Harvard Science Review
The undergraduate science publication of Harvard University since 1988, looking to bring cutting-edge research, central debates, and new implications of scientific ideas to a wide community of scientists and non-scientists alike.

A History of Microscopy

By: Kelvin Li
INTRODUCTION
Sight, if not the most brainpower hungry sense, is certainly the one we use the most. Think about it, our lives are inundated with visual stimulation from intellectual processes like reading text, watching videos, and interpreting body language and facial expressions to more simple tasks such as walking in a straight line and anything that requires hand-eye coordination. Simply put, being alive depends a great deal upon the power of eyesight.
And yet it’s startling to think about all the things that are invisible to human eyes, the tiny invisible things that power the whole world. After all, eyes are only cameras (albeit extremely versatile ones), and like all cameras, they have a limit to how much they can zoom in. The desire to view the things beyond the ability of the eye has long captivated humans. Only in relatively recent history (compared with the entirety of human existence) have they succeeded in beating the eye in viewing power. An entire field of science has been created to delve into the depths of the physical world; its name is microscopy and here is its history.
SIMPLE AND COMPOUND LIGHT MICROSCOPY
As early as the thirteenth century, there is record of lenses in the form of water-filled glass spheres being used as magnifying glasses by jewelers to cut gems. Even before that, Euclid in the third century BCE had elucidated the mechanics of image formation with mirrors and Ptolemy in the second century CE had posited a description of refraction (1,2). Interest in lenses initially stemmed from the desire to correct vision dysfunctions and indeed, accurate corrections for nearsightedness and farsightedness with concave and convex lenses respectively were described by mid-sixteenth century Italian scientists (2).
At its simplest, a microscope is just an extremely strong magnifying glass. The technical distinction between vision correction lenses and a microscope is just the degree of magnification. Our eyes can at best see differences of about 0.1 mm. Anything that has a resolving power stronger than that, anything that can clearly show images finer than 0.1 mm, is thus considered a microscope (3). It’s rather difficult to pin down who created the first lenses that had this sort of magnifying power because the 0.1 mm threshold was not a specification that opticians recorded. However, we do know that by the mid-seventeenth century, Antonie van Leeuwenhoek, an amateur Dutch biologist, had essentially perfected the art of crafting single lens microscopes.
Van Leeuwenhoek was not particularly skilled at using the microscope, rather it was his expert craftmanship of the instrument itself that secured his place in the history of microscopy. Leeuwenhoek’s ability to grind lenses to minute focal lengths and mount them securely in the instrument resulted in the best single lens (as opposed to compound or even non optical) microscopy work.1 His best microscope could resolve close to one micron (10-6 meters) or a magnification of about 300 times. He is credited with discovering sperm cells and blood cells, producing the first detailed drawings of rotifera and infusoria, and obtaining the first images of bacteria (1,3). The clarity of van Leeuwenhoek’s simple microscope images would not be surpassed until over a century later, a testament to the quality of his microscopes.
Simple microscopes, though, are not the microscopes that most people first encounter in their high school biology classes. Those would be compound microscopes, and allegedly the first was invented by the father and son team of Hans and Zacharias Jansen near the end of the sixteenth century (3).
Compound microscopes, from a mechanical standpoint, are equivalent to an inverted telescope. They consist of two lenses with the second magnifying the image produced by the first lens both affixed to a tube that can move relative to the specimen (4). The system of lenses used in compound microscopes affords them tremendous range in magnification and some of the earliest work was done by Robert Hooke, an English scientist who produced stunning images of small animals and plant cells that showed off the power of the microscope.
ABERRATIONS
Despite the quality of Hooke’s drawings, compound microscopes lagged behind simple microscopes in image clarity. This was due to optical aberrations that emerge when light is passed through multiple lenses, namely, chromatic and spherical aberrations. As the name chromatic aberration implies, compound microscopes had trouble with accurately transmitting color. As Newton showed with his famous experiment with the prism, white light is comprised of all the different colors of light, each having a different wavelength. This difference in wavelength is what causes problems for a compound microscope. Light is bent different amounts depending on its wavelength with shorter wavelengths refracting the greatest and this results in each color focusing at a different point. Obviously, this is not ideal since the retina is fixed and cannot separate to accommodate different focal points. What results is an image that is ringed in colored halos (3).
