Research & Innovation Office
- About the Research & Innovation Office
- Vice President for Research & Innovation
- RIO Leadership Team
- E4 by Design
- UMN Research Statistics
- Administrative Support Units
- Academic Centers & Institutes
- Council of Research Associate Deans (CRAD)
- Community Oversight Board
- Research Integrity & Safety Collaborative (RISC)
- Research Facilities & Expertise
- Research Tools & Applications
- The Office of Research Information Systems
- ORIS Staff List
- Research Animal Resources
- About BSL-3
- Frequently Asked Questions
- BSL-3/ABSL-3 Research Laboratory Suites
- Related Resources
- Contact BSL-3
- International Research Support & Guidance
- Disclosing Foreign Support to Federal Science Agencies
- Foreign Talent Recruitment Programs
- Hosting International Visitors, Scholars & Researchers
- Traveling Abroad
- International Institutional Agreements
- Export Controls & Sanctions Compliance
- Helpful Resources & Contacts
- About Artist-in-Residence Awards
- Award Recipients
- About Biotechnology & Biomanufacturing Seed Grants
- BBIC Awardees
- About Grant-in-Aid
- Exemplary Proposals
- Awarded Equipment
- NC A&T & UMN Research Partnership
- About the Program
- Past Awards: 2024
- Past Awards: 2023
- Past Awards: 2022
- Past Awards: 2021
- Past Awards: 2020
- Past Awards: 2019
- Past Awards: 2018
- Past Awards: 2017
- Past Awards: 2016
- Past Awards: 2015
- Past Awards: 2014
- Past Awards: 2013
- Past Awards: 2011
- About Social Justice Impact Grants
- SJIG Awardees
- Sustainable GeoCommunities Seed Grants
- About the Innovation Impact Case Award
- 2022 Awardees
- 2023 Awardees
- 2024 Awardees
- Matching Funds
- Research Technical Staff Award
- MnDRIVE Funding
- Research Computing Funding
- COVID-19 Rapid Response Grants
- About the International Institute for Biosensing
- About Minnesota Futures
- Minnesota Futures: Past Awards
- Review Committee
- U of M Internal Funding
- Limited Extramural Funding
- Proposals & Awards (SPA)
- F&A Costs, Effort Reporting
- Research Integrity & Compliance (RIC)
- Human Research Protection
- Institutional Review Board (IRB)
- Animal Care & Use (IACUC)
- Biotechnology Activities Oversight (OBAO)
- Export Controls
- Conflict of Interest
- Reporting Research Misconduct
- Research Compliance Training Guide
- Research Ethics Week
- Partnerships for Corporations & Industry
- Building Successful Industry Partnerships
- Research Agreements
- Confidentiality Agreements
- Starting a Company (Venture Center)
- Technology Commercialization
- About MnDrive
- Louis Stokes North Star STEM Alliance/MnDRIVE Partnership
- Transdisciplinary Research Program
- News & Announcements
- All Past News & Announcements
- UMN Research Newsletter
- UMN Washington Update

Earthworm Study Opens New Dimension in Climate Science
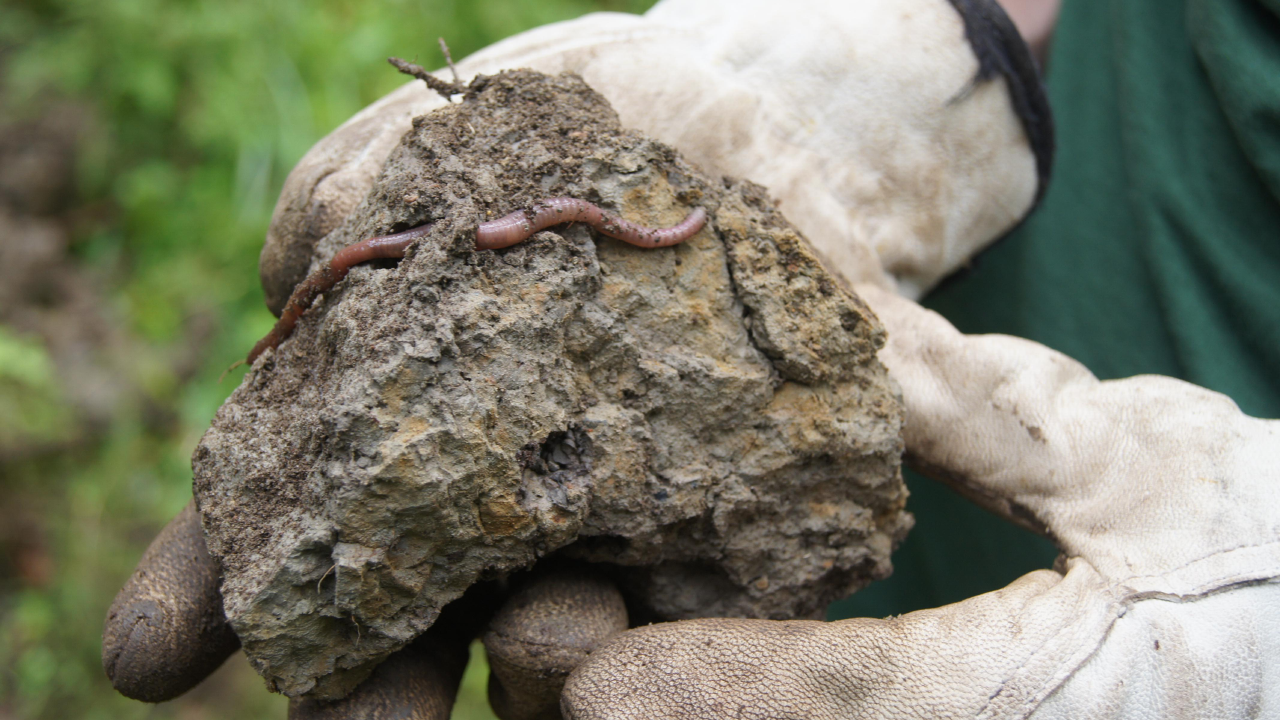
As the world waxes warmer, scientists are scrambling to prioritize conservation efforts.
A new global study of earthworm diversity suggests looking at soil, as climate change could seriously affect earthworms and the many functions they provide.
Based on data compiled from 6928 sites in 57 countries—including over 100 sites sampled by University of Minnesota researcher and study coauthor Adrian Wackett—the work reveals that climate variables—temperature and especially precipitation—influence earthworm distributions and diversity far more than the properties of the soils they inhabit.
Earthworms exert major controls over how soils function; along with other soil fauna, they regulate water and nutrient flows at the base of ecosystems. Conservation efforts that neglect them and focus solely on aboveground species could backfire and harm aboveground species dependent on soils for food and shelter.
To avoid this pitfall, “it’s helpful to have some grasp on where soil fauna like earthworms are most abundant, and the primary environmental factors driving their distributions,” said Wackett, a researcher in the Department of Soil, Water, and Climate (SWAC) in the College of Food, Agricultural, and Natural Resource Sciences.
The study, led by Helen Phillips, PhD, at the German Centre for Integrative Biodiversity Research in the Institute of Biology at Leipzig University, was a major international undertaking involving more than 100 scientists. It appears in the journal Science .
Digging the Data
In formerly glaciated lands such as northern North America, Scandinavia, and Finland, many sites contain diverse non-native earthworm communities introduced by European settlers after glaciers had wiped out native earthworm populations in the last ice age.
Wackett’s work, in the arctic and boreal areas of Sweden and Finland, bolstered the research group’s formerly sparse data from high latitudes. Modeling earthworms’ responses to several variables, the research team found that globally, the amount of precipitation most strongly affected the number of earthworm species, the number of individuals (i.e., earthworm abundance), and their biomass (total weight). Earthworm abundance also responded strongly to temperature and soil water retention. Non-climate-related factors—e.g., habitat cover, elevation, and even soil properties—wielded far less influence. Thus, climate change is predicted to severely affect earthworms and the numerous functions they perform within ecosystems.
“Earthworms are king underground. They’re the biggest builders and most voracious consumers in soils,” said Professor Kyungsoo Yoo, PhD, of SWAC, who was not involved in the study. “Their responses to climate will be different from aboveground dwellers. This study helps us understand these crucially important animals.”
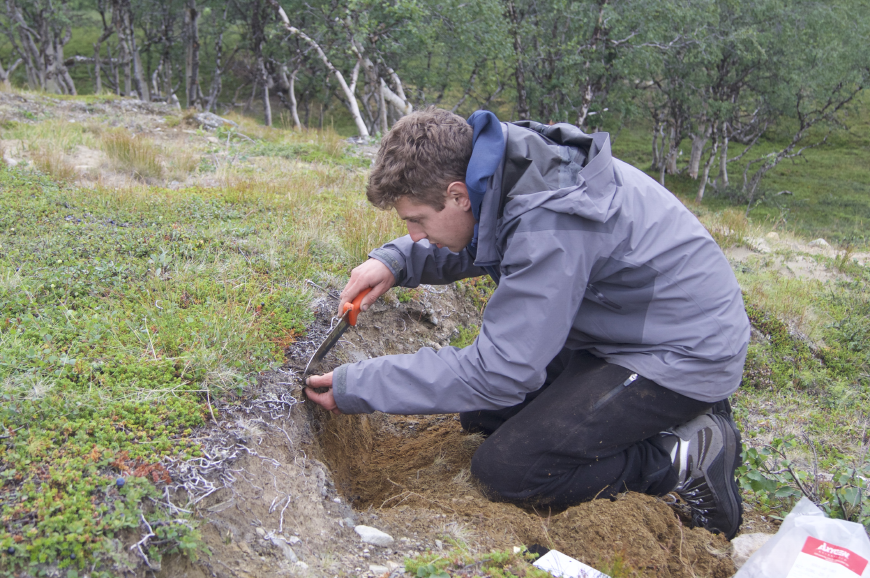
What’s at Stake
It has long been thought, said Wackett, that earthworm distributions are governed by where soils are most fertile; e.g., with suitable acidity, texture, and availability of calcium, nitrogen, and other essential nutrients. But he wasn’t shocked that these properties turned out to be of comparably less importance.
“I’ve seen some invasive earthworms living at acidities close to vinegar,” he said. “I view earthworms as regulating and enriching soils more than vice versa.”
For example, earthworms make excellent “bioreactors for nutrient recycling,” Wackett said. In their guts, they catalyze the decomposition of plant litter and unlock vital nutrients trapped in the soil matrix, then excrete those nutrients back into the soil. This turns “raw,” inaccessible elements like phosphorus, nitrogen, and calcium into plant-available forms that nourish plant and animal life aboveground. This recycling system is particularly vital in tropical ecosystems, where many soils are nutrient-poor.
But in high-latitude places like northern Minnesota, when earthworms eat leaf litter and move nutrients deeper into the soil, it can have adverse effects. Many native forest plants, like lady slippers and ferns, evolved with shallow roots to mine nutrients from the litter layers that accumulate in formerly glaciated forests without earthworms. When feeding by invasive earthworms thins these litter layers, many native forest plants are starved of nutrients, while native tree saplings are left unprotected against browsing by hungry deer. Eventually, this combination wipes out the entire forest understory, creating openings for invasive species like buckthorn.
Enter Climate Change
Earthworms’ responses to climate change can have positive or negative effects on a variety of other species.
Northern areas are getting warmer and wetter, and in Alaska’s Kenai Peninsula, spring snow melt washes newly introduced earthworms into salmon streams, providing extra protein for the fish. However, similar to Minnesota, invasive earthworms could harm native Alaskan forest plants and prevent litter pileup on the forest floor, leading to bank erosion, increased sedimentation, and polluted salmon streams.
In parts of the tropics, said Wackett, the “dry” season is getting hotter and drier, and it’s unclear whether earthworms—which generally prefer moist conditions—can tolerate these changes. If not, their ecosystem services may be lost and tropical forests would suffer.
“It remains unclear exactly how ‘nature’s gardeners’ will respond to future environmental change, but given earthworms’ profound ecological reach, a better understanding of their subterranean world and how they interact with it is a crucial first step,” Wackett said.
- Research article
- Open access
- Published: 07 May 2020
Soil properties changes earthworm diversity indices in different agro-ecosystem
- Sharanpreet Singh ORCID: orcid.org/0000-0003-1740-0054 1 ,
- Ayushi Sharma 2 ,
- Kiran Khajuria 2 ,
- Jaswinder Singh ORCID: orcid.org/0000-0003-3178-0516 2 &
- Adarsh Pal Vig 1
BMC Ecology volume 20 , Article number: 27 ( 2020 ) Cite this article
14k Accesses
45 Citations
Metrics details
Earthworm communities are generally very sensitive to physico-chemical properties of the soil in different agro-ecosystem i.e. cultivated or non-cultivated which directly or indirectly influence the earthworm survival. The difference in physico-chemical properties of soil at different sites contributed to the formation of population patches for earthworm species. Understanding the physico-chemical properties of soil at a particular site could facilitate the prediction of earthworm species at that site. The objective of the present study was to investigate the diversity, abundance, and distribution of earthworms in cultivated and non-cultivated agroecosystems and their physico-chemical properties affecting the earthworm diversity and abundance.
Total 10 species of earthworms i.e. Amynthas alexandri, Amynthas morrisi, Eutyphoeus incommodus, Eutyphoeus waltoni, Metaphire birmanica, Metaphire houlleti, Metaphire posthuma, Octochaetona beatrix, Perionyx excavatus, and Polypheretima elongata, were reported. Out of all the reported species, Metaphire posthuma was found to be the most abundant earthworm species in both cultivated and non-cultivated agroecosystems with the occurrence at 56.81% sites. The Shannon-Wiener index (H), Margalef species richness index (D Mg ) and Pielou species evenness (E) was ranged from 0 to 0.86, 0 to 0.64 and 0.78 to 1 respectively. The principal component analysis resulted in four principal components i.e. PC1, PC2, PC3 and PC4 which contributing variance (%) of 22.96, 19.37, 14.23 and 10.10 respectively. The principal component analysis also showed that physico-chemical parameters of soil such as EC, pH, TDS, texture, OC, moisture, etc. play a critical role in earthworm distribution.
The conventional farming system has a negative effect on the earthworm diversity in the soil while the physico-chemical properties of soil also have a determinant effect on the same. Earthworms abundance in the present study have significant direct relation with soil properties at a particular site and vice versa. The diversity indices also change due to the conventional farming system which directly affects the earthworm abundance.
