 1.57 mg kg −1 . Furthermore, the Soil CEC, Ca 2+ , Fe 2+ , and NH 4 + fluctuated across the SAR treatments whereas SEF generally remained unchanged (Table 3 ).
Effects of simulated acid rain (SAR) treatments on leachate properties
There was significant increase in K + and Mg 2+ concentrations in leachate as SAR levels were decreased from 4.0 to 3.5 (Table 4 ). K + ions increased from 5.62 mg L −1 (SAR at pH 6.0) to 6.65 mg L −1 (SAR at pH 3.5) whereas Mg 2+ ions increased from 0.72 mg L −1 (SAR at pH 6.0) to 0.83 mg L −1 (SAR at pH 3.5). The Na + in the leachate significantly increased from 1.92 to 4.63 mg L −1 with increasing SAR acidity. The continued acidification reduced Na + in the leachate to 2.68 mg L −1 (pH 3.5). The leachate of PO 4 2− concentration did not significantly differences regardless of SAR pH. Other variables fluctuated across the SAR pH (Table 4 ).
Relationship between soil and leachate properties
The relationship between the soil and its leachate properties was analyzed to determine acid deposition's effect on nutrients leaching or retention by the Nyalau series. The Pearson’s correlation analysis revealed that the changes in Ca 2+ and NH 4 + between the soil and its leachate positively correlated and the Pearson’s correlation coefficient (r) values were 0.84 and 0.86, respectively. However, the NO 3 − in the soil and its leachate was correlated negatively (r = − 0.82). The correlation for the other variables were not significant (Fig. 2 ).

Trends of selected soil and leachate properties of Nyalau series ( Typic paleudults ) soil after exposure to simulated acid rain. Correlation analysis was conducted, and the relationship was indicated by the Pearson’s correlation coefficient (r) and probability level significant at p ≤ 0.05.
Cluster analysis for soil and leachate properties
The findings of CA are presented in two hierarchical dendrograms representing soil (Fig. 3 A) and leachate (Fig. 3 B). The dendrogram for soil comprise 3 clusters (Fig. 3 A). NH 4 + and NO 3 − comprise first cluster and SO 4 2− , SFI, CEC and SEF comprise the second cluster and are associated with a low distance criterion around 1. The rest of the chemical properties acidity, pHwater, pH KCl , H + , Na + , Ca 2+ , Mg 2+ , Al 3+ , Cu 2+ , K + , Zn 2+ and Fe 2+ form the third cluster and they are associated in a very low distance at around 1. In Fig. 3 B, the first cluster contains Cu 2+ ,NO 3 − ,PO 4 2− , NO 2 − , NH 4 + , Mg 2+ , Cl − , Salinity and S 2− and they are positioned at a very low distance around 1. In the second cluster, pH, K + , Ca 2+ and Fe 2+ form a group with a distance of CA below 3 whereas electrical conductivity (EC) is placed separately than cluster 1 and 2 with a high distance criteria at 25.
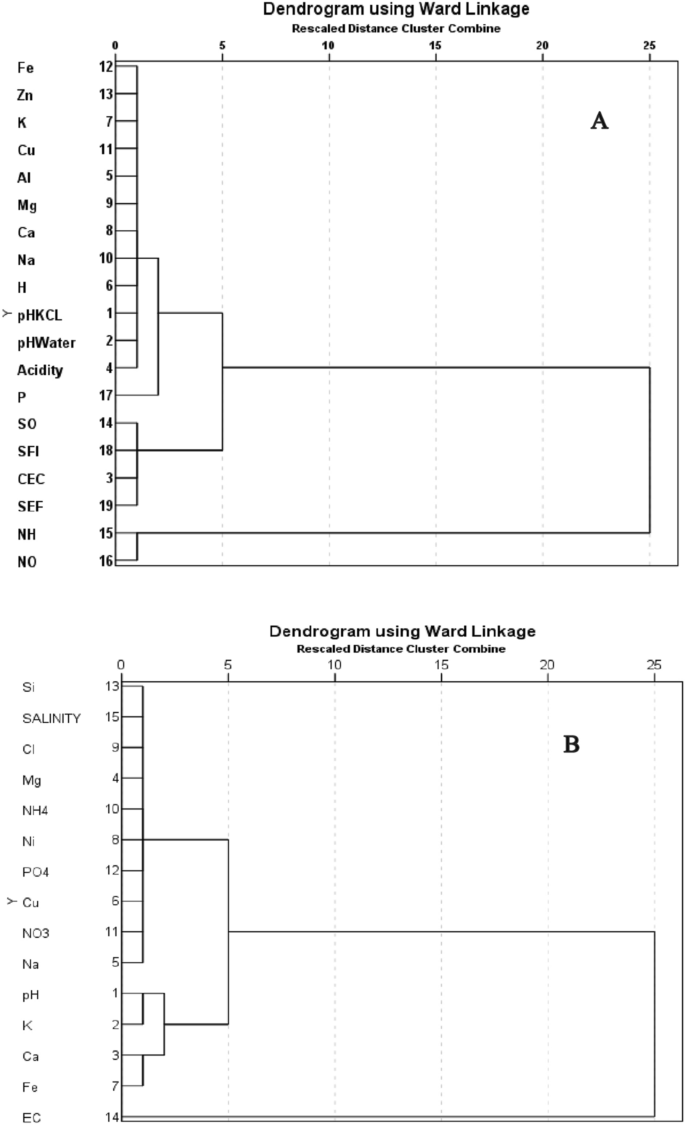
Hierarchical dendrogram for chemicals properties found in soil ( A ) and leachate ( B ) using Ward’s method.
Simulated acid rain and natural rainwater on soil properties of Nyalau series
Generally, the SAR treatments, including control, initially decreased soil pH (4.84). The pH ofsoilwith SAR pH below 4.5 (Table 3 ) was significantly low and this may cause reduction in the soil fertility index. Additionally, the soil exchangeable Al 3+ and H + were significantly increased because aluminium hydrolysis increases with increasing soil acidity. For example, a complete hydrolysis of one mole Al 3+ ions produces three moles H + ions to further decrease soil pH and this chemical reaction reduces soil CEC. This finding corroborates that of Zhang et al. 11 who explained that acid rain increases soil acidity and H + ions, leading to loss of mineral structure. Loss in mineral structure has been implicated in soil fertility decline. Wei et al. 51 also reported that acid rain reduces soil fertility because it reduces soil pH and cation retention capacity.
Although soils have strong pH buffering capacity, the SO 4 2− , H + , NO 3 − , and NH 4 + in acid rain favour the dominance of H + ions on the soil exchange sites such that soil CEC is disproportionately dominated by hydrogen ions instead of base cations, especially K. Significant leaching of K + in soil with SAR at pH 3.5 was expected due to the high acidity ofthis treatment. The dominance of stronger complementary adsorbed cations at the soil exchange sites could partly explain the loss of K into the leachate 26 , 51 . Acidic rainwater gradually diminishes exchangeable cations in topsoil because it facilitates changes in the nutrient pool and leaching of nutrients from the soil profile 44 . This observation is supported by Zhang et al. 11 , who reported significantly higher effluent K + concentration of SAR at pH 3 and below.
The low SAR pH were responsible for low variations in Ca 2+ , Mg 2+ , Na + , acidity, NH 4 + , and SO 4 2− in the soils compared with soil treated with natural rainwater. This finding is similar to that of Rampazzo and Blum 52 who reported that exposing parent rock material to acid rain, inspite of having 30–80% calcite, reduced CEC and base saturation, particularly Ca contents. This suggests the fertility and the overall productivity of soils will decline if they are exposed to acid deposition for a long time. A notable reduction in soil pH enhances the solubility of aluminium, consequently elevating the concentration of Al 3+ ions in the leachate. This finding aligns with Mulder et al. 53 observation, where they reported the phytotoxic effects due to increased dissolution of Al 3+ in soil leaching experiments conducted in both the Netherlands and New Hampshire, USA.
Soil Zn 2+ solubility has increase with decreasing pH (3.5–6.0) because the solubility of Zn decreases with increasing soil pH. High levels of soil contamination, with soluble Zn 2+ reaching 19,570 mg/kg and Cu 2+ up to 322.4 mg/kg 38 , enhance the phytoavailability of heavy metals 14 , leading to increased uptake by plants. The very acidic SAR treatments increased soil exchangeable sulfate 46.20% because of sulfate adsorption to form sulfuric acid which upon decomposing, releases H + and SO 4 2− ion. This reaction occurs at low soil pH 54 . Soil available ammonium increase with increasing acidity of SAR. The increase in NH 4 + concentration is consistent with the report of Johnson et al. 55 , who demonstrated that acid rain increases nitrogen mineralization and nitrification in forest soils.
Simulated acid rain and natural rainwater on leachate properties of Nyalau series
Leachate pH was highest with lower SAR pH compared with natural rainwater. According to De Walle et al. 56 , the increase in leachate pH was due to the accumulation of base cations, especially Ca 2+ and Mg 2+ (Table 4 ). This result also explains the movement of Ca 2+ and Mg 2+ down the soil profile, corroborating the results of Zhang et al. 11 on Latosol of Southern China. Low electrical conductivity and salinity values were recorded with lower SAR pH because the accumulation of base cations in the leachate increased the EC of the soil. The base cations in the leachate of the lower SAR pHs were higher than with the natural rainwater (Table 4 ). This present study suggests that acid rain causes leaching of the bases and this could cause ground water pollution through enrichment through lost nutrients from the soil profile.