The solution to this distortion came from two people, Chester Hall and John Dolland. Hall discovered that different types of glass dispersed colors different amounts (3). Dolland took that discovery and by experimenting, found that by combining two glass types and lens powers, he could bring the outlying colors of red and blue into focus and reducing the blur by a factor of ten (2). This set up is called an achromatic doublet—achromatic because it reduces chromatic aberrations and doublet because the lens is comprised of two pieces of glass. Though they were at first used only in telescopes because the large sized lenses were easier to craft, microscopes eventually caught up in the nineteenth century and the images produced were no longer color distorted.
The second major kind of distortion associated with compound microscopes was spherical aberration, which arose from the curved nature of small lenses. Light that strikes the edges of the lens is refracted significantly more than light striking near the center of the lens, resulting in a difference of focal length and a blurry image (5). Before the nineteenth century, there were two imperfect solutions to spherical aberrations. One approach was to use a lens with less curvature and consequently a smaller difference in refracting power between the edges and the center of the lens; however, this resulted in a lower magnifying power (3). The second approach was to limit the amount of light that could strike the lens to a narrow angle around the center, but this limited the resolution of the microscope.3 Both of these approaches did not allow for greater enlargement power. It wasn’t until the 1830s that the work of Joseph Lister managed to fix the problem.
Lister demonstrated that by lining up a series of lenses each with a small degree of magnification, only the first lens contributed to the spherical aberration (3). This resulted in high levels of magnification with minimal aberrations. Though the hassle of positioning and fixing the lenses limited its ease of use, compound microscopy’s stronger resolving power eventually won over scientists. To this day, compound microscopy is used commonly in all sorts of settings from high school biology classes to laboratory settings and is powerful enough to view bacteria in clarity.
SHIFT AWAY FROM OPTICAL MICROSCOPY
Optical microscopy is wonderfully convenient when viewing objects at the wavelength of visible light (approximately 400-700 nanometers), but with advances in theory in the early twentieth century, there was a need for better resolution in microscopy. Around this time, the French physicist Louis de Broglie theorized that electron beams could behave like waves and calculated that they would have a wavelength of about 5 picometers, or about 100,000 times smaller than visible light (6). This prediction paved the way for a new era of microscopy. Electron microscopes operated by shooting a beam of electrons rather than photons at a specimen and quickly surpassed optical microscopy in magnification.
Before diving into the advances in electron microscopes, it makes sense to reason out why electron microscopy returns such stunning results. It turns out the reason is that shorter wavelengths increase microscope resolution. Long waves have large spaces between successive peaks, and thus will pass over small specimens returning no signature and thus no image. Short waves, on the other hand, have peaks spaced much closer together and thus will hit the target and leave a detectable shadow or bounce back signature much in the same way that light leaves shadows when it hits an object. Though humans can’t see electrons like they can see photons, a fluorescent plate placed near the specimen is able to translate the invisible electrons into a visible image.
Electron microscopes, as one can imagine, are much more advanced than light microscopes. For starters, electrons cannot travel far in the atmosphere so electron microscopy must take place inside a vacuum (6). This increased difficulty in set up is balanced, however, by the fact that magnification is controlled not by accurately positioning the lenses and specimen, but by focusing the beam of electron to different degrees.6 Additionally, the microscope must also include a fluorescent plate to translate the scattered electrons into visible data, and as a result of all of the intricacies of electron microscopy, these microscopes tend to be bulky and finnicky in operation.
TRANSMISSION ELECTRON MICROSCOPY
In 1931, two German electrical engineers, Max Knoll and Ernst Ruska, succeeded in creating the first transmission electron microscope (TEM) (6). The instrument had essentially three components: a source of electrons, a series of lenses that directed the electrons through the specimen and to the detector, and equipment to interpret the electron signature (7). Similar to the way a light microscope displays a shadow where light could not pass through the specimen, the image produced via TEM shows relative levels of transmission of electrons throughout the specimen. “Darker” areas are more electron opaque while “lighter” areas are less so.
Unlike visible light which has a longer wavelength and is thus less energetic, electron beams tend to shred up lighter weight atoms resulting in large levels of scatter and a less contrasted image (3). To work around that, scientists must “stain” the specimen with heavier elements such as uranium, lead, and/or osmium (3). Though this allows for higher quality images, the staining process only adds to the list of difficulties of using TEMs. The microscopes are large, expensive, and difficult to operate and maintain. Suitable specimens must be able to withstand the complicated preparation process and vacuum imaging chamber, and the images produced are colorless. And finally, the stains result in a residue contaminated by heavy metal toxins that are difficult to dispose of properly.