In agro-ecosystems, earthworm communities are generally very sensitive to physico-chemical properties of the soil which directly or indirectly influence the availability of resources for earthworm survival [ 1 ]. The soil structure and its pore size also play a key role in the distribution of the earthworms within the soil and a minute changes in the same can adversely affect the earthworm’s community structure [ 2 ]. The richness and diversity of earthworm species were always observed higher in the undisturbed land as compared to disturbed land and likely to increase under favorable soil conditions [ 3 , 4 ]. In undisturbed land, soil characteristics such as soil quality [ 5 ]; pH [ 6 ]; moisture [ 7 ], as well as soil organic matter [ 8 ], affect the diversity and abundance of earthworm’s species. On the other hand, disturbed land such as the agricultural field, application of fertilizer [ 9 ]; pesticides [ 10 ]; tillage [ 11 ] and soil organization [ 12 ] have a strongest effect on earthworm distribution.
Earthworms act as an ecosystem engineer by modifying soil structure and its properties [ 13 , 14 , 15 ]. It also change the porosity of the soil by regulating the proportion of organic matter breakdown and nutrient release [ 16 ]. The difference in physico-chemical properties of soil at different sites contributed to the formation of population patches for earthworm species. By knowing the physico-chemical properties of soil at a particular site could facilitate the prediction of earthworm species at that site [ 17 ]. It is also important to quantify the spatial distribution of earthworms at different agroecosystems in order to understand the effect of abiotic soil processes and to link earthworm abundance to the spatial distribution of macropores in the soil. The information collected on type of earthworm species and their abundance at different habitats may also provide useful information on the efficiency and strength of that ecosystem. Besides the soil physico-chemical properties, the types of the agro-ecosystem selected at various site i.e. cultivated or non-cultivated are also play an important role in earthworms abundance and distribution.
The objective of the present study was to investigate the diversity, abundance, and distribution of earthworms in cultivated and non-cultivated agroecosystems and their physico-chemical properties affecting the earthworm diversity and abundance.
Earthworm communities and their diversity indices
We have explored the 44 different sites under cultivated and non-cultivated agroecosystem (Additional file 1 : Table S1) under which total 10 species of the earthworms viz. Amynthas alexandri, Amynthas morrisi, Eutyphoeus incommodus, Eutyphoeus waltoni, Metaphire birmanica, Metaphire houlleti, Metaphire posthuma, Octochaetona beatrix, Perionyx excavatus and Polypheretima elongata belonging to two families and six genera were reported. Out of these, 7 species belong to family Megascolecidae ( M. posthuma, A. morrisi, A. alexandri, M. houlleti, Polypheretima. elongata, Perionyx excavatus and M. birmanica) and three species belong to family Octochaetidae ( O. beatrix , E. waltoni and E. incommodus ). The distribution of above said species along with their ecological category and diversity indices at each sampling site is given in Table 1 . The non-cultivated agroecosystem has high earthworm abundance as compared to cultivated agroecosystem (Fig. 1 a). The cultivated and non-cultivated agroecosystem has 5 and 10 earthworm species respectively. The abundance pattern in cultivated and non-cultivated agro-ecosystem was in the order of M. posthuma > E. waltoni > M. houlleti > O. beatrix > A. alexandri and M. posthuma > O. beatrix > M. houlleti > E. waltoni > E. incommodus > A. morrisi > A. alexandri > M. birmanica > Polypheretima elongata > Perionyx excavatus respectively (Fig. 1 b). Out of all the reported species, M. posthuma was found to be the most abundant earthworm species in both cultivated as well non-cultivated agroecosystems with the occurrence at 56.81% sites. The Shannon-Wiener diversity index (H), Margalef species richness index (D Mg ) and Pielou species evenness (E) were ranged from 0 to 0.86, 0 to 0.64 and 0.78 to 1 respectively (Table 1 ). The different values of Shannon-Wiener diversity, species richness and species evenness index for the same number of earthworm individual at few sites were due to the difference in the number of earthworm species.
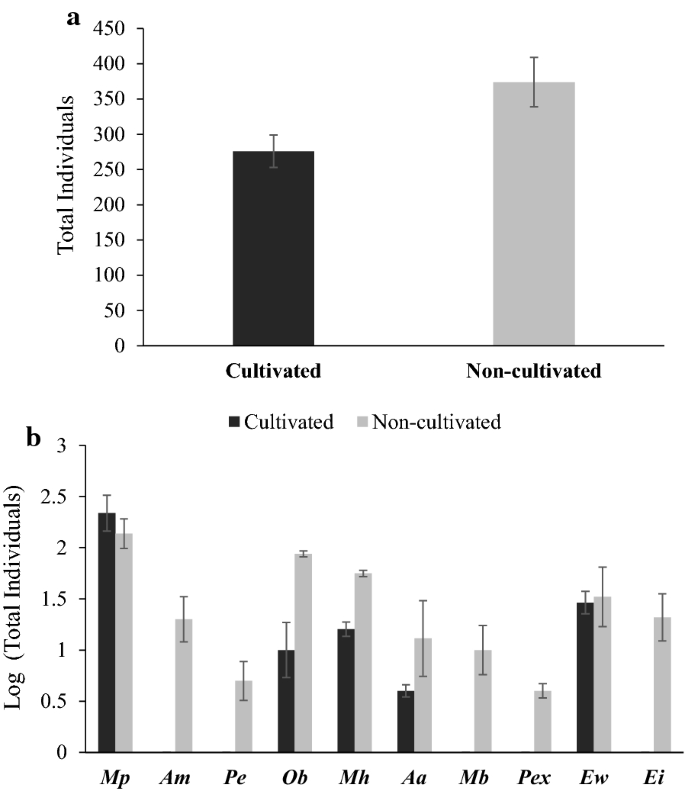
The total number of individuals sampled ( a ) and total individuals sampled (log transformed) in each earthworm species ( b ) in cultivated and non-cultivated sampling sites
Relation between physico-chemical properties of soil and earthworm abundance
The physico-chemical analysis of soils of all sampling sites is given in Table 2 . The texture of soil was found to be loam to sandy loam at maximum sites with sand (%), silt (%) and clay (%) were in the range of 43.28–81.47, 5.34–44.21 and 6.54–27.61 respectively. The moisture content of the soil at each sampling site was more than 45%. The earthworm species were distributed in the soil moisture range from 48–97%, pH from 5.96–8.65, EC from 27.3–897.5 µS, TDS from 27.45 to 166.5 mg/L, OC from 1.01–9.53%, N from 0.12–2.68 g/Kg, P from 0.06–0.26 g/Kg, K from 0.97 to 7.45 g/Kg, Ca from 1.18–107.34 g/Kg and Na from 0.44 to 1.45 g/Kg.
The distribution and abundance of 8 earthworm species w.r.t. range of physico-chemical properties of soil at different sampling sites is given in Table 3 . The range of physico-chemical properties of soil for Polypheretima elongata, and Perionyx excavatus is not given due to their presence at a single site only. The M. posthuma, A. morrisi, A. alexandri, E. incommodus and M. birmanica were present in slightly alkaline soil while E. waltoni, M. houlleti, and O. beatrix were present from slightly acidic to slightly alkaline soil. The organic carbon (%) range for M. posthuma (OC from 1.45 to 9.53) was high as compared to other species. High range of N (0.12–2.68 g/Kg) and P (0.06–0.26 g/Kg) content was also observed at M. posthuma sampling sites which is due to the use of nitrogen and phosphorus in the form of fertilizers in the cultivated fields.
Impact of soil properties on earthworm abundance
The Principal component analysis (PCA) was used on 13 different variables of soil for 44 different sites to study the influence of soil properties on the distribution and abundance of earthworm species. The PCA analysis gives four different principal components (PC) i.e. PC1, PC2, PC3 and PC4 which causing variance (%) of 22.96, 19.37, 14.23 and 10.10 respectively (Table 4 ). As indicated by Liu et al. [ 18 ], the factors were considered weak, moderate and strong when absolute loading values were < 0.50, 0.50–0.75 and > 0.75 respectively. The variance in PC1 is due to pH, EC, TDS, and K; in PC2 is due to sand, clay and silt; in PC3 is due to Moisture and OC; in PC4 is due to Ca, Na, P and N. The first two components of the PCA i.e. PC1 (22.96%) and PC2 (19.37%) of physico-chemical variables account for 42.33% of total variance with positive strong loading of pH, EC, TDS and K in PC1 while strong negative loading of clay and strong positive loading of silt and sand in PC2 (Fig. 2 a). On the other hand, PC1 (22.96%) and PC3 (14.23%) account for 37.19% of total variance with strong positive loading of OC and strong negative loading of moisture in PC3 (Fig. 2 b). The principal component PC4 accounts for the variance of 10.10% with positive loading of Ca, N, Na & P (Fig. 2 c). The earthworm abundance and soil properties have significant direct relation (PERMANOVA, F = 22.1, P < 0.05; Mantel test, R = 0.14, P < 0.05) and soil properties also favours the earthworm abundance at a particular site and vice versa.
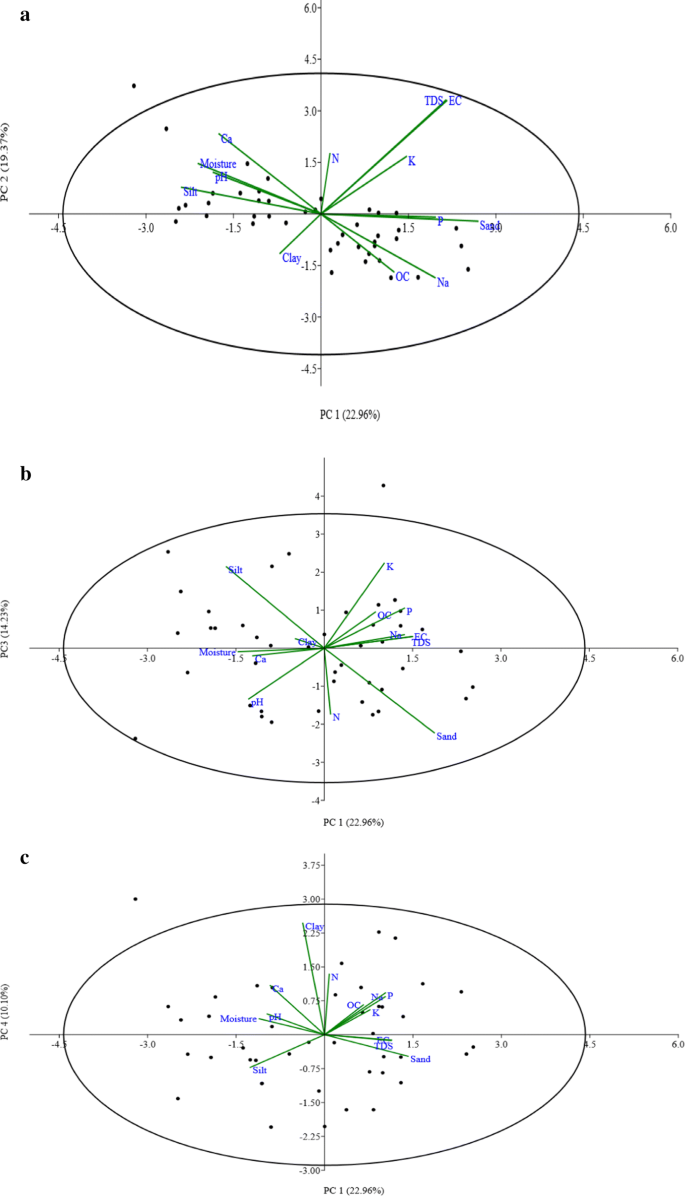
Biplot of PCA of 13 different physico-chemical properties of soil, a PC1 vs PC2; b PC1 vs PC3; c PC1 vs PC4. The PC1, PC2, PC3 and PC4 explain 22.96%, 19.37%, 14.23% and 10.10% of variance respectively in datasets. The components with variance less than 10% were excluded. The Varimax rotation with Kaiser Normalization method was used for the extraction of principal components
Effect of land use pattern on earthworm diversity
The abiotic factors, vegetation type and physico-chemical properties of soil determined the abundance of earthworms [ 1 ]. Metaphire posthuma was observed at maximum sampling sites including both cultivated and non-cultivated while Polypheretima elongata and Perionyx excavatus were restricted to a single site, least abundant and reported from non-cultivated sites. Thus , M. posthuma was found to be the most stable and adapted earthworm species in cultivated land use which corroborated with the findings of Singh et al. [ 1 ] who also observed the same results. The high abundance of M. posthuma at cultivated sites might be due to their endogeic ecological nature [ 1 ]. Mariotte et al. [ 19 ] also reported that earthworm species with endogeic ecological category were least affected as compare to epigeic and anecic earthworm species. On the other hand, ploughing damages the burrows of earthworm in the soil which directly disturbs anecic and epigeic earthworm species as compared to species with the endogeic ecological category. This might be the reason for the low abundance and diversity of anecic and epigeic species in agricultural fields as compared to gardens and nurseries [ 20 ]. On the other hand, in our study other endogeic earthworm species viz M. houlleti, O. beatrix, M. birmanica and Polypheretima elongata were also reported but their abundance is much less in cultivated fields as compare to non-cultivated fields. The reason for this may be due to agricultural practices. The more abundance of earthworm species was observed at margins of the paddy fields but no earthworm was reported inside fields because paddy cultivation requires intensive ploughing and water. This might be the reason for the abundance of earthworm at field margins. Thus, our study is corroborated with Frazão et al. [ 3 ] and van Schaik et al. [ 17 ] who also observed high density and species richness of earthworm in field margins as compared to the fields having wheat cultivation.
In the present study, the earthworm diversity indices were changed as the agro-ecosystem changed from cultivated to non-cultivated pattern. The highest values of diversity indices were observed in non-cultivated sites as compared to cultivated sites which were due to the availability of only one species of earthworm i.e. M. posthuma in cultivated sites as compare to non-cultivated sites. Solomou et al., [ 21 ] also observed that the diversity indices change from non-cultivated to cultivated agro-ecosystem. The agricultural management practices such as deep ploughing, fertilizer and pesticide application directly affect the earthworm species present within the soil which usually affects the diversity indices. Bartz et al. [ 22 ] also observed that minimum ploughing has greater earthworm richness as compared to the site with conventional tillage. Margerie et al. [ 23 ] and Goswami [ 24 ] also supported our observation that diversity indices usually change from one sampling site to another due to changes in the habitat.