Overall implication of varying simulated acid rain on soil and leachate properties
The incubation of Nyalau soil series with SAR generally had negative effects on pH, K, Fe and NO 3 of the soil and its leachate. This includes a decrease in soil pH, indicating increased acidity, and reductions in the concentrations of potassium (K), iron (Fe), and nitrate (NO 3 ) in the soil. The results indicate that when the pH of SAR decreases from 6.0 to 3.5, the pH and potassium (K) content in the soil and leachate also decrease. This is confirmed by the data in Tables 3 and 4 . The increased soil acidity with the low pH SAR is related to high H + concentration. The accumulation of H + from acid deposition increased the soil acidity 27 , 51 . Increase in the soil acidity through acid deposition might have affected the solubility of heavy metals such as Fe, as observed in the soil with low SAR pH. Furthermore, acidic pollutants can cause P fixation by Al and Fe in soils 57 and this explain low available P content in this present study (Table 3 ). The positive relationship between soil and leachate for Ca 2+ and NH 4 + was due to insufficient time (45 days) for leaching of cations from the soil. This slower leaching rate is due to the complex interplay of physical, chemical, and environmental factors within the soil. Essentially, these ions are not as readily mobilized or washed out of the soil compared to other elements, indicating a delayed response to the leaching process influenced by soil composition and conditions 58 .
More than that the similarities of SO 4 2− , SFI, CEC and SEF in hierarchical dendrograms of soil have shown that the fertility of Nyalau series soil have also influenced by SO 4 2− . We believe it was happening because of the presence of sulphuric acid (H 2 SO 4 ) from SAR treatments. Our argument is consistent with finding in Table 3 recorded higher SO 4 2− content under low SAR pH treatments. Similar study reported by Hüttl and Frielinghaus 59 in Eastern, Germany who shows that air pollutant or acid rain content with H 2 SO 4 could reduce the soil fertility accelerating soil acidification. In the leachate hierarchical dendrograms, there are similarities of soil water pH, K and Ca. This results reliable comes from accumulation of base cation while exposure to SAR as discussing in previous section.
Management implication of simulated acid rain on soil and leachate properties
Even with a short incubation study (45 days), we found a 2.21% reduction in the fertility of Nyalau series and 5.43% reduction in soil acidity as compared when exposed to natural rainwater (control treatment). The lower SFI of the soil in the present study (11.94) compared with research on a secondary forest in Lundu, Sarawak, by Perumal et al. 60 where SFI of 19.63 was recorded, indicates the prolonged negative impact of acid rain on soil fertility. These results showed that acid rain impacted soil and leachate properties, and it is possible that prolonged acid rain exposure will further modify soils of the Nyalau series detrimentally.
Therefore, for a comprehensive understanding of acid rain's effects, a long term study, possibly over a year, is recommended. This allows for observing long-term ecological and soil changes. Complementing this with advanced modeling would provide a holistic view, predicting future impacts and aiding in effective environmental management strategies, crucial for sustaining ecosystems and agricultural productivity in the face of environmental changes. Therefore, understanding the prolonged impacts of acid rain on soil properties is not only an ecological necessity but also crucial for human sustainability.
The study focused on the impact of simulated acid rain (SAR) on the Nyalau series soil, examining a range of acidity levels from less acidic (pH 5.5 and 5.0) to more acidic (pH 4.0 and 3.5). It was found that with increasing acidity, especially at pH 3.5, the soil experienced significant changes: a decrease in pH, potassium, and fertility, and an increase in magnesium, sodium, sulfate, nitrate, and overall acidity. The leachate from the soil also showed increased levels of potassium and magnesium, indicating a leaching effect that could lead to nutrient deficiencies for plants. The study also noted a positive correlation between changes in calcium and ammonium levels in both soil and leachate, and a negative correlation in nitrate levels, highlighting complex interactions between soil acidity and nutrient dynamics.
The results of our study have important practical implications for both land management and environmental policy. Land managers are suggested to regularly conduct comprehensive soil health assessments, especially in areas vulnerable to acid rain or soil acidification. These assessments should go beyond simply measuring pH and consider chemical properties such as K + , Mg 2+ and NO 3 − to inform soil treatment plans. In terms of policy, the observed deleterious effects of acidic treatments on soil properties call for stricter pollution regulations to curb acid rain, and the data could further guide the establishment of safe areas for agriculture and forestry based on the resilience of soils to acidification.
Data availability
The datasets generated and/or analysed during the current study are available from the corresponding author upon reasonable request.
Irvine, I. C., Greaver, T., Phelan, J., Sabo, R. D. & Van Houtven, G. Terrestrial acidification and ecosystem services: Effects of acid rain on bunnies, baseball, and Christmas trees. Ecosphere 8 , e01857. https://doi.org/10.1002/ecs2.1857 (2017).
Article Google Scholar
Debnath, B. & Ahammed, G. J. Effect of acid rain on plant growth and development: Physiological and molecular interventions. In Contaminants in Agriculture (eds Naeem, M. et al. ) 103–114 (Springer, 2020). https://doi.org/10.1007/978-3-030-41552-5_5 .
Chapter Google Scholar
NADP (National Atmospheric Deposition Program). National trends network. Illinois State Water Survey, Champaign, IL, U.S.A. Retrieved from http://nadp.sws.uiuc.edu/NTN (Accessed 4 December 2019) (2005).
US-EPA (United State Environmental Protection Agency). Report of Acid Rain Program Progress 2004 (Washington, United State, 2005).
Bhatti, N., Streets, D. G. & Foell, W. K. Acid rain in Asia. Environ. Manag. 16 , 541–562. https://doi.org/10.1007/BF02394130 (1992).
Article ADS Google Scholar
Burns, D. A., Aherne, J., Gay, D. A. & Lehmann, C. Acid rain and its environmental effects: Recent scientific advances. Atmos. Environ. 146 , 1–4. https://doi.org/10.1016/j.atmosenv.2016.10.019 (2016).
Article CAS ADS Google Scholar
Nicoletti, G., Arcuri, N., Nicoletti, G. & Bruno, R. A technical and environmental comparison between hydrogen and some fossil fuels. Energy Convers. Manag. 89 , 205–213. https://doi.org/10.1016/j.enconman.2014.09.057 (2015).
Charola, A. E. & Ware, R. Acid deposition and the deterioration of stone: A brief review of a broad topic. Geol. Soc. Lond. Spec. Publ. 205 , 393–406. https://doi.org/10.1144/GSL.SP.2002.205.01.28 (2002).
Dubey, S. Acid rain-the major cause of pollution: its causes, effects and solution. Int. J. Sci. Eng. Technol. 2 , 772–775 (2013).
Google Scholar
Saxena, P. & Sonwani, S. Criteria air pollutants: Chemistry, sources, and sinks. In Criteria Air Pollutants and Their Impact on Environmental Health (eds Saxena, P. & Sonwani, S.) 7–48 (Springer, 2019). https://doi.org/10.1007/978-981-13-9992-3_2 .
Zhang, X., Jiang, H., Jin, J., Xu, X. & Zhang, Q. Analysis of acid rain patterns in northeastern China using a decision tree method. Atmos. Environ. 46 , 590–596. https://doi.org/10.1016/j.atmosenv.2011.03.004 (2012).
Ouyang, X. J. et al. Effect of simulated acid rain on potential carbon and nitrogen mineralization in forest soils. Pedosphere 18 , 503–514. https://doi.org/10.1016/S1002-0160(08)60041-7 (2008).
Article CAS Google Scholar
Lal, N. Effects of acid rain on plant growth and development. J. Sci. Technol. 11 , 85–108 (2016).
Kim, A. Y., Kim, J. Y., Ko, M. S. & Kim, K. W. Acid rain impact on phytoavailability of heavy metals in soils. Geosyst. Eng. 13 , 133–138. https://doi.org/10.1080/12269328.2010.10541320 (2010).
Kirk, G. J., Bellamy, P. H. & Lark, R. M. Changes in soil pH across England and Wales in response to decreased acid deposition. Glob. Change Biol. 16 , 3111–3119. https://doi.org/10.1111/j.1365-2486.2009.02135.x (2010).
Stevens, C. J., Thompson, K., Grime, J. P., Long, C. J. & Gowing, D. J. Contribution of acidification and eutrophication to declines in species richness of calcifuge grasslands along a gradient of atmospheric nitrogen deposition. Funct. Ecol. 24 , 478–484. https://doi.org/10.1111/j.1365-2435.2009.01663.x (2010).
Jonard, M. et al. Deterioration of Norway spruce vitality despite a sharp decline in acid deposition: A long-term integrated perspective. Glob. Change Biol. 18 , 711–725. https://doi.org/10.1111/j.1365-2486.2011.02550.x (2012).
Likens, G. E. & Bailey, S. W. The discovery of acid rain at the Hubbard Brook Experimental Forest: a story of collaboration and long-term research. In USDA Forest Service Experimental Forests and Ranges 463–482 (Springer, New York, NY, 2014). https://doi.org/10.1007/978-1-4614-1818-4_20
Maskell, L. C., Smart, S. M., Bullock, J. M., Thompson, K. E. N. & Stevens, C. J. Nitrogen deposition causes widespread loss of species richness in British habitats. Glob. Change Biol. 16 , 671–679. https://doi.org/10.1111/j.1365-2486.2009.02022.x (2010).
Simkin, S. M. et al. Conditional vulnerability of plant diversity to atmospheric nitrogen deposition across the United States. Proc. Natl. Acad. Sci. 113 , 4086–4091. https://doi.org/10.1073/pnas.1515241113 (2016).
Article CAS PubMed PubMed Central ADS Google Scholar
Park, J. H., Duan, L., Kim, B., Mitchell, M. J. & Shibata, H. Potential effects of climate change and variability on watershed biogeochemical processes and water quality in Northeast Asia. Environ. Int. 36 , 212–225. https://doi.org/10.1016/j.envint.2009.10.008 (2010).
Article CAS PubMed Google Scholar
Yang, S. et al. Effectiveness of amendments on re-acidification and heavy metal immobilization in an extremely acidic mine soil. J. Environ. Monit. 13 (7), 1876. https://doi.org/10.1039/c1em10028a (2011).
