
An official website of the United States government
The .gov means it’s official. Federal government websites often end in .gov or .mil. Before sharing sensitive information, make sure you’re on a federal government site.
The site is secure. The https:// ensures that you are connecting to the official website and that any information you provide is encrypted and transmitted securely.
- Publications
- Account settings
Preview improvements coming to the PMC website in October 2024. Learn More or Try it out now .
- Advanced Search
- Journal List
- Front Bioeng Biotechnol

Editorial: Cell-Free Synthetic Biology
1 School of Physical Science and Technology, ShanghaiTech University, Shanghai, China
Yong-Chan Kwon
2 Department of Biological and Agricultural Engineering, Louisiana State University, Baton Rouge, LA, United States
3 Department of Chemical Engineering, Tsinghua University, Beijing, China
Simon J. Moore
4 School of Biosciences, University of Kent, Canterbury, United Kingdom
Cell-free systems have historically been used to decipher the genetic code and elucidate key principles of biological systems ( Silverman et al., 2020 ; Garenne et al., 2021 ). Recently, renewed scientific interest in cell-free systems has driven the rapid development of cell-free synthetic biology, including the establishment of new cell-free platforms, bottom-up design of genetic circuits and artificial cells, prototyping of metabolic pathways, and designing of medical diagnostics and biosensors, among others ( Lu, 2017 ; Moore et al., 2017 ; Liu et al., 2019 ; Copeland et al., 2021 ). Today, with the renaissance of cell-free biotechnology, more and more scientists are entering this field to explore the frontiers of synthetic biology. To highlight the current progress of cell-free research, this Research Topic aims to bring together diverse and exciting achievements in cell-free synthetic biology.
Here, we collected a total of 17 papers. These papers present a broad range of cell-free research across the development of new cell-free protein synthesis (CFPS) systems and construction of an educational “Genetic Code Kit.” The papers published in this topic are briefly introduced below.
Laohakunakorn et al. highlighted the current capabilities and challenges in the rapid growing field of cell-free synthetic biology. Due to the open nature of cell-free systems and breakthroughs in enabling technologies, bottom-up construction of increasingly complex biomolecular networks using cell-free systems will help address a wide range of fundamental biological questions such as the design of minimal life.
Kelwick et al. introduced key concepts and recent advancements in cell-free synthetic biology, with a focus on examples relevant to the materials sciences. In particular, the biological materials of industrial and societal importance manufactured by cell-free systems were summarized. While some challenges still remain, cell-free synthetic biology together with interdisciplinary collaborations and emerging technologies will lead to a new frontier for sustainable cell-free materials production and the growing bioeconomy.
Wu et al. summarized recent progresses on the efficient and extensive incorporation of non-canonical amino acids into proteins using CFPS. Different methods were used to increase the incorporation efficiency by elimination of endogenous competition factors, engineering of protein translation factors, and in vitro aminoacylation.
McSweeney and Styczynski reviewed the strategies of using linear DNA as expression templates in CFPS systems. Using linear DNA templates has some advantages, however, they can be quickly degraded by native exonucleases present in the cell extracts. This review described several approaches to stabilize linear DNA and compared their effectiveness and limitations, as well as future developments and applications.
Müller et al. introduced the modelling approaches and applications of CFPS systems, which can lay the foundation for understanding the production and degradation dynamics of macromolecules in CFPS. With the help of CFPS models, bottlenecks in the transcription and translation processes can be identified and key kinetic parameters can be determined from the modelled data, to aid the future development of enhanced CFPS systems.
Two papers discussed synthetic cells with different focuses. Damiano and Stano presented their opinion on the “life-likeness” of synthetic cells. Based on previous work, they promoted an “organizational approach” to the assessment of life-likeness and proposed the transition from behavioral assays (e.g., Turing test) to systemic strategies according to concepts like organization, complexity, networks, and emergence. Abil and Danelon pointed out in their perspective article that life is more than the sum of its constituted parts. These authors proposed a semi-rational, system’s level evolutionary approach for bottom-up building a synthetic cell.
Lin et al. developed a flow platform by combining CFPS systems and a tube-in-tube reactor that can measure and analyze the effect of oxygen on protein synthesis. Using this platform, energy, transcription, translation, and pathway networks in the reaction system were analyzed. The results showed that a maximum protein yield could be obtained with 21% oxygen, which is the same as the natural atmosphere oxygen condition.
Zhang et al. established a eukaryotic Pichia pastoris -based CFPS system. After a systematic optimization, this cell-free system was able to synthesize 50 μg/ml of protein, which is comparable to other eukaryotic CFPS platforms. As a result, it is a valuable addition to the current CFPS toolbox and will provide an alternative for synthesizing complex proteins requiring post-translational modifications.
Williams et al. reported the transformation of the research-based CFPS biotechnology into a hands-on module called the “Genetic Code Kit” for implementation into teaching laboratories. This kit can help improve students’ understanding of transcription and translation and prepare undergraduate students for careers in laboratory research.
Kim et al. reported the production of monomeric filaggrin, a human therapeutic protein, using an E. coli -based CFPS system. By relieving the limiting factors in transcription and translation, both protein yield and solubility were significantly improved with the enhanced CFPS system. This work demonstrates the potential of the E. coli CFPS to be adapted for studying therapeutic proteins.
Yang et al. designed modular cell-free systems for tunable biosynthesis of value-added chemicals. This approach was able to build long metabolic pathways (e.g., 6-7 enzymes) in vitro for defined biotransformation. Using two aromatic compounds (sharing the same upstream intermediate styrene) as examples, modular CFPS systems showed more than 80% conversion rates based on the same starting substrate of L-phenylalanine.
Arce et al. investigated cell-free RNA sensors using in-house made cell extracts instead of the commercial PURE CFPS system. CRISPRi-based technology was used to engineer the source strain to obtain nucleases silenced lysates, enabling CFPS from linear PCR-derived templates with high stability in the reaction. The home-made cell lysates were cost-effective and capable of virus detection using cell-free RNA toehold sensors.
Yue et al. described a systematic method for the expression and functional characterization of aquaporins based on an E. coli CFPS system, including template design, aquaporin expression and purification, proteoliposome preparation, and an aquaporin water permeability assay. This protocol can be adapted for the preparation of samples involving other channel proteins or transporters for their function assays.
Heide et al. provided a detailed protocol for the stepwise development and optimization of a CFPS platform derived from mammalian Chinese hamster ovary (CHO) cells. With the optimized CHO CFPS system, the protein yields were significantly improved, making it a useful research tool and a rapid production and screening platform for therapeutic protein development.
In addition to the above described cell lysates based cell-free systems, two research papers report the use of purified enzymes to implement in vitro biocatalysis. Fehlau et al. constructed a one-pot enzymatic cascade reaction for the synthesis of natural and modified nucleotides. Especially, a (d)ATP regeneration system was coupled in the reaction, leading to high-yielding production of nucleotides from nucleosides with high conversion rates. Liu et al. utilized T4 phage capsid as a protein scaffold for the immobilization of a three-enzyme cascade through SpyTag/SpyCatcher pairing. By doing this, the spatially organized enzymes exhibited higher enzymatic activity than the free enzymes, demonstrating the naturally occurring T4 phage scaffold is adaptable for multi-enzyme cascade assembly with enhanced biocatalytic activity.
In summary, the articles collated in this Research Topic demonstrate the potential of cell-free synthetic biology for a wide range of applications. Looking forward, cell-free synthetic biology research will continue to expand further to address current challenges in the field of synthetic biology when cell-based platforms are difficult or not amenable. With that, we now launch the second Research Topic on “Cell-Free Synthetic Biology, Volume II” and warmly welcome your significant contribution and submission.
Author Contributions
All authors listed have made a substantial, direct and intellectual contribution to the work and approved it for publication.
Conflict of Interest
The authors declare that the research was conducted in the absence of any commercial or financial relationships that could be construed as a potential conflict of interest.
Publisher’s Note
All claims expressed in this article are solely those of the authors and do not necessarily represent those of their affiliated organizations, or those of the publisher, the editors and the reviewers. Any product that may be evaluated in this article, or claim that may be made by its manufacturer, is not guaranteed or endorsed by the publisher.
- Copeland C. E., Langlois A., Kim J., Kwon Y.-C. (2021). The Cell-free System: A New Apparatus for Affordable, Sensitive, and Portable Healthcare . Biochem. Eng. J. 175 , 108124. 10.1016/j.bej.2021.108124 [ CrossRef ] [ Google Scholar ]
- Garenne D., Haines M. C., Romantseva E. F., Freemont P., Strychalski E. A., Noireaux V. (2021). Cell-free Gene Expression . Nat. Rev. Methods Primers 1 , 49. 10.1038/s43586-021-00046-x [ CrossRef ] [ Google Scholar ]
- Liu W.-Q., Zhang L., Chen M., Li J. (2019). Cell-free Protein Synthesis: Recent Advances in Bacterial Extract Sources and Expanded Applications . Biochem. Eng. J. 141 , 182–189. 10.1016/j.bej.2018.10.023 [ CrossRef ] [ Google Scholar ]
- Lu Y. (2017). Cell-free Synthetic Biology: Engineering in an Open World . Synth. Syst. Biotechnol. 2 , 23–27. 10.1016/j.synbio.2017.02.003 [ PMC free article ] [ PubMed ] [ CrossRef ] [ Google Scholar ]
- Moore S. J., MacDonald J. T., Freemont P. S. (2017). Cell-free Synthetic Biology for In Vitro Prototype Engineering . Biochem. Soc. Trans. 45 , 785–791. 10.1042/bst20170011 [ PMC free article ] [ PubMed ] [ CrossRef ] [ Google Scholar ]
- Silverman A. D., Karim A. S., Jewett M. C. (2020). Cell-free Gene Expression: an Expanded Repertoire of Applications . Nat. Rev. Genet. 21 , 151–170. 10.1038/s41576-019-0186-3 [ PubMed ] [ CrossRef ] [ Google Scholar ]
Session commentaries: synthetic and constructive biology
- Published: 20 August 2024
Cite this article
- Tomoaki Matsuura 1 na1 &
- Sheref S. Mansy 2 , 3 na1
Synthetic biology is a broad field with a unifying theme of learning through building. At IUPAB2024 in Kyoto, Japan, five researchers gave lectures focusing on in vitro synthetic biology, where the built objects were not living cells but in vitro systems composed of biomolecules. The talks sparked lively discussions on the knowledge gained about living cells from in vitro reconstructions.
This is a preview of subscription content, log in via an institution to check access.
Access this article
Subscribe and save.
- Get 10 units per month
- Download Article/Chapter or eBook
- 1 Unit = 1 Article or 1 Chapter
- Cancel anytime
Price includes VAT (Russian Federation)
Instant access to the full article PDF.
Rent this article via DeepDyve
Institutional subscriptions