SCANNING ELECTRON MICROSCOPY
As an alternative to the TEM, another German physicist, Manfred von Ardenne, described and produced the first images using a scanning electron microscope (SEM) in 1940 (6). The microscope was smaller than the TEM because it recorded electrons that bounced off the surface (secondary electrons) of the specimen with detectors placed closer to the source than the target (8). This is also what makes SEM images look three dimensional because rather than projecting the electron signature onto a single flat surface, the SEM measures electrons that travel at all angles and combines it to form an image with variable depth (3).
SEMs are also more amenable to researchers because the specimen preparation process is less involved. While specimens for TEM require extremely thin slicing and treatment with stains, SEM specimens only need a thin layer of conductive coating such as a gold film before being placed in the vacuum container, a process not even necessary if the electron beams are strong enough.8 The elimination of destructive preparation techniques has allowed researchers to image larger objects such as insects or small mechanical parts (3). The images produced show such fine details of everyday objects in new ways that they at times seem alien. The microscope is still expensive and needs to be precisely configured for optimal results, but overall, SEMs are easier to use compared to other forms of electron microscopy.
ATOMIC LEVEL MICROSCOPY
As if intracellular imaging weren’t impressive enough, the 1980’s brought the invention of the scanning tunneling microscope (STM), which has the ability to view individual molecules and atoms. This level of magnification grew out of a desire to describe the inner workings of the atom. Like electron microscopy, it relies on the wave nature of electrons (9). The first STM was built in 1981 by Swiss physicists Gerd Binnig and Heinrich Rohrer and was used to study the topography of a gold surface; it worked by measuring the level of electron tunneling at different points along the surface (9). STM relies on tunneling, a quantum mechanical phenomenon in which electrons have some probability of traveling through an impermeable surface, something unexplainable by classical mechanics. What’s useful about tunneling is that it is highly sensitive to distance, specifically, atomic level distances.
The STM operates with an extremely fine pointed probe positioned extremely close to the sample surface (3). Voltage applied to the tip allows for electrons to tunnel to the surface and the number of electrons that make it across the gap is correlated with the distance between the tip and the surface (9). The image produced by all the data is a topographical map of relative “heights,” which is useful because it can determine the atomic structure of the surfaces of different substances. STM has been used to investigate the arrangement of atoms of different metals and crystals and the details of absorption and diffusion of various gases on metal surfaces (9). The advantage of STM over other forms of electron microscopy, beyond its resolution, is that tunneling can take place in a variety of environments and does not need an external source of electrons or lens to focus the beam (3,9). This eliminates much of the specificity involved in undertaking electron microscopy and vastly opens up the domain of imageable surfaces. In fact, STM has been performed in water, ionic solutions, and even insulating fluids that wouldn’t allow electrons to travel conventionally (9).
As with any technology, there are complications that come with using STM. First, the STM setup is still rather complicated since the probe is extremely sensitive. A series of springs and magnets are used to counteract outside disturbances, and the movement of the probe is controlled via the piezoelectric effect where applied current generates compression or expansion of a material (3). The construction of the tip is also problematic because it is only one atom in width. This is accomplished by precisely manipulating extremely strong electric fields to shave off excess atoms. Despite the intricate nature of the STM equipment, the versatility of the probe makes it one of the most powerful imaging tools for modern scientists.
Microscopy has come a long way from its humble optical beginnings. Though born out of human curiosity and the desire to view the invisible world lurking all around us, microscopy has been instrumental for scientific and societal advancement. Being able to view bacteria and other pathogens validated germ theory allowing for the development of antibiotics and other therapies that have vastly improved the quality of life over the last century. Being able to view chemical reactions at the atomic level has advanced the efficiency of the chemical industry. And the recently awarded 2017 Nobel Prize in Chemistry was for biomolecular imaging techniques that have allowed for real time observation of biochemical processes.10 Microscopy will always be integral to scientific discovery, and the future of imaging techniques will undoubtedly have far reaching benefits for all humanity.
Kelvin Li ’21 is a freshman in Wigglesworth Hall.
WORKS CITED
[1] Singer, C. J. R. Soc. Med. 1914, 7, 247- 268.
[2] Walker, B. H. In Optical Engineering Fundamentals, 2nd ed.; SPIE Press: Bellingham, Wash, 2008; pp 5-11, 113-116.
[3] Croft, W. J. In Under the Microscope: A Brief History of Microscopy; Weiss, R. J., Ed.; Series in Popular Science; World Scientific Publishing Co.: Singapore, SG, 2006; 5, pp. 5-13, 57-81, 105-112.