Dai, P. et al. Alleviating soil acidification and increasing the organic carbon pool by long-term organic fertilizer on tobacco planting soil. Agronomy 11 (11), 2135. https://doi.org/10.3390/agronomy11112135 (2021).
Araújo, R. A. Advances in soil engineering: sustainable strategies for rhizosphere and bulk soil microbiome enrichment. Frontiers in Bioscience-Landmark, 27(6), 195 (2022). https://doi.org/10.31083/j.fbl2706195 .
Bashir, M. A. et al. Soil survey techniques determine nutrient status in soil profile and metal retention by calcium carbonate. Catena 173 , 141–149. https://doi.org/10.1016/j.catena.2018.10.015 (2019).
Zheng, S. A., Zheng, X. & Chen, C. Leaching behavior of heavy metals and transformation of their speciation in polluted soil receiving simulated acid rain. PLoS ONE 7 , e49664. https://doi.org/10.1371/journal.pone.0049664 (2012).
Article PubMed PubMed Central ADS Google Scholar
Zhang, J. E., Yu, J., Ouyang, Y. & Xu, H. Impact of simulated acid rain on trace metals and aluminum leaching in Latosol from Guangdong Province, China. Soil Sedim. Contam. Int. J. 23 , 725–735. https://doi.org/10.1080/15320383.2014.866934 (2014).
Wen, X. J., Duan, C. Q. & Zhang, D. C. Effect of simulated acid rain on soil acidification and rare earth elements leaching loss in soils of rare earth mining area in Southern Jiangxi Province of China. Environ. Earth Sci. 69 , 843–853. https://doi.org/10.1007/s12665-012-1969-4 (2013).
Tian, D. & Niu, S. A global analysis of soil acidification caused by nitrogen addition. Environ. Res. Lett. 10 , 024019. https://doi.org/10.1088/1748-9326/10/2/024019 (2015).
Theng, C. S. Keys to Soil Classification in Sarawak. Technical paper 10, 47 pp. Soil Division, Department of Agriculture, Sarawak (1993).
Sabang, J., Kendawang, J. J. & Lee, H. S. Soil characteristics of an abandoned shifting cultivation land in Sarawak, Malaysia. Tropics 10 , 251–263. https://doi.org/10.3759/tropics.10.251 (2000).
Tan, S. et al. Review of soils on the 52 ha long term ecological research plot in mixed dipterocarp forest at Lambir, Sarawak, Malaysian Borneo. Tropics 18 , 61–86. https://doi.org/10.3759/tropics.18.61 (2009).
EANET (Acid Deposition Monitoring Network in East Asia). Report on the State of Acid Deposition in East Asia. (Niigata, Japan, 2020).
Mohamed, R. M. S. R., Rahim, A. F. H. & Kassim, A. H. M. A monitoring of air pollutants (CO, SO 2 and NO) in ambient air near an industrial area. In: MATEC Web of Conferences Vol. 47, p. 05022 (EDP Sciences, 2016).
Norela, S., Nurfatihah, M. Z., Mainon, A. & Ismail, B. S. Wet deposition in the residential area of the Nilai industrial park in Negeri Sembilan, Malaysia. App. Sci. J. 7 , 170–179 (2009).
CAS Google Scholar
EANET (Acid Deposition Monitoring Network in East Asia). Report on Data 2011. (Niigata, Japan, 2013)
Du, Y. J., Wei, M. L., Reddy, K. R., Liu, Z. P. & Jin, F. Effect of acid rain pH on leaching behavior of cement stabilized lead-contaminated soil. J. Hazard. Mater. 271 , 131–140. https://doi.org/10.1016/j.jhazmat.2014.02.002 (2014).
Li, J., Jia, C., Lu, Y., Tang, S. & Shim, H. Multivariate analysis of heavy metal leaching from urban soils following simulated acid rain. Microchem. J. 122 , 89–95. https://doi.org/10.1016/j.microc.2015.04.015 (2015).
Tan, K. H. Soil Sampling, Preparation, and Analysis 2nd edn. (CRC Press, 2005).
Book Google Scholar
Allen, S. E., Grimshaw, H. M., Parkinson, J. A. & Quarmby, C. Chemical Analysis of Ecological Materials (Blackwell Scientific Publications, 1989).
Keeney, D. R. & Nelson, D. W. Nitrogen-inorganic forms. In Methods of Soil Analysis Part 2 (eds Page, A. L., Keeney, D. R., Baker, D. E. et al. ) (ASA and SSAA, 1982).
Rowell, D. L. Soil Science: Method and Applications (Pearson Education, Inc., 1994).
Cheftetz, B., Hatcher, P. H., Hadar, Y. & Chen, Y. Chemical and biological characterization of organic matter during composting of municipal solid waste. J. Environ. Qual. 25 , 776–785. https://doi.org/10.2134/jeq1996.00472425002500040018x (1996).
Brady, N. C. & Weil, R. R. The Nature and Properties of Soils 13th edn. (Pearson Education, Inc., 2002).
Ayers, G. P., Leong, C. P., Gillett, R. W. & Lim, S. F. Rainwater composition and acidity at five sites in Malaysia in 1996. Water Air Soil Pollut. 133 , 15–30. https://doi.org/10.1023/A:1012967614759 (2002).
Moran, E. F. et al. Effects of soil fertility and land-use on forest succession in Amazonia. For. Ecol. Manage 139 , 93–108. https://doi.org/10.1016/S0378-1127(99)00337-0 (2000).
Lu, D., Moran, E. & Mausel, P. Linking Amazonian secondary succession forest growth to soil properties. Land Degrad. Dev. 13 , 331–343. https://doi.org/10.1002/ldr.516 (2002).
Statistical Analysis System, SAS. SAS/STAT software (Version 9.2) [Statistical software]. https://www.sas.com/en_us/software/stat.html (2009).
Shajib, M. T. I., Hansen, H. C. B., Tao, L. & Holm, P. E. Metals in surface-specific urban runoff in Beijing, China. Environ. Pollut. 248 , 584–598. https://doi.org/10.1016/j.envpol.2019.02.039 (2019).
Shajib, M. T. I., Hansen, H. C. B., Tao, L. & Holm, P. E. Rare earth element in surface specific urban runoff in Northern Beijing. Sci. Total Environ. 717 , 136969. https://doi.org/10.1016/j.scitotenv.2020.136969 (2020).
Article CAS PubMed ADS Google Scholar
Wei, H. et al. Soil pH responses to simulated acid rain leaching in three agricultural soils. Sustainability 12 , 280. https://doi.org/10.3390/su12010280 (2020).
Rampazzo, N. & Blum, W. E. Changes in chemistry and mineralogy of forest soils by acid rain. Water Air Soil Pollut. 61 , 209–220. https://doi.org/10.1007/BF00482605 (1992).
Mulder, J., Van Breemen, N. & Eijck, H. C. Depletion of soil aluminium by acid deposition and implications for acid neutralization. Nature 337 , 247–249. https://doi.org/10.1038/337247a0 (1989).
Sokolova, T. A. & Alekseeva, S. A. Adsorption of sulfate ions by soils (a review). Eurasian Soil Sci. 41 , 140–148. https://doi.org/10.1134/S106422930802004X (2008).
Johnson, D. W., Turner, J. & Kelly, J. M. The effects of acid rain on forest nutrient status. Water Resour. Res. 18 , 449–461. https://doi.org/10.1029/WR018i003p00449 (1982).
De Walle, D. R., Ribblett, G. C., Helvey, J. D. & Kochenderfer, J. Laboratory investigation of soil solution chemistry from six Appalachian Forest floor types subjected to simulated acid rain. J. Environ. Qual. 14 , 234–240. https://doi.org/10.1007/BF00284237 (1985).
David, M. B. & Driscoll, C. T. Aluminum speciation and equilibria in soil solution of a haplorthod in the Adirondack Mountain (New York, U.S.A.). Geoderma 33 , 297–318. https://doi.org/10.1016/0016-7061(84)90031-4 (1984).
Zarabi, M. & Jalali, M. Leaching of nitrogen and base cations from calcareous soil amended with organic residues. Environ. Technol. 33 (14), 1577–1588. https://doi.org/10.1080/09593330.2011.638675 (2012).
Hüttl, R. F. & Frielinghaus, M. Soil fertility problems—An agriculture and forestry perspective. Sci. Total Environ. 143 , 63–74. https://doi.org/10.1016/0048-9697(94)90533-9 (1994).
Perumal, M., Wasli, M. E., Ying, H. S., Lat, J. & Sani, H. Association between soil fertility and growth performance of planted Shorea macrophylla (de Vriese) after enrichment planting at rehabilitation sites of Sampadi Forest Reserve, Sarawak, Malaysia. Int. J. For. Res. https://doi.org/10.1155/2017/6721354 (2017).
Download references
Acknowledgements
The authors would like to acknowledge Department of Environment of Malaysia and Malaysia Meteorological Department for the providing atmospheric concentration data on 2020 and Bintulu temperature and rainfall data. We also aknowlwdge the technical support provided by Muhamad Fuad Ibrahim, Palanivell Perumal, UPMKB staff Arni Japar, Awang Marzuki Awang Mustapha, Elizabeth Andrew Anyah, and Awangku Ahmad Nizam Awang during the conduct of the research.
This research was funded by the Universiti Malaysia Sarawak under PILOT Research Grant Scheme (UNI/F07/PILOT/85193/2022), Ministry of Higher Education Education (Malaysia) under the Fundamental Research Grant Scheme (FRGS: 5523701), Universiti Putra Malaysia under the Research University Grant Scheme (RUGS: 9199765), Ministry of the Environment (Japan) under the Environmental Research and Technology Development Fund (B-0801), and Mitsubishi Corporation Trust Fund (6380500).