Soil variables and earthworm abundance
The physico-chemical properties of soil directly affect the earthworm abundance and hence diversity indices. In the present study, high contents of N, P and TDS were reported in the cultivated fields and these cultivated fields have also less earthworm diversity with restriction to single earthworm species. We have also observed that sites having the application of cattle dung as organic manure like in gardens have more earthworm species than agriculture land. The input of organic manures and non-conventional farming system also improves soil quality which promotes earthworm presence within the soil [ 21 , 25 ]. Singh et al. [ 26 ] also reported that the use of sheep dung in the intensive grassland management increase the earthworm population and their abundance and number increase up to four times which directly affected the earthworm diversity indices [ 27 ]. The present study also reported that agricultural management practices such as ploughing, tillage etc. also has an effect on the abundance of earthworms at a particular site.
The principal component analysis was also applied to the 13 different physico-chemcial variables which resulted in total of 4 principal components (PC) which explained 66.67% of the total variance. The PC1 explained 22.96% of total variance which was due to pH, EC, TDS and K. This validates the outcomes of Sanchez et al. [ 28 ] which explained that earthworm prefers soil having salt concentrations. The pH at a particular site is also an important factor for earthworm distribution as earthworms can survive only in neutral but also in slightly acidic to slightly alkaline soil conditions. Soil pH did not directly affect the abundance of earthworms at a particular site but indirectly pH drives other chemical processes in earthworms which affect nutrient availability [ 29 ]. In the present study, the pH of the soil varied from 5.96 to 8.6. The earthworm’s species respond very quickly to variations in pH at a particular site and they are generally avoiding soil having pH values less than 4.5, favoring pH between 5.0 and 7.4 [ 30 ]. McCallum et al. [ 31 ] also observed that diversity and abundance of earthworms are very low in soil having a pH near 4.5. Most of the studies reported that earthworms can tolerate a pH range of 5.0 to 8.0 and an abundance of earthworms increase as pH was shifted from acidic or basic to neutral. De Wandeler et al. [ 16 ] also observed that the earthworm’s abundance and diversity in soil increased with an increase in pH from acidic to neutral and maximum earthworm abundance was found near pH 7. The EC also plays a vital role in earthworm metabolism [ 32 ]. Thus, PC1 explained chemical factors for earthworm’s distributions. The PC2 explained 19.37% of total variance respectively which was due to sand, silt and clay. Yvan et al. [ 33 ] described that soil texture also influences the activity and growth of earthworm. Higher clay content in soil favours growth and abundance of earthworm’s species [ 34 ] which is clearly represented in our study. Thus, PC2 explained the soil texture factor. The PC3 explained 14.23% of total variance which was due to OC and moisture. According to Chan and Barchia [ 35 ], organic carbon is the critical factor for the earthworm distribution as it helps in determines the type and nature of food for an earthworm. Bartz et al. [ 22 ] and Jänsch et al. [ 36 ] also observed a significant relationship between soil OC and earthworm abundance. They observed that sites with high earthworm abundance have high soil OC content and vice versa. The presence of leaf litter in the soil also favors the earthworm population due to the easy availability of organic matter [ 37 ]. The Moisture is a critical factor for earthworm distribution due to the cutaneous mode of respiration [ 38 ]. Walsh and Johnson-Maynard [ 39 ] reported that earthworms were absent from the driest sites and their high density and biomass depend on local conditions like soil properties and management. Talavera et al. [ 40 ] have reported that both moisture and OC are the key factors for the prediction of earthworm communities at a particular site. Thus, PC3 explains the growth factor. The PC4 explained 10.10% of variance which was due to N, Ca, Na and P; which explain the edaphic factors for earthworm’s distributions. Thus, PCA explained that earthworm communities and their diversity indices are directly correlated with the physico-chemical characteristics of soil at the particular site.
This study provides information about the pattern of earthworm diversity in cultivated and non-cultivated fields and how soil physico-chemical properties affect the earthworm diversity indices in different agro-ecosystem. It was concluded that cultivated fields having less earthworm diversity as compared to non-cultivated fields. The M. posthuma was observed at maximum sampling sites and found in all types of vegetation especially in the cultivated fields having paddy plantation while others endogeic earthworm species were absent in paddy plantation. The change in physico-chemical properties of soil also alters the earthworm diversity indices. The principal component analysis also showed that the physico-chemical properties of soil play a critical role in earthworm distribution. The diversity indices also change due to the conventional farming system which directly affects the earthworm abundance. The farmers should be aware of the roles of earthworms in soil and also must be encouraged to shift their agricultural practices from conventional to organic. These practices not only increase earthworm diversity and abundance but also helps to maintain soil enriched with various types of major and macro-nutrients.
The earthworm sampling was done during two consecutive years in monsoon and post-monsoon seasons of the year 2015 and 2016 in the district Kathua (Jammu & Kashmir, India) (Fig. 3 ). This monsoon and post-monsoon period were chosen for sampling due to easy availability and high maturity of earthworms. The Kathua district is situated in 32°34′ N 75°29′ E with annual rainfall in this district is approximately 1672 mm. The summer temperature arises as high as 48 °C in the plains and in winter temperature touches to sub-zero mainly in upper hilly areas. The area under Kathua district experiences a wide range of climate from subtropical to temperate areas. The major crop of the study site is paddy, wheat, barley, and maize.
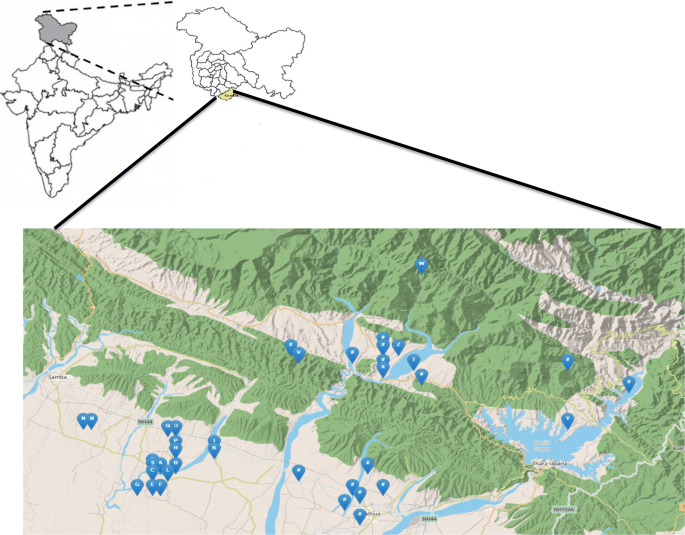
Map showing the location of study sites
Earthworm sampling and Identification
Earthworm’s sampling was done at 44 sites having cultivated and non-cultivated agro-ecosystem which were chosen randomly (Additional file 1 : Table S1). The collections of earthworms were mainly done during their activation period i.e. morning by using hand sorting (1 m × 1 m area) followed by AITC extraction solution [ 15 ] to collect deep burrowing earthworm species. The collected earthworms from all the diverse sites with a reasonable amount of soil were placed in plastic bags, named with the site name, sampling date, etc. The earthworms were washed with tap water and narcotized with 70% ethyl alcohol. The narcotized earthworms were transferred to the flat tray with a 5% formalin solution in a straight position for 4–6 h followed by preservation in test tubes containing 5% formalin. All the test tubes were labeled with the place of collection, date of collection and their habitat. The earthworms were identified according to the keys provided by Julka [ 41 ].
Physico-chemical analysis of soil
The soil samples were analyzed for texture, total dissolved solids (TDS), pH, electrical conductivity (EC), organic carbon (OC), nitrogen (N), sodium (Na), calcium (Ca), phosphorus (P), potassium (K). The method of Bouyoucos [ 42 ] as used for soil texture analysis. The content of pH, EC, and TDS was analyzed by making the suspension of 1:10 (soil sample: distilled water) using a shaker and finally measured with a digital meter (Eutech Instruments). The OC was measured by using Nelson and Sommers [ 43 ] method after igniting the soil samples in a muffle furnace at 550 °C. The N was analyzed according to Bremner and Mulvaney [ 44 ] method after digesting the soil sample with concentrated H 2 SO 4 followed by running the sample in Kjeldahl assembly and finally titration was done with 0.01 N HCl. The process of John [ 45 ] was used for phosphorus estimation after digesting the soil with 1:4 of perchloric acid and nitric acid respectively followed by using a spectrophotometer (Systronics). The content of Na, K and Ca was analyzed from samples digested for phosphorus by using Flame Photometer-128 (Systronics).
Statistical analysis
The data for physico-chemical properties of the soil is represented as mean ± S.E. of triplicate data. The Shannon-Wiener index, species richness index and species evenness for each sampling site were also calculated by using standard calculation as suggested by Shannon and Wiener [ 46 ], Margalef [ 47 ] and Pielou [ 48 ] respectively. Principal Component Analysis (PCA) was used to know the major components in the soil with one or more variable which affects the diversity of earthworms at a particular site. PERMANOVA and Mantel test were also applied to test the relationship between soil variables and earthworm abundance by using similarity matrices and the resemblance between the matrices was done by using Bray–Curtis similarity measures with 9999 random permutations. The past statistical software (version 3) and SPSS 16 (version 21) programme were used for the statistical analysis of the data.
Availability of data and materials
The data sets used and/or interpreted during the present research are accessible from the Corresponding author on reasonable request.
Abbreviations
- Principal component analysis
Principal component
Permutational multivariate analysis of variance
Standard error
Allyl isothiocyanate
Total dissolved solids
Electrical conductivity
Organic carbon
Hydrochloric acid
Sulphuric acid
Shannon-Wiener Diversity Index
Margalef species richness index
Pielou species evenness
Metaphire posthuma
Eutyphoeus waltoni
Eutyphoeus incommodus
Amynthas morrisi
Metaphire houlleti
Amynthas alexandri
Octochaetona beatrix
Metaphire birmanica
Perionyx excavatus
Polypheretima elongata
Singh S, Singh J, Vig AP. Effect of abiotic factors on the distribution of earthworms in different land use patterns. J Basic Appl Zool. 2016;74:41–50. https://doi.org/10.1016/j.jobaz.2016.06.001 .
Article Google Scholar
Schon NL, Mackay AD, Gray RA, Van Koten C, Dodd MB. Influence of earthworm abundance and diversity on soil structure and the implications for soil services throughout the season. Pedobiologia. 2017;62:41–7. https://doi.org/10.1016/j.pedobi.2017.05.001 .
Frazão J, de Goede RG, Brussaard L, Faber JH, Groot JC, Pulleman MM. Earthworm communities in arable fields and restored field margins, as related to management practices and surrounding landscape diversity. Agric Ecosyst Environ. 2017;248:1–8. https://doi.org/10.1016/j.agee.2017.07.014 .
Schmidt O, Clements RO, Donaldson G. Why do cereal–legume intercrops support large earthworm populations? Appl Soil Ecol. 2003;22(2):181–90. https://doi.org/10.1016/S0929-1393(02)00131-2 .
Baker GH, Carter PJ, Curry JP, Cultreri O, Beck A. Clay content of soil and its influence on the abundance of Aporrectodea trapezoides Duges (Lumbricidae). Appl Soil Ecol. 1998;9(1):333–7. https://doi.org/10.1016/S0929-1393(98)00085-7 .
Baker GH, Whitby WA. Soil pH preferences and the influences of soil type and temperature on the survival and growth of Aporrectodea longa (Lumbricidae): the 7th international symposium on earthworm ecology· Cardiff· Wales· 2002. Pedobiologia. 2003;47(5–6):745–53. https://doi.org/10.1078/0031-4056-00254 .
Perreault JM, Whalen JK. Earthworm burrowing in laboratory microcosms as influenced by soil temperature and moisture. Pedobiologia. 2006;50(5):397–403. https://doi.org/10.1016/j.pedobi.2006.07.003 .
Jiménez JJ, Decaëns T, Amézquita E, Rao I, Thomas RJ, Lavelle P. Short-range spatial variability of soil physico-chemical variables related to earthworm clustering in a neotropical gallery forest. Soil Biol Biochem. 2011;43(5):1071–80. https://doi.org/10.1016/j.soilbio.2011.01.028 .
Article CAS Google Scholar
Sharpley A, McDowell R, Moyer B, Littlejohn R. Land application of manure can influence earthworm activity and soil phosphorus distribution. Commun Soil Sci Plant Anal. 2011;42(2):194–207. https://doi.org/10.1080/00103624.2011.535070 .
Pelosi C, Barot S, Capowiez Y, Hedde M, Vandenbulcke F. Pesticides and earthworms. A review. Agron Sustain Dev. 2014;34(1):199–228. https://doi.org/10.1007/s13593-013-0151-z .
Pelosi C, Pey B, Hedde M, Caro G, Capowiez Y, Guernion M, Peigné J, Piron D, Bertrand M, Cluzeau D. Reducing tillage in cultivated fields increases earthworm functional diversity. Appl Soil Ecol. 2014;83:79–86. https://doi.org/10.1016/j.apsoil.2013.10.005 .
Crittenden SJ, Eswaramurthy T, De Goede RG, Brussaard L, Pulleman MM. Effect of tillage on earthworms over short-and medium-term in conventional and organic farming. Appl Soil Ecol. 2014;83:140–8. https://doi.org/10.1016/j.apsoil.2014.03.001 .
Blouin M, Hodson ME, Delgado EA, Baker G, Brussaard L, Butt KR, Dai J, Dendooven L, Pérès G, Tondoh JE, Cluzeau D. A review of earthworm impact on soil function and ecosystem services. Eur J Soil Sci. 2013;64(2):161–82. https://doi.org/10.1111/ejss.12025 .
Singh S, Singh J, Vig AP. Earthworm as ecological engineers to change the physico-chemical properties of soil: soil vs vermicast. Ecol Eng. 2016;90:1–5. https://doi.org/10.1016/j.ecoleng.2016.01.072 .
Singh J, Singh S, Bhat SA, Vig AP, Schädler M. Eco-friendly method for the extraction of earthworms: comparative account of formalin, AITC and Allium cepa as extractant. Appl Soil Ecol. 2018;124:141–5. https://doi.org/10.1016/j.apsoil.2017.11.007 .