Similar content being viewed by others
Synthetic Biology and Darwinism
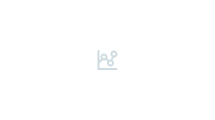
Who are the users of synthetic DNA? Using metaphors to activate microorganisms at the center of synthetic biology
Erasing borders: a brief chronicle of early synthetic biology, data availability.
Should any raw data files be needed they are available from the corresponding author upon reasonable request.
Aw R, Spice AJ, Polizzi KM (2020) Methods for expression of recombinant proteins using a Pichia pastoris cell-free system. Curr Protoc Protein Sci 102:e115. https://doi.org/10.1002/cpps.115
Eto S, Matsumura R, Shimane Y et al (2022) Phospholipid synthesis inside phospholipid membrane vesicles. Commun Biol 5:1016. https://doi.org/10.1038/s42003-022-03999-1
Article CAS PubMed PubMed Central Google Scholar
Furuta K (2023) Making motors work - potential applications in biocomputing and synthetic biology. J Cell Sci 136. https://doi.org/10.1242/jcs.261035
Ibusuki R, Morishita T, Furuta A et al (2022) Programmable molecular transport achieved by engineering protein motors to move on DNA nanotubes. Science 375:1159–1164. https://doi.org/10.1126/science.abj5170
Article CAS PubMed Google Scholar
Makrydaki E, Donini R, Krueger A et al (2024) Immobilized enzyme cascade for targeted glycosylation. Nat Chem Biol 20:732–741. https://doi.org/10.1038/s41589-023-01539-4
Nader S, Baccouche A, Connolly F et al (2023) Model atmospheric aerosols convert to vesicles upon entry into aqueous solution. ACS Earth Space Chem 7:252–259. https://doi.org/10.1021/acsearthspacechem.2c00328
Sword TT, Abbas GSK, Bailey CB (2024) Cell-free protein synthesis for nonribosomal peptide synthetic biology. Front Nat Prod 3. https://doi.org/10.3389/fntpr.2024.1353362
Toparlak ÖD, Sebastianelli L, Egas Ortuno V et al (2023) Cyclophospholipids enable a protocellular life cycle. ACS Nano 17:23772–23783. https://doi.org/10.1021/acsnano.3c07706
Venter JC, Glass JI, Hutchison CA 3rd, Vashee S (2022) Synthetic chromosomes, genomes, viruses, and cells. Cell 185:2708–2724. https://doi.org/10.1016/j.cell.2022.06.046
Download references
This symposium was in part supported by Iketani Science and Technology Foundation, No 0364003-D (TM).
Author information
Tomoaki Matsuura and Sheref S. Mansy contributed equally to this work.
Authors and Affiliations
Earth-Life Science Institute, Tokyo Institute of Technology, 2-12-1E Ookayama, Meguro-Ku, Tokyo, 152-8550, Japan
Tomoaki Matsuura
Department of Chemistry, University of Alberta, Edmonton, Alberta, T6G 2N4, Canada
Sheref S. Mansy
DiCIBIO, University of Trento, Trento, Italy
You can also search for this author in PubMed Google Scholar
Contributions
TM and SM wrote the main manuscript text and reviewed the manuscript.
Corresponding authors
Correspondence to Tomoaki Matsuura or Sheref S. Mansy .
Ethics declarations
Competing interests.
The authors declare no competing interests.
Additional information
Publisher's note.
Springer Nature remains neutral with regard to jurisdictional claims in published maps and institutional affiliations.
Rights and permissions
Springer Nature or its licensor (e.g. a society or other partner) holds exclusive rights to this article under a publishing agreement with the author(s) or other rightsholder(s); author self-archiving of the accepted manuscript version of this article is solely governed by the terms of such publishing agreement and applicable law.
Reprints and permissions
About this article
Matsuura, T., Mansy, S.S. Session commentaries: synthetic and constructive biology. Biophys Rev (2024). https://doi.org/10.1007/s12551-024-01219-0
Download citation
Received : 25 July 2024
Accepted : 13 August 2024
Published : 20 August 2024
DOI : https://doi.org/10.1007/s12551-024-01219-0
Share this article
Anyone you share the following link with will be able to read this content:
Sorry, a shareable link is not currently available for this article.
Provided by the Springer Nature SharedIt content-sharing initiative
- In vitro synthetic biology
- Cell-free protein synthesis
- Bottom-up biology
- Find a journal
- Publish with us
- Track your research
Synthetic Biology 101
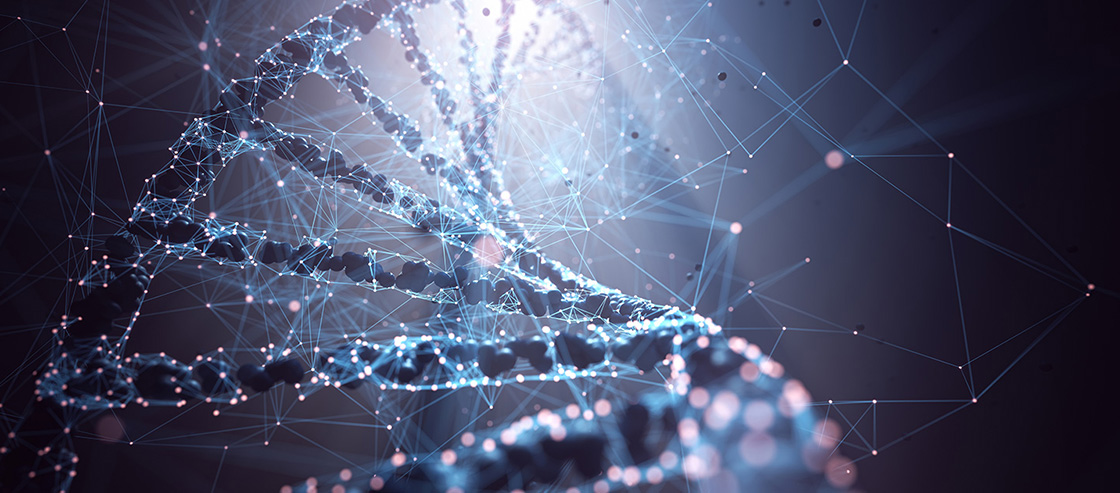
What is Synthetic Biology?
Synthetic biology is a discipline that uses biological components as building blocks in the design of new systems to solve crucial global challenges
By combining concepts across disciplines, researchers can build with biology in much the same way an engineer creates a high-tech device; but instead of the program running on a computer, it does so within a biological system. Advances in DNA sequencing, DNA synthesis, machine learning, and artificial intelligence have fueled synthetic biology’s growth, allowing for the development and scaling of new bioengineered solutions. These solutions can interface with every facet of our lives.
As estimated by the National Academies of Sciences, Engineering and Medicine, the bioeconomy is about five percent of US gross domestic product (one trillion dollars) and growing, compared to the approximate one percent that represents the U.S. semiconductor industry. Investments in the bioeconomy have given rise to billion-dollar companies that rely on synthetic biology for their products. Often, these companies are using biology to redesign traditional processes to produce familiar items in ways that are more environmentally sustainable.
Synthetic Biology Technology is All Around Us
Examples of synthetic biology appear everywhere
In agriculture, synthetic biologists have developed microbes that can make their own fertilizer, a development that could combat global hunger by increasing crop yield and aiding farmers in some of the world’s poorest regions. In the commodities industry, synthetic biologists have engineered a bacterium that can pull carbon dioxide and carbon monoxide out of the air, turning them into common chemicals like acetone and isopropanol—redirecting carbon emissions that are normally funneled into the atmosphere. In medicine, synthetic biology innovations such as mRNA vaccines are being used as tools to treat infectious diseases, helping public health experts and society stay a step ahead of the next pandemic and providing new approaches to teach our own immune systems to better detect and eliminate cancers.
The grocery store offers an even more well-known product of synthetic biology’s potential and reach. The Impossible Burger, a popular meat alternative, uses a lab-engineered non-meat-based heme molecule to help its product look and taste more like a traditional beef hamburger. The difference, according to an environmental analysis by independent auditor Quantis, is that production of one Impossible Burger patty uses 96 percent less land and 87 percent less water as compared to one beef patty, while also releasing 89 percent less carbon into the atmosphere. Such advances show just how significant synthetic biology’s benefits can be.
Investing in the Future
Synthetic biology is a national priority
The U.S. government took an early interest in synthetic biology research, with the National Science Foundation (NSF) providing a $37 million, 10-year grant in 2006 to fund a national Synthetic Biology Engineering Research Center. DARPA followed suit with multiple programs focused on synthetic biology. From 2008 to 2014, estimates suggest the government invested $820 million in synthetic biology research, spread across the NSF, the Department of Energy, the Department of Defense, and the National Institutes of Health (NIH).
The U.S. government underscored its intention to accelerate the growth of synthetic biology research when the White House issued an executive order in September 2022, launching a national biotechnology and biomanufacturing initiative that places synthetic biology as a centerpiece of our strategies for sustainability, competitiveness, and economic growth across all levels of government. The order’s impact has the potential to be far reaching including: significant investments to develop medicines and commodities, reduce waste, and advance sustainable farming, while also mitigating climate change impacts.
A Brief History
History in the Making
The roots of synthetic biology are planted with a landmark investigation by researchers François Jacob and Jacques Monod, whose study of E. coli led them to posit the existence of regulatory circuits that underpin the response of a cell to its environment.
January 2000
Two side-by-side reports are published by researchers at Boston University and Princeton University showing that biological protein parts can be engineered into genetic circuits to carry out designed functions.
January 2003
MIT launches an independent study course in which students develop biological devices to make cells blink; the following year the course evolves into a summer competition known as iGEM (International Genetically Engineered Machines). Northwestern would go on to launch its first iGEM program in 2010.
The first international conference for the field, Synthetic Biology 1.0, is held at MIT; future conferences in Switzerland and Hong Kong would help to establish the field’s global growth.
BioBricks Foundation is established as a nonprofit organization to catalog and standardize synthetic biology parts, providing a database of resources for scientists and researchers.
Funded by a 10-year grant from the National Science Foundation (NSF), the Synthetic Biology Engineering Research Center (SynBERC) is founded to advance research, innovation, training, and education in synthetic biology. In this time, SynBERC trains and provides early career support to future Northwestern synthetic biology faculty.
The World Health Organization approves the use of a semi-synthetic, non-plant-derived version of the antimalarial drug artemisinin as a low-cost alternative to treat malaria in developing nations—the first large-scale synthetic biology commercial endeavor.
The US Department of Energy prepares a report to Congress on synthetic biology, offering a comprehensive plan for federally supported research and development in support of energy and environmental goals.
Northwestern University launches its interdisciplinary Center for Synthetic Biology (CSB).
A group of leading scientists, including Northwestern CSB faculty, proposes a large-scale synthetic biology initiative, leading to the creation of the Human Genome Project-Write, which is focused on the synthesis of human genomes.
Northwestern University receives an NSF grant to fund a 10-week summer research experience in synthetic biology for undergraduate students, the first synthetic biology-focused, NSF-funded program of its kind.
September 2018
After SynBERC’s 10-year funding grant ends, Northwestern CSB faculty help found the Engineering Biology Research Consortium with an NSF award to support and sustain the impact of research, products, discoveries, and ideas from the synthetic biology community.
Northwestern University receives a $3 million NSF grant to support Synthesizing Biology Across Scales (SynBAS), a program focused on convergent synthetic biology training for graduate students, another first-of-its-kind synthetic biology-focused program.
September 2022
The United States launches a national biotechnology and biomanufacturing initiative.
share this!
August 24, 2024
This article has been reviewed according to Science X's editorial process and policies . Editors have highlighted the following attributes while ensuring the content's credibility:
fact-checked
peer-reviewed publication
trusted source
The promise of synthetic cells
by Lawrence Goodman, National Institute of Standards and Technology
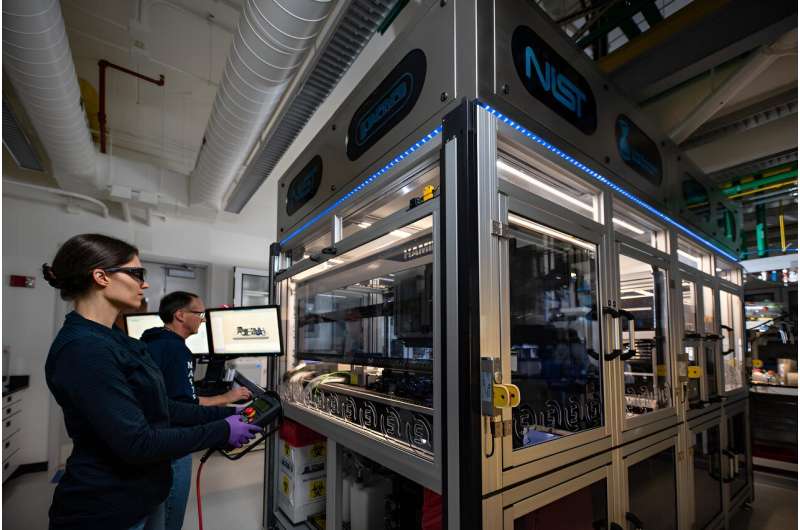
For over a decade, scientists have made extraordinary progress on the long-held dream of fabricating an entire cell from nonliving molecules and materials.
Such synthetic (or " engineered ") cells would behave similarly to the ones in our bodies, though they would also have built-in safeguards that ensure safety and ethics. By studying them, we could transform our understanding of the rules of life. They could also be used to manipulate living organisms and achieve astounding breakthroughs in medicine and science.
In 2010, the J. Craig Venter Institute announced it had created the first "self-replicating, synthetic bacterial cell" containing a genome synthesized outside the cell and then transplanted into it. It was then able to divide and reproduce according to instructions from its new DNA code.
Since then, researchers have only grown more ambitious, seeking to synthesize other cellular components and build a whole cell from scratch.
"We're closer than we've ever been before," said National Institute of Standards and Technology physicist Elizabeth A. Strychalski . The quest to create a synthetic cell from scratch "is a capability that is, if not on our doorstep, maybe, you know, at our mailbox."
Much of the recent progress rests on technological advances that have made it easier and cheaper to synthesize long strands of DNA in laboratories.
Scientists worldwide have also figured out ingenious methods for producing basic versions of membranes, mitochondria and other cellular components. And using new techniques that allow them to manipulate tiny amounts of fluid, they are beginning to coax these synthesized cell parts into interacting and communicating.
At NIST, Strychalski's research group is helping to establish the measurements and standards foundational to further progress in engineering biology (also called synthetic biology ).
NIST is also collaborating with the J. Craig Venter Institute on the " minimal cell ," a stripped-down synthetic cell. Instead of multiple synthetic parts and components, only its genome is synthesized. Strychalski said the minimal cell will help researchers achieve "the holy grail of understanding what every single gene in the human cell does."
We spoke with her about her work and a recent paper she co-authored in ACS Synthetic Biology that explores the state of research in her field.
Let's start with the most fundamental question. How will we know when we've built a synthetic cell from scratch?
It will likely have some important properties, like the ability to replicate, a metabolism, and some kind of internal organization or compartmentalization. Some properties emerge when you begin to assemble the components of a cell, such as the ability to respond to some kind of stimuli in your surroundings and the ability to move.
Now, will we require that our synthetic cell have all or just some of these properties? That's still an open question and will depend on its application. But certainly, these are all attributes of a synthetic cell built from scratch that we would eventually want to incorporate.
How could such synthetic cells be used to treat disease?
So much of what makes people sick can be traced back to cells not working properly.
So, let's say cells are taken as a capsule, and we've engineered them to sense when there's a certain disease state. It could be that some harmful bacteria is poisoning you, or your body is missing the ability to make a certain protein.
The synthetic cells could fix this by maybe killing those harmful bacteria or helping your body make all the molecules it's supposed to make so that you don't have that disease.
You write in your journal article about the role synthetic cells could play in space exploration.
One of the exciting things about building synthetic cells is that we get to think about making synthetic cells or cell-like systems that could be much better adapted to a space environment, whether that's in a spaceship or on the surface of another planet.
There's also so much opportunity to use cells as factories for making products, medicine, building materials, food or whatever you might need in these resource-limited settings. And what's nice about synthetic cells is you don't have to leave the earth's surface with very much of them to grow them in space, where you might want a whole lot of them.
Could we synthesize designer cells or cell-like systems that explore biological diversity beyond what currently exists in nature?
You know, we study cells as they evolved on Earth. We don't know how much of what we see now was because it had to happen that way and couldn't have happened any other way.
How can we go into the laboratory, roll back the clock, and look at other possibilities?
For example, the nucleic acids in DNA are made from four bases — adenine (A), thymine (T), guanine (G) and cytosine (C). It's possible to make additional bases in the laboratory that we don't find in nature that seem to work just as well.
How can we ensure ethics and safety?
It's essential that everyone has a say in how we develop these technologies, how we use them and who has access to them.
We now have the opportunity to build in safety instead of looking back and trying to put the safety back in. So, I'm a big fan of starting with a safety mindset.
For example, how do we ensure that synthetic cells cannot grow outside of where we want them to be? Can we build "kill switches" inside synthetic cells? When they exit your body, they might sense the temperature difference, triggering a stress response in the cells that causes them to die.
Another thing we need is robust screening measures to ensure that people who are ordering synthetic snippets of DNA code or synthetic cells aren't ordering ones that could be harmful.
Tell us about NIST's role in all of this.
I like to think of building synthetic cells from the perspective of control. Researchers are trying to control the function of these systems and do it safely.
To have this kind of control, we need to measure what the system is doing quantitatively with confidence. How else are we going to know it's achieved its intended function?
And we're thinking about ways that these synthetic cells can do the measuring. It's about building biomolecular circuits to make measurements and even perform computations inside living systems.
What motivates you in your research?
We are in the biotechnological revolution right now. We have real problems that we need to face as a society, and we need biotechnologies to help us solve those problems.
It's also about discovery. Once we understand how to build synthetic cells from scratch, we can better understand what it means to be human.
Journal information: ACS Synthetic Biology
Provided by National Institute of Standards and Technology
Explore further
Feedback to editors

Exploring peptide clumping for improved drug and material solutions
3 hours ago

Large sharks may be hunting each other—and scientists know because of a swallowed tracking tag

Human mouth bacteria reproduce through rare form of cell division, research reveals
13 hours ago

Chemists create gel to prevent leaks and boost lithium-ion battery life

Study suggests gun-free zones do not attract mass shootings

Double-peaked supernovae offer clues to pre-supernova outbursts
15 hours ago

New discovery of how bacteria navigate their environment could change how we treat infection

Engineers smash rocks to see what occurs when top layer of an asteroid-like object is hit with extreme external force

Copper-based catalyst paves the way for sustainable ammonia production
16 hours ago

Electricity generated by earthquakes might be the secret behind giant gold nuggets
17 hours ago
Relevant PhysicsForums posts
The predictive brain (stimulus-specific error prediction neurons).
Sep 1, 2024
Any suggestions to dampen the sounds of a colostomy bag?
Aug 31, 2024
Will cryosleep ever be a reality?
Aug 30, 2024
Any stereo audio learning resources for other languages?
Aug 25, 2024
Cannot find a comfortable side-sleeping position
Therapeutic interfering particle.
Aug 24, 2024
More from Biology and Medical
Related Stories

Researchers develop 'founding document' on synthetic cell development
May 3, 2024

Researchers create artificial cells that act like living cells
Apr 23, 2024

Scientists create simple synthetic cell that grows and divides normally
Mar 29, 2021

Researchers create Cyborg Cells—natural-artificial cell hybrids
Jan 11, 2023

Artificial cells react to environmental changes
Jan 23, 2020

'Synthetic' cell shown to follow chemical directions and change shape, a vital biological function
Jun 12, 2024
Recommended for you

Cytophysics: How cells migrate through gaps smaller than their nucleus
18 hours ago

Cells use alternative splicing to regulate gene expression, research suggests
23 hours ago

Scientists discover molecular mechanism that plays key role in gene transcription and macrophage functional activation