Author information
Md. Tariqul Islam Shajib
Present address: Department of Natural Resources and Environmental Design, North Carolina Agricultural and Technical State University, Greensboro, NC, USA
Authors and Affiliations
Agrotechnology Programme, Faculty of Resources Science and Technology, Universiti Malaysia Sarawak, 94300, Kota Samarahan, Sarawak, Malaysia
Mohamad Hilmi Ibrahim
Department of Land Management, Faculty of Agriculture, Universiti Putra Malaysia, 43400, Serdang, Selangor Darul Ehsan, Malaysia
Susilawati Kasim
Universiti Islam Sultan Sharif Ali, Kampus Sinaut, Km 33 Jln Tutong Kampong Sinaut, Tutong, TB1741, Negara Brunei Darussalam
Osumanu Haruna Ahmed
Faculty of Sustainable Agriculture, Universiti Malaysia Sabah, 90000, Sandakan, Sabah, Malaysia
Mohd. Rashid Mohd. Rakib & Nur Aainaa Hasbullah
Division of Soil, Water and Environment, Care to People Denmark, 2400, Copenhagen, NV, Denmark
You can also search for this author in PubMed Google Scholar
Contributions
[I.M.H] conceptualized the study, developed the main research framework, and was primarily responsible for the laboratory leaching and incubation study. He also contributed to data curation, methodology, and wrote the original draft of the manuscript. [K.S] played a pivotal role in data analysis and interpretation. She employed the necessary statistical tools to determine the correlations and were responsible for generating all the data charts and figures. [Author B] also assisted in writing and revising the manuscript, ensuring the technical details were articulated effectively. [A.O.H] managed the logistics and resources for the entire study. He supervised the application of SAR treatments to the soil samples and were instrumental in ensuring that standard procedures were adhered to. [Author C] also played a role in manuscript revision, particularly overseeing the accuracy and authenticity of the methodology. [M.R.M.R., H.N.A and I.M.T] contributed to the literature review and provided essential insights into the implications of the research findings, particularly concerning tropical forest ecosystems. They also assisted in drafting the discussion and conclusion sections of the manuscript and provided critical feedback for overall improvement.
Corresponding author
Correspondence to Mohamad Hilmi Ibrahim .
Ethics declarations
Competing interests.
The authors declare no competing interests.
Additional information
Publisher's note.
Springer Nature remains neutral with regard to jurisdictional claims in published maps and institutional affiliations.
Rights and permissions
Open Access This article is licensed under a Creative Commons Attribution 4.0 International License, which permits use, sharing, adaptation, distribution and reproduction in any medium or format, as long as you give appropriate credit to the original author(s) and the source, provide a link to the Creative Commons licence, and indicate if changes were made. The images or other third party material in this article are included in the article's Creative Commons licence, unless indicated otherwise in a credit line to the material. If material is not included in the article's Creative Commons licence and your intended use is not permitted by statutory regulation or exceeds the permitted use, you will need to obtain permission directly from the copyright holder. To view a copy of this licence, visit http://creativecommons.org/licenses/by/4.0/ .
Reprints and permissions
About this article
Cite this article.
Ibrahim, M.H., Kasim, S., Ahmed, O.H. et al. Impact of simulated acid rain on chemical properties of Nyalau series soil and its leachate. Sci Rep 14 , 3534 (2024). https://doi.org/10.1038/s41598-024-52758-1
Download citation
Received : 26 October 2023
Accepted : 23 January 2024
Published : 12 February 2024
DOI : https://doi.org/10.1038/s41598-024-52758-1
Share this article
Anyone you share the following link with will be able to read this content:
Sorry, a shareable link is not currently available for this article.
Provided by the Springer Nature SharedIt content-sharing initiative
By submitting a comment you agree to abide by our Terms and Community Guidelines . If you find something abusive or that does not comply with our terms or guidelines please flag it as inappropriate.
Quick links
- Explore articles by subject
- Guide to authors
- Editorial policies
Sign up for the Nature Briefing: Anthropocene newsletter — what matters in anthropocene research, free to your inbox weekly.

Acid Rain and Our Ecosystem
More than 150 years after acid rain was first identified, scientists now see success in recovery from its damaging effects
Cassandra Willyard
/https://tf-cmsv2-smithsonianmag-media.s3.amazonaws.com/filer/acid-rain-black-crust-gravestones-Madison-Street-Cemetery-Hamilton-New-York-631.jpg)
Geologist Rich April climbs the small hill behind Colgate University and makes his way into the cemetery. He stops before a white marble pillar erected in 1852. The inscription is nearly illegible. Over time, any stone exposed to the elements will weather, April explains, but this marble has weathered unnaturally fast. The culprit? Acid rain.
April pulls a vial of acid from his pocket to demonstrate. He unscrews the cap and lets a few drops leak onto the stone, where they fizz and bubble. The rain that fell throughout the Northeast in the latter half of the 20th century wasn’t as acidic as the liquid in April’s vial, but the principle is the same. Acid eats marble. Given enough time, it can erase even words meant to last an eternity.
The effects of acid rain extend far beyond graveyards. Acid rain destroyed fish populations in lakes and streams, harmed fragile soils and damaged millions of acres of forest worldwide.
These far-reaching effects illustrate the profound impact air pollution can have on the land. But the story of acid rain is also a tale of how understanding air pollution can lead to solutions. Due to overwhelming scientific evidence linking power plant emissions to acid rain and acid rain to the death of lakes, new regulations have dramatically cut emissions and cleaned up the rain that falls on the United States.
The term ‘acid rain’ was coined in the mid-1800s, when Robert Angus Smith, a Scottish chemist working in London, noticed that rain tended to be more acidic in areas with more air pollution and that buildings crumble faster in areas where coal is burned. But it took another century for scientists to realize that acid rain was a widespread environmental problem. Scandinavian scientists began to document acidic damage to lakes and streams in the 1950s. In 1963, Gene Likens, then at Dartmouth, and colleagues began collecting and testing the pH of rainwater in New Hampshire’s White Mountains as part of an ecosystem study. They were surprised to find that it was quite acidic, but they didn’t have much basis for comparison; at that time, scientists weren’t regularly measuring the pH of rainwater.
Likens took a job at Cornell a few years later and set up instruments to collect rainwater in the Finger Lakes region and soon observed that the rain in New York was roughly as acidic as rain in New Hampshire. “That was the first clue that we had that this might be some kind of a regional phenomenon,” he says. But neither Likens nor his colleagues had a clear idea what the cause might be.
Likens won a fellowship that took him to Sweden in 1969, a serendipitous event, he says, because he met Svante Odén, a scientist at Uppsala University who had observed the same trends in Sweden that Likens had been observing in the Northeastern United States. Odén had his finger on a potential cause. “He was trying to build a case that [acid rain] might be due to emissions coming from the more industrialized areas of Europe,” Likens recalls.
Likens and his colleagues traced the emissions from coal-fired power plants and examined satellite and aircraft data, and they found a similar long-distance link. “Sure enough, the emissions were coming primarily from Midwestern states like Indiana, Ohio, Illinois and Kentucky,” Likens recalls. “They were making their way literally thousands of kilometers to New England and southeastern Canada and coming back down as acids.”
He reported his findings in Science in 1974, and the story was immediately picked up by newspapers. The phone didn’t stop ringing for months, Likens recalls. “It was that media exposure that really put acid rain on the map in North America.”
Acid rain occurs, Likens and Odén and other scientists realized, when sulfur dioxide and nitrogen oxide enter the atmosphere and react with water to form sulfuric and nitric acids. Natural sources of these gases exist—volcanoes, for instance, belch out sulfur dioxide—but the vast majority comes from the burning of fossil fuels, especially by coal-fired power plants. The tall smokestacks allow pollution to travel long distances. According to studies conducted by Likens and his colleagues, normal rainwater has a pH of 5.2. During the 1970s and 1980s, when acid rain was at its worst, scientists recorded pH levels as low as 2.1, roughly 1,000 times more acidic.
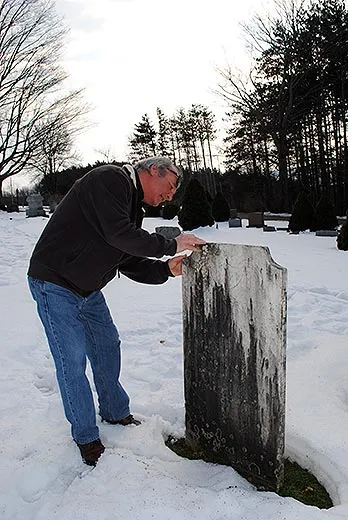
Acid rain affected many parts of the United States, but the Northeast suffered the most ecological damage. The Adirondack Mountains proved especially susceptible. Many soils contain calcium carbonate or other minerals that can neutralize acid rain before it seeps into lakes and streams. “Unfortunately the Adirondacks have almost none,” April says. As a result, lakes and streams quickly became acidic, killing fish and other aquatic animals.
In the late 1970s, researchers surveyed 217 lakes above 2,000 feet in the Adirondacks and found that 51 percent were highly acidic. The news was so grim that scientists began attempting to breed more acid-tolerant strains of trout. One New York State employee compared the area to Death Valley. A decade later, a larger study that included 849 lakes higher than 1,000 feet found that 55 percent were either completely devoid of life or on the brink of collapse.
As the scientific evidence linking acid rain to power plant emissions and ecological damage mounted, battles erupted among industry, scientists and environmentalists. “The 1980s is a period I call the ‘acid rain wars,’” Likens says. “There was huge rancorous nasty controversy.” Environmentalists from Greenpeace climbed power plant smokestacks and hung banners in protest; scientists testified before Congress about the link between emissions and acid rain, the severity of the effects, and whether proposed legislation would have an impact; and the power industry questioned the science and argued that regulations would drive electricity rates sky high.