De Wandeler H, Sousa-Silva R, Ampoorter E, Bruelheide H, Carnol M, Dawud SM, Dănilă G, Finer L, Hättenschwiler S, Hermy M, Jaroszewicz B. Drivers of earthworm incidence and abundance across European forests. Soil Biol Biochem. 2016;99:167–78. https://doi.org/10.1016/j.soilbio.2016.05.003 .
van Schaik L, Palm J, Klaus J, Zehe E, Schröder B. Potential effects of tillage and field borders on within-field spatial distribution patterns of earthworms. Agric Ecosyst Environ. 2016;228:82–90. https://doi.org/10.1016/j.agee.2016.05.015 .
Liu CW, Lin KH, Kuo YM. Application of factor analysis in the assessment of groundwater quality in a blackfoot disease area in Taiwan. Sci Total Environ. 2003;313(1–3):77–89. https://doi.org/10.1016/S0048-9697(02)00683-6 .
Article CAS PubMed Google Scholar
Mariotte P, Le Bayon RC, Eisenhauer N, Guenat C, Buttler A. Subordinate plant species moderate drought effects on earthworm communities in grasslands. Soil Biol Biochem. 2016;96:119–27. https://doi.org/10.1016/j.soilbio.2016.01.020 .
Suthar S. Earthworm communities a bioindicator of arable land management practices: a case study in semiarid region of India. Ecol indic. 2009;9(3):588–94. https://doi.org/10.1016/j.ecolind.2008.08.002 .
Solomou AD, Sfougaris AI, Vavoulidou EM, Csuzdi C. Species richness and density of earthworms in relation to soil factors in olive orchard production systems in Central Greece. Commun Soil Sci Plant Anal. 2013;44(1–4):301–11. https://doi.org/10.1080/00103624.2013.741904 .
Bartz ML, Pasini A, Brown GG. Earthworms as soil quality indicators in Brazilian no-tillage systems. Appl Soil Ecol. 2013;69:39. https://doi.org/10.1016/j.apsoil.2013.01.011 .
Margerie P, Decaëns T, Bureau F, Alard D. Spatial distribution of earthworm species assemblages in a chalky slope of the Seine Valley (Normandy, France). Eur J Soil Biol. 2001;37(4):291–6. https://doi.org/10.1016/S1164-5563(01)01100-1 .
Goswami R. Determination of ecological diversity indices to assess the interrelationship between earthworm diversity and different habitats of Indian Botanic garden, Howrah, India. Biol Forum. 2015;7(1):128.
Google Scholar
Singh J, Singh S, Vig AP, Bhat SA, Hundal SS, Yin R, Schädler M. Conventional farming reduces the activity of earthworms: assessment of genotoxicity test of soil and vermicast. Agri Nat Res. 2018;52(4):366–70. https://doi.org/10.1016/j.anres.2018.10.012 .
Singh J, Cameron E, Reitz T, Schädler M, Eisenhauer N. Grassland management effects on earthworm communities under ambient and future climatic conditions. Eur J Soil Sci. 2020. https://doi.org/10.1111/ejss.12942 .
Bacher MG, Fenton O, Bondi G, Creamer RE, Karmarkar M, Schmidt O. The impact of cattle dung pats on earthworm distribution in grazed pastures. BMC Ecol. 2018;18(1):1–12. https://doi.org/10.1186/s12898-018-0216-6 .
Sanchez EG, Munoz B, Garvin MH, Jesus JB, Cosín DD. Ecological preferences of some earthworm species in southwest Spain. Soil Biol Biochem. 1997;29(3–4):313–6. https://doi.org/10.1016/S0038-0717(96)00169-1 .
Cesarz S, Craven D, Dietrich C, Eisenhauer N. Effects of soil and leaf litter quality on the biomass of two endogeic earthworm species. Eur J Soil Biol. 2016;77:9–16. https://doi.org/10.1016/j.ejsobi.2016.09.002 .
Curry JP, Schmidt O. The feeding ecology of earthworms–a review. Pedobiologia. 2007;50(6):463–77. https://doi.org/10.1016/j.pedobi.2006.09.001 .
McCallum HM, Wilson JD, Beaumont D, Sheldon R, O’Brien MG, Park KJ. A role for liming as a conservation intervention? Earthworm abundance is associated with higher soil pH and foraging activity of a threatened shorebird in upland grasslands. Agri Ecosys Environ. 2016;223:182–9. https://doi.org/10.1016/j.agee.2016.03.005 .
Mir TA, Najar IA. Earthworm from doodhpathri (Budgam), Jammu and Kashmir. India. Int Res J Environ Sci. 2016;5:33–9.
Yvan C, Stéphane S, Stéphane C, Pierre B, Guy R, Hubert B. Role of earthworms in regenerating soil structure after compaction in reduced tillage systems. Soil Biol Biochem. 2012;55:93–103. https://doi.org/10.1016/j.agee.2016.03.005 .
Nordstrom S, Rundgren S. Environmental factors and lumbricid associations in southern Sweden. Pedobiologia. 1974;14:1–27.
Chan KY, Barchia I. Soil compaction controls the abundance, biomass and distribution of earthworms in a single dairy farm in south-eastern Australia. Soil Till Res. 2007;94(1):75–82. https://doi.org/10.1016/j.still.2006.07.006 .
Jänsch S, Steffens L, Höfer H, Horak F, Roß-Nickoll M, Russell D, Toschki A, Römbke J. State of knowledge of earthworm communities in German soils as a basis for biological soil quality assessment. Soil Org. 2013;85:215–33.
Singh S, Singh J, Sharma A, Vig AP, Ahmed S. First report of the earthworm Pontoscolex corethrurus (Müller, 1857) from Punjab, India. Int Lett Nat Sci. 2018;68:1–8. https://doi.org/10.18052/www.scipress.com/ILNS.68.1 .
Sharma RK, Bharadwaj P. Earthworm diversity in trans-gangetic habitats of Haryana. India. Res J Agri For Sci. 2014;2(2):1–7.
Walsh CL, Johnson-Maynard JL. Earthworm distribution and density across a climatic gradient within the Inland Pacific Northwest cereal production region. Appl Soil Ecol. 2016;104:104–10. https://doi.org/10.1016/j.apsoil.2015.12.010 .
Talavera JA, Cunha L, Arévalo JR, Talavera IP, Kille P, Novo M. Anthropogenic disturbance and environmental factors drive the diversity and distribution of earthworms in São Miguel Island (Azores, Portugal). Appl Soil Ecol. 2020. https://doi.org/10.1016/j.apsoil.2019.06.004 .
Julka JM. Know your Earthworms, Rashtriya Vigyan Evam Prodyogiki Sanchar Parishad (RVPSP) Solan (HP), 2008: 40-47.
Bouyoucos GJ. Hydrometer method improved for making particle size analyses of soils. Agron J. 1962;54(5):464–5. https://doi.org/10.2134/agronj1962.00021962005400050028x .
Nelson DW, Sommers LE. Total carbon, organic carbon, and organic matter. Methods Soil Anal. 1996;5:961–1010.
Bremner JM, Mulvaney RG. Nitrogen total. In: Page AL, Miller RH, Keeney DR, editors. Methods of Soil Analysis. Madison: Am Sco Agron; 1982. p. 575–624.
John MK. Colorimetric determination of phosphorus in soil and plant materials with ascorbic acid. Soil Sci. 1970;109(4):214–20.
Shannon CE, Wiener W. The Mathematical Theory of Communication. Urbana. University of Illinois Press. 1949; 177.
Margalef R. Temporal succession and spatial heterogeneity in phytoplankton. In: Buzzati-Traverso A, editor. Perspectives in Marine Biology. Berkeley: Univ Calif Press; 1958. p. 323–47.
Pielou EC. The measurement of diversity in different types of biological collections. J Theor Biol. 1996. https://doi.org/10.1016/0022-5193(66)90013-0 .
Download references
Acknowledgements
We acknowledge the University Grant Commission-UPE (University with Potential for Excellence) and Science & Engineering Research Board, Department of Science and Technology, New Delhi. We also acknowledge the Department of Botanical and Environmental Sciences, Guru Nanak Dev University, Amritsar, and Post Graduate Department of Zoology, Khalsa College Amritsar for providing the necessary infrastructure and lab facility to carry out the research work. Authors are also very grateful to Dr. J. M. Julka (Retd. Scientist, Zoological Survey of India), Shoolini University, Solan (Himachal Pradesh, India) for affirmation of earthworms species. We are also thankful to anonymous reviewers for their constructive comments which improved the manuscript.
Not applicable.
Author information
Authors and affiliations.
Department of Botanical & Environmental Sciences, Guru Nanak Dev University, Amritsar, Punjab, India
Sharanpreet Singh & Adarsh Pal Vig
Post Graduate Department of Zoology, Khalsa College, Amritsar, Punjab, India
Ayushi Sharma, Kiran Khajuria & Jaswinder Singh
You can also search for this author in PubMed Google Scholar
Contributions
The paper was conceived and designed by: SS, JS and APV. The sampling of earthworms and analysis of soil samples were done by SS, AS and KK. The data and articles were analyzed by JS and SS. The manuscript’s first draft was written by SS. The critical revisions and the final version were done by APV and JS. All authors have read and approved the final manuscript.
Corresponding authors
Correspondence to Jaswinder Singh or Adarsh Pal Vig .
Ethics declarations
Ethics approval and consent to participate.
The sampling of earthworms did not require permission and the species are not classified as endangered, and are not under any protection in any of the sampled areas.
Consent for publication
Competing interests.
The authors declare that there is no competing interest.
Additional information
Publisher's note.
Springer Nature remains neutral with regard to jurisdictional claims in published maps and institutional affiliations.
Supplementary information
Additional file 1: table s1..
The different sampling sites along with their agroecosystem, vegetation and GPS coordinates.
Rights and permissions
Open Access This article is licensed under a Creative Commons Attribution 4.0 International License, which permits use, sharing, adaptation, distribution and reproduction in any medium or format, as long as you give appropriate credit to the original author(s) and the source, provide a link to the Creative Commons licence, and indicate if changes were made. The images or other third party material in this article are included in the article's Creative Commons licence, unless indicated otherwise in a credit line to the material. If material is not included in the article's Creative Commons licence and your intended use is not permitted by statutory regulation or exceeds the permitted use, you will need to obtain permission directly from the copyright holder. To view a copy of this licence, visit http://creativecommons.org/licenses/by/4.0/ . The Creative Commons Public Domain Dedication waiver ( http://creativecommons.org/publicdomain/zero/1.0/ ) applies to the data made available in this article, unless otherwise stated in a credit line to the data.
Reprints and permissions
About this article
Cite this article.
Singh, S., Sharma, A., Khajuria, K. et al. Soil properties changes earthworm diversity indices in different agro-ecosystem. BMC Ecol 20 , 27 (2020). https://doi.org/10.1186/s12898-020-00296-5
Download citation
Received : 12 August 2019
Accepted : 29 April 2020
Published : 07 May 2020
DOI : https://doi.org/10.1186/s12898-020-00296-5
Share this article
Anyone you share the following link with will be able to read this content:
Sorry, a shareable link is not currently available for this article.
Provided by the Springer Nature SharedIt content-sharing initiative
- Land use pattern
- Soil variables
BMC Ecology
ISSN: 1472-6785
- General enquiries: [email protected]
Information
- Author Services
Initiatives
You are accessing a machine-readable page. In order to be human-readable, please install an RSS reader.
All articles published by MDPI are made immediately available worldwide under an open access license. No special permission is required to reuse all or part of the article published by MDPI, including figures and tables. For articles published under an open access Creative Common CC BY license, any part of the article may be reused without permission provided that the original article is clearly cited. For more information, please refer to https://www.mdpi.com/openaccess .
Feature papers represent the most advanced research with significant potential for high impact in the field. A Feature Paper should be a substantial original Article that involves several techniques or approaches, provides an outlook for future research directions and describes possible research applications.
Feature papers are submitted upon individual invitation or recommendation by the scientific editors and must receive positive feedback from the reviewers.
Editor’s Choice articles are based on recommendations by the scientific editors of MDPI journals from around the world. Editors select a small number of articles recently published in the journal that they believe will be particularly interesting to readers, or important in the respective research area. The aim is to provide a snapshot of some of the most exciting work published in the various research areas of the journal.
Original Submission Date Received: .
- Active Journals
- Find a Journal
- Proceedings Series
- For Authors
- For Reviewers
- For Editors
- For Librarians
- For Publishers
- For Societies
- For Conference Organizers
- Open Access Policy
- Institutional Open Access Program
- Special Issues Guidelines
- Editorial Process
- Research and Publication Ethics
- Article Processing Charges
- Testimonials
- Preprints.org
- SciProfiles
- Encyclopedia
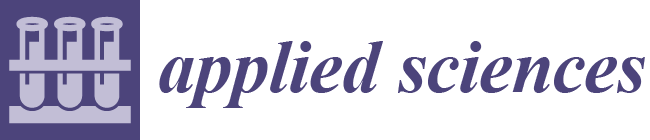
Article Menu

- Subscribe SciFeed
- Recommended Articles
- Google Scholar
- on Google Scholar
- Table of Contents
Find support for a specific problem in the support section of our website.
Please let us know what you think of our products and services.
Visit our dedicated information section to learn more about MDPI.
JSmol Viewer
A systematic review on earthworms in soil bioremediation.
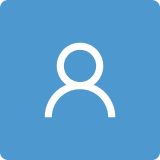
1. Introduction
2. materials and methods, 2.1. data sources and search strategies, 2.2. graphical construction, 3.1. alone inorganic vermiremediation, 3.2. alone organic vermiremediation, 3.3. combined vermiremediation for inorganic pollution.
Click here to enlarge figure
3.4. Combined Vermiremediation for Organic Pollution
3.5. combined vermiremediation for co-contamination pollution, 4. conclusions, author contributions, conflicts of interest.