[4] In A Dictionary of Physics; Oxford University Press: Oxford, UK, 2014; 7.
[5] Optical Abberations. https://www . olympus-lifescience.com/en/microscope-resource/primer/anatomy/ aberrations/ (accessed Sept. 9, 2017).
[6] Bradbury, S. et al. Electron Microscope. Britannica Academic [Online], Aug. 13, 2017. http://academic . eb.com (accessed Sept. 9, 2017).
[7] Bradbury, S. et al. Transmission Electron Microscope (TEM). Britannica Academic [Online], July 22, 2011. http://academic.eb.com (accessed Sept. 9, 2017).
[8] Bradbury, S. et al. Scanning Electron Microscope (SEM). Britannica Academic [Online], May 17, 2016. http:// academic.eb.com (accessed Sept. 9, 2017).
[9] Quate, C. F. Scanning Tunneling Microscope (STM). Britannica Academic [Online], Aug. 13, 2017. http:// academic.eb.com (accessed Sept. 9, 2017).
[10] Press Release: The Nobel Prize in Chemistry 2017. https://www.nobelprize.org/nobel_prizes/chemistry/ laureates/2017/press.html (accessed Oct. 8, 2017).
Share this:
Leave a comment cancel reply.

- Already have a WordPress.com account? Log in now.
- Subscribe Subscribed
- Copy shortlink
- Report this content
- View post in Reader
- Manage subscriptions
- Collapse this bar
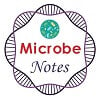
Microbe Notes
Microscopy: History, Classification, and Terms
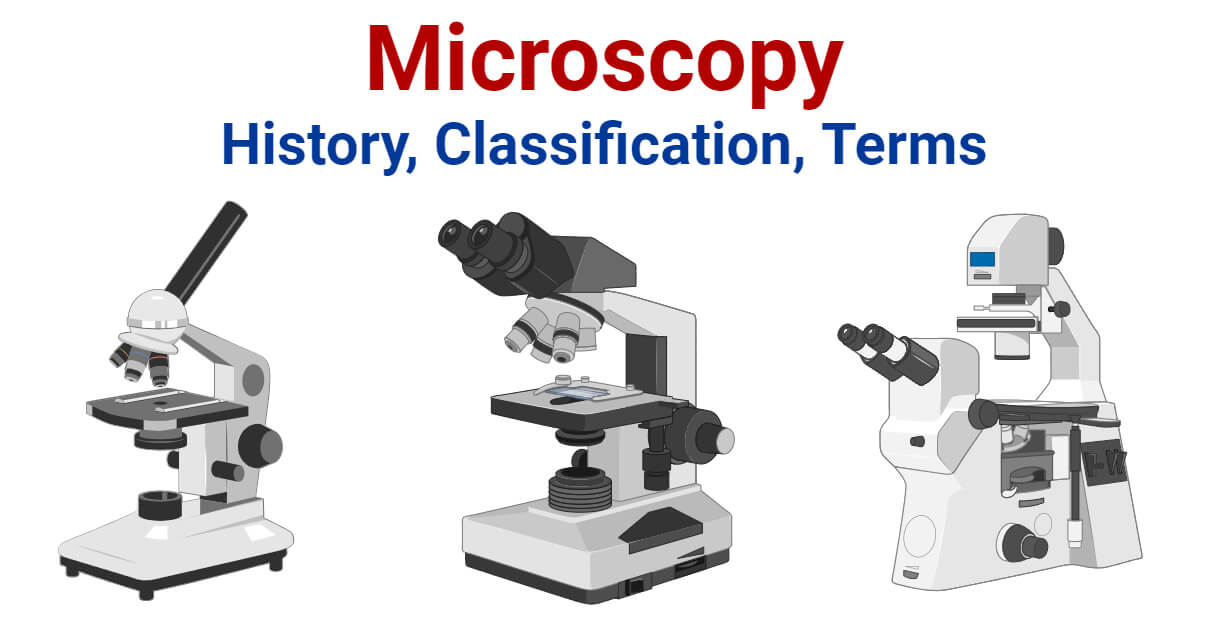
Table of Contents
Interesting Science Videos
History of Microscope
- In the 1 st Century AD, the Romans invented the glass and used them to magnify objects.
- In the early 14 th Century AD, eyeglasses were made by Italian spectacle makers.