Congress passed several amendments to the Clean Air Act in 1990 that cut emissions of sulfur dioxide through a cap-and-trade scheme. The goal was a 50 percent reduction in sulfur dioxide emissions from 1980 levels. That goal was achieved in 2008, two years before the deadline, which was set for 2010. Sulfur dioxide emissions fell from 17.3 million tons in 1980 to 7.6 million tons in 2008, less than the 8.95 million tons required by 2010.
The effect has been remarkable. Doug Burns, a scientist at the U.S. Geological Survey in Troy, New York, who directs the National Acid Precipitation Assessment Program, says the rain falling in the Northeast today is about half as acidic as it was in the early 1980s. Consequently, surface waters have become less acidic and fragile ecosystems are beginning to recover.
In many places, however, recovery has been painfully slow. Scientists now know that acid rain not only acidified lakes and streams, it also leached calcium from forest soils. That calcium depletion has had devastating effects on trees, especially sugar maples and red spruce. Acid rain leaches calcium from the needles of red spruce, making them more susceptible to cold. It also leaches calcium and magnesium from the soil, which can stress sugar maples. In addition, acid rain allows aluminum to accumulate in the soil. When trees take up aluminum, their roots can become brittle.
Some researchers have tried adding calcium back into the forests to speed recovery. April is currently involved in one such experiment in the Adirondacks. Over the past four and a half years, the calcium has penetrated only the top 15 centimeters of forest soil. “It takes a really long time for [the calcium] to get back down into the soil,” April says, so it won’t be a quick fix.
April would like to see sulfur dioxide and other emissions curtailed even further. “We still have acid rain coming in,” he says. “Some lakes look like they might be ready to come back, and if we cut the emissions more they would.”
Princeton University’s Michael Oppenheimer, who was a key player in the acid wars as chief scientist for the conservation group Environmental Defense Fund, agrees. “I think sulfur dioxide and nitrogen oxide need to be effectively eliminated,” he says. “We ought to head towards zero and see how close we can get.”
Although some effects of acid rain are lingering, most scientists consider it an environmental success story. “Science identified the problem. Science provided the guidelines for how to try to resolve the problem,” Likens says. “The success is that we have taken action as a society to try to deal with the problem.”
Get the latest Science stories in your inbox.
- Search Menu
- Sign in through your institution
- Advance articles
- Editor's Choice
- Special Collections
- Author Guidelines
- Submission Site
- Open Access
- Reasons to submit
- About BioScience
- Journals Career Network
- Editorial Board
- Advertising and Corporate Services
- Self-Archiving Policy
- Potentially Offensive Content
- Terms and Conditions
- Journals on Oxford Academic
- Books on Oxford Academic

Article Contents
- < Previous
Acid Rain: Researchers Addressing Its Lingering Effects
- Article contents
- Figures & tables
- Supplementary Data
Lesley Evans Ogden, Acid Rain: Researchers Addressing Its Lingering Effects, BioScience , Volume 68, Issue 11, November 2018, Page 928, https://doi.org/10.1093/biosci/biy113
- Permissions Icon Permissions
Acid rain, linked to fossil fuel–burning emissions, featured prominently in 1970 s to 1990 s headlines. Concern over dying lakes triggered international solution seeking, but as emissions-reducing agreements were enacted, attention to the problem faded. But the legacy of acid rain remains. Despite water chemistry's return to normal pH levels, many biological communities remain altered. The problem, explains David Schindler, professor emeritus at University of Alberta, is that although sulfur oxides were controlled, “We did nothing to control oxides of nitrogen.” Nitric acid remains high enough to deplete soil calcium in many parts of eastern Canada and elsewhere.
Now, Canadian scientists are exploring whether biological recovery for one key fish food—a freshwater shrimp that disappeared completely in many acidified lakes—might be given a helping hand. Heading the study is Mike Rennie, Canada Research Chair in Freshwater Ecology and Fisheries at Lakehead University in Northwestern Ontario and Research Fellow at Canada's International Institute for Sustainable Development Experimental Lakes Area (IISD-ELA), where the experiment is taking place. Since 1968, this research hub, renowned for experimental manipulations of whole lakes, has drawn scientists internationally.
ELA’s isolated soft-water lakes had escaped acidification. So unlike many contaminated lakes elsewhere, where biological community composition preacidification was poorly documented, “Here we were able to add the acid right in the lake experimentally and show how the whole ecosystem responded,” Rennie says. In acidification experiments, a scientist protectively suited up “rather like Darth Vader,” piped a sulfuric acid solution, and mixed it by boat propeller into lake 223, says Schindler, ELA founding director. Over 7 years, researchers reduced pH from 6.8 to 5.0. Neighboring lake 224, untreated, was their experimental control. Although lab studies suggested a 5.0 pH would not harm trout, in this ecosystem context, it did. By pH 5.6, most trout food—including tiny organisms requiring calcium to form their exoskeleton—had died, their protective coatings dissolved.
Photographs of starving fish helped persuade policymakers to legislate more rigorous air-quality standards. Reduced emissions addressed the root cause, but decades of acid rain had already transformed many limnological food webs. Crustaceans such as Mysis relicta, a freshwater shrimp and keystone species as vital trout food, were extirpated from some lakes. Some fish starved or switched to different prey. But even today, brook trout and lake trout populations in thousands of North American and European lakes have yet to fully recover.
After nearly a half century, lake 223 is no longer acidic. The chemistry and other ecosystem components look normal, says Rennie, with one key caveat. “When you look at lake trout in this lake, they grow much slower than they used to.” That, his team hypothesizes, is because Mysis are still missing, even though they are abundant in nearby lake 224. In lake 223, with Mysis missing, fish had few other prey options. So the team wants to know: Can Mysis reintroductions jumpstart the ecosystem?
IISD-ELA food web biologist Cyndy Desjardins spent last summer characterizing the “before” conditions. Then, in spring 2018, 10,000 Mysis, painstakingly counted by hand, were introduced into lake 223. Students, using white trays divided into quadrats, ferried critters from one side to the other as they counted them one by one, explains Desjardins. It took three people four nights to count them. Another 10,000 Mysis were to be added in October. The crew will then study what happens next.
John Smol, heading the Paleoecological Environmental Assessment and Research Lab at Queen's University in Kingston, Ontario, has studied acid rain impacts since the 1980 s across Eastern Canada, New York's Adirondacks, and elsewhere. Lake sediment cores are his proxy time machine, incorporating fossils, radioisotopic decay, and modeled pH–biodiversity relationships to create a timeline of how chemistry affects biology. Bottlenecks caused by extirpations such as that of Mysis may be a biological missing piece in recovery of some formerly acidified lake systems, explains Smol, but chemical missing pieces may be critical too. A deficiency in dissolved calcium—stripped out by acidification—may continue to be a roadblock to recovery for zooplankton and other organisms that require this structurally supportive element to survive and thrive. Moreover, says Smol, “a lot of other things have happened besides acidification, not least of which is climate change, which is also affecting lakes quite dramatically.” Full biological recovery may be overly optimistic given that environmental change is a moving target. Nevertheless, the return of Mysis, Rennie's team hopes, may restore at least one piece of the ever-changing puzzle.
Lesley Evans Ogden is a scientist-turned multimedia storyteller based near Vancouver, Canada. Say hello at www.lesleyevansogden.com or on Twitter @ljevanso.
Month: | Total Views: |
---|---|
October 2018 | 17 |
November 2018 | 285 |
December 2018 | 88 |
January 2019 | 61 |
February 2019 | 74 |
March 2019 | 75 |
April 2019 | 200 |
May 2019 | 197 |
June 2019 | 69 |
July 2019 | 70 |
August 2019 | 52 |
September 2019 | 96 |
October 2019 | 151 |
November 2019 | 159 |
December 2019 | 122 |
January 2020 | 107 |
February 2020 | 145 |
March 2020 | 144 |
April 2020 | 173 |
May 2020 | 62 |
June 2020 | 80 |
July 2020 | 44 |
August 2020 | 47 |
September 2020 | 99 |
October 2020 | 91 |
November 2020 | 111 |
December 2020 | 96 |
January 2021 | 49 |
February 2021 | 44 |
March 2021 | 141 |
April 2021 | 87 |
May 2021 | 96 |
June 2021 | 21 |
July 2021 | 14 |
August 2021 | 23 |
September 2021 | 28 |
October 2021 | 56 |
November 2021 | 55 |
December 2021 | 36 |
January 2022 | 50 |
February 2022 | 108 |
March 2022 | 118 |
April 2022 | 166 |
May 2022 | 103 |
June 2022 | 76 |
July 2022 | 49 |
August 2022 | 35 |
September 2022 | 77 |
October 2022 | 116 |
November 2022 | 89 |
December 2022 | 60 |
January 2023 | 31 |
February 2023 | 43 |
March 2023 | 96 |
April 2023 | 84 |
May 2023 | 53 |
June 2023 | 36 |
July 2023 | 38 |
August 2023 | 33 |
September 2023 | 44 |
October 2023 | 83 |
November 2023 | 36 |
December 2023 | 55 |
January 2024 | 48 |
February 2024 | 63 |
March 2024 | 58 |
April 2024 | 87 |
May 2024 | 65 |
June 2024 | 51 |
July 2024 | 66 |
August 2024 | 51 |
Email alerts
Citing articles via.
- Recommend to your Library
Affiliations
- Online ISSN 1525-3244
- Copyright © 2024 American Institute of Biological Sciences
- About Oxford Academic
- Publish journals with us
- University press partners
- What we publish
- New features
- Open access
- Institutional account management
- Rights and permissions
- Get help with access
- Accessibility
- Advertising
- Media enquiries
- Oxford University Press
- Oxford Languages
- University of Oxford
Oxford University Press is a department of the University of Oxford. It furthers the University's objective of excellence in research, scholarship, and education by publishing worldwide
- Copyright © 2024 Oxford University Press
- Cookie settings
- Cookie policy
- Privacy policy
- Legal notice
This Feature Is Available To Subscribers Only
Sign In or Create an Account
This PDF is available to Subscribers Only
For full access to this pdf, sign in to an existing account, or purchase an annual subscription.