- De Sousa, C. Contaminated Sites: The Canadian Situation in an International Context. J. Environ. Manage. 2001 , 62 , 131–154. [ Google Scholar ] [ CrossRef ] [ PubMed ]
- Liang, L.; Liu, W.; Sun, Y.; Huo, X.; Li, S.; Zhou, Q. Phytoremediation of Heavy Metal Contaminated Saline Soils Using Halophytes: Current Progress and Future Perspectives. Environ. Rev. 2017 , 25 , 269–281. [ Google Scholar ] [ CrossRef ]
- Zeb, A.; Li, S.; Wu, J.; Lian, J.; Liu, W.; Sun, Y. Insights into the Mechanisms Underlying the Remediation Potential of Earthworms in Contaminated Soil: A Critical Review of Research Progress and Prospects. Sci. Total Environ. 2020 , 740 , 140145. [ Google Scholar ] [ CrossRef ] [ PubMed ]
- Uwizeyimana, H.; Wang, M.; Chen, W.; Khan, K. The Eco-Toxic Effects of Pesticide and Heavy Metal Mixtures towards Earthworms in Soil. Environ. Toxicol. Pharmacol. 2017 , 55 , 20–29. [ Google Scholar ] [ CrossRef ] [ PubMed ]
- Bell, J. Pollution: Causes, Effects and Control. Environ. Pollut. 1997 , 96 , 276–277. [ Google Scholar ] [ CrossRef ]
- Hassan Al-Taai, S.H. Soil Pollution-Causes and Effects. IOP Conf. Ser. Earth Environ. Sci. 2021 , 790 , 012009. [ Google Scholar ] [ CrossRef ]
- Zhang, S.; Han, Y.; Peng, J.; Chen, Y.; Zhan, L.; Li, J. Human Health Risk Assessment for Contaminated Sites: A Retrospective Review. Environ. Int. 2023 , 171 , 107700. [ Google Scholar ] [ CrossRef ]
- Alengebawy, A.; Abdelkhalek, S.T.; Rana Qureshi, S.; Wang, M.-Q. Plants: Ecological Risks and Human Health Implications. Toxics 2021 , 9 , 42. [ Google Scholar ] [ CrossRef ]
- Sharma, A.; Shukla, A.; Attri, K.; Kumar, M.; Kumar, P.; Suttee, A.; Singh, G.; Barnwal, R.P.; Singla, N. Global Trends in Pesticides: A Looming Threat and Viable Alternatives. Ecotoxicol. Environ. Saf. 2020 , 201 , 110812. [ Google Scholar ] [ CrossRef ]
- Kole, R.K.; Banerjee, H.; Bhattacharyya, A. Monitoring of Market Fish Samples for Endosulfan and Hexachlorocyclohexane Residues in and Around Calcutta. Bull. Environ. Contam. Toxicol. 2001 , 67 , 0554–0559. [ Google Scholar ] [ CrossRef ]
- Food and Agriculture Organization. FAO Pesticides Use, Pesticides Trade and Pesticides Indicators 1990–2019 ; FAOSTAT. 29; Food and Agriculture Organization: Rome, Italy, 2021; pp. 1–22. [ Google Scholar ]
- Cara, I.G.; Țopa, D.; Puiu, I.; Jităreanu, G. Biochar a Promising Strategy for Pesticide-Contaminated Soils. Agriculture 2022 , 12 , 1579. [ Google Scholar ] [ CrossRef ]
- Raffa, C.M.; Chiampo, F. Bioremediation of Agricultural Soils Polluted with Pesticides: A Review. Bioengineering 2021 , 8 , 92. [ Google Scholar ] [ CrossRef ] [ PubMed ]
- Tarfeen, N.; Nisa, K.U.; Hamid, B.; Bashir, Z.; Yatoo, A.M.; Dar, M.A.; Mohiddin, F.A.; Amin, Z.; Ahmad, R.A.; Sayyed, R.Z. Microbial Remediation: A Promising Tool for Reclamation of Contaminated Sites with Special Emphasis on Heavy Metal and Pesticide Pollution: A Review. Processes 2022 , 10 , 1358. [ Google Scholar ] [ CrossRef ]
- Khan, M.J.; Zia, M.S.; Qasim, M. Use of Pesticides and Their Role in Environmental Pollution. World Acad. Sci. Eng. Technol. 2010 , 72 , 122–128. [ Google Scholar ]
- Sharma, A.; Kumar, V.; Shahzad, B.; Tanveer, M.; Sidhu, G.P.S.; Handa, N.; Kohli, S.K.; Yadav, P.; Bali, A.S.; Parihar, R.D.; et al. Worldwide Pesticide Usage and Its Impacts on Ecosystem. SN Appl. Sci. 2019 , 1 , 1446. [ Google Scholar ] [ CrossRef ]
- Terzano, R.; Rascio, I.; Allegretta, I.; Porfido, C.; Spagnuolo, M.; Khanghahi, M.Y.; Crecchio, C.; Sakellariadou, F.; Gattullo, C.E. Fire Effects on the Distribution and Bioavailability of Potentially Toxic Elements (PTEs) in Agricultural Soils. Chemosphere 2021 , 281 , 130752. [ Google Scholar ] [ CrossRef ]
- Kabata-Pendias, A. Trace Elements in Soils and Plants ; CRC Press: Boca Raton, FL, USA, 2000; ISBN 042919112X. [ Google Scholar ]
- Akram, R.; Turan, V.; Hammad, H.M.; Ahmad, S.; Hussain, S.; Hasnain, A.; Maqbool, M.M.; Rehmani, M.I.A.; Rasool, A.; Masood, N.; et al. Fate of Organic and Inorganic Pollutants in Paddy Soils. Environ. Pollut. Paddy Soils 2018 , 197–214. [ Google Scholar ] [ CrossRef ]
- Suman, J.; Uhlik, O.; Viktorova, J.; Macek, T. Phytoextraction of Heavy Metals: A Promising Tool for Clean-up of Polluted Environment? Front. Plant Sci. 2018 , 9 , 1476. [ Google Scholar ] [ CrossRef ]
- He, Z.; Shentu, J.; Yang, X.; Baligar, V.C.; Zhang, T.; Stoffella, P.J. Heavy Metal Contamination of Soils: Sources, Indicators, and Assessment. J. Environ. Indic. 2015 , 9 , 17–18. [ Google Scholar ]
- Raffa, C.M.; Chiampo, F.; Shanthakumar, S. Remediation of Metal/Metalloid-Polluted Soils: A Short Review. Appl. Sci. 2021 , 11 , 4134. [ Google Scholar ] [ CrossRef ]
- Zwolak, A.; Sarzyńska, M.; Szpyrka, E.; Stawarczyk, K. Sources of Soil Pollution by Heavy Metals and Their Accumulation in Vegetables: A Review. Water Air Soil Pollut. 2019 , 230 , 164. [ Google Scholar ] [ CrossRef ]
- Rafique, N.; Tariq, S.R. Distribution and Source Apportionment Studies of Heavy Metals in Soil of Cotton/Wheat Fields. Environ. Monit. Assess. 2016 , 188 , 309. [ Google Scholar ] [ CrossRef ]
- Rodrigues, A.A.Z.; De Queiroz, M.E.L.R.; De Oliveira, A.F.; Neves, A.A.; Heleno, F.F.; Zambolim, L.; Freitas, J.F.; Morais, E.H.C. Pesticide Residue Removal in Classic Domestic Processing of Tomato and Its Effects on Product Quality. J. Environ. Sci. Health Part B 2017 , 52 , 850–857. [ Google Scholar ] [ CrossRef ] [ PubMed ]
- Wiseman, C.L.S.; Zereini, F.; Püttmann, W. Metal and Metalloid Accumulation in Cultivated Urban Soils: A Medium-Term Study of Trends in Toronto, Canada. Sci. Total Environ. 2015 , 538 , 564–572. [ Google Scholar ] [ CrossRef ] [ PubMed ]
- Kuppusamy, S.; Maddela, N.R.; Megharaj, M.; Venkateswarlu, K.; Kuppusamy, S.; Maddela, N.R.; Megharaj, M.; Venkateswarlu, K. An Overview of Total Petroleum Hydrocarbons. In Total Petroleum Hydrocarbons–Environmental Fate, Toxicity, and Remediation ; Springer: Berlin/Heidelberg, Germany, 2020; pp. 1–27. [ Google Scholar ]
- Patowary, R.; Patowary, K.; Devi, A.; Kalita, M.C.; Deka, S. Uptake of Total Petroleum Hydrocarbon (TPH) and Polycyclic Aromatic Hydrocarbons (PAHs) by Oryza sativa L. Grown in Soil Contaminated with Crude Oil. Bull. Environ. Contam. Toxicol. 2017 , 98 , 120–126. [ Google Scholar ] [ CrossRef ]
- Patnaik, P. Health Hazard, Which Includes Toxic, Corrosive, Carcinogenic, and Teratogenic Properties, Exposure Limits. In A Comprehensive Guide to the Hazardous Properties of Chemical Substances ; Wiley: Hoboken, NJ, USA, 1992; pp. 425–445. [ Google Scholar ]
- Blumer, M.; Blumer, W.; Reich, T. Polycyclic Aromatic Hydrocarbons in Soils of a Mountain Valley: Correlation with Highway Traffic and Cancer Incidence. Environ. Sci. Technol. 1977 , 11 , 1082–1084. [ Google Scholar ] [ CrossRef ]
- Lawal, A.T. Polycyclic Aromatic Hydrocarbons. A Review. Cogent Environ. Sci. 2017 , 3 , 1339841. [ Google Scholar ] [ CrossRef ]
- Song, X.; Wu, X.; Song, X.; Shi, C.; Zhang, Z. Sorption and Desorption of Petroleum Hydrocarbons on Biodegradable and Nondegradable Microplastics. Chemosphere 2021 , 273 , 128553. [ Google Scholar ] [ CrossRef ] [ PubMed ]
- Kuppusamy, S.; Thavamani, P.; Venkateswarlu, K.; Lee, Y.B.; Naidu, R.; Megharaj, M. Remediation Approaches for Polycyclic Aromatic Hydrocarbons (PAHs) Contaminated Soils: Technological Constraints, Emerging Trends and Future Directions. Chemosphere 2017 , 168 , 944–968. [ Google Scholar ] [ CrossRef ]
- Haider, F.U.; Ejaz, M.; Cheema, S.A.; Khan, M.I.; Zhao, B.; Liqun, C.; Salim, M.A.; Naveed, M.; Khan, N.; Núñez-Delgado, A.; et al. Phytotoxicity of Petroleum Hydrocarbons: Sources, Impacts and Remediation Strategies. Environ. Res. 2021 , 197 , 111031. [ Google Scholar ] [ CrossRef ]
- Grifoni, M.; Rosellini, I.; Angelini, P.; Petruzzelli, G.; Pezzarossa, B. The Effect of Residual Hydrocarbons in Soil Following Oil Spillages on the Growth of Zea Mays Plants. Environ. Pollut. 2020 , 265 , 114950. [ Google Scholar ] [ CrossRef ] [ PubMed ]
- Patel, A.B.; Shaikh, S.; Jain, K.R.; Desai, C.; Madamwar, D. Polycyclic Aromatic Hydrocarbons: Sources, Toxicity, and Remediation Approaches. Front. Microbiol. 2020 , 11 , 562813. [ Google Scholar ] [ CrossRef ] [ PubMed ]
- Adeniji, A.O.; Okoh, O.O.; Okoh, A.I. Levels of Polycyclic Aromatic Hydrocarbons in the Water and Sediment of Buffalo River Estuary, South Africa and Their Health Risk Assessment. Arch. Environ. Contam. Toxicol. 2019 , 76 , 657–669. [ Google Scholar ] [ CrossRef ] [ PubMed ]
- Abdel-Shafy, H.I.; Mansour, M.S.M. A Review on Polycyclic Aromatic Hydrocarbons: Source, Environmental Impact, Effect on Human Health and Remediation. Egypt. J. Pet. 2016 , 25 , 107–123. [ Google Scholar ] [ CrossRef ]
- Kim, K.H.; Jahan, S.A.; Kabir, E.; Brown, R.J.C. A Review of Airborne Polycyclic Aromatic Hydrocarbons (PAHs) and Their Human Health Effects. Environ. Int. 2013 , 60 , 71–80. [ Google Scholar ] [ CrossRef ]
- Sampaio, G.R.; Guizellini, G.M.; da Silva, S.A.; de Almeida, A.P.; Pinaffi-Langley, A.C.C.; Rogero, M.M.; de Camargo, A.C.; Torres, E.A.F.S. Polycyclic Aromatic Hydrocarbons in Foods: Biological Effects, Legislation, Occurrence, Analytical Methods, and Strategies to Reduce Their Formation. Int. J. Mol. Sci. 2021 , 22 , 6010. [ Google Scholar ] [ CrossRef ] [ PubMed ]
- Li, R.; Zhang, J.; Krebs, P. Global Trade Drives Transboundary Transfer of the Health Impacts of Polycyclic Aromatic Hydrocarbon Emissions. Commun. Earth Environ. 2022 , 3 , 170. [ Google Scholar ] [ CrossRef ] [ PubMed ]
- Shen, H.; Huang, Y.; Wang, R.; Zhu, D.; Li, W.; Shen, G.; Wang, B.; Zhang, Y.; Chen, Y.; Lu, Y.; et al. From 1960 to 2008 and Future Predictions. Environ. Sci. Technol. 2013 , 47 , 6415–6424. [ Google Scholar ] [ CrossRef ]
- da Silva, I.G.S.; de Almeida, F.C.G.; da Rocha e Silva, N.M.P.; Casazza, A.A.; Converti, A.; Sarubbo, L.A. Soil Bioremediation: Overview of Technologies and Trends. Energies 2020 , 13 , 4664. [ Google Scholar ] [ CrossRef ]