AI tool maps out cell metabolism with precision
Let us know if there is a problem with our content.
Use this form if you have come across a typo, inaccuracy or would like to send an edit request for the content on this page. For general inquiries, please use our contact form . For general feedback, use the public comments section below (please adhere to guidelines ).
Please select the most appropriate category to facilitate processing of your request
Thank you for taking time to provide your feedback to the editors.
Your feedback is important to us. However, we do not guarantee individual replies due to the high volume of messages.
E-mail the story
Your email address is used only to let the recipient know who sent the email. Neither your address nor the recipient's address will be used for any other purpose. The information you enter will appear in your e-mail message and is not retained by Phys.org in any form.
Newsletter sign up
Get weekly and/or daily updates delivered to your inbox. You can unsubscribe at any time and we'll never share your details to third parties.
More information Privacy policy
Donate and enjoy an ad-free experience
We keep our content available to everyone. Consider supporting Science X's mission by getting a premium account.
E-mail newsletter
Information
- Author Services
Initiatives
You are accessing a machine-readable page. In order to be human-readable, please install an RSS reader.
All articles published by MDPI are made immediately available worldwide under an open access license. No special permission is required to reuse all or part of the article published by MDPI, including figures and tables. For articles published under an open access Creative Common CC BY license, any part of the article may be reused without permission provided that the original article is clearly cited. For more information, please refer to https://www.mdpi.com/openaccess .
Feature papers represent the most advanced research with significant potential for high impact in the field. A Feature Paper should be a substantial original Article that involves several techniques or approaches, provides an outlook for future research directions and describes possible research applications.
Feature papers are submitted upon individual invitation or recommendation by the scientific editors and must receive positive feedback from the reviewers.
Editor’s Choice articles are based on recommendations by the scientific editors of MDPI journals from around the world. Editors select a small number of articles recently published in the journal that they believe will be particularly interesting to readers, or important in the respective research area. The aim is to provide a snapshot of some of the most exciting work published in the various research areas of the journal.
Original Submission Date Received: .
- Active Journals
- Find a Journal
- Proceedings Series
- For Authors
- For Reviewers
- For Editors
- For Librarians
- For Publishers
- For Societies
- For Conference Organizers
- Open Access Policy
- Institutional Open Access Program
- Special Issues Guidelines
- Editorial Process
- Research and Publication Ethics
- Article Processing Charges
- Testimonials
- Preprints.org
- SciProfiles
- Encyclopedia
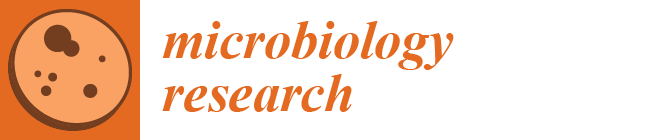
Article Menu
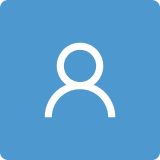
- Subscribe SciFeed
- Recommended Articles
- Google Scholar
- on Google Scholar
- Table of Contents
Find support for a specific problem in the support section of our website.
Please let us know what you think of our products and services.
Visit our dedicated information section to learn more about MDPI.
JSmol Viewer
Multifaceted applications of synthetic microbial communities: advances in biomedicine, bioremediation, and industry.
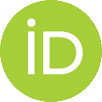
1. Introduction
2. definition and importance of microbial communities, 2.1. synthetic microbial communities, 2.2. artificial communities, 2.3. semi-synthetic communities, 3. interactions in microbial communities, 4. methodologies and tools in design of synthetic microbial communities, 4.1. computational models, 4.2. importance of genetic circuits in microbial communities, 4.3. crispr, 4.4. quorum sensing (qs), 5. applications of synthetic microbial communities, 5.1. biomedicine and health, 5.2. bioremediation and industry, 6. bioethics: potential risks in implementing synthetic microbial communities, 7. conclusions, author contributions, institutional review board statement, informed consent statement, data availability statement, acknowledgments, conflicts of interest.
- Callieri, C.; Eckert, E.M.; Di Cesare, A.; Bertoni, F. Microbial Communities. In Encyclopedia of Ecology , 2nd ed.; Fath, B., Ed.; Elsevier: Amsterdam, The Netherlands, 2019; pp. 126–134. [ Google Scholar ] [ CrossRef ]
- Gautam, S.S.; Gondwal, M.; Soni, R.; Gautam, B.P.S. Potash biofertilizers: Current development, formulation, and applications. In Trends of Applied Microbiology for Sustainable Economy ; Academic Press: Cambridge, MA, USA, 2022; pp. 481–500. [ Google Scholar ] [ CrossRef ]
- Ding, Q.; Diao, W.; Gao, C.; Chen, X.; Liu, L. Microbial cell engineering to improve cellular synthetic capacity. Biotechnol. Adv. 2020 , 45 , 107649. [ Google Scholar ] [ CrossRef ] [ PubMed ]
- Sgobba, E.; Wendisch, V.F. Synthetic microbial consortia for small molecule production. Curr. Opin. Biotechnol. 2020 , 62 , 72–79. [ Google Scholar ] [ CrossRef ] [ PubMed ]
- Ben Said, S.; Or, D. Synthetic Microbial Ecology: Engineering Habitats for Modular Consortia. Front. Microbiol. 2017 , 8 , 1125. [ Google Scholar ] [ CrossRef ]
- Jagmann, N.; Philipp, B. Design of synthetic microbial communities for biotechnological production processes. J. Biotechnol. 2014 , 184 , 209–218. [ Google Scholar ] [ CrossRef ] [ PubMed ]
- Johns, N.I.; Blazejewski, T.; Gomes, A.L.; Wang, H.H. Principles for designing synthetic microbial communities. Curr. Opin. Microbiol. 2016 , 31 , 146–153. [ Google Scholar ] [ CrossRef ] [ PubMed ]
- Großkopf, T.; Soyer, O.S. Synthetic microbial communities. Curr. Opin. Microbiol. 2014 , 18 , 72–77. [ Google Scholar ] [ CrossRef ] [ PubMed ]
- Tan, X.; Letendre, J.H.; Collins, J.J.; Wong, W.W. Synthetic biology in the clinic: Engineering vaccines, diagnostics, and therapeutics. Cell 2021 , 184 , 881–898. [ Google Scholar ] [ CrossRef ]
- Cermak, N.; Datta, M.S.; Conwill, A. Rapid, Inexpensive Measurement of Synthetic Bacterial Community Composition by Sanger Sequencing of Amplicon Mixtures. iScience 2020 , 23 , 100915. [ Google Scholar ] [ CrossRef ]
- Konopka, A. Ecology, microbial. In Encyclopedia of Microbiology ; Academic Press: Cambridge, MA, USA, 2009; pp. 91–106. [ Google Scholar ] [ CrossRef ]
- Bernstein, H.C.; Carlson, R.P. Microbial Consortia Engineering for Cellular Factories: In vitro to in silico systems. Comput. Struct. Biotechnol. J. 2012 , 3 , e201210017. [ Google Scholar ] [ CrossRef ]
- Blasche, S.; Kim, Y.; Oliveira, A.P.; Patil, K.R. Model microbial communities for ecosystems biology. Curr. Opin. Syst. Biol. 2017 , 6 , 51–57. [ Google Scholar ] [ CrossRef ]
- Zhang, Y.; Yu, J.; Wu, Y.; Li, M.; Zhao, Y.; Zhu, H.; Chen, C.; Wang, M.; Chen, B.; Tan, T. Efficient production of chemicals from microorganism by metabolic engineering and synthetic biology. Chin. J. Chem. Eng. 2021 , 30 , 14–28. [ Google Scholar ] [ CrossRef ]
- Aguirre-Cárcer, D. Experimental and computational approaches to unravel microbial community assembly. Comput. Struct. Biotechnol. J. 2020 , 18 , 4071–4081. [ Google Scholar ] [ CrossRef ]
- Mccarty, N.S.; Ledesma-Amaro, R. Synthetic Biology Tools to Engineer Microbial Communities for Biotechnology. Trends Biotechnol. 2019 , 37 , 181–197. [ Google Scholar ] [ CrossRef ]
- Antoniewicz, M.R. A guide to deciphering microbial interactions and metabolic fluxes in microbiome communities. Curr. Opin. Biotechnol. 2020 , 64 , 230–237. [ Google Scholar ] [ CrossRef ]
- Ibáñez, J. La Inteligencia de las Plantas y el Ensamblaje de sus Rizosferas: Conversaciones Mutualistas Entre Raíces y Los Microorganismos del Suelo (Rizosferas) un Universo Invisible Bajo Nuestros Pies. Consejo Superior de Investigaciones Científicas (CSIC). Available online: http://www.madrimasd.org/blogs/universo/2018/04/27/149561 (accessed on 10 July 2024).
- Benigno, O.N.W. Evaluación de Consorcios Microbianos Diseñados Sobre el Desarrollo del Cultivo de Tomate (Solanum lycopersicum) Bajo Invernadero. 2020. Available online: http://dspace.ucuenca.edu.ec/bitstream/123456789/35313/1/Trabajo%20de%20titulacion.pdf (accessed on 26 February 2024).
- Eng, A.; Borenstein, E. Microbial community design: Methods, applications, and opportunities. Curr. Opin. Biotechnol. 2019 , 58 , 117–128. [ Google Scholar ] [ CrossRef ]
- Rojas-Badía, M. Quorum sensing in beneficial plant-bacteria associations. Rev. Colomb. Biotecnol. 2011 , 13 , 135–143. [ Google Scholar ]
- Honjo, H.; Iwasaki, K.; Soma, Y.; Tsuruno, K.; Hamada, H.; Hanai, T. Synthetic microbial consortium with specific roles designated by genetic circuits for cooperative chemical production. Metab. Eng. 2019 , 55 , 268–275. [ Google Scholar ] [ CrossRef ] [ PubMed ]
- Bengtsson-Palme, J. Microbial model communities: To understand complexity, harness the power of simplicity. Comput. Struct. Biotechnol. J. 2020 , 18 , 3987–4001. [ Google Scholar ] [ CrossRef ] [ PubMed ]
- Brenner, K.; Karig, D.K.; Weiss, R.; Arnold, F.H. Engineered bidirectional communication mediates a consensus in a microbial biofilm consortium. Proc. Natl. Acad. Sci. USA 2007 , 104 , 17300–17304. [ Google Scholar ] [ CrossRef ] [ PubMed ]
- Zhang, M.; Qiao, C.; Luan, G.; Luo, Q.; Lu, X. Systematic Identification of Target Genes for Cellular Morphology Engineering in Synechococcus elongatus PCC7942. Front. Microbiol. 2020 , 11 , 1608. [ Google Scholar ] [ CrossRef ]
- García-Jiménez, B.; Torres-Bacete, J.; Nogales, J. Metabolic modelling approaches for describing and engineering microbial communities. Comput. Struct. Biotechnol. J. 2021 , 19 , 226–246. [ Google Scholar ] [ CrossRef ] [ PubMed ]
- Gallardo-Navarro, O.; Aguilar-Salinas, B.; Rocha, J.; Olmedo-Álvarez, G. Higher-Order Interactions and Emergent Properties of Microbial Communities: The Power of Synthetic Ecology. Heliyon 2024 , 10 , E33896. [ Google Scholar ] [ CrossRef ]
- Bruno, J.F.; Stachowicz, J.J.; Bertness, M.D. Inclusion of facilitation into ecological theory. Trends Ecol. Evol. 2003 , 18 , 119–125. [ Google Scholar ] [ CrossRef ]
- Zhang, H.; Hu, J.; Peng, X.; Zhou, L.; Zhang, T.; Zhang, Y.; Yin, H.; Meng, D. Impacts of ammoniacal odour removal bioagent on air bacterial community. Adv. Biotechnol. 2024 , 2 , 8. [ Google Scholar ] [ CrossRef ]
- Cao, P.; Wei, X.; Wang, G.; Chen, X.; Han, J.; Li, Y. Microbial inoculants and garbage fermentation liquid reduced root-knot nematode disease and As uptake in Panax quinquefolium cultivation by modulating rhizosphere microbiota community. Chin. Herb. Med. 2022 , 14 , 58–69. [ Google Scholar ] [ CrossRef ]
- Faust, K.; Raes, J. Microbial interactions: From networks to models. Nat. Rev. Microbiol. 2012 , 10 , 538–550. [ Google Scholar ] [ CrossRef ]
- Wang, Y.; Zhang, M.; Dong, L.; Zhang, G.; Bai, X.; Wang, J.; Li, Y.; Hu, S.; Yu, X. Interactions between root and rhizosphere microbes mediate phosphorus acquisition in Pinus tabulaeformis . Ind. Crops Prod. 2024 , 215 , 118624. [ Google Scholar ] [ CrossRef ]
- Maqsood, Q.; Sumrin, A.; Waseem, R.; Hussain, M.; Imtiaz, M.; Hussain, N. Bioengineered microbial strains for detoxification of toxic environmental pollutants. Environ. Res. 2023 , 227 , 115665. [ Google Scholar ] [ CrossRef ] [ PubMed ]
- Requena, T.; Velasco, M. Microbioma humano en la salud y la enfermedad. Rev. Clín. Esp. 2019 , 221 , 233–240. [ Google Scholar ] [ CrossRef ]
- De Lorenzo, V.; Krasnogor, N.; Schmidt, M. For the sake of the Bioeconomy: Define what a Synthetic Biology Chassis is! New Biotechnol. 2021 , 60 , 44–51. [ Google Scholar ] [ CrossRef ]
- Bittihn, P.; Din, M.O.; Tsimring, L.S.; Hasty, J. Rational engineering of synthetic microbial systems: From single cells to consortia. Curr. Opin. Microbiol. 2018 , 45 , 92–99. [ Google Scholar ] [ CrossRef ] [ PubMed ]
- Kapoore, R.V.; Padmaperuma, G.; Maneein, S.; Vaidyanathan, S. Co-culturing microbial consortia: Approaches for applications in biomanufacturing and bioprocessing. Crit. Rev. Biotechnol. 2021 , 42 , 46–72. [ Google Scholar ] [ CrossRef ] [ PubMed ]
- Troncoso, C.; Pavez, M.; Santos, A.; Salazar, R.; Barrientos, L. Structural and physiological implications of bacterial cell in antibiotic resistance mechanisms. Int. J. Morphol. 2017 , 35 , 1214–1223. [ Google Scholar ] [ CrossRef ]
- Foresto, E.; Bogino, P.C. Quorum sensing: Un lenguaje común entre bacterias y plantas con importancia en la producción agrícola. Rev. Bol. Biol. 2020 , 22 , 10–55. [ Google Scholar ]
- Malla, M.A.; Ansari, F.A.; Bux, F.; Kumari, S. Re-vitalizing wastewater: Nutrient recovery and carbon capture through microbe-algae synergy using omics-biology. Environ. Res. 2024 , 259 , 119439. [ Google Scholar ] [ CrossRef ] [ PubMed ]
- Trilla Fuertes, L. Biblos-e Archivo. Modelización Computacional de las Alteraciones Metabólicas en Cáncer de Mama. 2019. Available online: https://repositorio.uam.es/handle/10486/687664 (accessed on 30 May 2024).
- Porter, J.R.; Batchelor, E. Using Computational Modeling and Experimental Synthetic Perturbations to Probe Biological Circuits. In Methods in Molecular Biology ; Humana Press: New York, NY, USA, 2014; pp. 259–276. [ Google Scholar ] [ CrossRef ]
- Zomorrodi, A.R.; Segrè, D. Synthetic ecology of microbes: Mathematical models and applications. J. Mol. Biol. 2016 , 428 , 837–861. [ Google Scholar ] [ CrossRef ] [ PubMed ]
- Hsu, R.H.; Clark, R.L.; Tan, J.W.; Ahn, J.C.; Gupta, S.; Romero, P.A.; Venturelli, O.S. Microbial Interaction Network Inference in Microfluidic Droplets. Cell Syst. 2019 , 9 , 229–242.e4. [ Google Scholar ] [ CrossRef ] [ PubMed ]
- Glass, D.S.; Riedel-Kruse, I.H. A Synthetic Bacterial Cell-Cell Adhesion Toolbox for Programming Multicellular Morphologies and Patterns. Cell 2018 , 174 , 649–658.e16. [ Google Scholar ] [ CrossRef ] [ PubMed ]
- Jin, X.; Riedel-Kruse, I.H. Biofilm lithography enables high-resolution cell patterning via optogenetic adhesin expression. Proc. Natl. Acad. Sci. USA 2018 , 115 , 3698–3703. [ Google Scholar ] [ CrossRef ]
- Gardner, T.S.; Cantor, C.R.; Collins, J.J. Construction of a genetic toggle switch in Escherichia coli . Nature 2000 , 403 , 339–342. [ Google Scholar ] [ CrossRef ]
- Elowitz, M.B.; Leibler, S. A synthetic oscillatory network of transcriptional regulators. Nature 2000 , 403 , 335–338. [ Google Scholar ] [ CrossRef ] [ PubMed ]
- Miller, M.B.; Bassler, B.L. Quorum sensing in bacteria. Annu. Rev. Microbiol. 2001 , 55 , 165–199. [ Google Scholar ] [ CrossRef ] [ PubMed ]
- He, Y.; Yun, H.; Peng, L.; Ji, J.; Wang, W.; Li, X. Deciphering the potential role of quorum quenching in efficient aerobic denitrification driven by a synthetic microbial community. Water Res. 2024 , 251 , 121162. [ Google Scholar ] [ CrossRef ] [ PubMed ]
- Han, Y.H.; Kim, G.; Seo, S.W. Programable synthetic biology tools for developing microbial cell factories. Curr. Opin. Biotechnol. 2023 , 79 , 102874. [ Google Scholar ] [ CrossRef ]
- Selberg, J.; Gomez, M.; Rolandi, M. The potential for convergence between synthetic biology and bioelectronics. Cell Syst. 2018 , 7 , 231–244. [ Google Scholar ] [ CrossRef ] [ PubMed ]
- Didovyk, A.; Borek, B.; Hasty, J.; Tsimring, L. Orthogonal Modular Gene Repression in Escherichia coli Using Engineered CRISPR/Cas9. ACS Synth. Biol. 2016 , 5 , 81–88. [ Google Scholar ] [ CrossRef ] [ PubMed ]
- Barreto, A.C. Quorum Sensing: Sistemas de comunicación bacteriana. Cienc. Actual 2011 , 2 , 43–50. [ Google Scholar ]
- Zhang, L.; Zou, W.; Ni, M.; Hu, Q.; Zhao, L.; Liao, X.; Huang, Q.; Zhou, R. Development and application of two inducible expression systems for Streptococcus suis . Microbiol. Spectrum. 2022 , 10 , e0036322. [ Google Scholar ] [ CrossRef ]
- Charubin, K.; Bennett, R.K.; Fast, A.G.; Papoutsakis, E.T. Engineering Clostridium organisms as microbial cell-factories: Challenges & opportunities. Metab. Eng. 2018 , 50 , 173–191. [ Google Scholar ] [ CrossRef ]
- Rivas Lagos, M. Repositorio Académico—Universidad de Chile. Detector de CRISPR en Genoma de Bacterias. 2020. Available online: https://repositorio.uchile.cl/handle/2250/176846 (accessed on 30 May 2024).
- Jeong, S.H.; Lee, H.J.; Lee, S.J. Recent advances in CRISPR-Cas technologies for synthetic biology. J. Microbiol. 2023 , 61 , 13–36. [ Google Scholar ] [ CrossRef ]
- Hutinet, G.; Kot, W.; Cui, L.; Hillebrand, R.; Balamkundu, S.; Gnanakalai, S.; Neelakandan, R.; Carstens, A.B.; Lui, C.F.; Tremblay, D.; et al. 7-Deazaguanine modifications protect phage DNA from host restriction systems. Nat. Commun. 2019 , 10 , 5442. [ Google Scholar ] [ CrossRef ]
- Mojica, F.J.M.; Juez, G.; Rodriguez-Valera, F. Transcription at different salinities of Haloferax mediterranei sequences adjacent to partially modified PstI sites. Mol. Microbiol. 1993 , 9 , 613–621. [ Google Scholar ] [ CrossRef ] [ PubMed ]
- Lammoglia-Cobo, M.F.; Lozano-Reyes, R.; García-Sandoval, C.D.; Avilez-Bahena, C.M.; Trejo-Reveles, V.; Muñoz-Soto, R.B.; López-Camacho, C. La revolución en ingeniería genética: Sistema CRISPR/Cas. Investig. Discapacidad. 2016 , 5 , 116–128, ISSN 2992-779X. [ Google Scholar ]
- Doudna, J.A.; Charpentier, E. The new frontier of genome engineering with CRISPR-Cas9. Science 2014 , 346 , 1258096. [ Google Scholar ] [ CrossRef ]
- Rubin, B.E.; Diamond, S.; Cress, B.F.; Crits-Christoph, A.; Lou, Y.C.; Borges, A.L.; Shivram, H.; He, C.; Xu, M.; Zhou, Z.; et al. Species- and site-specific genome editing in complex bacterial communities. Nat. Microbiol. 2021 , 7 , 34–47. [ Google Scholar ] [ CrossRef ] [ PubMed ]
- Stephens, K.; Bentley, W.E. Synthetic Biology for Manipulating Quorum Sensing in Microbial Consortia. Trends Microbiol. 2020 , 28 , 633–643. [ Google Scholar ] [ CrossRef ]
- Nealson, K.H.; Hastings, J.W. Bacterial bioluminescence: Its control and ecological significance. Microbiol. Rev. 1979 , 43 , 496–518. [ Google Scholar ] [ CrossRef ] [ PubMed ]
- Sarkar, K.; Sil, P.C. Effect of diet, pharmaceuticals, and environmental toxicants on gut microbiota imbalance and increased intestinal membrane permeability. In Toxicological Risk Assessment and Multi-System Health Impacts from Exposure ; Tsatsakis, A.M., Ed.; Academic Press: Cambridge, MA, USA, 2021; pp. 403–413. [ Google Scholar ] [ CrossRef ]
- Thursby, E.; Juge, N. Introduction to the human gut microbiota. Biochem. J. 2017 , 474 , 1823–1836. [ Google Scholar ] [ CrossRef ]
- Afzaal, M.; Saeed, F.; Shah, Y.A.; Hussain, M.; Rabail, R.; Socol, C.T.; Hassoun, A.; Pateiro, M.; Lorenzo, J.M.; Rusu, A.V.; et al. Human gut microbiota in health and disease: Unveiling the relationship. Front. Microbiol. 2022 , 26 , 999001. [ Google Scholar ] [ CrossRef ]
- Zhang, J.S.; Chu, C.H.; Yu, O.Y. Oral Microbiome and Dental Caries Development. Dent. J. 2022 , 10 , 184. [ Google Scholar ] [ CrossRef ]
- Van Leeuwen, P.T.; Brul, S.; Zhang, J.; Wortel, M.T. Synthetic microbial communities (SynComs) of the human gut: Design, assembly, and applications. FEMS Microbiol. Rev. 2023 , 47 , fuad012. [ Google Scholar ] [ CrossRef ] [ PubMed ]
- Donkers, J.M.; van der Vaart, J.I.; van de Steeg, E. Gut-on-a-chip research for drug development: Implications of chip design on preclinical oral bioavailability or intestinal disease studies. Biomimetics 2023 , 8 , 226. [ Google Scholar ] [ CrossRef ] [ PubMed ]
- Liu, J.; Zhu, W.; Lessing, D.J.; Chu, W. Synthetic microbial consortia for the treatment of Clostridioides difficile infection in mice model. Microb. Biotechnol. 2023 , 16 , 1985–2006. [ Google Scholar ] [ CrossRef ] [ PubMed ]
- Gurbatri, C.R.; Lia, I.; Vincent, R.; Coker, C.; Castro, S.; Treuting, P.M.; Hinchliffe, T.E.; Arpaia, N.; Danino, T. Engineered probiotics for local tumor delivery of checkpoint blockade nanobodies. Sci. Transl. Med. 2020 , 12 , eaax0876. [ Google Scholar ] [ CrossRef ] [ PubMed ]
- Anderson, J.C.; Clarke, E.J.; Arkin, A.P.; Voigt, C.A. Environmentally controlled invasion of cancer cells by engineered bacteria. J. Mol. Biol. 2006 , 355 , 619–627. [ Google Scholar ] [ CrossRef ] [ PubMed ]
- Patel, V.K.; Sarim, K.M.; Patel, A.K.; Rout, P.K.; Kalra, A. Synthetic microbial ecology and nanotechnology for the production of Taxol and its precursors: A step towards sustainable production of cancer therapeutics. In Design of Nanostructures for Theranostics Applications ; Grumezescu, A.M., Ed.; William Andrew Publishing: Norwich, NY, USA, 2018; pp. 563–587. [ Google Scholar ] [ CrossRef ]
- Chen, D.S.; Irving, B.A.; Hodi, F.S. Molecular pathways: Next generation immunotherapy—Inhibiting programmed death ligand 1 and programmed death-1. Clin. Cancer Res. 2012 , 18 , 6580–6587. [ Google Scholar ] [ CrossRef ] [ PubMed ]
- Buchbinde, A.; Desai, A. CTLA-4 y PD-1 Pathways. Am. J. Clin. Oncol. 2016 , 39 , 98–106. [ Google Scholar ] [ CrossRef ]
- Hu, Y.; Liu, C.; Muyldermans, S. Nanobody-Based Delivery Systems for Diagnosis and Targeted Tumor Therapy. Front. Immunol. 2017 , 8 , 1442. [ Google Scholar ] [ CrossRef ] [ PubMed ]
- Rylott, E.L.; Bruce, N.C. How synthetic biology can help bioremediation. Curr. Opin. Chem. Biol. 2020 , 58 , 86–95. [ Google Scholar ] [ CrossRef ]
- Fuentes, S.; Méndez, V.; Aguila, P.; Seeger, M. Bioremediation of petroleum hydrocarbons: Catabolic genes, microbial communities, and applications. Appl. Microbiol. Biotechnol. 2014 , 98 , 4781–4794. [ Google Scholar ] [ CrossRef ]
- Schwab, S.; de Souza Pires, A.; Candido, G.Z.; Saggin Júnior, O.J.; Reis, V.M.; Cruz, L.M. Analysis of the endophytic microbiota of roots and culms of two commercial sugarcane cultivars inoculated with a synthetic microbial community. Appl. Soil. Ecol. A Sect. Agric. Ecosyst. Environ. 2024 , 195 , 105235. [ Google Scholar ] [ CrossRef ]