Acid rain: Causes, effects and solutions
How acid rain affects nearly everything it touches, and what we can do about it.
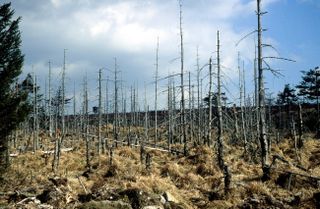
Solutions and prevention
Additional resources, bibliography.
Acid rain, or acid deposition, is a broad term that includes any form of precipitation that contains acidic components, such as sulfuric acid or nitric acid. The precipitation is not necessarily wet or liquid; the definition includes dust, gases, rain, snow, fog and hail. The type of acid rain that contains water is called wet deposition. Acid rain formed with dust or gases is called dry deposition.
The precipitation is not necessarily wet or liquid; the definition includes dust, gasses, rain, snow, fog and hail. The type of acid rain that contains water is called wet deposition. Acid rain formed with dust or gasses is called dry deposition.
Causes of acid rain
The term acid rain was coined in 1852 by Scottish chemist Robert Angus Smith, according to the Royal Society of Chemistry, which calls him the "father of acid rain." Smith decided on the term while examining rainwater chemistry near industrial cities in England and Scotland. He wrote about his findings in 1872 in the book " Air and Rain: The Beginnings of a Chemical Climatology ."
In the 1950s, scientists in the United States started studying the phenomenon, and in the 1960s and early 1970s, acid rain became recognized as a regional environmental issue that affected Western Europe and eastern North America.
Though manmade pollutants are currently affecting most acidic precipitation, natural disasters can be a factor as well. For example, volcanoes can cause acid rain by blasting pollutants into the air. These pollutants can be carried around the world in jet streams and turned into acid rain far from the volcano. After an asteroid supposedly wiped out the dinosaurs 65.5 million years ago, sulfur trioxide was blasted into the air. When it hit the air, it turned into sulfuric acid, generating a downpour of acid rain.
Even before that, over 4 billion years ago, it is suspected that the air may have had 10,000 times as much carbon dioxide as today. Geologists from the University of Wisconsin-Madison backed up this theory by studying rocks and publishing the results in a 2008 issue of the journal Earth and Planetary Science Letters. "At [those levels of carbon dioxide], you would have had vicious acid rain and intense greenhouse [effects]. That is a condition that will dissolve rocks," said study team member John Valley.
Sulfur dioxide (SO2) and nitrogen oxides (NOx) released into the air by fossil-fuel power plants, vehicles and oil refineries are the biggest cause of acid rain today, according to the Environmental Protection Agency (EPA). Two thirds of sulfur dioxide and one fourth of nitrogen oxide found in the atmosphere come from electric power generators.
A chemical reaction happens when sulfur dioxide and nitrogen oxides mix with water, oxygen and other chemicals in the air. They then become sulfuric and nitric acids that mix with precipitation and fall to the ground. Precipitation is considered acidic when its pH level is about 5.2 or below. The normal pH of rain is around 5.6.
Environmental affects of acid rain
Acid rain affects nearly everything. Plants, soil, trees, buildings and even statues can be transformed by the precipitation.
Acid rain has been found to be very hard on trees. It weakens them by washing away the protective film on leaves, and it stunts growth. A United States Environmental Protection Agency ( EPA ) study showed that acid rain is particularly hard on trees.
"By providing the only preserved soil in the world collected before the acid rain era, the Russians helped our international team track tree growth for the first time with changes in soil from acid rain," said Greg Lawrence, a U.S. Geological Survey scientist. "We've known that acid rain acidifies surface waters, but this is the first time we've been able to compare and track tree growth in forests that include soil changes due to acid rain."
Acid rain can also change the composition of soil and bodies of water, making them uninhabitable for local animals and plants. For example, healthy lakes have a pH of 6.5 or higher. As acid rain raises the level of acidity, fish tend to die off. Most fish species can't survive a water pH of below 5. When the pH becomes a 4, the lake is considered dead, according to National Atmospheric Deposition Program .
It can additionally deteriorate limestone and marble buildings and monuments, like gravestones.
There are several solutions to stopping human-caused acid rain. Regulating the emissions coming from vehicles and buildings is an important step, according to the EPA. This can be done by restricting the use of fossil fuels and focusing on more renewable energy sources such as solar and wind power.
Related: How do solar panels work?
Also, each person can do their part by reducing their vehicle use. Using public transportation, walking, riding a bike or carpooling is a good start, according to the EPA. People can also reduce their use of electricity, which is widely created with fossil fuels , or switch to a solar plan. Many electricity companies offer solar packages to their customers that require no installation and low costs.
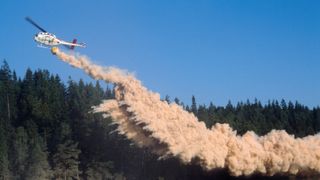
It is also possible to prevent acid rain forming, by adding lime deposits to major water sources. This method has been used to neutralize the Ph levels in the water, which reduced the acidity, for thousands of years, the LA Times reported . These so-called "liming" operations have also been used to restore wildlife. In Wales, a liming operation was conducted in 2003 to restore salmon to the Wye river. The water had become too acidic for the fish to survive, causing them to disappear from the river 18 years earlier, Young People's Trust for the Environment , a U.K. non-profit organization, reported.
Discover key facts about acid rain on Young Peoples Trust for the Environment , watch this National Geographic video about the role of fossil fuels and pollution in creating acid rain, and learn more about what the WWF is doing to reduce emissions.
- Peringe Grennfelt, Anna Engleryd, Martin Forsius, Øystein Hov, Henning Rodhe & Ellis Cowling: Acid rain and air pollution: 50 years of progress in environmental science and policy
- Douglas A.Burns, Julian Aherne, David A.Gay, Christopher M.B.Lehmann: Acid rain and its environmental effects: Recent scientific advances
- Lesley Evans Ogden: Acid Rain: Researchers Addressing Its Lingering Effects
Sign up for the Live Science daily newsletter now
Get the world’s most fascinating discoveries delivered straight to your inbox.
Rare 'moonbows' light up night sky across US as blue supermoon rises — and you could still spot another one
Why scientists are blown away by 'Twister' and 'Twisters'
Rare 'doomsday fish' said to bring earthquakes spotted in California days before LA quake
Most Popular
- 2 The brain stores at least 3 copies of every memory
- 3 This transparent sea creature can age in reverse
- 4 Ultra-thin solar 'coating' can turn phone cases and EVs into mini power generators
- 5 PureGuardian H950AR Ultrasonic Cool Mist Humidifier review

An official website of the United States government
Here’s how you know
Official websites use .gov A .gov website belongs to an official government organization in the United States.
Secure .gov websites use HTTPS A lock ( Lock A locked padlock ) or https:// means you’ve safely connected to the .gov website. Share sensitive information only on official, secure websites.
JavaScript appears to be disabled on this computer. Please click here to see any active alerts .
- Acid Rain Program Results
The Acid Rain Program (ARP) has delivered significant reductions of sulfur dioxide (SO 2 ) and nitrogen oxides (NO X ) emissions from fossil fuel-fired power plants, extensive environmental and human health benefits, and far lower-than-expected costs. Together with more recent power sector regulations, including the Cross-State Air Pollution Rule (CSAPR) programs, and a rapidly changing energy sector, the ARP has helped deliver annual SO 2 reductions of over 95% and annual NO X e missions reductions of over 89%. The Power Plant Emissions Reductions page has maps and data highlighting these emissions reductions, and the Power Sector Programs Progress Report provide an annual overview of program features and results, from compliance to air quality impacts.
Swipe left and right over the maps to see the changes before and after.
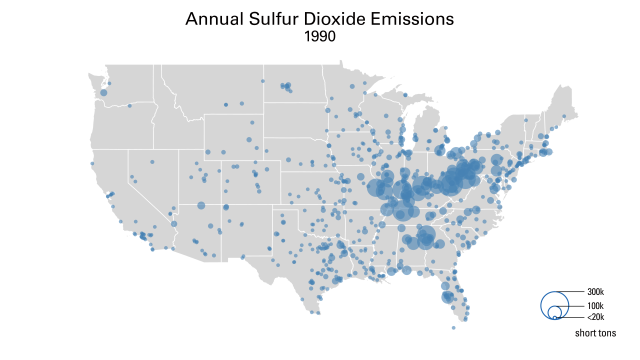
These emissions reductions have led to major decreases in acid rain nationwide. Wet sulfate deposition – a common indicator of acid rain – dropped by more than 70% between 1989-1991 and 2020-2022. Additional data and maps detailing deposition and ambient air pollution are available on the Clean Air Status and Trends Network (CASTNET) website.