- Shen, X.; Dai, M.; Yang, J.; Sun, L.; Tan, X.; Peng, C.; Ali, I.; Naz, I. A Critical Review on the Phytoremediation of Heavy Metals from Environment: Performance and Challenges. Chemosphere 2022 , 291 , 132979. [ Google Scholar ] [ CrossRef ]
- Vischetti, C.; Marini, E.; Casucci, C.; De Bernardi, A. Nickel in the Environment: Bioremediation Techniques for Soils with Low or Moderate Contamination in European Union. Environments 2022 , 9 , 133. [ Google Scholar ] [ CrossRef ]
- Berti, W.R.; Cunningham, S.D. Phytostabilization of Metals. In Phytoremediation Toxic Metals: Using Plants to Clean Up Environ ; Wiley: New York, NY, USA, 2000; pp. 71–88. [ Google Scholar ]
- Sarwar, N.; Imran, M.; Shaheen, M.R.; Ishaque, W.; Kamran, M.A.; Matloob, A.; Rehim, A.; Hussain, S. Phytoremediation Strategies for Soils Contaminated with Heavy Metals: Modifications and Future Perspectives. Chemosphere 2017 , 171 , 710–721. [ Google Scholar ] [ CrossRef ] [ PubMed ]
- Fagnano, M.; Agrelli, D.; Pascale, A.; Adamo, P.; Fiorentino, N.; Rocco, C.; Pepe, O.; Ventorino, V. Copper Accumulation in Agricultural Soils: Risks for the Food Chain and Soil Microbial Populations. Sci. Total Environ. 2020 , 734 , 139434. [ Google Scholar ] [ CrossRef ] [ PubMed ]
- Chen, L.; Beiyuan, J.; Hu, W.; Zhang, Z.; Duan, C.; Cui, Q.; Zhu, X.; He, H.; Huang, X.; Fang, L. Phytoremediation of Potentially Toxic Elements (PTEs) Contaminated Soils Using Alfalfa ( Medicago sativa L.): A Comprehensive Review. Chemosphere 2022 , 293 , 133577. [ Google Scholar ] [ CrossRef ]
- Tripathi, S.; Singh, V.K.; Srivastava, P.; Singh, R.; Devi, R.S.; Kumar, A.; Bhadouria, R. Phytoremediation of Organic Pollutants: Current Status and Future Directions. Abat. Environ. Pollut. 2020 , 81–105. [ Google Scholar ] [ CrossRef ]
- Salt, D.E.; Smith, R.D.; Raskin, I. Phytoremediation. Annu. Rev. Plant Biol. 1998 , 49 , 643–668. [ Google Scholar ] [ CrossRef ]
- Jacob, J.M.; Karthik, C.; Saratale, R.G.; Kumar, S.S.; Prabakar, D.; Kadirvelu, K.; Pugazhendhi, A. Biological Approaches to Tackle Heavy Metal Pollution: A Survey of Literature. J. Environ. Manage. 2018 , 217 , 56–70. [ Google Scholar ] [ CrossRef ]
- Subhashini, V.; Swamy, A.V.V.S. Open Access Phytoremediation of Pb and Ni Contaminated Soils Using Catharanthus roseus (L.). Univers. J. Environ. Res. Technol. 2013 , 3 , 465–472. [ Google Scholar ]
- Susarla, S.; Medina, V.F.; McCutcheon, S.C. Phytoremediation: An Ecological Solution to Organic Chemical Contamination. Ecol. Eng. 2002 , 18 , 647–658. [ Google Scholar ] [ CrossRef ]
- Awa, S.H.; Hadibarata, T. Removal of Heavy Metals in Contaminated Soil by Phytoremediation Mechanism: A Review. Water Air Soil Pollut. 2020 , 231 , 47. [ Google Scholar ] [ CrossRef ]
- Limmer, M.; Burken, J. Phytovolatilization of Organic Contaminants. Environ. Sci. Technol. 2016 , 50 , 6632–6643. [ Google Scholar ] [ CrossRef ]
- Yan, A.; Wang, Y.; Tan, S.N.; Mohd Yusof, M.L.; Ghosh, S.; Chen, Z. Phytoremediation: A Promising Approach for Revegetation of Heavy Metal-Polluted Land. Front. Plant Sci. 2020 , 11 , 359. [ Google Scholar ] [ CrossRef ]
- Olguìn, E.J.; Sànchez-Galvàn, G. Heavy Metal Removal in Phytofiltration and Phycoremediation: The Need to Differentiate between Bioadsorption and Bioaccumulation. New Biotechnol. 2012 , 30 , 3–8. [ Google Scholar ] [ CrossRef ]
- Cycoń, M.; Mrozik, A.; Piotrowska-Seget, Z. Bioaugmentation as a Strategy for the Remediation of Pesticide-Polluted Soil: A Review. Chemosphere 2017 , 172 , 52–71. [ Google Scholar ] [ CrossRef ] [ PubMed ]
- Nwankwegu, A.S.; Zhang, L.; Xie, D.; Onwosi, C.O.; Muhammad, W.I.; Odoh, C.K.; Sam, K.; Idenyi, J.N. Bioaugmentation as a Green Technology for Hydrocarbon Pollution Remediation. Problems and Prospects. J. Environ. Manage. 2022 , 304 , 114313. [ Google Scholar ] [ CrossRef ] [ PubMed ]
- Thompson, I.P.; Van Der Gast, C.J.; Ciric, L.; Singer, A.C. Bioaugmentation for Bioremediation: The Challenge of Strain Selection. Environ. Microbiol. 2005 , 7 , 909–915. [ Google Scholar ] [ CrossRef ] [ PubMed ]
- Taccari, M.; Milanovic, V.; Comitini, F.; Casucci, C.; Ciani, M. Effects of Biostimulation and Bioaugmentation on Diesel Removal and Bacterial Community. Int. Biodeterior. Biodegrad. 2012 , 66 , 39–46. [ Google Scholar ] [ CrossRef ]
- Semrany, S.; Favier, L.; Djelal, H.; Taha, S.; Amrane, A. Bioaugmentation: Possible Solution in the Treatment of Bio-Refractory Organic Compounds (Bio-ROCs). Biochem. Eng. J. 2012 , 69 , 75–86. [ Google Scholar ] [ CrossRef ]
- Shi, Z.; Liu, J.; Tang, Z.; Zhao, Y.; Wang, C. Vermiremediation of Organically Contaminated Soils: Concepts, Current Status, and Future Perspectives. Appl. Soil Ecol. 2020 , 147 , 103377. [ Google Scholar ] [ CrossRef ]
- Rodriguez-Campos, J.; Dendooven, L.; Alvarez-Bernal, D.; Contreras-Ramos, S.M. Potential of Earthworms to Accelerate Removal of Organic Contaminants from Soil: A Review. Appl. Soil Ecol. 2014 , 79 , 10–25. [ Google Scholar ] [ CrossRef ]
- Dada, E.O.; Akinola, M.O.; Owa, S.O.; Dedeke, G.A.; Aladesida, A.A.; Owagboriaye, F.O.; Oludipe, E.O. Efficacy of Vermiremediation to Remove Contaminants from Soil. J. Heal. Pollut. 2021 , 11 , 210302. [ Google Scholar ] [ CrossRef ] [ PubMed ]
- Yatoo, A.M.; Ali, N.; Zaheen, Z.; Ahmad, Z.; Shafat, B.; Saiema, A.; Ahmad, T.; Mika, S.; Kumar, P.; Burhan, G.; et al. Assessment of Pesticide Toxicity on Earthworms Using Multiple Biomarkers: A Review. Environ. Chem. Lett. 2022 , 20 , 2573–2596. [ Google Scholar ] [ CrossRef ]
- Pelosi, C.; Barot, S.; Capowiez, Y.; Hedde, M.; Vandenbulcke, F. Pesticides and Earthworms. A Review. Agron. Sustain. Dev. 2014 , 34 , 199–228. [ Google Scholar ] [ CrossRef ]
- Krishnaswamy, V.G.; Jaffar, M.F.; Sridharan, R.; Ganesh, S.; Kalidas, S.; Palanisamy, V.; Mani, K. Effect of Chlorpyrifos on the Earthworm Eudrilus Euginae and Their Gut Microbiome by Toxicological and Metagenomic Analysis. World J. Microbiol. Biotechnol. 2021 , 37 , 76. [ Google Scholar ] [ CrossRef ]
- Alvarez, M.F.; Poch, R.M.; Olarieta, J.R.; Wiedner, K. Charcoal and Biological Activity in Formiguer Soils of Catalonia (Spain): Application of a Micromorphological Approach. Soil Tillage Res. 2023 , 234 , 105810. [ Google Scholar ] [ CrossRef ]
- Spurgeon, D.J.; Weeks, J.M.; Van Gestel, C.A.M. A Summary of Eleven Years Progress in Earthworm Ecotoxicology. Pedobiologia 2003 , 47 , 588–606. [ Google Scholar ] [ CrossRef ]
- Schaefer, M. Assessing 2,4,6-Trinitrotoluene (TNT)-Contaminated Soil Using Three Different Earthworm Test Methods. Ecotoxicol. Environ. Saf. 2004 , 57 , 74–80. [ Google Scholar ] [ CrossRef ]
- Svobodovà, M.; Smídovà, K.; Hvezdovà, M.; Hofman, J. Uptake Kinetics of Pesticides Chlorpyrifos and Tebuconazole in the Earthworm Eisenia Andrei in Two Different Soils. Environ. Pollut. 2018 , 236 , 257–264. [ Google Scholar ] [ CrossRef ]
- Cao, J.; Ji, D.; Wang, C. Interaction between Earthworms and Arbuscular Mycorrhizal Fungi on the Degradation of Oxytetracycline in Soils. Soil Biol. Biochem. 2015 , 90 , 283–292. [ Google Scholar ] [ CrossRef ]
- Shi, Z.M.; Xu, L.; Hu, F. A Hierarchic Method for Studying the Distribution of Phenanthrene in Eisenia Fetida. Pedosphere 2014 , 24 , 743–752. [ Google Scholar ] [ CrossRef ]
- Schlatter, D.C.; Reardon, C.L.; Johnson-Maynard, J.; Brooks, E.; Kahl, K.; Norby, J.; Huggins, D.; Paulitz, T.C. Mining the Drilosphere: Bacterial Communities and Denitrifier Abundance in a No-till Wheat Cropping System. Front. Microbiol. 2019 , 10 , 1339. [ Google Scholar ] [ CrossRef ] [ PubMed ]
- Kuzyakov, Y.; Blagodatskaya, E. Microbial Hotspots and Hot Moments in Soil: Concept & Review. Soil Biol. Biochem. 2015 , 83 , 184–199. [ Google Scholar ] [ CrossRef ]
- Thakur, S.S.; Lone, A.R.; Singh, K.; Bhattacharyya, S.S.; Ratnasari, A.; Yadav, A.N.; Jain, S.K.; Yadav, S. Polycyclic Aromatic Hydrocarbon (PAH)–Contaminated Soil Decontamination Through Vermiremediation. Water Air Soil Pollut. 2023 , 234 , 247. [ Google Scholar ] [ CrossRef ]
- OECD. Test 222: Earthworms Reproduction Test (Eisenia Fetida/Eisenia Andrei) Guidel ; Testing Chemical; Organisation for Economic Cooperation and Development: Paris, France, 2016. [ Google Scholar ]
- OECD. Guideline for Testing of Chemicals “Earthworm, Acute Toxicity Tests” 207 ; Organisation for Economic Cooperation and Development: Paris, France, 1984. [ Google Scholar ] [ CrossRef ]
- Karwal, M.; Kaushik, A. Co-Composting and Vermicomposting of Coal Fly-Ash with Press Mud: Changes in Nutrients, Micro-Nutrients and Enzyme Activities. Environ. Technol. Innov. 2020 , 18 , 100708. [ Google Scholar ] [ CrossRef ]
- Paul, S.; Choudhury, M.; Deb, U.; Pegu, R.; Das, S.; Bhattacharya, S.S. Assessing the Ecological Impacts of Ageing on Hazard Potential of Solid Waste Landfills: A Green Approach through Vermitechnology. J. Clean. Prod. 2019 , 236 , 117643. [ Google Scholar ] [ CrossRef ]
- Paul, S.; Goswami, L.; Pegu, R.; Kumar Chatterjee, S.; Sundar Bhattacharya, S. Epigenetic Regulations Enhance Adaptability and Valorization Efficiency in Eisenia Fetida and Eudrilus Eugeniae during Vermicomposting of Textile Sludge: Insights on Repair Mechanisms of Metal-Induced Genetic Damage and Oxidative Stress. Bioresour. Technol. 2022 , 345 , 126493. [ Google Scholar ] [ CrossRef ]
- Sohal, B.; Singh, S.; Indra, S.; Singh, K.; Bhat, S.A.; Kaur, J. Comparing the Nutrient Changes, Heavy Metals, and Genotoxicity Assessment before and after Vermicomposting of Thermal Fly Ash Using Eisenia Fetida. Environ. Sci. Pollut. Res. Int. 2021 , 28 , 48154–48170. [ Google Scholar ] [ CrossRef ]
- Paul, S.; Das, S.; Raul, P.; Bhattacharya, S.S. Vermi-Sanitization of Toxic Silk Industry Waste Employing Eisenia Fetida and Eudrilus Eugeniae: Substrate Compatibility, Nutrient Enrichment and Metal Accumulation Dynamics. Bioresour. Technol. 2018 , 266 , 267–274. [ Google Scholar ] [ CrossRef ]