- Chen, J.; Cai, Y.; Wang, Z.; Xu, Z.; Li, J.; Ma, X.; Zhuang, W.; Liu, D.; Wang, S.; Song, A.; et al. Construction of a synthetic microbial community based on multiomics linkage technology and analysis of the mechanism of lignocellulose degradation. Bioresour. Technol. 2023 , 389 , 129799. [ Google Scholar ] [ CrossRef ] [ PubMed ]
- Revelo-Romo, D.M.; Hurtado Gutiérrez, N.H.; Ruiz Pazos, J.O.; Pabón Figueroa, L.V.; Ordóñez Ordóñez, L.A. Bacterial diversity in the Cr(VI) reducing biocathode of a Microbial Fuel Cell with salt bridge. Rev. Argent. Microbiol. 2019 , 51 , 110–118. [ Google Scholar ] [ CrossRef ] [ PubMed ]
- Verma, N.; Sharma, R. Bioremediation of Toxic Heavy Metals: A Patent Review. Recent. Pat. Biotechnol. 2017 , 11 , 171–187. [ Google Scholar ] [ CrossRef ]
- Tripathi, S.; Arora, N.; Gupta, P.; Pruthi, P.A.; Poluri, K.M.; Pruthi, V. Microalgae. In Advanced Biofuels ; Woodhead Publishing: Sawston, UK, 2019; pp. 97–128. [ Google Scholar ] [ CrossRef ]
- Chakravorty, M.; Nanda, M.; Bisht, B.; Sharma, R.K.; Kumar, S.; Mishra, A.; Vlaskin, M.S.; Chauhan, P.K.; Kumar, V. Heavy metal tolerance in microalgae: Detoxification mechanisms and applications. Aquat. Toxicol. 2023 , 260 , 106555. [ Google Scholar ] [ CrossRef ] [ PubMed ]
- Ardila, L. Medición de la Capacidad de Chlorella Vulgaris y Scenedesmus Acutus para la Remoción de Cromo de Aguas de Curtiembre. Universidad Nacional de Colombia Sede Bogotá Facultad de Ingeniería Departamento de Ingeniería Química y Ambiental. 2012. Available online: https://repositorio.unal.edu.co/handle/unal/20027 (accessed on 26 February 2024).
- Vacca-Jimeno, V.A.; Angulo-Mercado, E.R.; Puentes Ballesteros, D.M.; Torres-Yépez, J.G.; Plaza-Vega, M.E. Using the microalgae Chlorella sp. live suspended in decoloration wastewater from a textile factory. Prospects 2017 , 15 , 93–99. [ Google Scholar ] [ CrossRef ]
- Marrero-Coto, J.; Díaz-Valdivia, A.; Coto-Pérez, O. Mecanismos moleculares de resistencia a metales pesados en las bacterias y sus aplicaciones en la biorremediación. RCCB 2009 , 41 , 67–78. Available online: https://www.redalyc.org/articulo.oa?id=181221644010 (accessed on 12 February 2024).
- Quiroga, M.V.; Stegen, J.C.; Mataloni, G.; Cowan, D.; Lebre, P.H.; Valverde, A. Microdiverse bacterial clades prevail across Antarctic wetlands. Mol. Ecol. 2024 , 33 , e17189. [ Google Scholar ] [ CrossRef ]
- Abele, D.; Vazquez, S.; Buma, A.; Hernandez, E.; Quiroga, C.; Held, C.; Frickenhaus, S.; Harms, L.; Lopez, J.L.; Helmke, E.; et al. Pelagic and benthic communities of the Antarctic ecosystem of Potter Cove: Genomics and ecological implications. Mar. Genom. 2017 , 33 , 1–11. [ Google Scholar ] [ CrossRef ]
- Roell, M.S.; Zurbriggen, M.D. The impact of synthetic biology for future agriculture and nutrition. Curr. Opin. Biotechnol. 2020 , 61 , 102–109. [ Google Scholar ] [ CrossRef ] [ PubMed ]
- Valenzuela-Ruiz, V.; Ayala-Zepeda, M.; Arellano-Wattenbarger, G.L.; Parra-Cota, F.I.; García-Pereyra, G.; Aviña-Martínez, G.N.; de los Santos-Villalobos, S. Las colecciones microbianas y su potencial contribución a la seguridad alimentaria actual y futura. Rev. Latinoam. Rec. Nat. 2018 , 14 , 18–25, ISSN 2594-0384. [ Google Scholar ]
- Mardanov, A.V.; Kotlyarov, R.V.; Beletsky, A.V.; Pimenov, N.V.; Nikolaev, Y.A.; Grachev, V.A.; Berestovskaya, Y.Y.; Ravin, N.V. Metagenomic data of the microbial community of lab-scale nitritation-anammox sequencing-batch bioreactor performing nitrogen removal from synthetic wastewater. Data Brief 2019 , 27 , 104722. [ Google Scholar ] [ CrossRef ] [ PubMed ]
- Schnell, S.; Bak, F.; Pfennig, N. Anaerobic degradation of aniline and dihydroxybenzenes by newly isolated sulfate-reducing bacteria and description of Desulfobacterium anilini . Arch. Microbiol. 1989 , 152 , 556–563. [ Google Scholar ] [ CrossRef ] [ PubMed ]
- Xie, X.; Müller, N. Enhanced aniline degradation by Desulfatiglans anilini in a synthetic microbial community with the phototrophic purple sulfur bacterium Thiocapsa roseopersicina . Syst. Appl. Microbiol. 2019 , 42 , 125998. [ Google Scholar ] [ CrossRef ] [ PubMed ]
- Cui, J.; Huang, L.; Wang, W.; Xu, P.; Zanaroli, G.; Tang, H. Maximization of the petroleum biodegradation using a synthetic bacterial consortium based on minimal value algorithm. Int. Biodeterior. Biodegrad. 2020 , 150 , 104964. [ Google Scholar ] [ CrossRef ]
- Silva, R.M.P.; Pozo, M.I.C.; de Oca, J.M.M.; Rodríguez, A.A.; Viñas, M.; Moreno, D.C. Aislamiento y selección de una cepa bacteriana degradadora de hidrocarburos a partir de suelos contaminados con petróleo. Rev. CENIC Cienc. Biol. 2008 , 39 , 44–51. Available online: https://www.redalyc.org/articulo.oa?id=181214889004 (accessed on 18 November 2023).
- Chen, W.; Li, J.; Sun, X.; Min, J.; Hu, X. High efficiency degradation of alkanes and crude oil by a salt-tolerant bacterium Dietzia species CN-3. Int. Biodeterior. Biodegrad. 2017 , 118 , 110–118. [ Google Scholar ] [ CrossRef ]
- Reyes-Reyes, M.A.; Puentes-Cala, E.A.; Casanova-Montes, E.L.; López-Deluque, F.; Panqueva-Álvarez, J.H.; Castillo-Villamizar, G.A. Immobilization of potentially crude oil degrading bacteria in synthetic and natural organic matrices. Rev. Int. Contam. Ambient. 2018 , 34 , 597–609. [ Google Scholar ] [ CrossRef ]
- Du, R.; Jiang, J.; Qu, G.; Wu, Q.; Xu, Y. Directionally controlling flavor compound profile based on the structure of synthetic microbial community in Chinese liquor fermentation. Food Microbiol. 2023 , 114 , 104305. [ Google Scholar ] [ CrossRef ]
- Jiang, X.; Peng, Z.; Zhang, J. Starting with screening strains to construct synthetic microbial communities (SynComs) for traditional food fermentation. Int. Food Res. 2024 , 190 , 114557. [ Google Scholar ] [ CrossRef ]
- Xin, W.; Zhang, J.; Yu, Y.; Tian, Y.; Li, H.; Chen, X.; Li, W.; Liu, Y.; Lu, T.; He, B.; et al. Root microbiota of tea plants regulate nitrogen homeostasis and theanine synthesis to influence tea quality. Curr. Biol. 2024 , 34 , 868–880.e6. [ Google Scholar ] [ CrossRef ] [ PubMed ]
- Yuan, J.; Zhao, K.; Tan, X.; Xue, R.; Zeng, Y.; Ratti, C.; Trivedi, P. Perspective on the development of synthetic microbial community (SynCom) biosensors. Trends Biotechnol. 2023 , 41 , 1227–1236. [ Google Scholar ] [ CrossRef ] [ PubMed ]
- Yu, W.; Xu, X.; Jin, K.; Liu, Y.; Li, J.; Du, G.; Lv, X.; Liu, L. Genetically encoded biosensors for microbial synthetic biology: From conceptual frameworks to practical applications. Biotechnol. Adv. 2023 , 62 , 108077. [ Google Scholar ] [ CrossRef ] [ PubMed ]
- Li, X.; Zheng, X.; Yadav, N.; Saha, S.; Salama, E.-S.; Li, X.; Wang, L.; Jeon, B.-H. Rational management of the plant microbiome for the Second Green Revolution. Plant Commun. 2024 , 5 , 100812. [ Google Scholar ] [ CrossRef ]
- Hao, X.; Wang, X.; Chen, C.; Liu, R.; Yin, Y.; Yao, J.; Xiao, Z.; Liu, X.; Shen, X.; Liu, X. Synthetic bacterial communities reshape microbial communities and enhance nutrient supply in desertified land of Northwest China. Appl. Soil. Ecol. A Sect. Agric. Ecosyst. Environ. 2023 , 189 , 104972. [ Google Scholar ] [ CrossRef ]
- Hays, S.G.; Yan, L.L.; Silver, P.A.; Ducat, D.C. Synthetic photosynthetic consortia define interactions leading to robustness and photoproduction. J. Biol. Eng. 2017 , 11 , 4. [ Google Scholar ] [ CrossRef ]
- Ding, M.-Z.; Song, H.; Wang, E.-X.; Liu, Y.; Yuan, Y.-J. Design and construction of synthetic microbial consortia in China. Synth. Syst. Biotechnol. 2016 , 1 , 230–235. [ Google Scholar ] [ CrossRef ]
- Trump, B.D.; Foran, C.; Rycroft, T.; Wood, M.D.; Bandolin, N.; Cains, M.; Hamilton, K. Development of community of practice to support quantitative risk assessment for synthetic biology products: Contaminant bioremediation and invasive carp control as cases. Environ. Syst. Decis. 2018 , 38 , 517–527. [ Google Scholar ] [ CrossRef ]
- Sivasubramaniam, D.; Franks, A.E. Bioengineering microbial communities: Their potential to help, hinder and disgust. Bioengineered 2016 , 7 , 137–144. [ Google Scholar ] [ CrossRef ]
Click here to enlarge figure
The statements, opinions and data contained in all publications are solely those of the individual author(s) and contributor(s) and not of MDPI and/or the editor(s). MDPI and/or the editor(s) disclaim responsibility for any injury to people or property resulting from any ideas, methods, instructions or products referred to in the content. |
Share and Cite
Contreras-Salgado, E.A.; Sánchez-Morán, A.G.; Rodríguez-Preciado, S.Y.; Sifuentes-Franco, S.; Rodríguez-Rodríguez, R.; Macías-Barragán, J.; Díaz-Zaragoza, M. Multifaceted Applications of Synthetic Microbial Communities: Advances in Biomedicine, Bioremediation, and Industry. Microbiol. Res. 2024 , 15 , 1709-1727. https://doi.org/10.3390/microbiolres15030113
Contreras-Salgado EA, Sánchez-Morán AG, Rodríguez-Preciado SY, Sifuentes-Franco S, Rodríguez-Rodríguez R, Macías-Barragán J, Díaz-Zaragoza M. Multifaceted Applications of Synthetic Microbial Communities: Advances in Biomedicine, Bioremediation, and Industry. Microbiology Research . 2024; 15(3):1709-1727. https://doi.org/10.3390/microbiolres15030113
Contreras-Salgado, Edgar Adrian, Ana Georgina Sánchez-Morán, Sergio Yair Rodríguez-Preciado, Sonia Sifuentes-Franco, Rogelio Rodríguez-Rodríguez, José Macías-Barragán, and Mariana Díaz-Zaragoza. 2024. "Multifaceted Applications of Synthetic Microbial Communities: Advances in Biomedicine, Bioremediation, and Industry" Microbiology Research 15, no. 3: 1709-1727. https://doi.org/10.3390/microbiolres15030113
Article Metrics
Article access statistics, further information, mdpi initiatives, follow mdpi.
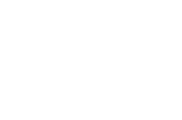
Subscribe to receive issue release notifications and newsletters from MDPI journals
St anford | Synthetic Biology
Enabling flourishing futures in partnership with life.
What is synthetic biology?
Synthetic Biology (SB) explores new forms of engagement with life and living systems. From molecular to ecological, cultural to political, SB is about understanding life's fundamental mysteries and translating knowledge to imagine a biotic civilization that flourishes in partnership with Earth.
The Stanford Synthetic Biology community enables interdisciplinary activities, supporting an ecosystem of research and learning. Our holistic approach encompasses diverse areas of work, each exploring fundamental questions and possibilities with the ultimate desire of addressing societal needs.
All together now!
Stewart Brand, Stanford Class of 19 60 (Biology), wrote,
"I propose six significant levels of pace and size in the working structure of a robust and adaptable civilization. [...] In a healthy society each level is allowed to operate at its own pace, safely sustained by the slower levels below and kept invigorated by the livelier levels above." ( Long Now )
With pace-layer thinking in mind we are exploring and advancing synthetic biology (SB) among and across all aspects of civilization, inclusive of but not limited to biotechnology and bioeconomy. We also note that intrinsic to pace layers thinking is a bias towards the human, a concept we suspect may evolve along with SB. Thus, we have adopted a coupled-rings visual metaphor, in place of layers, for now.
Stanford SB News
Read how Professors Rogelio Hernandez-Lopez, Hawa Racine Thiam, Drew Endy, Michael Jewett, and Kyle Daniels are engineering cells for new purposes
August 2024
Read in Stanford Med Magazine
A frugal CRISPR kit, a gene editing tool for K-12 education. T his kit brings hands-on CRISPR experiments to classrooms to explore genome engineering without equipment.
Read in Nature
On Global Health: Smallpox Biosecurity in a New Era of Technology
Read the article
Curious about CRISPR? Stanley Qi explains CRISPR, gene editing, and beyond
Read in Stanford Report
Stanford bioengineers host Secretary of State Anthony Blinken for a roundtable discussion on synthetic biology.
Read the Press Release
Professor Sang Yup Lee of KAIST joins us for the inaugural Stanford Frontiers in Synthetic Biology Distinguished Lecture to discuss metabolic engineering for sustainability and health
View Event Details
Chris Voigt joins the Synthesis community to discuss tracking engineered microbiomes from space and over decades
Hosted by Stanford BioE Department Colloquium
Dr. Christina Smolke joined the Sustainability Accelerator for an enlightening discussion on the profound impact of synthetic biology on pharmaceutical biomanufacturing and global sustainability.
April 2024
Watch the event recording
Jay Daniels, Founding Scientist at Moonlight Bio, shares insights on his journey from academia to industry at SB.Talk.
The Wu Tsai Neurosciences Institute , Sarafan Chem-H , and Bio-X seek proposals for the inaugural Synthetic Neuroscience Grant Program to spark new collaborations between neuroscience and synthetic biology researchers across Stanford University.
February 2024
Learn more and apply
Stanford Emerging Technology Review launch at the Hoover office in Washington, DC.
January 202 4
The second Synthetic Neuroscience Forum was held with talks from Michael Lin and Xiaojing Gao. Hosted by Sarafan ChEM-H, Bio-X, Wu Tsai Neurosciences Institute.
January 2024
Event Information
Building Biology student symposium brought in guest speakers: Patrick Hsu (UCB), Katie Galloway (MIT), Magdalena Zernika-Goetz (Caltech), Neil Shubin (UChicago)
Hosted by Stanford Genetics and Developmental Biology Training Program
January 2024
The Hoover Institution and Stanford School of Engineering have launched the inaugural edition of the Stanford Emerging Technology Review, which aims to help policymakers understand a range of fast-developing new technologies, from AI and cryptography to robotics and synthetic biology.
December 2023
Read the Report
The first Synthetic Neuroscience Forum was held with Drew Endy and Sergiu Pasca, leading a discussion on the opportunities of synthetic biology in neuroscience. Hosted by Sarafan ChEM-H, Bio-X, Wu Tsai Neurosciences Institute.
December 2023
Stanford Bioengineering Department launches "Engineering Life for Planet Health" f aculty search and seeking candidates who align with the department's vision of rapidly advancing large-scale solutions to address critical planetary challenges.
November 2023
Qi Lab develops an ultrasound method for precise control in gene regulation and base editing - both in cells and living animals.
October 2023
Congratulations to Xiaojing Gao, Steven Banik, and Stanley Qi for being awarded NIH High Risk, High Reward program grants for their work in synthetic biology.
Mike Jewett kicks off the new school year by facilitating a community discussion at SB.Talk on synthetic biology for sustainability.
September 2023
Upcoming Events
Stanford 2023 iGEM team designed and developed Phil's Laberia, an educational video game where students can learn bioengineering wet lab skills.
August 2023
Play the Game
Fischbach Lab provides new insights into how T cells respond to commensal microbes.
Qi Lab develops a CRISPR/Cas9 approach to edit dendritic cells for wound healing
Read in Nature Comms
Bintu Lab works on high-throughput functional characterization of combinations of transcriptional activators and repressors
Read in Cell Systems
Endy Lab publishes a paper on engineering tRNA abundances for synthetic cellular systems
Learn from Jenn Brophy and Drew Endy 's EdEquityLab x Stanford 's Intro to Bioengineering course. Now available online!
Watch the lectures
SB.Talk sparks discussion around synthetic biology at Stanford. Join the conversation in the Shriram Tea Room every other Tuesday 12pm.
Jewett Lab developed a cell-free method to rapidly discover and characterize functional antibodies in under 24 hours.
Qi Lab reports on 'CLIP' (CRISPR for long-fragment integration via pseudovirus) method for stable expression of large transgenes via the knock-in of an integrate-deficient lentivirus.
Prof. Alice Ting has been elected to the National Academy of Sciences. Ting is a Professor of Genetics, of Biology and, by courtesy, of Chemistry.
Read in NAS News
The inaugural Synthetic Biology for Sustainability Symposium had over 200 attendees representing across schools of Medicine, Engineering, Sustainability, and H&S.
Event Website
Read highlights in Stanford News
Synthetic Biology for Sustainability Symposium will take place on May 1.
Hosted by the Deans of the School of Medicine, the School of Engineering, and the Stanford Doerr School of Sustainability, this symposium will focus on how we might tackle some of the biggest challenges in sustainability using some of the newest innovations in synthetic biology.
Agenda and Speaker Information
Fischbach Lab engineered a common skin bacterium, S. epidermidis to produce a tumor antigen . When applied to mice, it resulted in a potent immune response against a distant tumor.
Read the Science article
Read in Stanford News
Bintu Lab leads efforts towards large-scale mapping and mutagenesis of human transcriptional effector domains
Read the Nature article
Stanford Synthetic Biology meets for the first community event of 2023
View our community portrait
Sattely Lab discovered 22 enzymes for biosynthesis of limonoids in Citrus and Melia.
January 2023
Prof. Mike Jewett joins Stanford Bioengineering as their newest faculty member.
Read the Stanford Article
Join the Jewett lab!
Gao Lab develops new tool -- programmable RNA sensing using ADAR editing in living cells.
October 2022
Read in Nature Biotechnology
Read the story!
Biden Administration issued an Executive Order on Advancing Biotechnology and Biomanufacturing Innovation for a Sustainable, Safe, and Secure American Bioeconomy.
September 2022
Learn more from Endy and Palmer
White House Executive Order
Does synthetic biology offer anything new? Watch the non-technical conversation with Drew Endy about advancements in synthetic biology and related economic and governance opportunities with t he Hoover Institution.
August 2022
Watch the video!
Sergiu Pasca explains how to reverse engineer the human brain by growing organoids.
August 2022
Learn from the TED talk!
Dr. Alice Cheng of Fischbach Lab & colleagues make human microbiome from scatch.
Read NY Times article
Study the science!
The Bintu lab takes a synthetic approach to understand and program chromatin-mediated gene silencing & activation.
Brophy et al. pioneer synthetic genetic circuits in plant roots.
Read the news!
Townshend, Kaplan, & Smolke report over 200 new RNA biosensors to potential drug molecules.
Read the preprint!
Endy & McKelvey reflect on synthetic biology's potential impacts for democracy and national security, hosted by Los Alamos National Lab. and moderated in part by Stanford's Prof. Hank Greely.
Synthetic Biology for Democracy
National Security Implications
Preparing for and preventing future pandemics. Two new grants provide key resources
Seed grants available Fall '22
Antiviral drug discovery funded!
"What is synthetic biology and what is its potential? These stories explain." Megan Palmer's WEF Council partners with Faber Futures to explore and elaborate on inclusive futures.
Download the stories!
"Building a Bottom-Up Bioeconomy," Stanford's Dr. Megan Palmer and colleagues make the case by reimagining industrialization .
Read the article!
Making With Mushrooms! 17 years later the second-ever issue of Adventures in Synthetic Biology begins to emerge.
Mycological mysteries await!
"Mother Nature, Bioweapons, & Lab Accidents: Guarding Against the Next Global Biological Catastrophe," Stanford's Freeman Spogli's CISAC welcomes Dr. Jaime Yassif of NTI. March 2022
View the presentation!
Prof. Pasca explores how to understand the mysteries of the human mind by growing neural circuits from scratch .
Train your neurons!
Prof. Skylar-Scott & team explain what must be made real to ultimately print working hearts from scratch.
"Who wouldn't want to ride on a Wooly Mammoth?," Stanford undergraduates explore dystopian and utopian futures that might arise via resurrecting extinct species. March 2022
Extinction Reversal Dystopia!
De-Extinction Utopia!
Prof. Ting's team pioneers LuCID, a genetically-encoded tool for realtime measurement of calcium dynamics in live cells, including neurons and immune cells.
Read the paper!
Prof. Steinmetz and colleagues pioneer synthetic genomics for understanding the fundamental rules of genome architecture.
Study the Science paper!
Read the news story!
The New Yorker explores if what biology needs right now is more synthesis. Prof. Zia chimes in.
February 2022
Prof. Brophy & Dinneny pioneer living Boolean logic in plants for climate resilience.
Study the preprint
Gao lab pioneers the engineering of protein circuits that let cells talk to each other.
Novozymes recognizes Adjunct Prof. Smolke & team with 100,000 DKK prize for sustainable medicines.
See the announcement!
How can American strengthen its bioeconomy? Adjunct Prof. Palmer and colleagues chart the path.
Read the plan!
Can we engineer crops to withstand climate change? Prof. Brophy makes the case.
January 2022
See the news!
NY Times explores what's happening and might be possible via synthetic biology. E.g., it's personal!
November 2021
Megan Palmer, co-chair of World Economic Forum Council on Synthetic Biology, and team release report on synthetic biology.
Read the report!
Danielle Mai's group discusses the role of synthetic biology in a class of protein-based materials.
September 202 1
Stanley Qi's team develops CRISPR-Cas13 resource for targeting RNA viruse s.
Prashanth in the Smolke lab pioneers brewing of tropane alkaloids from scratch.
September 2020
Read the coverage!
- Search Menu
- Sign in through your institution
- Volume 9, Issue 1, 2024 (In Progress)
- Volume 8, Issue 1, 2023
- Advance articles
- Special issues
- Research articles
- Review articles
- Synthetic biology news
- Author Guidelines
- Submission Site
- Open Access
- About Synthetic Biology
- Editorial Board
- Self Archiving policy
- Advertising and Corporate Services
- Journals on Oxford Academic
- Books on Oxford Academic