- In 1590, two Dutch spectacle makers, Hans, and Zacharias Jansen created the first microscope. It was a simple tube with 2 lenses system and had 9X magnification.
- In 1670, Robert Hooke, an English Chemist, Mathematician, Physicist, and Inventor, improvise the microscope of that time and developed the compound microscope. He first developed the 3 lenses microscope.
- In 1675, Anton Van Leeuwenhoek ground a glass ball into a convex lens and used it to make a single-lens microscope with 270X magnification. Using this microscope, he first observed the bacterial cells.
- In 1729, Chester Moore Hall first presented and used achromatic lenses in the microscope.
- In 1830, Joseph Jackson Lister suggested the use of multiple low-power lenses to achieve clear magnification.
- In 1878, Ernst Abbe, a German Physicist and Optical Scientist, developed a mathematical theory relating wavelength with image resolution. He was the first to develop and use water and oil immersion lenses.
- In 1903, Richard Zsigmondy invented the ultramicroscope. This could view objects smaller than the wavelength of light.
- In 1932, Frits Zernike invented the phase-contrast microscope.
- In 1938, Max Knoll and Ernst Ruska invented the first electron microscope. It was a transmission electron microscope (TEM). It used a beam of an electron instead of light to make an enlarged image.
- In early 1940, Russian physicist Sergey Y. Sokolov developed concept of ultrasound microscope. But, only in 1970, its working model was developed in America.
- In 1942, Ernst Ruska improved TEM into a scanning electron microscope (SEM). In this type, electron beams are passed across the specimen instead of passing through it as in TEM.
- In 1951, British physicists William Nixon and Ellis Coslett invented X-ray microscope.