- Singh, S.I.; Singh, W.R.; Bhat, S.A.; Sohal, B.; Khanna, N.; Vig, A.P.; Ameen, F.; Jones, S. Vermiremediation of Allopathic Pharmaceutical Industry Sludge Amended with Cattle Dung Employing Eisenia Fetida. Environ. Res. 2022 , 214 , 113766. [ Google Scholar ] [ CrossRef ]
- Mupondi, L.T.; Mnkeni, P.N.S.; Muchaonyerwa, P.; Mupambwa, H.A. Vermicomposting Manure-Paper Mixture with Igneous Rock Phosphate Enhances Biodegradation, Phosphorus Bioavailability and Reduces Heavy Metal Concentrations. Heliyon 2018 , 4 , e00749. [ Google Scholar ] [ CrossRef ]
- Mago, M.; Yadav, A.; Gupta, R.; Garg, V.K. Management of Banana Crop Waste Biomass Using Vermicomposting Technology. Bioresour. Technol. 2021 , 326 , 124742. [ Google Scholar ] [ CrossRef ] [ PubMed ]
- Suthar, S. Metal Remediation from Partially Composted Distillery Sludge Using Composting Earthworm Eisenia Fetida. J. Environ. Monit. 2008 , 10 , 1099–1106. [ Google Scholar ] [ CrossRef ] [ PubMed ]
- Chakraborty, P.; Sarkar, S.; Mondal, S.; Agarwal, B.K.; Kumar, A.; Bhattacharya, S.; Bhattacharya, S.S.; Bhattacharyya, P. Eisenia Fetida Mediated Vermi-Transformation of Tannery Waste Sludge into Value Added Eco-Friendly Product: An Insight on Microbial Diversity, Enzyme Activation, and Metal Detoxification. J. Clean. Prod. 2022 , 348 , 131368. [ Google Scholar ] [ CrossRef ]
- Khan, M.B.; Cui, X.; Jilani, G.; Ting, Y.; Zehra, A.; Hamid, Y.; Hussain, B.; Tang, L.; Yang, X.; He, Z. Preincubation and Vermicomposting of Divergent Biosolids Exhibit Vice Versa Multielements Stoichiometry and Earthworm Physiology. J. Environ. Manage. 2019 , 243 , 144–156. [ Google Scholar ] [ CrossRef ]
- Žaltauskaitė, J.; Kniuipytė, I.; Praspaliauskas, M. Earthworm Eisenia Fetida Potential for Sewage Sludge Amended Soil Valorization by Heavy Metal Remediation and Soil Quality Improvement. J. Hazard. Mater. 2022 , 424 , 127316. [ Google Scholar ] [ CrossRef ]
- Zhang, J.; Sugir, M.E.; Li, Y.; Yuan, L.; Zhou, M.; Lv, P.; Yu, Z.; Wang, L. Effects of Vermicomposting on the Main Chemical Properties and Bioavailability of Cd/Zn in Pure Sludge. Environ. Sci. Pollut. Res. Int. 2019 , 26 , 20949–20960. [ Google Scholar ] [ CrossRef ] [ PubMed ]
- Iheme, P.O.; Ajayi, A.T.; Njoku, K.L. Vermiremediation Potentials of Lumbricus Terrestris and Eudrilus Euginae in Heavy Metal Contaminated Soil from Mechanic, Welder Workshop and Metallic Dumpsite. Niger. J. Biotechnol. 2021 , 38 , 118–132. [ Google Scholar ] [ CrossRef ]
- Paul, S.; Goswami, L.; Pegu, R.; Sundar Bhattacharya, S. Vermiremediation of Cotton Textile Sludge by Eudrilus Eugeniae: Insight into Metal Budgeting, Chromium Speciation, and Humic Substance Interactions. Bioresour. Technol. 2020 , 314 , 123753. [ Google Scholar ] [ CrossRef ]
- Barnhart, J. Occurrences, Uses, and Properties of Chromium. Regul. Toxicol. Pharmacol. 1997 , 26 , S3–S7. [ Google Scholar ] [ CrossRef ] [ PubMed ]
- Goswami, L.; Mukhopadhyay, R.; Sundar, S.; Das, P. Bioresource Technology Detoxi Fi Cation of Chromium-Rich Tannery Industry Sludge by Eudrillus Eugeniae: Insight on Compost Quality Forti Fi Cation and Microbial Enrichment. Bioresour. Technol. 2018 , 266 , 472–481. [ Google Scholar ] [ CrossRef ]
- Yuvaraj, A.; Karmegam, N.; Thangaraj, R. Vermistabilization of Paper Mill Sludge by an Epigeic Earthworm Perionyx Excavatus: Mitigation Strategies for Sustainable Environmental Management. Ecol. Eng. 2018 , 120 , 187–197. [ Google Scholar ] [ CrossRef ]
- Lescano, M.R.; Masin, C.E.; Rodríguez, A.R.; Godoy, J.L.; Zalazar, C.S. Earthworms to Improve Glyphosate Degradation in Biobeds. Environ. Sci. Pollut. Res. 2020 , 27 , 27023–27031. [ Google Scholar ] [ CrossRef ] [ PubMed ]
- Liu, M.; Cao, J.; Wang, C. Bioremediation by Earthworms on Soil Microbial Diversity and Partial Nitrification Processes in Oxytetracycline-Contaminated Soil. Ecotoxicol. Environ. Saf. 2020 , 189 , 109996. [ Google Scholar ] [ CrossRef ] [ PubMed ]
- Rubiyatno; Teh, Z.C.; Lestari, D.V.; Yulisa, A.; Musa, M.; Chen, T.-W.; Darwish, N.M.; AlMunqedhi, B.M.; Hadibarata, T. Tolerance of Earthworms in Soil Contaminated with Polycyclic Aromatic Hydrocarbon. Ind. Domest. Waste Manag. 2022 , 2 , 9–16. [ Google Scholar ] [ CrossRef ]
- Zenteno-Rojas, A.; Martinez-Romero, E.; Rincón-Molina, C.I.; Ruíz-Valdiviezo, V.M.; Meza-Gordillo, R.; Villalobos-Maldonado, J.J.; Rincón-Rosales, R. Removal of High Concentrations Decachlorobiphenyl of Earthworm Eisenia Fetida and Its Symbiotic Bacteria in a Vermicomposting System. Water Air Soil Pollut. 2019 , 230 , 5–6. [ Google Scholar ] [ CrossRef ]
- Owagboriaye, F.; Dedeke, G.; Bamidele, J.; Aladesida, A.; Isibor, P.; Feyisola, R.; Adeleke, M. Biochemical Response and Vermiremediation Assessment of Three Earthworm Species ( Alma millsoni , Eudrilus eugeniae and Libyodrilus violaceus ) in Soil Contaminated with a Glyphosate-Based Herbicide. Ecol. Indic. 2020 , 108 , 105678. [ Google Scholar ] [ CrossRef ]
- Mohammadi-Moghadam, F.; Khodadadi, R.; Sedehi, M.; Arbabi, M. Bioremediation of Polycyclic Aromatic Hydrocarbons in Contaminated Soils Using Vermicompost. Int. J. Chem. Eng. 2022 , 2022 , 5294170. [ Google Scholar ] [ CrossRef ]
- Nassar, S.E. Bioremediation Assessment, Hematological, and Biochemical Responses of the Earthworm ( Allolobophora caliginosa ) in Soil Contaminated with Crude Oil. Environ. Sci. Pollut. Res. Int. 2021 , 28 , 54565–54574. [ Google Scholar ] [ CrossRef ]
- Fawole, C.; Salami, S.J.; Dashak, D.A.; Chimezie-Nwosu, H.A. Bioengineered Approach for Ex Situ Vermiextraction of Acenaphthylene, Benzo (E)Pyrene and Benzo (Ghi) Perylene Soil Contamination. Curr. J. Appl. Sci. Technol. 2021 , 40 , 29–36. [ Google Scholar ] [ CrossRef ]
- Ma, W.; Immerzeel, J.; Bodt, J. Earthworm and Food Interaction on Bioaccumulation and Disappearance in Soil of Polycyclic Aromatic Hydrocarbons: Studies on Phenanthrene and Fluoranthene. Ecotoxicol. Environ. Saf. 1995 , 32 , 226–232. [ Google Scholar ] [ CrossRef ]
- Nobili, S.; Elisabet, C.; Susana, C.; Raquel, M. Bioremediation of Hydrocarbon Contaminated Soil Using Local Organic Materials and Earthworms. Environ. Pollut. 2022 , 314 , 120169. [ Google Scholar ] [ CrossRef ] [ PubMed ]
- Rajadurai, M.; Karmegam, N.; Kannan, S.; Yuvaraj, A.; Thangaraj, R. Vermiremediation of Engine Oil Contaminated Soil Employing Indigenous Earthworms, Drawida Modesta and Lampito Mauritii. J. Environ. Manage. 2022 , 301 , 113849. [ Google Scholar ] [ CrossRef ] [ PubMed ]
- Njoku, K.L.; Ogwara, C.A.; Adesuyi, A.A.; Akinola, M.O. Vermiremediation of Pesticide Contaminated Soil Using Eudrilus Euginae and Lumbricus Terrestris. EnvironmentAsia 2018 , 11 , 133–147. [ Google Scholar ] [ CrossRef ]
- Delgado-Moreno, L.; Nogales, R.; Romero, E. Vermiremediation of Biomixtures from Biobed Systems Contaminated with Pesticides. Appl. Sci. 2020 , 10 , 3173. [ Google Scholar ] [ CrossRef ]
- Lin, Z.; Zhen, Z.; Liang, Y.; Li, J.; Yang, J.; Zhong, L.; Zhao, L.; Li, Y.; Luo, C.; Ren, L.; et al. Changes in Atrazine Speciation and the Degradation Pathway in Red Soil during the Vermiremediation Process. J. Hazard. Mater. 2019 , 364 , 710–719. [ Google Scholar ] [ CrossRef ] [ PubMed ]
- Baran, W.; Adamek, E.; Ziemiańska, J.; Sobczak, A. Effects of the Presence of Sulfonamides in the Environment and Their Influence on Human Health. J. Hazard. Mater. 2011 , 196 , 1–15. [ Google Scholar ] [ CrossRef ]
- Lima, T.; Domingues, S.; Silva, G.J. Da Manure as a Potential Hotspot for Antibiotic Resistance Dissemination by Horizontal Gene Transfer Events. Vet. Sci. 2020 , 7 , 110. [ Google Scholar ] [ CrossRef ]
- Xu, L.; Zhang, H.; Xiong, P.; Zhu, Q.; Liao, C.; Jiang, G. Occurrence, Fate, and Risk Assessment of Typical Tetracycline Antibiotics in the Aquatic Environment: A Review. Sci. Total Environ. 2021 , 753 , 141975. [ Google Scholar ] [ CrossRef ]
- Lundström, S.V.; Östman, M.; Bengtsson-Palme, J.; Rutgersson, C.; Thoudal, M.; Sircar, T.; Blanck, H.; Eriksson, K.M.; Tysklind, M.; Flach, C.F.; et al. Minimal Selective Concentrations of Tetracycline in Complex Aquatic Bacterial Biofilms. Sci. Total Environ. 2016 , 553 , 587–595. [ Google Scholar ] [ CrossRef ]
- ECDC. Antimicrobial Consumption in the EU/EEA (ESAC-Net) ; European Centre for Disease Prevention and Control: Solna, Sweden, 2021.
- Lin, Z.; Zhen, Z.; Luo, S.; Ren, L.; Chen, Y.; Wu, W.; Zhang, W.; Liang, Y.Q.; Song, Z.; Li, Y.; et al. Effects of Two Ecological Earthworm Species on Tetracycline Degradation Performance, Pathway and Bacterial Community Structure in Laterite Soil. J. Hazard. Mater. 2021 , 412 , 125212. [ Google Scholar ] [ CrossRef ]
- Yin, B.; Zhang, M.; Zeng, Y.; Chen, H.; Fan, T.; Wu, Z.; Cao, L.; Zhao, Q. The Changes of Antioxidant System and Intestinal Bacteria in Earthworms ( Metaphire guillelmi ) on the Enhanced Degradation of Tetracycline. Chemosphere 2021 , 265 , 129097. [ Google Scholar ] [ CrossRef ] [ PubMed ]
- Zhang, L.; He, N.; Chang, D.; Liu, X.; Zhang, X.; Xu, Y.; Zhao, C.; Sun, J.; Li, W.; Li, H.; et al. Does Ecotype Matter? The Influence of Ecophysiology on Benzo[a]Pyrene and Cadmium Accumulation and Distribution in Earthworms. Soil Biol. Biochem. 2018 , 121 , 24–34. [ Google Scholar ] [ CrossRef ]
- Zhang, Y.; Song, K.; Zhang, J.; Xu, X.; Ye, G.; Cao, H.; Chen, M.; Cai, S.; Cao, X.; Zheng, X.; et al. Removal of Sulfamethoxazole and Antibiotic Resistance Genes in Paddy Soil by Earthworms ( Pheretima guillelmi ): Intestinal Detoxification and Stimulation of Indigenous Soil Bacteria. Sci. Total Environ. 2022 , 851 , 158075. [ Google Scholar ] [ CrossRef ] [ PubMed ]
- Ross, G. The Public Health Implications of Polychlorinated Biphenyls (PCBs) in the Environment. Ecotoxicol. Environ. Saf. 2004 , 59 , 275–291. [ Google Scholar ] [ CrossRef ] [ PubMed ]
- EPA. PCBs: Cancer Dose-Response Assessment and Application to Environmental Mixtures ; U.S. Environmental Protection Agency: Washington, DC, USA, 1996; p. 74.