Article Contents
1. introduction, 2. course fundamentals, 3. course outline, 4. course formulation, 5. impact and metrics, 6. conclusions, supplementary data, acknowledgements.
- < Previous
Principles of synthetic biology: a MOOC for an emerging field

Authors Daniel A Anderson and Ross D Jones contributed equally to this work.
- Article contents
- Figures & tables
- Supplementary Data
Daniel A Anderson, Ross D Jones, Adam P Arkin, Ron Weiss, Principles of synthetic biology: a MOOC for an emerging field, Synthetic Biology , Volume 4, Issue 1, 2019, ysz010, https://doi.org/10.1093/synbio/ysz010
- Permissions Icon Permissions
Synthetic biology requires students and scientists to draw upon knowledge and expertise from many disciplines. While this diversity is one of the field’s primary strengths, it also makes it challenging for newcomers to acquire the background knowledge necessary to thrive. To address this gap, we developed a course that provides a structured approach to learning the biological principles and theoretical underpinnings of synthetic biology. Our course, Principles of Synthetic Biology (PoSB), was released on the massively open online course platform edX in 2016. PoSB seeks to teach synthetic biology through five key fundamentals: (i) parts and layers of abstraction, (ii) biomolecular modeling, (iii) digital logic abstraction, (iv) circuit design principles and (v) extended circuit modalities. In this article, we describe the five fundamentals, our formulation of the course, and impact and metrics data from two runs of the course through the edX platform.
Over the past two decades, the field of synthetic biology has grown to encompass a powerful set of integrated molecular and cellular engineering technologies, linked by an increasingly coherent set of standards for design and characterization ( 1 , 2 ). The field has had successful applications in health, agriculture and chemical/pharmaceutical production ( 1 , 3–5 ). However, the practice of synthetic biology draws on skills spanning cell and molecular biology, biophysics, chemical engineering, computer science, control theory and statistics. Due to this diversity, it is difficult to generate comprehensive educational and training resources for students interested in entering this field.
In recent years, several approaches have arisen to expand synthetic biology education. At the collegiate and high school level, the most well-known method of getting involved with synthetic biology is the annual International Genetically Engineered Machine (iGEM) competition ( 6 , 7 ). In iGEM, students can gain research experience through the design, construction and sharing of novel genetic circuits. While iGEM teams do learn relevant molecular biology and modeling techniques, a structured framework for understanding synthetic biology is not present. Another growing high school-level program is BioBuilder ( 8 ), through which students are introduced to synthetic biology via a variety of hands-on labs and activities ( 9 ). While BioBuilder provides an excellent introductory experience for students to learn basic synthetic biology theory and molecular biology skills, college-level students can benefit from a deeper theoretical foundation.
To accomplish the goal of providing a strong, unified framework for synthetic biology, we adapted Principles of Synthetic Biology (PoSB)—an upper-division course taught jointly at MIT and UC Berkeley by Professors Ron Weiss and Adam Arkin—for the massively online open course (MOOC) platform, edX ( https://www.edx.org/course/principles-synthetic-biology-mitx-20-305x-0 ). MOOCs are a modern educational development that makes college-level education material accessible to anyone with an internet connection. MOOCs can push beyond the traditional educational capacity of textbooks via a combination of videos, lecture notes, problems and user forums, which have been shown to increase information retention rates and positively influence motivation ( 10 , 11 ). Importantly, MOOCs are uniquely suited for emerging disciplines like synthetic biology because MOOCs can be easily updated every year as new literature is published. Finally, MOOCs can reach a large audience; edX is one of the most successful MOOC platforms with over 2.4 million unique users accessing one or more of its 290 courses between 2012 and 2016 ( 12 ).
In this article, we lay out the course content of PoSB and the core concepts used to guide its creation. Then, data from the first two runs of the course are discussed to assess the impact of the course and suggest alterations. We envision this article being of particular interest to educators and prospective students. Educators interested in integrating synthetic biology into their own curricula should find the Course Fundamentals and Course Outline sections particularly useful. These sections provide pedagogical principles and specific references to the course content that should be helpful in adapting portions of our course into new curricula. Prospective students should focus on the Course Outline and Course Formulation sections. These sections can be used to identify and target specific knowledge gaps as well as provide an understanding into the process of taking PoSB through the edX platform.
We expect that students with a background in biology will particularly benefit from the course’s insights into model design, digital logic and design-build-test approaches. Those with engineering backgrounds can learn how to model gene expression, how biological ‘machines’ can implement familiar functions, and how biological systems have fundamental limitations. PoSB is an upper-division course and, as such, we recommend prerequisite courses covering: molecular biology, math through integral calculus, basic programming and at least one organic chemistry course. Recommended subjects include: differential equations, electronic circuits, control theory and biochemistry.
PoSB is structured around five fundamentals: (i) parts and layers of abstraction; (ii) biomolecular modeling; (iii) digital abstractions; (iv) circuit design principles; and (v) extended circuit modalities ( Figure 1A ). This next section details these specific fundamentals and some of their pedagogical choices.

Course fundamentals and formulation. ( A ) The fundamental methodologies and abstractions used to design the course curriculum. ( B ) The many different modes that students use to engage with the course.
2.1 Parts and layers of abstraction
One of the first things we seek to teach in this course is our definition of a part in synthetic biology. From an engineering perspective, a ‘part’ is a useful abstraction for understanding and building larger systems ( 13 ). For synthetic biologists, these systems are often genetic regulatory networks. A biologist—caring about the mechanistic underpinnings of the system—may define their ‘part’ as a protein or a piece of DNA; a synthetic biologist—concerned with the capabilities of the system—will define their ‘part’ differently. For synthetic biology, we choose to define a part as a process that modifies the state of the cell. While the cell state modifications are enacted by a set of physical components within the cell, we seek to abstract ourselves from those components and define the part in terms of how it changes our system of interest ( Figure 2A ). In PoSB, we define the input of a part to be the current state of the cell (i.e. the concentrations of molecular species) and the output as the rate of change of those species (i.e. a differential equation for each of the species). We note that this definition of part is not yet a universally accepted definition within synthetic biology. Different approaches can benefit from other part definitions; however, this abstraction is well-suited for the analytical approaches used in PoSB and we believe this definition also has relevance for the general community. In the following paragraphs, we demonstrate the general utility of a PoSB part by using it to construct a hypothetical pulse generator circuit via hierarchical composition ( Figure 2 ).