- In 1981, Gerd Binnig and Heinrich Rohrer invented the scanning tunneling microscope. This allowed us to get the 3-D image of an object.
What is Microscopy?
Microscopy can simply be understood as the ‘use of microscope’. Microscopy can be defined as the scientific discipline of using microscopes for getting a magnified view of objects that can’t be viewed by naked eyes.
It is a very important tool in biology and nanotechnology. In microbiology, it is one of the most important tools used in observing microbial cells. Medical sectors, pathology, histology, molecular biology, and cytology are in great debt of microscopy.
Microscopy Classification
A. optical microscopy (light microscopy).
Optical Microscopy (Light Microscopy) is the microscopy technique that uses transmitted visible light, either natural or artificial, for developing the image of an object. It is the most common type of microscopy. It is further classified into several groups;
1 Bright Field Microscopy
Bright Field Microscopy is the simplest and the most common type. A simple or compound light microscope is used in this technique. It uses transmitted visible light to develop magnified images.
It has a low contrasting capacity, low optical resolution, requires staining and has a limited magnification of around 1300X.
It is simple and can be used to observe living cells and microorganisms.
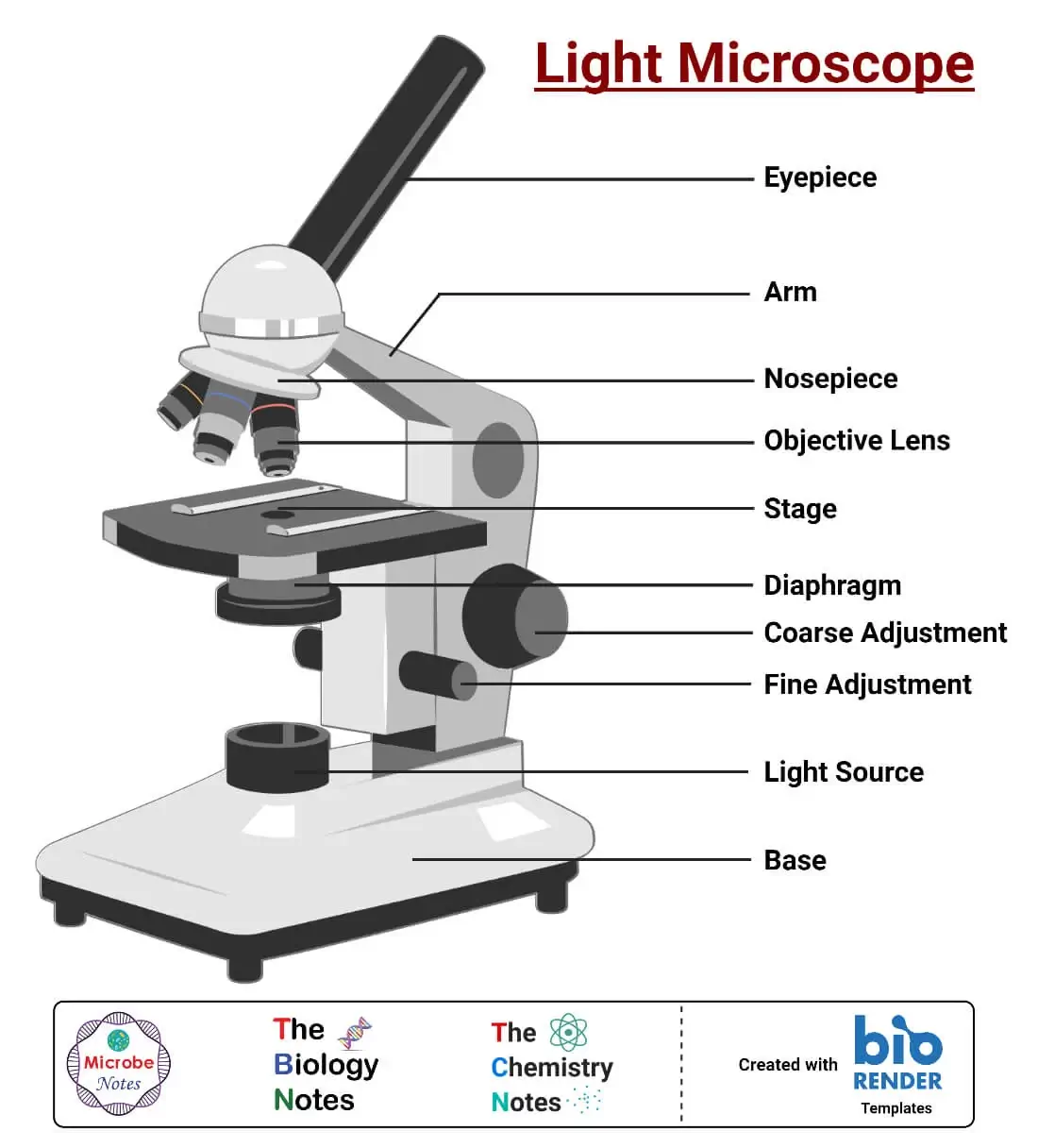
2 Dark Field Microscopy
Dark Field Microscopy uses dark-ground microscopes. The reflected light is used, instead of transmitted light, to form a magnified image.
It is used to observe very thin specimens and motility of flagella and cilia.
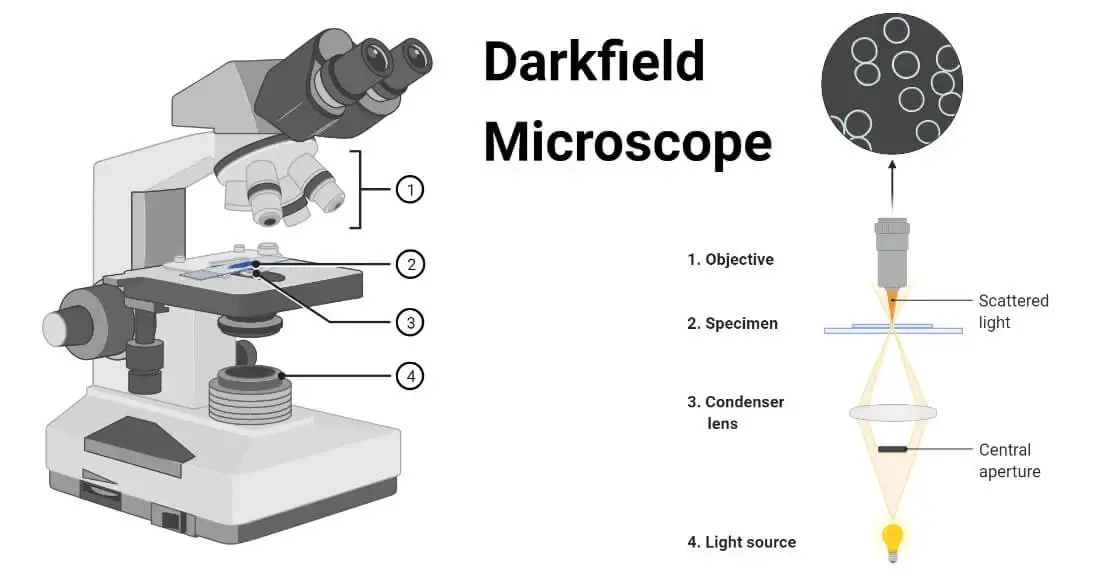
3 Phase Contrast Microscopy
In Phase Contrast Microscopy , the phase-contrast microscope is used. It converts phase shifts into differences in intensity of light-producing more contrast images.