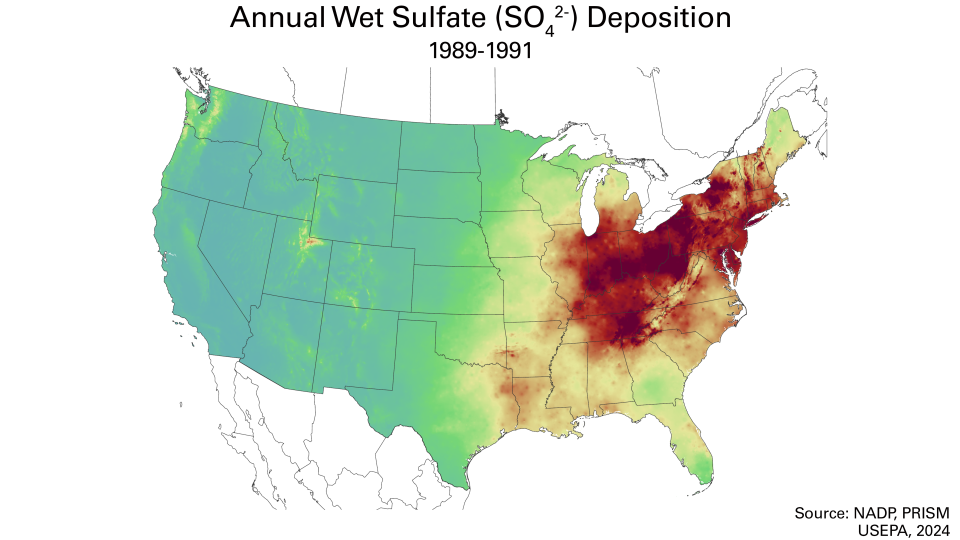
Next, learn more about the details of the Acid Rain Program .
Or, learn more about:
- Clean Air Power Sector Programs
- Clean Air Status and Trends Network (CASTNET)
- National Atmospheric Deposition Program (NADP)
- Long-Term Monitoring (LTM) Network
- Acid Rain Home
- What is Acid Rain?
- Effects of Acid Rain
- Acid Rain Program

Advertisement
How Acid Rain Works
- Share Content on Facebook
- Share Content on LinkedIn
- Share Content on Flipboard
- Share Content on Reddit
- Share Content via Email
Key Takeaways
- Acid rain, with a pH of 5.0 or less, is formed when pollutants like sulfur dioxide and nitrogen oxides from burning fossil fuels react with water vapor in the atmosphere, creating sulfuric and nitric acids.
- Acid rain can damage aquatic ecosystems by lowering pH levels and killing sensitive species, weakening trees and contributing to nitrogen deposition that leads to harmful algae blooms.
- Efforts to monitor and reduce the emissions that cause acid rain include national programs like the National Atmospheric Deposition Program (NADP) and the Clean Air Status and Trends Network (CASTNET).
If you hike through the Appalachian Mountains, you'll spot stands of dead and weakened trees. If you live in a city, you might notice worn stone buildings, streaks on your car roof or corroded metal railings and statues. You can see the effects of acid rain nearly everywhere you go, but with media and public attention turned to the more ominous prospect of global warming , acid rain has fallen by the wayside. The scourge from the sky almost seems like a 20th-century problem -- an issue dealt with in the 1980s and 1990s by legislation.
Acid rain occurs mostly in the Northern Hemisphere -- the more industrialized, dirtier half of the globe. Winds can sweep up emissions from high smokestacks and carry pollutants far from their original sources, crossing state lines and national borders in the process. Acid rain may not have the complete global range of greenhouse gases, but it is a transboundary , and therefore international, issue.
Acid rain, also known as acid deposition, is caused by emissions of sulfur dioxide (SO2) and nitrogen oxides (NOx) from power plants, cars and factories. Natural sources like volcanoes , forest fires and lightning strikes also add to the man-made pollution. SO2 and NOx become acids when they enter the atmosphere and react with water vapor. The resulting sulfuric and nitric acids can fall as wet or dry depositions. Wet deposition is precipitation: acid rain, snow, sleet or fog. Dry deposition falls as acidic particulates or gases.
The pH of Acid Rain
The effects of acid rain, reducing acid rain.
Scientists express the acidity of acid rain using the pH scale . The scale defines a solution's acidity, neutrality or alkalinity based on its concentration of hydrogen ions. Acids have a high concentration of hydrogen ions and a low pH. The scale ranges from zero to 14, with pure water at a neutral 7.0. Most water, however, is not exactly pure. Even clean, normal rain has a pH of about 5.6. This is because it reacts with carbon dioxide in the atmosphere and forms mildly acidic carbonic acid before it becomes rain.
Acid rain has a pH of 5.0 or less. Most acid deposition ranges from pH 4.3 to 5.0 -- somewhere between the acidity of orange juice and black coffee . But comparing acid rain to safe, natural acids can be misleading. Even at its weakest, acid rain wrecks ecosystems by stunting sensitive plants and killing delicate aquatic eggs.
Programs that monitor acid rain analyze hydrogen content to determine pH. They also measure atmospheric concentrations of nitric acid, nitrate, sulfur dioxide, sulfate and ammonium. In the United States, the National Atmospheric Deposition Program (NADP) supervises wet deposition while the Clean Air Status and Trends Network (CASTNET) observes dry deposition. Monitoring acid deposition helps determine critical loads , or the amount of pollutants an ecosystem can support before damage. Accurate critical loads help set effective targets for SO2 and NOx reductions.
Now we'll learn about the harmful effects of acid rain on aquatic environments, forests, finishes, building materials and human health.
Surface Waters
Surface waters and their fragile ecosystems are perhaps the most famous victims of acid rain. Most of the precipitation that enters a lake, river, stream or marsh must first pass over and seep through soil. All soil has a buffering capacity , or ability to resist changes in acidity and alkalinity. The soil's buffering capacity determines a water body's acidity. If the capacity is low, or has reached its limit, acid rain can pass through un-neutralized.
Most life is comfortable at a near-neutral pH -- stray too far from pH 7.0, and delicate organisms begin to die. Plankton and invertebrates are sensitive to changes in acidity and die first. At pH 5.0, fish eggs degrade and young cannot develop. Adult fish and frogs can sometimes tolerate acidities as low as pH 4.0, but they starve as their weaker food sources die out. When acid rain disrupts the food chain, biodiversity decreases.
Nitrogen deposition from acid rain also damages coastal waters and estuaries. Nitrogen-rich water supports massive algae growth and algal blooms. Bacteria decompose the dead algae, flourish themselves and soak up the water's available oxygen. Fish, shellfish, sea grass beds and coral reefs die in the algae-choked, oxygen-depleted waters. Scientists estimate that 10 percent to 45 percent of human-produced nitrogen that winds up in coastal waters comes from atmospheric deposition [Source: Environmental Protection Agency ].
Most acidic bodies of water do not look polluted. As decaying organic matter settles, acidified water can appear clear and blue. Some species, like rushes and moss, even thrive in acidic conditions. But the greenery and clear waters belie an unwholesome environment. Diversity drops, and species left without predators often grow disturbingly large.
Acid rain also damages forests, as we'll see in the next section.
Forests rely on their soil's buffering capacity to protect them from acid rain. Acidic waters draw out soil toxins like aluminum. Trees take in the poisonous substances, and runoff dumps it in lakes, rivers and streams. Acid rain also dissolves helpful minerals and nutrients like calcium, magnesium and potassium before trees can absorb them. Acid rain rarely kills a forest outright but instead stunts its growth through years of soil degradation. Nutrient deprivation and exposure to toxins make trees more likely to topple in storms or die in cold weather.
Even trees in well-buffered soil can weaken in harsh acid fog. High-elevation forests soak in acidic clouds, which strip leaves of nutrients and break down trees' ability to resist cold. The bald peaks of the Appalachian Mountains tell of the poisonous effect of acid rain on high-elevation forests.
Materials and Finishes
Acid rain has the unsettling ability to erase and obliterate stone and metal, the most durable of materials. Old buildings, monuments and tombstones bear the smooth signs of acidic corrosion and deterioration. Acid deposition speeds up natural weathering caused by rain, sun, snow and wind.
Acid rain also mars automotive paint. The auto industry considers acid deposition one type of corrosive environmental fallout , along with tree sap, pollen and bird droppings. Acid markings leave irregular, etched shapes on horizontal surfaces. Repainting is the only way to fix a car finish disfigured by acid rain.
Since acid rain can kill aquatic animals, weaken trees and dissolve stone, it seems like it could also scald or burn humans. But it doesn't affect people in the same way as it does fish or plants. Acid rain feels the same as regular rain -- it's even safe to swim in an acidic lake. But the sulfate and nitrate particulates of dry deposition can cause asthma, bronchitis and heart problems. The NOx in acid deposition also reacts with volatile organic compounds (VOCs) to form ground-level ozone. Ozone, or smog , aggravates and weakens the respiratory system.
Acid rain has existed since the first factories of the Industrial Revolution began spitting out toxic emissions. An English scientist, Robert Angus Smith, coined the term "acid rain" in 1872 when he wrote of its corroding touch on buildings and deadly effect on plants. But acid rain did not become a government-monitored environmental problem until more than a century later. Scientists had by then determined that acid rain was a transboundary rather than a local concern. In 1980, the Acid Deposition Act launched a 10-year study on acid rain under the direction of the National Acidic Precipitation Assessment Program (NAPAP) to monitor sites around the country.
In 1990, armed with the NAPAP's study, Congress changed the existing Clean Air Act to include acid rain. The new Title IV amendment of the Clean Air Act called for SO2 and NOx reductions. The Acid Rain Program (ARP) was formed in 1995 to bring Title IV into effect.
The ARP places limits on the power industry to reduce annual emissions of SO2 and NOx. The ARP uses a cap and trade program to cut SO2 emissions. It sets a cap on the total amount of SO2 that power plants in the contiguous United States can produce. After setting a cap, the ARP distributes allowances to power plant units. Units are only allowed to produce as much SO2 as they have credit for. If they reduce emissions faster than the ARP requires, they can bank allowances for future use or sell them to other plants. The final 2010 cap will be 8.95 million tons allowed per year, a remarkable 50 percent less than power plant emissions from 1980 [Source: EPA ].
The ARP regulates NOx reductions with a more conventional rate-based regulatory system . The program sets a limit on allowable pounds of NOx per million British thermal units (lb/mmBtu) for every power plant's boiler. Owners either meet target reductions for individual boilers or average the emissions of all units owned and meet a combined target. The ARP aims to reduce NOx to 2 million tons below the projected 2000 level had Title IV not existed [Source: EPA ].