Parts and layers of abstractions in the context of a pulse generator circuit. ( A ) The difference between physical components and functional parts in the example of a fluorescent reporter. ( B ) The individually profiled steady-state transfer functions of each pulse generator circuit part in isolation. The Input Comparator part has two inputs and its output is represented as a heatmap color (B) A full pulse-generator genetic circuit system (gray) made by combining parts (red) and modules (blue). A hypothetical time trace is shown for GFP fluorescence upon a step input of inducer.
Transcription factor (TF) activated GFP production is an example of a part that is presented early in the course. The input into this part is the concentration of an activator protein and the output is the production rate of GFP ( Figure 2A , Part). We note that this functional definition of a part is distinct from the physical components that make up the part: an activator, a promoter and the GFP gene ( Figure 2A , components).
A key aspect of parts is that they can be characterized in isolation and then predictably composed into larger systems. Higher-order functionalities can then be designed when individual parts are combined through well-defined input/output responses and interfaces. This compositional approach can be used in a hierarchical manner to design modules from individual parts and then systems from individual modules. In the case of the Pulse Generator Circuit system, the key functionality originates from the Pulse Dynamics Generator module. This module is created from two parts that take the activating Act1 input through two branches ( Figure 2B ). In the Input Comparator part, Act1 activates the output of the module, Act2. Soon after, delayed production of a repressor from Repressor Delay part overrides the activation in the Input Comparator part. As a whole, this results in only a brief spike of Act2 production. Once the Pulse Dynamics Generator module has been created, we can interface it with parts that have experimentally relevant inputs and outputs. The Inducible Input part allows for the initiation of a pulse and the Fluorescent Output part facilitates the readout of circuit state ( Figure 2B ). The final result is the Pulse Generator Circuit system ( Figure 2C ).
Layers of abstraction are a key engineering principle that allows the separation of high-level functional design from physical implementation. Our Pulse Generator Circuit is an example of how layers of abstraction can be leveraged to build complex systems. The first layer of abstraction is the definition of a functional part from physical components ( Figure 2A ). This abstracts the designer from the physical components that act on the system. The next layer of abstraction is the creation of a Pulse Dynamics Generator module from the Repression Delay and Input Comparator parts ( Figure 2C ). When working with the module, the designer only needs to know that providing an appropriate amount of Act1 input will result in a spike of Act2 production. They do not need to concern themselves with the specifics of two parts within the module, nor the numerous physical components that make up each part. The highest layer of abstraction is the whole Pulse Generator Circuit system. This system is built by combining the Pulse Dynamics Generator module with input- and output-interfacing parts. While a strong understanding of biology is required to design and connect these modular components, if the system is properly designed, much of the complex biology can be abstracted away. The designer is then free to focus on understanding higher-order functionalities of the system, such as the effect of inducer addition and removal on pulse shape and timing.
While the Pulse Generator Circuit system is the highest level of abstraction presented here, one can imagine how this system could, itself, become a part of an even larger system using the same abstraction methodologies.
2.2 Biomolecular modeling
A central premise of engineering disciplines is that model-driven design and diagnostics are critical for scaling devices, improving their reliability and learning from failures. As such, mathematical modeling has been an important aspect of synthetic biology since its inception ( 14 , 15 ). It has helped guide design, understand failure modes and propose new mechanisms for genetic circuits ( 16–18 ). Models can vary widely in complexity and ease of manipulation. As most of the analyses in PoSB focus on small genetic circuits, we chose to use relatively simple, state-space models built on mass-action kinetics principles ( 19 ). We first teach how to apply these models to very simple systems such as enzymatic reactions (Michaelis–Menten kinetics). Then, using the notion of parts, these models are progressively built up to eventually encapsulate entire genetic circuits. These models allow students to investigate the effect of parameters on circuit functionality in silico . To complement these models, we also include discussion on how and when our deterministic models may fail to capture real-world genetic circuits functionality due to phenomena such as stochasticity, small molecular counts and noise propagation. We mention that for these scenarios, probabilistic approaches such as Gillespie algorithms or chemical master equations are more informative. Building and manipulation of all models is completed within the edX system through an integrated MATLAB toolbox (Section 4.3).
2.3 Digital logic abstraction
After the first few sections of the course, students have learned how to compose and simulate the basic components of genetic circuits. Once the input–output functions for a part are defined, we demonstrate how in some parameter regimes, certain parts can exhibit ultrasensitive response functions to yield digital-like behavior. We then use these parts as building blocks to develop higher-order genetic circuit functionality like computing digital logic functions. We focus on digital abstractions because they are a useful tool for simplifying the high-level design and analysis of genetic circuits.
Students are taught digital circuitry from an electrical engineering perspective to motivate and guide the construction of circuits with complex capabilities. This requires students to first learn how to define problems and solutions in terms of combinational logic problems (e.g. identify a cell as a cancer cell if certain miRNAs are present and/or absent). Once combinational circuits are outlined and motivated, we apply some basic computer science tools for reducing and manipulating digital logic circuits: Boolean cubes and Karnaugh maps. After students have learned to form basic digital circuits, we present the biological components that can be used to enact digital logic.
2.4 Circuit design principles
After the basic components of genetic circuits have been presented, we dive in to some of the higher-order properties that emerge when larger circuits are built. First, we discuss simple cascades in both steady-state and dynamical situations. We then discuss common circuit topologies such as feedforward and feedback and highlight their unique dynamical properties. From there, we study the design features of toggle switches and oscillators to demonstrate how they can be used to create advanced circuits for coordinated timing and control of cellular state. Finally, problems of scalability and modularity are discussed to illustrate current engineering challenges in the field.
Throughout these discussions, we emphasize using modeling methods to identify and design around possible failure modes for circuits. Collectively, these topics teach students about fundamental biological circuit motifs, their composition into larger circuits, and how to reduce trial-and-error when experimentally implementing a circuit. This helps students understand how modeling can be used to avoid problems such as timing hazards and mismatched transfer functions.
2.5 Extended circuit modalities
In the final section of the course, we introduce a variety of genetic circuit modalities that exhibit expanded input/output types, dynamics, safety and robustness. Since many of the early course concepts are demonstrated with TF-based circuitry, we start with a survey of scalable TF-based circuit components including TetR homologs, zinc-finger nucleases (ZFNs), TALEs and CRISPRi. We then present RNA-based components in both bacterial and mammalian systems. For the RNA components, we highlight the unique sensing capabilities of miRNA circuits and aptazymes, as well as the improved safety of RNA-only circuits, which do not require DNA encoding. Entirely protein-based circuits are then discussed and their fast dynamics are highlighted through the load driver application ( 20 ). Lastly, we present recombinase circuits and highlight their improved robustness for genetically encoded memory. Together, these diverse modalities expose students to the breadth of engineering capabilities that synthetic biologists use to control cellular processes.
The fundamental goal of the PoSB curriculum is to bring together engineering principles with biological implementations. In Table 1 , we outline the primary course contents of the most recent PoSB iteration (as of this writing, Spring 2017) and organize them with respect to each course fundamental. A brief description accompanies each topic along with pointers to the relevant course material (L indicating lectures and PS indicating problem sets). As synthetic biology fundamentally necessitates and builds upon biology, relevant biological background material is provided in the ungraded Problem Set 0 (PS0). Within PS0, we also provide links to free online introductory biology courses through MIT OpenCourseWare. As shown in Table 1 , PS0 also contains background materials for differential equations, digital logic and measurement methods.
Primary course content
Fundamental . | Specific content . | Description . | Pointer . |
---|---|---|---|
Parts and layers of abstraction | Top-down design | Methods for designing composable and modular biological circuits | L2/3 |
Parts and composition | Defining parts as processes in biological systems and simple modeling introduction | L5/6 | |
Biomolecular modeling | ODE background | Introduction to mass-action kinetics and using ODEs to describe cellular processes | PS0 |
Michaelis–Menten | Modeling and engineering enzymatic reactions with Michaelis–Menten kinetics | L6 | |
Gene expression | Capturing simple transcription and translation with mathematical equations | L7 | |
Digital logic abstractions | Digital background | Introduction to basic digital logic ideas including gates, circuits and truth tables | PS0 |
Digital biology | Digital logic abstractions in biology with transcriptional repressors as NOT/NOR gates | L9 | |
Logic minimization | Designing and simplifying arbitrary digital circuits with uniting theorem and Karnaugh maps | L16 | |
Designing with cello | Description of how Cello genetic circuit design software works ( ) | L15 | |
Circuit design principles | Cascades | Layering transcriptional components and predicting function and timing hazards | L10/11 |
Feedback loops | Negative and positive gene autoregulation and 1D graphical analysis of ODEs | L8 | |
Feedforward loops | Feedforward loop network motifs, their dynamical properties and biological implications | L12 | |
Toggle Switches | Using feedback to build memory devices and 2D graphical ODE analysis | L13 | |
Oscillators | Using feedback and feedforward to build oscillating biological circuits | L14 | |
Extended circuit modalities | Transcription factors | Survey of scalable transcriptional regulators: TetR homologs, TALEs, ZFNs and CRISPRi/a | L17 |
Prokaryotic RNAs | Survey of RNA control in prokaryotes: RNA-IN/OUT and aptamers | L18 | |
Eukaryotic RNAs | Survey of RNA control in eukaryotes: RNAi and replicons | L19 | |
Protein circuits | Survey of protein-based circuits: phosphorelays, allostery, scaffolding and the load driver | L20 | |
Recombinases | Survey of recombinase-based circuits used for memory and digital logic | L21 |
Fundamental . | Specific content . | Description . | Pointer . |
---|---|---|---|
Parts and layers of abstraction | Top-down design | Methods for designing composable and modular biological circuits | L2/3 |
Parts and composition | Defining parts as processes in biological systems and simple modeling introduction | L5/6 | |
Biomolecular modeling | ODE background | Introduction to mass-action kinetics and using ODEs to describe cellular processes | PS0 |
Michaelis–Menten | Modeling and engineering enzymatic reactions with Michaelis–Menten kinetics | L6 | |
Gene expression | Capturing simple transcription and translation with mathematical equations | L7 | |
Digital logic abstractions | Digital background | Introduction to basic digital logic ideas including gates, circuits and truth tables | PS0 |
Digital biology | Digital logic abstractions in biology with transcriptional repressors as NOT/NOR gates | L9 | |
Logic minimization | Designing and simplifying arbitrary digital circuits with uniting theorem and Karnaugh maps | L16 | |
Designing with cello | Description of how Cello genetic circuit design software works ( ) | L15 | |
Circuit design principles | Cascades | Layering transcriptional components and predicting function and timing hazards | L10/11 |
Feedback loops | Negative and positive gene autoregulation and 1D graphical analysis of ODEs | L8 | |
Feedforward loops | Feedforward loop network motifs, their dynamical properties and biological implications | L12 | |
Toggle Switches | Using feedback to build memory devices and 2D graphical ODE analysis | L13 | |
Oscillators | Using feedback and feedforward to build oscillating biological circuits | L14 | |
Extended circuit modalities | Transcription factors | Survey of scalable transcriptional regulators: TetR homologs, TALEs, ZFNs and CRISPRi/a | L17 |
Prokaryotic RNAs | Survey of RNA control in prokaryotes: RNA-IN/OUT and aptamers | L18 | |
Eukaryotic RNAs | Survey of RNA control in eukaryotes: RNAi and replicons | L19 | |
Protein circuits | Survey of protein-based circuits: phosphorelays, allostery, scaffolding and the load driver | L20 | |
Recombinases | Survey of recombinase-based circuits used for memory and digital logic | L21 |
In addition to the content centered around the five course fundamentals, we also included material on methods and applications in synthetic biology, as depicted in Table 2 .
Additional course content
Topic . | Specific content . | Description . | Pointer . |
---|---|---|---|
Methods | Measurement | How biological measurements are made: reporters, flow cytometry, microscopy | PS0 |
DNA assembly | Survey of DNA assembly: restriction enzymes, Gibson, Golden Gate, Gateway | L4 | |
Applications | Tissue homeostasis | Design of a synthetic circuit to maintain β cell populations in type I diabetes patients | L3 |
Cancer classifier | Design of a circuit to kill cancer cells through miRNA detection and digital logic | L19 | |
Morphogenesis | Designing cellular patterning and organoids with cell-to-cell communication | L22 |
Topic . | Specific content . | Description . | Pointer . |
---|---|---|---|
Methods | Measurement | How biological measurements are made: reporters, flow cytometry, microscopy | PS0 |
DNA assembly | Survey of DNA assembly: restriction enzymes, Gibson, Golden Gate, Gateway | L4 | |
Applications | Tissue homeostasis | Design of a synthetic circuit to maintain β cell populations in type I diabetes patients | L3 |
Cancer classifier | Design of a circuit to kill cancer cells through miRNA detection and digital logic | L19 | |
Morphogenesis | Designing cellular patterning and organoids with cell-to-cell communication | L22 |
The PoSB online content interleaves lecture videos, written notes, practice problems and discussion boards. The course is designed as an instructor-paced course running the length of a semester. Each week, two complete lectures are released. There are five problem sets, a midterm, a final exam and a final course project. In this section, we will describe the formulation of the course, including how we developed lectures, the types of problems we created and how we managed the students’ final projects ( Figure 1B ). While the course content was initially designed to fit into a semester timeline, we believe it could be adapted to a two-quarter course, with the first quarter focusing on the core theory and the second quarter expanding upon this theory to more complex circuits and applications. Following this strategy, our recommendation for the first quarter would be to teach the first four fundamentals: (i) parts and layers of abstraction, (ii) biomolecular modeling, (iii) digital logic abstraction and (iv) circuit design principles. The second quarter could then cover the final fundamental, Extended Circuit Modalities, as well as the Methods and Applications sections.
4.1 Interactive lectures
Most of the PoSB course content is delivered via interactive lectures, which include: video lectures delivered by professors Weiss and Arkin, detailed lecture notes, practice problems and discussion boards tied to specific questions raised during the lectures. As a residential course, PoSB is cotaught via a videoconference set up between MIT and Berkeley. In the edX adaptation of these lectures, we chose to include some in-class discussions from the residential course. EdX students are given a chance to share their thoughts on these in-class discussion topics with their peers via embedded discussion boards. Each video segment is also accompanied by detailed notes that reiterate the major points, provide additional context and supply links to referenced papers.
To help reinforce student learning, we added occasional problems in line with the lecture notes. Some of these problems are designed to give students practice with technical topics, while other problems encourage student exploration of model systems. For example, there are many live MATLAB coding boxes which can be used to modify and simulate the models discussed in lecture. We also built a genetic circuit interpreter that students can use to evaluate the logic of TF and miRNA-based regulation. When combined with the discussion elements, these types of problems allow for deeper student engagement beyond simple multiple-choice problems.
4.2 Moderated discussions
Discussion boards are placed alongside lecture content and can also be accessed through a central forum. These discussion boards end up serving many different purposes. Discussion boards within interactive lectures have thought-provoking questions such as, ‘is a cell more like a computer or a burrito?’. Discussion boards in problem sets allow students to work together to solve the problems as if they are in a study group. For the final project, discussion boards help students develop and share their ideas.
The discussion boards are pivotal to scaling the course to thousands of students. Although our resources are limited, students can help to answer each other’s questions through the boards. Additionally, the discussion boards allow us to easily solicit course improvements and identify bugs. For many students, getting to work together and interact with other students provides a unique opportunity to engage with synthetic biology in a way that may have otherwise been inaccessible.
4.3 Parts-Compositors MATLAB framework
To put into practice our concept of a part, we built a custom MATLAB-based numerical simulation framework that students can use to quickly build and simulate genetic circuits ( https://github.com/Weiss-Lab/Parts-Compositors ). The framework is designed to be used in an object-oriented manner, with each part of the biological system being an object. Our framework allows students to simulate models without having to directly code ordinary differential equation (ODE) simulations themselves. MATLAB code is run directly in the browser using interactive coding boxes placed within lectures, problem sets and exams. The coding boxes were developed by MathWorks, the creators of MATLAB.
4.4 Problem sets and exams
Problem set and exam questions in the course can be divided into four categories: multiple choice, drag-and-drop, free response and MATLAB-based. Many of our drag-and-drop problems involve placing labels for genetic components onto circuit diagrams. These test the students’ ability to process a description of a model system, reason through the logic of how components interact, and then place those components into a valid arrangement that satisfies a given specification. Free-response problems require students to enter quantitatively correct information. For example, some questions request students to analyze logic gates, input reaction rates and compute steady-state solutions to a given model. The MATLAB-based questions have students use the Parts-Compositors framework to gain insights into circuit function through various questions integrated with the MATLAB code boxes.
Before being released on edX, the problem sets and exams were prototyped with residential classes at UC Berkeley and MIT. We used feedback from residential students and members of the Weiss and Arkin labs to improve problem design, adjust problem sets/exam composition and debug complicated problems in advance of the wider edX release.
4.5 Student projects
A final project is included to encourage students to design and analyze a synthetic biology system by building upon what they learned in the course. For this project, students identify a biological problem of interest, design a synthetic biology-based solution and model their synthetic system to identify key parameters and design constraints. Through the discussion boards, students can discuss their ideas, solicit feedback and eventually share their final projects for all to see. To facilitate the grading of projects, we require students submitting final projects to peer-evaluate each other’s work. This allows for the large-scale evaluation of student projects. While peer review can be inaccurate ( 22 ), review accuracy may be improved by moving from numerical to ranked peer-scoring in future iterations of PoSB ( 23 ). Ultimately, peer evaluation provides one final opportunity for students to engage with the diverse subject matter of synthetic biology before finishing the course.
The edX version of PoSB has had two runs: Spring 2016 (v1) and Spring 2017 (v2). As of November 2018, there were 28 623 signups for v1 and v2. However, only 17 229 students viewed any course material (a 60% follow-through rate compared with an average edX follow-through rate of 53%) ( 12 ). We further stratify students who viewed the course material into three types: (i) 11 768 on-time students (signed up before the second problem set was due); (ii) 1595 late students (signed up after the second problem set was due, but before the course archive date); and (iii) 3866 archival students (signed up after the course was archived). All further analyses only consider students who signed up on time and therefore experienced the desired course pacing ( N = 11 768). In this next section, we analyze these students’ demographics and course activity in the context other edX STEM courses.
The broad reach of our course is apparent when looking at the global distribution of students. Across both runs of the course, students hailed from 150 different countries ( Figure 3A ). Students most frequently came from the USA (28%). This is similar to the median domestic percentage of all edX STEM courses (25%) ( 12 ). The next most frequent countries were India (7%) and Mexico (5%). Additionally, the median PoSB student age was 26, identical to that of all edX STEM courses ( Figure 3B ) ( 12 ). Interestingly, our student demographics deviated from edX median values in two ways: we had a higher proportion of female-declared participants ( Figure 3C ) and a lower percentage of bachelor-degree-holding students ( Figure 3D ). The reasons for these deviations were not clear.

Course demographics and engagement metrics. ( A ) Counts of the total number of participating students from each country shown on a logarithmic scale. Countries with no students are grey. ( B ) Age distribution for both runs of the course. The median age for edX STEM courses is indicated with a dashed line. ( C ) The self-identified gender distributions of v1 and v2 of the course alongside edX STEM median gender values. ( D ) The highest level of education indicated by students. Cumulative edX data were only available for the Bachelor+ category (Bachelor, Masters and/or PhD). ( E ) Percentages of students that correctly answered at least one problem (‘Correct Problem’), made at least one post in the discussion board (‘Posted’), and viewed more than 50% of the course content (‘Explorer’). Median values for edX STEM course are displayed alongside.
When looking at student engagement in the course, we found some fairly large differences from the edX STEM median values ( Figure 3E ). Students in PoSB were significantly less likely to correctly answer at least one problem in the course than the edX median (6.5% compared with the edX median of 16%). This may be the result of higher problem difficulty or less general interest in completing problems. Students were also less likely to become ‘explorers’ (a term for students who viewed at least half of the course). This indicated a higher than usual course attrition rate. As shown by student posting rates, the discussion board activity was healthy and similar to standard edX STEM courses ( Figure 3E ). We initially considered certification rate as an engagement metric, but it is an unreliable method of comparing courses due to the fact that edX courses vary greatly in their requirements for certification (grade value, payment required, problem difficulty, etc.) ( 12 ). Other MOOCs have had their effectiveness evaluated by comparing baseline knowledge tests given before and after students take the course ( 24 ). While we did not administer these tests, we plan to do this in future iterations of the course.
Between the two runs of the course, the main difference was the enrollment number. The first run of the course attracted 9282 participating students, while the second run had only 2459 (26% of the original). We note that it is normal to observe a drop in enrollment for the second run of a course (edX median is 75% of the original course enrollment rate) ( 12 ). While our drop in enrollment was a bit more than the edX median, the demographic and engagement profiles were similar for both runs of our course. This indicates that the drop is likely due to differences in advertising and/or course demand rather than specific differences in course content/management.
Overall, the two runs of PoSB have managed to reach a broad audience that is generally similar to the student demographics of a typical edX STEM course. While we do see a healthy and active discussion board, we acknowledge that our course does have a higher-than-average attrition rate. We plan to address this issue in future iterations of the course by streamlining content to improve approachability and by adding easier and more frequent in-lecture problems to improve engagement.
Our goal with the PoSB edX course was to create a broadly accessible, structured introduction to synthetic biology. From the first two runs of the course, we believe that we were successful in this goal. Over two years, PoSB has managed to reach over 10 000 students from more than 150 countries. This is a scale of education that is only possible through a MOOC platform like edX.
Synthetic biology is a rapidly progressing field and PoSB represents our effort to deliver the latest research insights while simultaneously grounding students in the engineering and biological principles needed to understand the field. With this article, we aimed to break down the course structure, content and ideas so that students and educators interested in learning about and teaching synthetic biology can begin to engage with and use our content. For the students and educators who are interested in a more detailed understanding of the course, all PoSB material is accessed through the edX platform. We note that for the first two iterations of the course, all PoSB content was freely available—in perpetuity—to all students. A recent policy change by edX requires students to pay a small fee for access to exam/project materials and archived versions of the course. However, the core aspects of PoSB—lecture videos/notes, practice problems and problem sets—will still be freely available for the duration of course runs.
Course demographics and engagement data are not publicly available for reasons of student privacy.
We would like to thank everyone who helped make PoSB on edX possible. Former TAs that helped develop class content: Jeremy Gam, Eerik Kaseniit, Jacob Becraft, Mohammad Soheilypour, Tracy Washington, Sarvesh Varma, Sebastian Palacios, Guillaume Kugener and Sepideh Dolatshahi. Current TAs working on PoSB v3: Yuge Ji, Raphaël Gayet and Ching Fang. MITx Staff: Brad Goodman, Kyle Boots, Colleen Cressman, Lana Scott, Lisa Eichel, Garrett Beazley and Edwin Cabrera. MATLAB support: Michael Reardon. Administrative support: Olga Parkin. The Synthetic Biology Institute at UC Berkeley and Synthetic Biology Center at MIT. The Departments of Biological Engineering at MIT and Bioengineering at UC Berkeley. And all the MIT, UC Berkeley and edX students who gave feedback on the problem sets and exams.
Development of PoSB on edX was supported by funding from the MIT Department of Biological Engineering, MIT Department of Electrical Engineering and Computer Science, UC Berkeley Department of Bioengineering, and a generous donation from the Wertheimer Foundation. Additional funding was provided by the National Science Foundation [1521925].
Conflict of interest statement . None declared.
Cameron D.E. , Bashor C.J. , Collins J.J. ( 2014 ) A brief history of synthetic biology . Nat. Rev. Microbiol ., 12 , 381 – 390 .
Google Scholar
Bartley B.A. , Rodriguez C.A. , Misirli G. , Hillson N.J. , Galdzicki M. , Endy D. , Grünberg R. , Hallinan J. , Roehner N. , Stan G.-B. et al. ( 2014 ) The Synthetic Biology Open Language (SBOL) provides a community standard for communicating designs in synthetic biology . Nat. Biotechnol ., 32 , 545 – 550 .
Khalil A.S. , Collins J.J. ( 2010 ) Synthetic biology: applications come of age . Nat. Rev. Genet ., 11 , 367 – 379 .
Weber W. , Fussenegger M. ( 2012 ) Emerging biomedical applications of synthetic biology . Nat. Rev. Genet ., 13 , 21 – 35 .
Shih P.M. , Liang Y. , Loqué D. ( 2016 ) Biotechnology and synthetic biology approaches for metabolic engineering of bioenergy crops . Plant J ., 87 , 103 – 117 .
Mitchell R. , Dori Y.J. , Kuldell N.H. ( 2011 ) Experiential engineering through iGEM—an undergraduate summer competition in synthetic biology . J. Sci. Educ. Technol ., 20 , 156 – 160 .
Dubé S. , Orr D. , Dempsey B. , Wieden H.J. ( 2017 ) A synthetic biology approach to integrative high school STEM training . Nat. Biotechnol ., 35 , 591 – 595 .
Dixon J. , Kuldell N. ( 2011 ) Chapter twelve - BioBuilding: Using Banana-Scented Bacteria to Teach Synthetic Biology. In: Voigt,C. (ed). Synthetic Biology, Part A . Vol. 497 . Academic Press, pp. 255 – 271 .
Google Preview
Kuldell N , Bernstein R. , Ingram K. , Hart K.M. ( 2015 ) Chapter 5: Introduction to the BioBuilder Labs. In: Loukides M. , MacDonald B. (eds). BioBuilder | Synthetic Biology in the Lab First . O’Reilly Media , Sebastopol, CA , pp. 91–94.
Epstein M.L. , Lazarus A.D. , Calvano T.B. , Matthews K.A. , Hendel R.A. , Epstein B.B. , Brosvic G.M. ( 2002 ) Immediate feedback assessment technique promotes learning and corrects inaccurate first responses . Psychol. Rec ., 52 , 187 – 201 .
Barak M. , Watted A. , Haick H. ( 2016 ) Motivation to learn in massive open online courses: examining aspects of language and social engagement . Comput. Educ ., 94 , 49 – 60 .
Chuang I. , Ho A. ( 2016 ) HarvardX and MITx: four years of open online courses—fall 2012-summer 2016. Available at SSRN: https://ssrn.com/abstract=2889436 or http://dx.doi.org/10.2139/ssrn.2889436 .
Endy D. ( 2005 ) Foundations for engineering biology . Nature , 438 , 449 – 453 .
Elowitz M.B. , Leibler S. ( 2000 ) A synthetic oscillatory network of transcriptional regulators . Nature , 403 , 335 – 338 .
Gardner T.S. , Cantor C.R. , Collins J.J. ( 2000 ) Construction of a genetic toggle switch in Escherichia coli . Nature , 403 , 339 – 342 .
Endler L. , Rodriguez N. , Juty N. , Chelliah V. , Laibe C. , Li C. , Novere N.L. ( 2009 ) Designing and encoding models for synthetic biology . J. R. Soc. Interface , 6 , S405 – S417 .
Chandran D. , Copeland W.B. , Sleight S.C. , Sauro H.M. ( 2008 ) Mathematical modeling and synthetic biology . Drug Discov. Today Dis. Model , 5 , 299 – 309 .
Hucka M. , Finney A. , Sauro H.M. , Bolouri H. , Doyle J.C. , Kitano H. , Arkin A.P. , Bornstein B.J. , Bray D. , Cornish-Bowden A. et al. ( 2003 ) The systems biology markup language (SBML): a medium for representation and exchange of biochemical network models . Bioinformatics , 19 , 524 – 531 .
Domitilla D.V., , Murray R.M. ( 2014 ) Biomolecular Feedback Systems . Princeton University Press, Princeton and Oxford. ISBN: 9780691161532.
Mishra D. , Rivera P.M. , Lin A. , Del Vecchio D. , Weiss R. ( 2014 ) A load driver device for engineering modularity in biological networks . Nat. Biotechnol ., 32 , 1268 – 1275 .
Nielsen A.A.K. , Der B.S. , Shin J. , Vaidyanathan P. , Paralanov V. , Strychalski E.A. , Ross D. , Densmore D. , Voigt C.A. ( 2016 ) Genetic circuit design automation . Science , 352 , aac7341 .
Suen H.K . ( 2014 ) Peer assessment for Massive Open Online Courses (MOOCs. ) . Int. Rev. Res. Open Distrib. Learn ., 15 , 312–327.
Shah N.B. , Bradley J.K. , Parekh A. , Wainwright M. , Ramchandran K. ( 2013 ) A case for ordinal peer-evaluation in MOOCs . NIPS Workshop on Data Driven Education , pp. 1–8.
Colvin K.F. , Champaign J. , Liu A. , Zhou Q. , Fredericks C. , Pritchard D.E. ( 2014 ) Learning in an introductory physics MOOC: all cohorts learn equally, including an on-campus class . Int. Rev. Res. Open Distrib. Learn ., 15 , 263 – 283 .
Author notes
Month: | Total Views: |
---|---|
May 2019 | 122 |
June 2019 | 205 |
July 2019 | 450 |
August 2019 | 421 |
September 2019 | 445 |
October 2019 | 600 |
November 2019 | 585 |
December 2019 | 554 |
January 2020 | 640 |
February 2020 | 547 |
March 2020 | 496 |
April 2020 | 291 |
May 2020 | 352 |
June 2020 | 348 |
July 2020 | 205 |
August 2020 | 258 |
September 2020 | 222 |
October 2020 | 190 |
November 2020 | 175 |
December 2020 | 183 |
January 2021 | 228 |
February 2021 | 195 |
March 2021 | 177 |
April 2021 | 148 |
May 2021 | 171 |
June 2021 | 127 |
July 2021 | 115 |
August 2021 | 103 |
September 2021 | 148 |
October 2021 | 125 |
November 2021 | 89 |
December 2021 | 119 |
January 2022 | 116 |
February 2022 | 99 |
March 2022 | 120 |
April 2022 | 111 |
May 2022 | 104 |
June 2022 | 76 |
July 2022 | 64 |
August 2022 | 86 |
September 2022 | 89 |
October 2022 | 72 |
November 2022 | 62 |
December 2022 | 69 |
January 2023 | 106 |
February 2023 | 79 |
March 2023 | 62 |
April 2023 | 53 |
May 2023 | 59 |
June 2023 | 65 |
July 2023 | 90 |
August 2023 | 91 |
September 2023 | 76 |
October 2023 | 67 |
November 2023 | 91 |
December 2023 | 84 |
January 2024 | 93 |
February 2024 | 91 |
March 2024 | 115 |
April 2024 | 102 |
May 2024 | 77 |
June 2024 | 109 |
July 2024 | 66 |
August 2024 | 63 |