It is used for observing living cells and cellular structures.
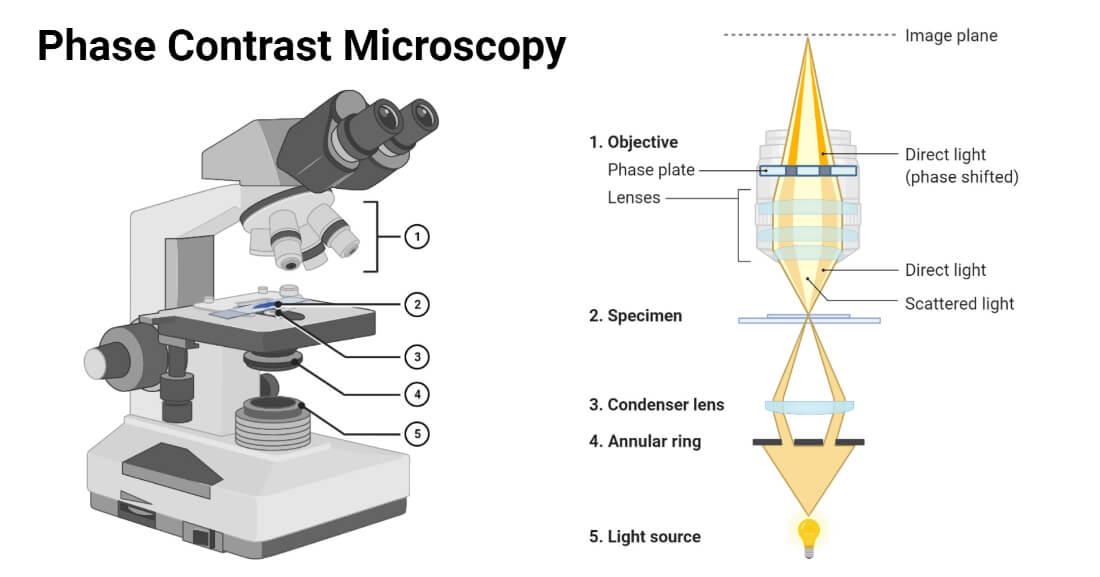
4 Fluorescence Microscopy
Fluorescence Microscopy is a microscopy technique that uses a fluorescent microscope with a UV light source. It is widely used in detecting antigens, antibodies, and other macromolecules.
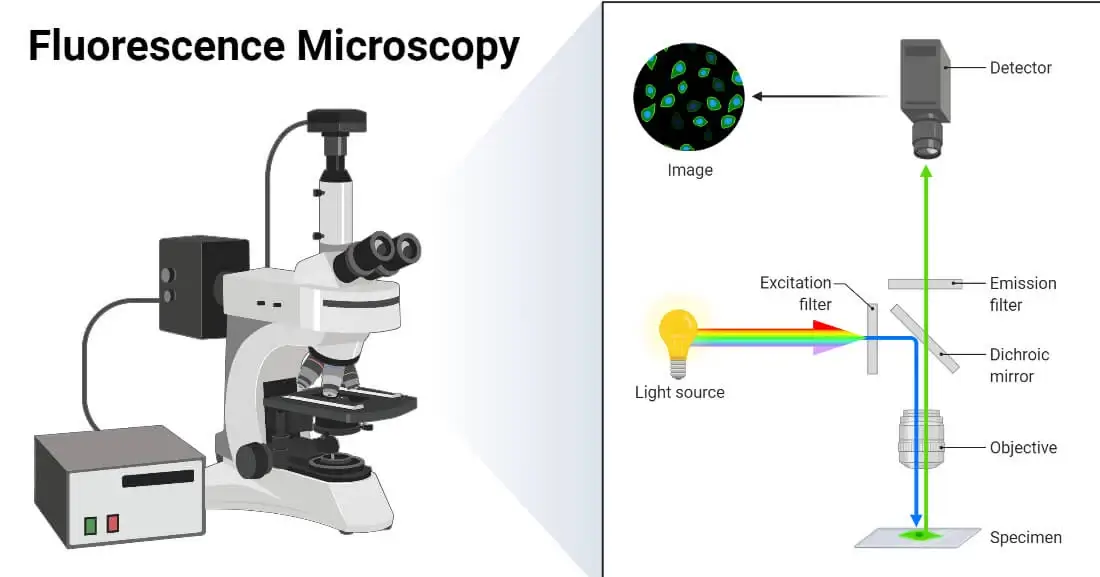
5. Confocal Microscopy
Confocal Microscopy is a newer microscopy technique that uses a focused laser beam. It is used to get high-resolution 3-D images of biological samples.