Power plants meet their ARP targets by using low sulfur coal, "wet scrubbers" or flue gas desulphurization systems, low NOx burners and other clean coal technologies. They can also trade SO2 credits amongst themselves.
Even with an increased energy demand, the ARP has successfully reduced emissions of SO2 and NOx. But NAPAP suggests that for ecosystems to fully recover, reductions will have to drop an additional 40 percent to 80 percent below the full-force limits of 2010 [Source: EPA ].
Cars also emit NOx. Newer designs of catalytic converters help treat exhaust and remove NOx and other pollutants like carbon monoxide and the VOCs that contribute to smog.
Even with remarkable clean coal technologies, catalytic converters and strong caps and regulations, fossil fuels are still a dirty power source. Alternative forms of energy like nuclear , solar and hydropower do not emit the millions of tons of SO2 and NOx that upend ecosystems, blight buildings and monuments and weaken people's health.
Frequently Asked Questions
Can acid rain affect human health directly, how can individuals reduce their contribution to acid rain, lots more information.
- “Acid Rain.” Encyclopedia of the Atmospheric Environment. http://www.ace.mmu.ac.uk/eae/Acid_Rain/acid_rain.html
- “Acid Rain.” Environmental Literacy Council. http://www.enviroliteracy.org/article.php/2.html
- “Acid Rain Program: 2005 Progress Report.” U.S. Environmental Protection Agency. http://www.epa.gov/airmarkets/progress/docs/2005report.pdf
- “A Brief History.” U.S. Environmental Protection Agency. http://www.epa.gov/region1/eco/acidrain/history.html
- “Clean Air Status and Trends Network (CASTNET).” U.S. Environmental Protection Agency. http://www.epa.gov/castnet/
- “Effects of Acid Rain - Forests.” U.S. Environmental Protection Agency. http://www.epa.gov/acidrain/effects/forests.html
- “Effects of Acid Rain - Surface Waters and Aquatic Animals.” U.S. Environmental Protection Agency. http://www.epa.gov/acidrain/effects/surface_water.html
- Ganguly, Meenhakshi. “At The Taj Mahal, Grime Amid Grandeur.” Time. September 10, 2001. http://www.time.com/time/magazine/article/0,9171,1000714-1,00.html
- “Measuring Acid Rain.” U.S. Environmental Protection Agency. http://www.epa.gov/acidrain/measure/index.html
- “NADP History and Overview.” National Atmospheric Deposition Program. http://nadp.sws.uiuc.edu/nadpoverview.asp
Please copy/paste the following text to properly cite this HowStuffWorks.com article:

IMAGES
COMMENTS
Acid rain (AR) can be defined as a combination of dry and wet deposition from the atmosphere having higher than normal concentrations of nitric (HNO 3), sulfuric acids (H 2 SO 4), and acidifying compounds which lead to a decrease in the pH of rainwater to less than 5.61.In 1845, AR was first been mentioned by Ducros, although a detailed study of AR was conducted by Robert Angus Smith and ...
When continuous moderators were singly included in our analysis, treatment pH showed a significant positive relationship with the log response ratios of aboveground biomass and belowground biomass (both p < 0.001; Fig. 3 a and b). Besides, acid rain interval showed significant and positive effects on the responses of aboveground biomass (p = 0.001, Fig. 3 c), whereas belowground biomass was ...
Due to its deleterious and large-scale effects on the ecosystem and long-range transboundary nature, acid rain has attracted the attention of scientists and policymakers. Acid rain (AR) is a prominent environmental issue that has emerged in the last hundred years. AR refers to any form of precipitation leading to a reduction in pH to less than 5.6. The prime reasons for AR formation encompass ...
Acid rain (AR) pollution has led to frequent ecological disasters and economic loss worldwide since the beginning of the industrial revolution (Singh and Agrawal, 2008; Grennfelt et al., 2020).Generally, emissions from human activities, including those caused by coal burning, industrial production, and automobile exhaust, produce acidic substances such as sulfur dioxide (SO 2) and nitrogen ...
Against our hypothesis, acid rain impact on the transfer and distribution of photosynthate 13 C to soil was not simply decreased or increased, but was highly concentration- and duration-dependent, i.e., the proportion of net fixed 13 C to soil significantly increased under acid rain of pH 4.5 and 3.0 ...
The formation of acid rain is essentially attributable to the presence in the atmosphere of different pollutants or acid precursors: carbon dioxide (CO 2), sulfur oxides or SO x (mainly SO 2), and, to a lesser extent, nitrogen oxides or NO x (NO and NO 2).. The presence of these substances may be due to natural phenomena, such as: volcanic eruptions, lightning, wildfires, bacterial degradation ...
The term 'acid rain' refers to atmospheric deposition of acidic constituents that impact the earth as rain, snow, particulates, gases, and vapor. Acid rain was first recognized by Ducros (1845) and subsequently described by the English chemist Robert Angus Smith (Smith, 1852) whose pioneering studies linked the sources to industrial emissions and included early observations of deleterious ...
Although efforts have been made to assess the effects of acid rain on terrestrial ecosystems, a systematic assessment of growth-related traits across plant aboveground and belowground is lacking. Hence, we performed a phylogenetically controlled meta-analysis of 755 observations from 69 independent studies to quantify the effects of acid rain ...
Acid rain, the common term for acidic deposition of wet (rain, snow, sleet, hail, fog water) and dry (gases and particles) deposition of acidic substances from the atmosphere to the Earth's surface, in combination with the associated air pollution from precursor emissions, has been a major and vexing environmental problem on several continents (e.g., Likens and Butler 2014).
Acid rain and acidification research are indeed a multidisciplinary field. This field evolved from the first attempts to mitigate acid freshwater in the 1920s, then linking acid rain to the acidification in late 1950s, to the broad project-concepts on cause and effect from the late 1960s. Three papers from 1974, 1976 and 1988 demonstrate a broad approach and comprise scientific areas from ...
This minibrief describes the major hypothesis explaining why acid rain may be contributing to forest decline, along with the major arguments against this hypothesis. For additional information on acid rain and current legislation for pollutant emissions controls, see IB83016 -- Acid Rain: Current Issues, and IB83005 -- Clean Air Act: An Overview.
Observations of increased clarity of lakes formed the hypothesis of "oligotrophication". Increased concentrations of several trace elements like Pb and Zn were also described. This paper was extremely important for the onset of similar projects and programs in many countries suffering from acid rain.
Greenhouse gases can cause acid rain, which in turn degrades soil chemical properties. This research was conducted to determine the effects of simulated acid rain (SAR) on the chemical properties ...
According to studies conducted by Likens and his colleagues, normal rainwater has a pH of 5.2. During the 1970s and 1980s, when acid rain was at its worst, scientists recorded pH levels as low as ...
Modeling the efects of acid rain on bacterial growth. ackTenafly Middle School, Tenafly New JerseySUMMARYAcid rain has caused. devastating decreases in ecosystems across the globe. This problem h. s been escalating due to the burning of fossil fuels. To mimic the efect of acid rain on the environment, we analyzed the growth of gram-negative ...
Effects of acid rain on soil respiration, their temperature sensitivity and soil characteristics. Additionally, AR significantly reduced soil pH by 7.84 % and increased SOC by 13.25 % (Fig. 1). AR significantly reduced MBC by 12.15 % and FRB by 5.67 %, but increased F:B by 15.91 %. ... The carbon quality-temperature hypothesis suggests that, ...
Effects of Acid Rain on Materials. Not all acidic deposition is wet.Sometimes dust particles can become acidic as well, and this is called dry deposition.When acid rain and dry acidic particles fall to earth, the nitric and sulfuric acid that make the particles acidic can land on statues, buildings, and other manmade structures, and damage their surfaces.
Acid rain, linked to fossil fuel-burning emissions, featured prominently in 1970 s to 1990 s headlines. Concern over dying lakes triggered international solution seeking, but as emissions-reducing agreements were enacted, attention to the problem faded. But the legacy of acid rain remains. Despite water chemistry's return to normal pH levels ...
Acid rain can also change the composition of soil and bodies of water, making them uninhabitable for local animals and plants. For example, healthy lakes have a pH of 6.5 or higher. As acid rain ...
The Acid Rain Program (ARP) has delivered significant reductions of sulfur dioxide (SO 2) and nitrogen oxides (NO X) emissions from fossil fuel-fired power plants, extensive environmental and human health benefits, and far lower-than-expected costs.Together with more recent power sector regulations, including the Cross-State Air Pollution Rule (CSAPR) programs, and a rapidly changing energy ...
Introduction. Acid rain occurs when pollution in the atmosphere (sulfur dioxide and nitrogen oxide) is chemically changed and absorbed by water droplets in clouds. When there is precipitation, the droplets fall to earth as rain, snow, or sleet. The polluting chemicals in the water droplets form an acid by combining with the hydrogen and oxygen in the water.
Acid Rain. Step 1 - State the Problem. Step 2 - Research, and Hypothesize or Predict. Step 3 - Plan Experiment and Gather Data. Step 4 - Analyze the Data and Make a Conclusion. Step 5 - Take ...
Acid rain, with a pH of 5.0 or less, is formed when pollutants like sulfur dioxide and nitrogen oxides from burning fossil fuels react with water vapor in the atmosphere, creating sulfuric and nitric acids. Acid rain can damage aquatic ecosystems by lowering pH levels and killing sensitive species, weakening trees and contributing to nitrogen ...
1. Introduction. Acid rain is a polluting atmospheric precipitation, including rain, snow, and hail, with a pH < 5.6. Since the 1980s, acid rain has become a major concern for China, as the main contributor to the world's third-largest acid rain area after Europe and North America (Hou and Zhao, 2012; Liu et al., 2011).In addition to natural factors, the major contributors to acid rain are ...