Email alerts
Citing articles via.
- Journals Career Network
Affiliations
- Online ISSN 2397-7000
- Copyright © 2024 Oxford University Press
- About Oxford Academic
- Publish journals with us
- University press partners
- What we publish
- New features
- Open access
- Institutional account management
- Rights and permissions
- Get help with access
- Accessibility
- Advertising
- Media enquiries
- Oxford University Press
- Oxford Languages
- University of Oxford
Oxford University Press is a department of the University of Oxford. It furthers the University's objective of excellence in research, scholarship, and education by publishing worldwide
- Copyright © 2024 Oxford University Press
- Cookie settings
- Cookie policy
- Privacy policy
- Legal notice
This Feature Is Available To Subscribers Only
Sign In or Create an Account
This PDF is available to Subscribers Only
For full access to this pdf, sign in to an existing account, or purchase an annual subscription.
Please enable JavaScript in your web browser to get the best experience.
We use cookies to track usage and preferences.
Primary navigation
- PhD students
- Exhibitions
- Translation
- Work experience
Synthetic Biology

Synthetic biology brings together knowledge of biological processes with engineering principles to build novel systems from a biological set of parts, or reveal more about the control of natural biological pathways.
Research groups
Paula booth.
Membrane Protein Folding laboratory
James Briscoe
Developmental Dynamics Laboratory
Aleksey Chudnovskiy
Tumour-Immune Communication Laboratory
Erika Alden DeBenedictis
Biodesign Laboratory
Greg Findlay
Genome Function Laboratory
Fabian Fröhlich
Dynamics of Living Systems Laboratory
Charlie McTernan
Artificial Molecular Machinery Laboratory
Naomi Moris
Developmental Models Laboratory
Cell Cycle Laboratory
David Riglar
Gut Microbiome Engineering Laboratory
Sam Rodriques
Applied Biotechnology Laboratory
Anne Schreiber
Cellular Degradation Systems Laboratory
Ben Schumann
Chemical Glycobiology Laboratory
Vahid Shahrezaei
Computational Molecular Systems Biology Laboratory
Molly Stevens
Biomaterials Design Lab
Chemical Biology & Therapeutic Discovery Satellite Laboratory
Jean-Paul Vincent
Epithelial Cell Interactions Laboratory
Science Technology Platforms
Share the page.
- Share on Twitter
- Share on Facebook
- Share on LinkedIn
- Share on Email
The Francis Crick Institute is a unique partnership between

- Introduction to Genomics
- Educational Resources
- Policy Issues in Genomics
- The Human Genome Project
- Funding Opportunities
- Funded Programs & Projects
- Division and Program Directors
- Scientific Program Analysts
- Contacts by Research Area
- News & Events
- Research Areas
- Research Investigators
- Research Projects
- Clinical Research
- Data Tools & Resources
- Genomics & Medicine
- Family Health History
- For Patients & Families
- For Health Professionals
- Jobs at NHGRI
- Training at NHGRI
- Funding for Research Training
- Professional Development Programs
- NHGRI Culture
- Social Media
- Broadcast Media
- Image Gallery
- Press Resources
- Organization
- NHGRI Director
- Mission and Vision
- Policies and Guidance
- Institute Advisors
- Strategic Vision
- Leadership Initiatives
- Diversity, Equity, and Inclusion
- Partner with NHGRI
- Staff Search
Synthetic Biology
Synthetic biology is a field of science that involves redesigning organisms for useful purposes by engineering them to have new abilities. Synthetic biology researchers and companies around the world are harnessing the power of nature to solve problems in medicine, manufacturing and agriculture.
What can synthetic biology do?
Redesigning organisms so that they produce a substance, such as a medicine or fuel, or gain a new ability, such as sensing something in the environment, are common goals of synthetic biology projects. Some examples of what scientists are producing with synthetic biology are:
- Microorganisms harnessed for bioremediation to clean pollutants from our water, soil and air.
- Rice modified to produce beta-carotene , a nutrient usually associated with carrots, that prevents vitamin A deficiency . Vitamin A deficiency causes blindness in 250,000 - 500,000 children every year and greatly increases a child's risk of death from infectious diseases.
- Yeast engineered to produce rose oil as an eco-friendly and sustainable substitute for real roses that perfumers use to make luxury scents.
What is the difference between synthetic biology and genome editing?
In some ways, synthetic biology is similar to another approach called " genome editing " because both involve changing an organism's genetic code; however, some people draw a distinction between these two approaches based on how that change is made. In synthetic biology, scientists typically stitch together long stretches of DNA and insert them into an organism's genome. These synthesized pieces of DNA could be genes that are found in other organisms or they could be entirely novel. In genome editing, scientists typically use tools to make smaller changes to the organism's own DNA. Genome editing tools can also be used to delete or add small stretches of DNA in the genome.
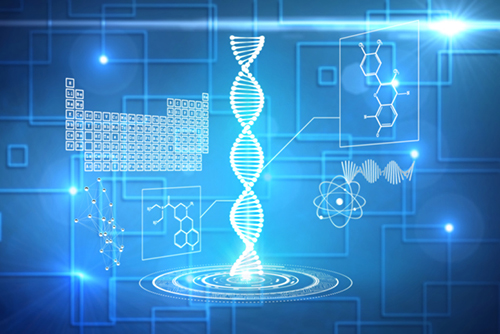
Can you synthesize an organism's entire genome?
Can researchers synthesize an organism's entire genome? The answer to this question is yes, and it has already been done. In 2002, scientists in the United States synthesized a viral genome for the first time. Viral genomes are much smaller compared to the genomes of most bacteria and microorganisms. Scientists showed that it was possible to create the polio virus from scratch and brought attention to the risk that synthetic biology could be used to develop biological weapons. While this group of researchers did not intend to cause harm with their research, their work understandably raised concerns that bad actors might use synthetic biology for malicious purposes. Please see the " What are the ethical and social implications ?" section of this resource to learn about the federal regulations in place to regulate so-called " dual use research of concern ", or research that could be directly misapplied to pose a significant threat to public health and safety, agricultural crops and other plants, animals, the environment, or national security.
The first synthetic bacterial genome was completed in 2008 with the synthesis of the genome of Mycoplasm genitalium , a bacterium that can cause urinary and genital tract infections in humans. In 2017, another group of scientists partially synthesized the genome of Saccharomyces cerevisiae , the yeast that is used to make bread and brew wine and beer.
Today, researchers are continuing to push the limits of existing DNA-synthesis technology to help understand how genomes work. One group of researchers, called the "Genome Project-Write" (GP-Write)", is seeking to synthesize, or "write" whole genomes from human cell lines and the genomes of other plants and animals important to agriculture and public health. The name of their project is a play on the Human Genome Project (HGP). In 2003, scientists working on the HGP sequenced , or "read", the more than 3 billion DNA letters, or base pairs, that make up the human genome. One of the leading motivations for GP-Write is to stimulate innovation in DNA synthesis technologies through the proposed research. Importantly, the research in GP-Write involving human genomes will occur only in cells and no human embryos will be used in this research.
What are the ethical and social implications?
Projects that propose to synthesize entire genomes raise important ethical questions about potential harms and benefits to society. Many of the ethical questions relevant to synthetic biology are similar to ethical discussions related to genome editing . Are humans crossing moral boundaries by redesigning organisms with synthetic biology techniques? If synthetic biology yields new treatments and cures for diseases, who in our society will have access to them? What are the environmental impacts of introducing modified organisms into the ecosystem? Such ethical questions have been the subject of research since the beginning of the HGP and will continue to be researched as technology evolves and changes. Most scientists, ethicists and policymakers agree that entire societies must discuss and weigh the potential harms and benefits of synthetic biology in order to answer these questions. Leading voices in bioethics, including the Presidential Commission for the Study of Bioethical Issues and the National Academies of Sciences, Engineering and Medicine , have expressed the importance of public engagement and dialogue in the governance of emerging synthetic biology and genome editing technologies.
As the synthesis of the polio virus demonstrates, there are also biosecurity concerns related to synthetic biology. The US government's Federal Select Agents Program regulates the possession of high-risk infectious agents like polio for research and other purposes. Additionally, federally-funded research, such as research supported by the National Institutes of Health (NIH) that involves high-risk infectious agents, is subject to additional oversight and risk management as laid out by the Dual Use Research of Concern (DURC) policy. For more information about the biosecurity policies that NIH has in place, please visit this website . More broadly, the federal government has a policy in place, called the Coordinated Framework for Regulation of Biotechnology , to oversee the introduction of synthetic biology products into the market.
For additional resources about the ethics, governance and societal implications of synthetic biology, please refer to the following websites and publications:
Resources Related to the Ethical and Social Implications of Synthetic Biology:
- Biodefense in the Age of Synthetic Biology (National Academies of Sciences, Engineering, and Medicine)
- Human Genome Editing: Science, Ethics, and Governance (National Academies of Sciences, Engineering, and Medicine)
- New Directions: The Ethics of Synthetic Biology and Emerging Technologies (Presidential Commission for the Study of Bioethical Issues)
Additional Resources
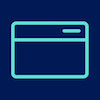
Building with Biology
Synthetic Biology News
Last updated: August 14, 2019
Thank you for visiting nature.com. You are using a browser version with limited support for CSS. To obtain the best experience, we recommend you use a more up to date browser (or turn off compatibility mode in Internet Explorer). In the meantime, to ensure continued support, we are displaying the site without styles and JavaScript.
- View all journals
- Explore content
- About the journal
- Publish with us
- Sign up for alerts
Synthetic biology articles within Scientific Reports
Article 01 August 2024 | Open Access
Engineering a GPCR-based yeast biosensor for a highly sensitive melatonin detection from fermented beverages
- Ricardo Bisquert
- , Alba Guillén
- & José M. Guillamón
Article 10 July 2024 | Open Access
Combination of error-prone PCR (epPCR) and Circular Polymerase Extension Cloning (CPEC) for improving the coverage of random mutagenesis libraries
- Natalia Ossa-Hernández
- , Luis Fernando Marins
- & Daniela Volcan Almeida
Article 18 June 2024 | Open Access
PBP-A, a cyanobacterial dd -peptidase with high specificity for amidated muropeptides, exhibits pH-dependent promiscuous activity harmful to Escherichia coli
- Gol Mohammad Dorrazehi
- , Matthias Winkle
- & Patrice Soumillion
Article 06 June 2024 | Open Access
Profiling expression strategies for a type III polyketide synthase in a lysate-based, cell-free system
- Tien T. Sword
- , Jaime Lorenzo N. Dinglasan
- & Constance B. Bailey
Article 18 May 2024 | Open Access
In vitro selection of DNA aptamers against staphylococcal enterotoxin A
- Ricardo Oliveira
- , Eva Pinho
- & Carina Almeida
Article 01 May 2024 | Open Access
Development of a new caged intein for multi-input conditional translation of synthetic mRNA
- Tingting Yang
- , Hideyuki Nakanishi
- & Keiji Itaka
Article 29 April 2024 | Open Access
Synthesis, molecular docking studies and biological evaluation of N -(4-oxo-2-(trifluoromethyl)-4 H -chromen-7-yl) benzamides as potential antioxidant, and anticancer agents
- Sumalatha Jorepalli
- , Sreedevi Adikay
- & Rama Rao Karri
Article 26 April 2024 | Open Access
Comprehensive evaluation of T7 promoter for enhanced yield and quality in mRNA production
- Yustika Sari
- , Sara Sousa Rosa
- & Marco P. C. Marques
Article 09 March 2024 | Open Access
Synthetic receptor scaffolds significantly affect the efficiency of cell fate signals
- Kirato Umene
- & Masahiro Kawahara
Article 29 January 2024 | Open Access
T7 phage-assisted evolution of riboswitches using error-prone replication and dual selection
- Eduardo Goicoechea Serrano
- , Carlos Blázquez-Bondia
- & Alfonso Jaramillo
Article 25 September 2023 | Open Access
Scaling logical density of DNA storage with enzymatically-ligated composite motifs
- , Nimesh Pinnamaneni
- & Raja Appuswamy
Article 20 September 2023 | Open Access
Phenotypic screening of signaling motifs that efficiently induce cell proliferation
- , Teruyuki Nagamune
Article 11 September 2023 | Open Access
Synthetic circuits based on split Cas9 to detect cellular events
- Alicja Przybyszewska-Podstawka
- , Jakub Czapiński
- & Adolfo Rivero-Müller
Article 04 September 2023 | Open Access
A hybrid in silico/in-cell controller for microbial bioprocesses with process-model mismatch
- Tomoki Ohkubo
- , Yuki Soma
- & Katsuyuki Kunida
Article 26 May 2023 | Open Access
Heterologous production of the D-cycloserine intermediate O-acetyl-L-serine in a human type II pulmonary cell model
- Laurel Robbins
- , Ariane Balaram
- & William H. Conrad
Article 27 March 2023 | Open Access
Cloning, characterization, and heterologous expression of a candidate Hirudin gene from the salivary gland transcriptome of Hirudo nipponia
- & Zenghui Lu
Article 10 December 2022 | Open Access
Direct encapsulation of biomolecules in semi-permeable microcapsules produced with double-emulsions
- Grégoire Michielin
- & Sebastian J. Maerkl
Article 27 October 2022 | Open Access
A growth-based platform for detecting domain–peptide interactions in the cytoplasm of mammalian cells
- Yosuke Kimura
- , Daiki Kashima
Article 03 October 2022 | Open Access
Cell-free protein crystallization for nanocrystal structure determination
- Satoshi Abe
- , Junko Tanaka
- & Takafumi Ueno
Article 12 August 2022 | Open Access
Evaluating and mitigating clinical samples matrix effects on TX-TL cell-free performance
- Peter L. Voyvodic
- , Ismael Conejero
- & Jerome Bonnet
Article 07 July 2022 | Open Access
Expanding luciferase reporter systems for cell-free protein expression
- Wakana Sato
- , Melanie Rasmussen
- & Katarzyna P. Adamala
Article 22 June 2022 | Open Access
Negative autoregulation controls size scaling in confined gene expression reactions
- Yusuke T. Maeda
Article 10 June 2022 | Open Access
Design of typical genes for heterologous gene expression
- Dominic Simm
- , Blagovesta Popova
- & Martin Kollmar
Article 07 March 2022 | Open Access
Single 3′-exonuclease-based multifragment DNA assembly method (SENAX)
- Viet Linh Dao
- , Sheena Chan
- & Chueh Loo Poh
Article 05 November 2021 | Open Access
Improved production of the non-native cofactor F 420 in Escherichia coli
- Mihir V. Shah
- , Hadi Nazem-Bokaee
- & Colin Scott
Article 19 August 2021 | Open Access
Rational design of heterodimeric receptors capable of activating target signaling molecules
- Tatphon Kongkrongtong
- , Ruolan Zhang
Article 06 August 2021 | Open Access
Development of robust isothermal RNA amplification assay for lab-free testing of RNA viruses
- Radhika Biyani
- , Kirti Sharma
- & Manish Biyani
Article 21 June 2021 | Open Access
A transgenic female killing system for the genetic control of Drosophila suzukii
- Marc F. Schetelig
- , Jonas Schwirz
- & Ying Yan
Article 28 April 2021 | Open Access
Coiled-coil heterodimers with increased stability for cellular regulation and sensing SARS-CoV-2 spike protein-mediated cell fusion
- Tjaša Plaper
- , Jana Aupič
- & Mojca Benčina
Article 18 March 2021 | Open Access
Site-specific antigen-adjuvant conjugation using cell-free protein synthesis enhances antigen presentation and CD8 + T-cell response
- Adam M. Weiss
- , Jainu Ajit
- & Aaron P. Esser-Kahn
Article 05 March 2021 | Open Access
Random and combinatorial mutagenesis for improved total production of secretory target protein in Escherichia coli
- David Gonzalez-Perez
- , James Ratcliffe
- & Kang Lan Tee
Article 25 January 2021 | Open Access
Engineering lithoheterotrophy in an obligate chemolithoautotrophic Fe(II) oxidizing bacterium
- Abhiney Jain
- & Jeffrey A. Gralnick
Designing P. aeruginosa synthetic phages with reduced genomes
- Diana P. Pires
- , Rodrigo Monteiro
- & Joana Azeredo
Article 21 January 2021 | Open Access
In vitro synthesis of 32 translation-factor proteins from a single template reveals impaired ribosomal processivity
- , David Foschepoth
- & Christophe Danelon
Article 13 January 2021 | Open Access
CRISPR-based transcriptional activation tool for silent genes in filamentous fungi
- László Mózsik
- , Mirthe Hoekzema
- & Arnold J. M. Driessen
Article 25 November 2020 | Open Access
Split drive killer-rescue provides a novel threshold-dependent gene drive
- Matthew P. Edgington
- , Tim Harvey-Samuel
- & Luke Alphey
Article 17 November 2020 | Open Access
Identification of synthetic chemosensitivity genes paired with BRAF for BRAF /MAPK inhibitors
- Kye Hwa Lee
- , Jinmin Goh
- & Kwangsoo Kim
Article 19 October 2020 | Open Access
Quantifying the distribution of protein oligomerization degree reflects cellular information capacity
- Lena Danielli
- , Ximing Li
- & Ramez Daniel
Article 18 September 2020 | Open Access
A novel method for achieving an optimal classification of the proteinogenic amino acids
- , Karel Mácha
- & Stefan Schuster
Article 20 August 2020 | Open Access
Generation of interconnected vesicles in a liposomal cell model
- Baharan Ali Doosti
- , Daniel Fjällborg
- & Tatsiana Lobovkina
Article 19 August 2020 | Open Access
Optimizing bacteriophage engineering through an accelerated evolution platform
- Andrew H. Favor
- , Carlos D. Llanos
- & Jorge A. Bardales
Article 18 August 2020 | Open Access
Rapid repair of human disease-specific single-nucleotide variants by One-SHOT genome editing
- Yuji Yokouchi
- , Shinichi Suzuki
- & Takumi Era
Article 17 August 2020 | Open Access
Plasmid-based complementation of large deletions in Phaeodactylum tricornutum biosynthetic genes generated by Cas9 editing
- Samuel S. Slattery
- , Helen Wang
- & David R. Edgell
Article 07 August 2020 | Open Access
Fully biological production of adipic acid analogs from branched catechols
- Nicholas S. Kruyer
- , Natalia Wauldron
- & Pamela Peralta-Yahya
Article 04 August 2020 | Open Access
Uncovering a possible role of reactive oxygen species in magnetogenetics
- Matthew I. Brier
- , Jordan W. Mundell
- & Jonathan S. Dordick
Article 20 May 2020 | Open Access
Integrative Circuit-Host Modeling of a Genetic Switch in Varying Environments
- Jordan J. Sickle
- , Congjian Ni
- & Ting Lu
Article 08 April 2020 | Open Access
Acclimation of bacterial cell state for high-throughput enzyme engineering using a DmpR-dependent transcriptional activation system
- Kil Koang Kwon
- , Soo-Jin Yeom
- & Seung-Goo Lee
Article 27 February 2020 | Open Access
Epistatic Effect of Regulators to the Adaptive Growth of Escherichia coli
- Yukari Miyake
- & Kaneyoshi Yamamoto
Article 25 February 2020 | Open Access
Rational Design of Photo-Electrochemical Hybrid Devices Based on Graphene and Chlamydomonas reinhardtii Light-Harvesting Proteins
- Martha I. Ortiz-Torres
- , Miguel Fernández-Niño
- & Andrés Fernando González Barrios
Article 27 December 2019 | Open Access
Phenoxyethyl Piperidine/Morpholine Derivatives as PAS and CAS Inhibitors of Cholinesterases: Insights for Future Drug Design
- Yaghoub Pourshojaei
- , Ardavan Abiri
- & Ali Asadipour
Browse broader subjects
- Systems biology
- Molecular engineering
- Chemical biology
Quick links
- Explore articles by subject
- Guide to authors
- Editorial policies