6. Differential Interference Contrast Microscopy
Differential Interference Contrast Microscopy is a newer microscopy technique used in unstained and transparent samples to enhance the contrast of their image.
B. Electron Microscopy
Electron Microscopy is a microscopy technique that uses a beam of electrons to develop a highly magnified image of microscopic samples.
In this technique, electron microscopes are used.
C. Scanning Probe Microscopy
Scanning Probe Microscopy is a microscopy technique that uses a physical probe to scan specimens and form magnified images. This measures the surface features of the specimen.
D. X-ray Microscopy
X-ray Microscopy is a microscopy technique that uses soft X-ray radiation to produce a magnified image of the specimen.
Microscopy Terms
What is magnification.
Magnification is the process of producing an enlarged image of a specimen by using a lens system. In a microscope, magnification can be computed by calculating the product of the magnification power of the eyepiece by the magnification power of the objective in use.
What is Magnifying Power?
Magnifying Power is defined as the ratio of the angle subtended by the image at the eye to the angle subtended by the object at the eye when placed at a minimum distance of distinct vision.
It can be mathematically defined as;
M=1+ D/F
M = magnifying power
D = least distance of distinct vision
F = Focal length of a convex lens
What is Refractive Index?
Refractive Index can be defined as velocity of light in a vacuum to velocity of light in a medium (substance). Simply it is the measure of bending of a light ray when passing from one medium to another.
Mathematically it can be defined as;
n = refractive index
c = speed of light in vacuum
v = velocity of light in a medium
What is Resolution?
Resolution can be defined as the shortest distance between two points on a specimen that can be distinguished by a microscope in its image. It is the ability of a microscope to distinguish details on a specimen.
Mathematically it is given as;
r = resolution
⋋ = imaging wavelength
NA = numerical aperture
- Images created using biorender.com
- Microscopy: Intro to microscopes & how they work (article) | Khan Academy
- What is Microscopy? (with pictures) (infobloom.com)
- Microscopy and Types of Microscopy (brainkart.com)
- What is Microscopy? | The University of Edinburgh
- Microscope Glossary of Terms: Microscope A-Z – Microscope and Laboratory Equipment Reviews (microscopespot.com)
- Resolution | Nikon’s MicroscopyU
- What is Microscope Resolution? – Microscope Clarity
- How does a microscope work? – Explain that Stuff
- Types of Microscopes: Definition, Working Principle, Diagram, Applications, FAQs (byjus.com)
- Fluorescence Microscopy – Principle, Components, Mechanism (noteshippo.com)
- https://www.olympus-lifescience.com/en/microscope-resource/primer/techniques/fluorescence/anatomy/fluoromicroanatomy/
- What is confocal microscopy ? | Cherry Biotech
- Fish K. N. (2009). Total internal reflection fluorescence (TIRF) microscopy. Current protocols in cytometry , Chapter 12 , Unit12.18. https://doi.org/10.1002/0471142956.cy1218s50
- Dark-field Microscopy: Principle and Uses • Microbe Online
- What is Dark Field Microscopy – Microscope Clarity
- Acoustic Microscopy – Benefits, Limitations and Uses (microscopemaster.com)
- X-Ray Spectroscopy Principle, Instrumentation and Applications (microbiologynote.com)
- Jacobsen, C. (2019). Index. In X-ray Microscopy (Advances in Microscopy and Microanalysis, pp. 573-579). Cambridge: Cambridge University Press.
- Principle, Instrumentation, Types and Applications of XRD (14impressions.in)
- Molecular Expressions Microscopy Primer: Specialized Microscopy Techniques – Polarized Light Microscopy – Compensators and Retardation Plates (fsu.edu)
About Author
Prashant Dahal
2 thoughts on “Microscopy: History, Classification, and Terms”
Superb notes
Leave a Comment Cancel reply
Save my name, email, and website in this browser for the next time I comment.
This site uses Akismet to reduce spam. Learn how your comment data is processed .

IMAGES