- U.S. Department of Health & Human Services
- National Institutes of Health

En Español | Site Map | Staff Directory | Contact Us
- News & Events
2024 NIH Synthetic Biology Consortium Meeting
Wednesday, november 20, 2024 - 1:00 pm to friday, november 22, 2024 - 5:00 pm, in person online.
Save the date for the annual NIH Synthetic Biology Consortium Meeting. The themes for the 2024 annual meeting include connecting clinical users and synthetic biology tool makers, and identifying clinical needs and research gaps. In addition to the workshop sessions this year's event will include a synthetic biology tool showcase.
Register Meeting Info
6001 Executive Blvd. First Floor Conference Room Rockville , MD 20852 United States
- Frontiers in Bioengineering and Biotechnology
- Synthetic Biology
- Research Topics
Education in Synthetic Biology
Total Downloads
Total Views and Downloads
About this Research Topic
It is about twenty years since Synthetic Biology suddenly emerged in the biotechnology panorama with its strongly innovative features, tools, and goals, and, to date, it already counts a large number of practitioners, several diversified and complementary approaches, dedicated departments, and research ...
Keywords : Education, Synthetic Biology, Early-Stage Researchers
Important Note : All contributions to this Research Topic must be within the scope of the section and journal to which they are submitted, as defined in their mission statements. Frontiers reserves the right to guide an out-of-scope manuscript to a more suitable section or journal at any stage of peer review.
Topic Editors
Topic coordinators, recent articles, submission deadlines.
Manuscript | |
Manuscript Extension |
Participating Journals
Manuscripts can be submitted to this Research Topic via the following journals:
total views
- Demographics
No records found
total views article views downloads topic views
Top countries
Top referring sites, about frontiers research topics.
With their unique mixes of varied contributions from Original Research to Review Articles, Research Topics unify the most influential researchers, the latest key findings and historical advances in a hot research area! Find out more on how to host your own Frontiers Research Topic or contribute to one as an author.
- Publications
- Inventories
What is Synthetic Biology? Good question! It's building as we speak. We'll give you a bird's eye view of what all the excitement and uncertainty is about.
Legislative, management and oversight issues of synthetic biology
What does the public know and think? How is synthetic biology covered in the media?
Ethical issues of synthetic biology research and application
Entrepreneurs and start-up companies working in synthetic biology
Scientists talk sustainability at inaugural synthetic biology symposium

Killer tomatoes, plant-based cement, lab-grown steak and other sustainability-minded projects took center stage at the first-ever Synthetic Biology for Sustainability Symposium in early May. The discussions, held at Schwab Residential Center at Stanford University, featured experts from the Stanford School of Medicine, the School of Engineering and the Doerr School of Sustainability.
“It’s clear that synthetic biology was and is going to play an increasingly important role in how we address the challenges associated with climate change and sustainability,” said Lloyd Minor, dean of the School of Medicine. Researchers are employing synthetic biology — the engineering of organisms’ genetic material — for purposes beyond sustainability as well: Think furniture made from durable fungi or yeast that are genetically modified to create medicine. During her opening remarks, Jennifer Widom, dean of the School of Engineering emphasized a commitment to fostering collaborations between engineers, scientists, as well as social scientists, ethicists, law scholars, and others – to ensure that we can harness the full potential of synthetic biology in a responsible manner. Keynote speaker Jennifer Doudna, a professor at the University of California, Berkeley, who garnered a Nobel Prize in chemistry in 2020, discussed some of her new genetic engineering projects harnessing the gene-editing tool CRISPR. She emphasized that science must be pursued with a social purpose in mind and that it’s crucial for the fruits of scientists to have an impact on real people. Researchers described five high-risk, high-reward projects stemming from new, bold — and somewhat eyebrow-raising — ideas. Some of them focused on developing tools to create new tools; some, on strategies to limit carbon emissions. Still others are devising techniques to make novel foods and useful materials from recycled plastics. Below are brief descriptions of each project: Artificial nitrogen-fixation in plants . Ellen Yeh, MD, PhD, associate professor of pathology and of microbiology and immunology; Jenn Brophy , PhD, assistant professor of bioengineering Plants need nitrogen, but they can’t just siphon it from the air. They can access it only when it’s “fixed” — in a form such as ammonium nitrate that they can absorb through their roots. The common solution is to use nitrogen-laden fertilizers, but excess nitrogen from fertilizers can make its way to the ocean, spurring algal growth, whose decomposition depletes the oxygen supply used by marine life, leading to dead zones. Yeh and Brophy have engineered plant organelles, a structure that lives inside a cell, that harbor a nitrogen-fixing bacteria, allowing a plant to create its own useable nitrogen and decreasing the need for fertilizers. Mussel-inspired binders for concrete. Michael Lepech , PhD, professor of civil and environmental engineering; Possu Huang , PhD, assistant professor of bioengineering Try to pry a mussel off a rock with your bare hands — it’s not easy. Scientists are taking inspiration from this bivalve’s natural super glue, composed of protein-laden stringy fibers, to create a more eco-friendly version of a key component of concrete, one of the world’s most common construction materials. Concrete is essentially a concoction of water, sand, gravel and something known as a binder. The binder, often composed of limestone and clay, requires a lot of resources to create, and is, sustainably speaking, problematic. Its production accounts for 4.5% of all greenhouse gases and 7% of CO2 emissions. Lepech and Huang are harnessing synthetic biology to build a sort of molecular “glue” using proteins and minerals. Weed-killing tomatoes. Jenn Brophy, PhD, assistant professor of bioengineering; Beth Sattely, PhD, assistant professor of chemical engineering What if we could turn the docile tomato plant we know and love into a master of self-defense? Brophy and Sattely hope to imbue the plant, the growth of which can be stunted by competing weeds, with a new power: herbicide secretion. Their goal is to create a tomato plant that secretes something called momilactone, a chemical that’s been shown to subdue weed growth. The idea is that the plant would selectively secrete the herbicide at the tips of the roots without compromising its fruit. Converting plastic waste into components of palm oil. Matteo Cargnello , PhD, associate professor of chemical engineering; Jennifer Cochran , PhD, professor of bioengineering Even if we recycle plastics, they pose a problem. A plastic water bottle doesn’t turn into another plastic water bottle — it degrades too much. And discarded plastic often ends up polluting the ocean. The researchers dream of something called chemical recycling, in which discarded items, such as plastic, are broken down into their component parts, which are then used to build something new. Cochran, Cargnello and their team plan to use chemicals to break down recycled plastic and convert it into palm oil. As manufacturers of palm oil raze forests to plant palm trees and plastics amass in oceans, such a process could protect the environment on both fronts. Lab meat . Helen Blau, PhD professor of microbiology and immunology; Sarah Heilshorn , PhD, professor of materials science and engineering Of all the meats, beef takes the biggest toll on the environment, yet global beef consumption is on the rise. That’s why Blau and Heilshorn are embarking on a new project in their lab: growing a steak from the cells of a cow. This new approach to satiating carnivores is known as lab-cultured meat, or cellular agriculture. The researchers’ aim is to sustainably create actual meat, rather than plant-based meat substitutes. Others have tried to grow beef in the lab, but it’s yielded food items that are more akin to ground beef than a slab of steak. So, how do you take a beef-ish paste and turn it into a filet? Scaffolding. The team is turning to 3D printing using biological materials to create a structure for the cultured cells to grow in a way that recapitulates genuine meat. To close out the symposium, Stanford University president Marc Tessier-Lavigne shared his vision for how researchers across campus will usher in a new era of discovery that’s rooted in synthetic biology. He highlighted three key components: deepening human knowledge, enabling interdisciplinary teams and translating research discoveries into real-world solutions. “There’s an expression I like to use: ‘No one can whistle a symphony. It takes a whole orchestra to play it,’” he said. “As I think about the sustainability challenges we face and the solutions they will require, this has never felt truer.”
Related Departments

The future of Russia and Ukraine

Turning carbon pollution into ethanol

New gels could protect buildings during wildfires

IMAGES
VIDEO
COMMENTS
Synthetic biology articles from across Nature Portfolio Synthetic biology is the design and construction of new biological parts, devices, and systems, and the re-design of existing, natural ...
Synthetic biology will transform how we grow food, what we eat, and where we source materials and medicines. Here I have selected six products that are now on the market, highlighting the ...
Synthetic biology is among the most hyped research topics this century, and in 2010 it entered its teenage years. But rather than these being a problematic time, we've seen synthetic biology ...
Despite the efforts by individual countries to establish synthetic biology research roadmaps, broader, international agreement on common standards (and red lines) across the field may help establish trust and to advance the best pre-competitive research into useful applications.
In this study, we employ topic modeling to comprehensively map research topics within synthetic biology, revealing subtopics and their relationships, as well as trends over time. We utilize metadata to identify the most significant journals and countries in the field and discuss potential policy impact on the research output. In addition, we ...
Synthetic biology has applications in healthcare, industry, and the environment. Several disciplines use the synthetic biology approach, including metabolic engineering, minimal genomes, regulatory circuits, and orthogonal biosystems, and ongoing research is essential in all disciplines to test new ideas and develop new products and research tools.
Currently, several virtual special issues are open for submissions, including Quantitative Synthetic Biology, Materials Design by Synthetic Biology, Mammalian Cell Synthetic Biology, IWBDA 2023, and Diagnostics, and we are selecting additional topics for upcoming issues.
This Research Topic is the 2022 collection of the 'Insights In' series. The previous collection, which has attracted 71 authors can be found here: Insights in Synthetic Biology 2021: Novel Developments, Current Challenges, and Future Perspectives.We are now entering the third decade of the 21st century, and, especially in the last years, the achievements made by scientists have been ...
Believe or not, AI is revolutionary and reshaping our daily lives. In the general Biology research field, Synthetic Biology is considered a revolution [ 8, 9 ]. Here, we are using ChatGPT to explore Synthetic Biology, and discover its research trends in the next decade. To see how cutting-edge AI can help practitioners of Synthetic Biology.
Today, with the renaissance of cell-free biotechnology, more and more scientists are entering this field to explore the frontiers of synthetic biology. To highlight the current progress of cell-free research, this Research Topic aims to bring together diverse and exciting achievements in cell-free synthetic biology.
Synthetic biology is a broad field with a unifying theme of learning through building. At IUPAB2024 in Kyoto, Japan, five researchers gave lectures focusing on in vitro synthetic biology, where the built objects were not living cells but in vitro systems composed of biomolecules. The talks sparked lively discussions on the knowledge gained about living cells from in vitro reconstructions.
Funded by a 10-year grant from the National Science Foundation (NSF), the Synthetic Biology Engineering Research Center (SynBERC) is founded to advance research, innovation, training, and education in synthetic biology.
Introduces new insights into new systems and engineering formalisms for the design and construction of synthetic biological parts and devices.
NIST researcher Elizabeth Strychalski's research group is helping to establish the measurements and standards needed for progress in engineering biology, also known as synthetic biology.
Synthetic biology is considered as an emerging research field that will bring new opportunities to biotechnology. There has been long time that synthetic biology was tinkered with the idea of creating "made to order" microbes from well-characterized biological parts.
The broad range of applications offered by synthetic biology and bioengineering has revolutionized the ability to design and redesign microorganisms to express specific functions, overcoming the limitations of natural biological systems. This advancement has been achieved through the use of mathematical models and genetic circuits, enabling the precise design of synthetic microbial communities.
The Stanford Synthetic Biology community enables interdisciplinary activities, supporting an ecosystem of research and learning. Our holistic approach encompasses diverse areas of work, each exploring fundamental questions and possibilities with the ultimate desire of addressing societal needs.
Synthetic biology is a promising field for medical and pharmaceutical applications. This review covers the latest advances and challenges in this area.
Synthetic biology requires students and scientists to draw upon knowledge and expertise from many disciplines. While this diversity is one of the field's primary strengths, it also makes it challenging for newcomers to acquire the background knowledge necessary to thrive. To address this gap, we developed a course that provides a structured ...
Synthetic biology is a new discipline that has recently emerged in close connection with systems biology. The ambition of synthetic biologists is to operate as engineers, and to introduce into organisms (in most cases, microorganisms) new functional modules that will allow these organisms to accomplish new tasks.
A collection of TED Talks (and more) on the topic of Synthetic biology.
e. Synthetic biology (SynBio) is a multidisciplinary field of science that focuses on living systems and organisms, and it applies engineering principles to develop new biological parts, devices, and systems or to redesign existing systems found in nature. [1]
Synthetic Biology. Purified motor proteins organise microtubules in micrometre-sized droplets, mimicking the organization of the microtubule cytoskeleton in cells. From Thomas Surrey's Microtubule Cytoskeleton Architecture and Dynamics Laboratory. Synthetic biology brings together knowledge of biological processes with engineering principles to ...
Synthetic Biology Synthetic biology is a field of science that involves redesigning organisms for useful purposes by engineering them to have new abilities. Synthetic biology researchers and companies around the world are harnessing the power of nature to solve problems in medicine, manufacturing and agriculture.
Read the latest Research articles in Synthetic biology from Scientific Reports
Save the date for the annual NIH Synthetic Biology Consortium Meeting. The themes for the 2024 annual meeting include connecting clinical users and synthetic biology tool makers, and identifying clinical needs and research gaps. In addition to the workshop sessions this year's event will include a synthetic biology tool showcase.
In this Research Topic, we would like to discuss topics related to Synthetic Biology Education referred to people of all ages and backgrounds, from graduate students to Ph.D. students, from young to senior researchers interested in this field. This topic will act as a resource for all actors that find themselves at ease in the wide Synthetic ...
Synthetic biology, once hailed as a moneymaker, meets tough times Three major startups have faltered over the past year, but smaller firms are finding a niche. 22 Aug 2024; ... and research, free to your inbox daily. Subscribe. ScienceInsider. 29 Aug 2024 By . Jon Cohen; New, scientist-run virus database vows to be transparently run and simple ...
Topics Ethics. Ethical issues of synthetic biology research and application. Topics Metaphors Project. Topics Entrepreneurs. Entrepreneurs and start-up companies working in synthetic biology. Ensuring benefits of synthetic biology are realized through responsible development. Synthetic biology specific news, events, publications and more.
Scientists gathered to discuss the future of synthetic biology and how it can help curb climate change and promote sustainability. ... Summer Undergraduate Research Fellowship (SURF) Meet our staff; ... Topics Future of Everything. Spotlights. Media Coverage. Browse News by Topic.