- Luo, S.; Ren, L.; Wu, W.; Chen, Y.; Li, G.; Zhang, W.; Wei, T.; Liang, Y.Q.; Zhang, D.; Wang, X.; et al. Impacts of Earthworm Casts on Atrazine Catabolism and Bacterial Community Structure in Laterite Soil. J. Hazard. Mater. 2022 , 425 , 127778. [ Google Scholar ] [ CrossRef ]
- Antoniadis, V.; Levizou, E.; Shaheen, S.M.; Ok, Y.S.; Sebastian, A.; Baum, C.; Prasad, M.N.V.; Wenzel, W.W.; Rinklebe, J. Trace Elements in the Soil-Plant Interface: Phytoavailability, Translocation, and Phytoremediation–A Review. Earth-Science Rev. 2017 , 171 , 621–645. [ Google Scholar ] [ CrossRef ]
- Sizmur, T.; Hodson, M.E. Do Earthworms Impact Metal Mobility and Availability in Soil?–A Review. Environ. Pollut. 2009 , 157 , 1981–1989. [ Google Scholar ] [ CrossRef ] [ PubMed ]
- Bhat, S.A.; Bhatti, S.S.; Singh, J.; Sambyal, V.; Nagpal, A.; Vig, A.P. Vermiremediation and Phytoremediation: Eco Approaches for Soil Stabilization. Austin Envronmental Sci. 2016 , 1 , 1–10. [ Google Scholar ]
- Wen, B.; Hu, X.Y.; Liu, Y.; Wang, W.S.; Feng, M.H.; Shan, X.Q. The Role of Earthworms ( Eisenia fetida ) in Influencing Bioavailability of Heavy Metals in Soils. Biol. Fertil. Soils 2004 , 40 , 181–187. [ Google Scholar ] [ CrossRef ]
- Tibihenda, C.; Zhang, M.; Zhong, H.; Xiao, L.; Wu, L.; Dai, J.; Liu, K.; Zhang, C. Growth and Pb Uptake of Brassica Campestris Enhanced by Two Ecological Earthworm Species in Relation to Soil Physicochemical Properties. Front. Environ. Sci. 2022 , 10 , 1–14. [ Google Scholar ] [ CrossRef ]
- Naseer, A.; Andleeb, S.; Basit, A.; Ali, S.; ud-Din, M.S.; Ali, N.M.; Liaqat, I.; Nazir, A. Efficacy of Cow and Buffalo Dung on Vermiremediation and Phytoremediation of Heavy Metals via Fourier-Transform Infrared Spectroscopy and Comet Assay. Environ. Sci. Pollut. Res. 2022 , 30 , 37912–37928. [ Google Scholar ] [ CrossRef ]
- Ruley, J.A.; Amoding, A.; Tumuhairwe, J.B.; Basamba, T.A.; Opolot, E.; Oryem-Origa, H. Enhancing the Phytoremediation of Hydrocarbon-Contaminated Soils in the Sudd Wetlands, South Sudan, Using Organic Manure. Appl. Environ. Soil Sci. 2020 , 2020 , 4614286. [ Google Scholar ] [ CrossRef ]
- Guo, F.; Proctor, G.; Larson, S.L.; Ballard, J.H.; Zan, S.; Yang, R.; Wang, X.; Han, F.X. Earthworm Enhanced Phytoremediation of U in Army Test Range Soil with Indian Mustard and Sun Fl Ower. ACS Earth Space Chem. 2022 , 6 , 746–754. [ Google Scholar ] [ CrossRef ]
- Verma, S.; Kuila, A. Bioremediation of Heavy Metals by Microbial Process. Environ. Technol. Innov. 2019 , 14 , 100369. [ Google Scholar ] [ CrossRef ]
- Munnoli, P.M.; Jaime, M.; Teixeira, A.; Saroj, S. Dynamics of the Soil-Earthworm-Plant Relationship: A Review. Dyn. Soils Dyn. Plant 2010 , 4 , 1–21. [ Google Scholar ]
- Lemtiri, A.; Colinet, G.; Alabi, T.; Cluzeau, D.; Zirbes, L.; Haubruge, É.; Francis, F. Impacts of Earthworms on Soil Components and Dynamics. A Review. Biotechnol. Agron. Soc. Environ. 2014 , 18 , 121–133. [ Google Scholar ]
- Nechitaylo, T.Y.; Yakimov, M.M.; Godinho, M.; Timmis, K.N.; Belogolova, E.; Byzov, B.A.; Kurakov, A.V.; Jones, D.L.; Golyshin, P.N. Effect of the Earthworms Lumbricus Terrestris and Aporrectodea Caliginosa on Bacterial Diversity in Soil. Microb. Ecol. 2010 , 59 , 574–587. [ Google Scholar ] [ CrossRef ]
- Šrut, M.; Menke, S.; Höckner, M.; Sommer, S. Earthworms and Cadmium–Heavy Metal Resistant Gut Bacteria as Indicators for Heavy Metal Pollution in Soils? Ecotoxicol. Environ. Saf. 2019 , 171 , 843–853. [ Google Scholar ] [ CrossRef ]
- El-Hassanin, A.S.; Samak, M.R.; Ahmed, S.M.; Afifi, M.M.I.; El-satar, M.A. Bioaccumulation of Heavy Metals during Composting and Vermicomposting Processes of Sewage Sludge. Egypt. J. Chem. 2022 , 65 , 1155–1162. [ Google Scholar ] [ CrossRef ]
- Xiao, R.; Liu, X.; Ali, A.; Chen, A.; Zhang, M.; Li, R.; Chang, H.; Zhang, Z. Bioremediation of Cd-Spiked Soil Using Earthworms ( Eisenia fetida ): Enhancement with Biochar and Bacillus Megatherium Application. Chemosphere 2021 , 264 , 128517. [ Google Scholar ] [ CrossRef ]
- Tara, N.; Arslan, M.; Hussain, Z.; Iqbal, M.; Khan, Q.M.; Afzal, M. On-Site Performance of Floating Treatment Wetland Macrocosms Augmented with Dye-Degrading Bacteria for the Remediation of Textile Industry Wastewater. J. Clean. Prod. 2019 , 217 , 541–548. [ Google Scholar ] [ CrossRef ]
- Wang, G.; Wang, L.; Ma, F.; You, Y.; Wang, Y.; Yang, D. Integration of Earthworms and Arbuscular Mycorrhizal Fungi into Phytoremediation of Cadmium-Contaminated Soil by Solanum nigrum L. J. Hazard. Mater. 2020 , 389 , 121873. [ Google Scholar ] [ CrossRef ] [ PubMed ]
- Lehmann, J.; Joseph, S. Biochar for Environmental Management: An Introduction. In Biochar for Environmental Management ; Routledge: Oxford, UK, 2015; pp. 1–13. ISBN 0203762266. [ Google Scholar ]
- Ahmad, M.; Upamali, A.; Eun, J.; Zhang, M.; Bolan, N.; Mohan, D.; Vithanage, M.; Soo, S.; Sik, Y. Chemosphere Biochar as a Sorbent for Contaminant Management in Soil and Water: A Review. Chemosphere 2014 , 99 , 19–33. [ Google Scholar ] [ CrossRef ] [ PubMed ]
- Ameen, F.; Al-Homaidan, A.A. Treatment of Heavy Metal–Polluted Sewage Sludge Using Biochar Amendments and Vermistabilization. Environ. Monit. Assess. 2022 , 194 , 861. [ Google Scholar ] [ CrossRef ]
- Blenis, N.; Hue, N.; Maaz, T.M.C.; Kantar, M. Biochar Production, Modification, and Its Uses in Soil Remediation: A Review. Sustainability 2023 , 15 , 3442. [ Google Scholar ] [ CrossRef ]
- Khan, M.B.; Cui, X.; Jilani, G.; Lazzat, U.; Zehra, A.; Hamid, Y.; Hussain, B.; Tang, L.; Yang, X.; He, Z. Eisenia Fetida and Biochar Synergistically Alleviate the Heavy Metals Content during Valorization of Biosolids via Enhancing Vermicompost Quality. Sci. Total Environ. 2019 , 684 , 597–609. [ Google Scholar ] [ CrossRef ]
- Kumar Badhwar, V.; Singh, S.; Singh, B. Vermiremediation of Paper Mill Sludge with Cow Dung and Tea Waste Amendments Using Epigeic Earthworm Eisenia Fetida (Savigny). Res. Sq. 2022 , 1–20. [ Google Scholar ] [ CrossRef ]
- Ghavidel, A.; Rad, S.N.; Alikhani, H.A.; Yakhchali, B.; Pourbabai, A.A. Presence of Eisenia fetida enhanced phytoremediation of anthracene by Lolium perenne . Biosci. J. 2018 , 34 , 888–898. [ Google Scholar ] [ CrossRef ]
- Alves, D.; Villar, I.; Mato, S. Joint Application of Biological Techniques for the Remediation of Waste Contaminated with Hydrocarbons. Waste Biomass Valorization 2023 , 14 , 977–987. [ Google Scholar ] [ CrossRef ]
- Wang, Z.; Xu, Y.; Zhao, J.; Li, F.; Gao, D.; Xing, B. Remediation of Petroleum Contaminated Soils through Composting and Rhizosphere Degradation. J. Hazard. Mater. 2011 , 190 , 677–685. [ Google Scholar ] [ CrossRef ]
- Remelli, S.; Scibona, A.; Nizzoli, D.; Mantovani, L.; Tribaudino, M.; Celico, F.; Menta, C. Vermiremediation Applied to PCB and PCDD/F Contaminated Soils and Its Implications for Percolating Water. Environ. Res. 2022 , 214 , 113765. [ Google Scholar ] [ CrossRef ] [ PubMed ]
- Ahmed, N.A.M.; Sulieman, H.N.A.; Ahmed, M.A.H.; Abd, E.S.A.I.; Azhari, O.A. Bacterial and Vermi-Remediation of Soil Contaminated with Chlorpyrifos Insecticide. African J. Biotechnol. 2020 , 19 , 26–32. [ Google Scholar ] [ CrossRef ]
- Koolivand, A.; Saeedi, R.; Coulon, F.; Kumar, V.; Villaseñor, J.; Asghari, F.; Hesampoor, F. Bioremediation of Petroleum Hydrocarbons by Vermicomposting Process Bioaugmentated with Indigenous Bacterial Consortium Isolated from Petroleum Oily Sludge. Ecotoxicol. Environ. Saf. 2020 , 198 , 110645. [ Google Scholar ] [ CrossRef ]
- Vázquez-Núñez, E.; Molina-Guerrero, C.E.; Peña-Castro, J.M.; Fernández-Luqueño, F.; de la Rosa-Álvarez, M.G. Use of Nanotechnology for the Bioremediation of Contaminants: A Review. Processes 2020 , 8 , 826. [ Google Scholar ] [ CrossRef ]
- Bharagava, R.N.; Saxena, G.; Mulla, S.I. Introduction to Industrial Wastes Containing Organic and Inorganic Pollutants and Bioremediation Approaches for Environmental Management. In Bioremediation of Industrial Waste for Environmental Safety ; Springer: Singapore, 2020; pp. 1–18. [ Google Scholar ]
- Zhang, J.; Zhang, L.; He, M.; Wang, Y.; Zhang, C.; Lin, D. Bioresponses of Earthworm-Microbiota Symbionts to Polychlorinated Biphenyls in the Presence of Nano Zero Valent Iron in Soil. Sci. Total Environ. 2023 , 856 , 159226. [ Google Scholar ] [ CrossRef ]
- Weissenfels, W.D.; Klewer, H.-J.; Langhoff, J. Adsorption of Polycyclic Aromatic Hydrocarbons (PAHs) by Soil Particles: Influence on Biodegradability and Biotoxicity. Appl. Microbiol. Biotechnol. 1992 , 36 , 689–696. [ Google Scholar ] [ CrossRef ] [ PubMed ]
- Franzetti, A.; Di Gennaro, P.; Bestetti, G.; Lasagni, M.; Pitea, D.; Collina, E. Selection of Surfactants for Enhancing Diesel Hydrocarbons-Contaminated Media Bioremediation. J. Hazard. Mater. 2008 , 152 , 1309–1316. [ Google Scholar ] [ CrossRef ]
- Rosenberg, E.; Ron, E.Z. High- and Low-Molecular-Mass Microbial Surfactants. Appl. Microbiol. Biotechnol. 1999 , 52 , 154–162. [ Google Scholar ] [ CrossRef ]
- Shi, Z.; Wang, C.; Zhao, Y. Effects of Surfactants on the Fractionation, Vermiaccumulation, and Removal of Fluoranthene by Earthworms in Soil. Chemosphere 2020 , 250 , 126332. [ Google Scholar ] [ CrossRef ]
- Zhen, Z.; Luo, S.; Chen, Y.; Li, G.; Li, H.; Wei, T.; Huang, F.; Ren, L.; Liang, Y.Q.; Lin, Z.; et al. Performance and Mechanisms of Biochar-Assisted Vermicomposting in Accelerating Di-(2-Ethylhexyl) Phthalate Biodegradation in Farmland Soil. J. Hazard. Mater. 2023 , 443 , 130330. [ Google Scholar ] [ CrossRef ]
- del Carmen Cuevas-Díaz, M.; Ramos-Morales, F.R.; Castro-Luna, A.; Vázquez-Luna, D.; Martínez-Hernández, S.; Guzmán-López, O.; Zavala-Cruz, J.; Ortiz-Ceballos, Á.I. Synergy of the Tropical Earthworm Pontoscolex Corethrurus and Oil Palm Bagasse in the Removal of Heavy Crude Oil. Bull. Environ. Contam. Toxicol. 2022 , 108 , 956–962. [ Google Scholar ] [ CrossRef ] [ PubMed ]
- Topoliantz, S.; Ponge, J.F. Burrowing Activity of the Geophagous Earthworm Pontoscolex Corethrurus (Oligochaeta: Glossoscolecidae) in the Presence of Charcoal. Appl. Soil Ecol. 2003 , 23 , 267–271. [ Google Scholar ] [ CrossRef ]
- Rodriguez-Campos, J.; Perales-Garcia, A.; Hernandez-Carballo, J.; Martinez-Rabelo, F.; Hernández-Castellanos, B.; Barois, I.; Contreras-Ramos, S.M. Bioremediation of Soil Contaminated by Hydrocarbons with the Combination of Three Technologies: Bioaugmentation, Phytoremediation, and Vermiremediation. J. Soils Sediments 2019 , 19 , 1981–1994. [ Google Scholar ] [ CrossRef ]
- Hernandez-Castellanos, B.; Zavala-Cru, J.; Martinez-H, S.; Dendooven, L.; Contreras-, S.M.; Noa-Carraz, J.C.; Fragoso, C.; Ortiz-Ceba, A.I. Earthworm Populations in an Aged Hydrocarbon Contaminated Soil. Res. J. Environ. Sci. 2013 , 7 , 27–37. [ Google Scholar ] [ CrossRef ]
- Urionabarrenetxea, E.; Garcia-Velasco, N.; Anza, M.; Artetxe, U.; Lacalle, R.; Garbisu, C.; Becerril, T.; Soto, M. Application of in Situ Bioremediation Strategies in Soils Amended with Sewage Sludges. Sci. Total Environ. 2021 , 766 , 144099. [ Google Scholar ] [ CrossRef ]
- Lacalle, R.G.; Aparicio, J.D.; Artetxe, U.; Urionabarrenetxea, E.; Polti, M.A.; Soto, M.; Garbisu, C.; Becerril, J.M. Gentle Remediation Options for Soil with Mixed Chromium (VI) and Lindane Pollution: Biostimulation, Bioaugmentation, Phytoremediation and Vermiremediation. Heliyon 2020 , 6 , e04550. [ Google Scholar ] [ CrossRef ] [ PubMed ]
- Aparicio, J.D.; Lacalle, R.G.; Artetxe, U.; Urionabarrenetxea, E.; Becerril, J.M.; Polti, M.A.; Garbisu, C.; Soto, M. Successful Remediation of Soils with Mixed Contamination of Chromium and Lindane: Integration of Biological and Physico-Chemical Strategies. Environ. Res. 2021 , 194 , 110666. [ Google Scholar ] [ CrossRef ]
The statements, opinions and data contained in all publications are solely those of the individual author(s) and contributor(s) and not of MDPI and/or the editor(s). MDPI and/or the editor(s) disclaim responsibility for any injury to people or property resulting from any ideas, methods, instructions or products referred to in the content. |
Share and Cite
Tagliabue, F.; Marini, E.; De Bernardi, A.; Vischetti, C.; Casucci, C. A Systematic Review on Earthworms in Soil Bioremediation. Appl. Sci. 2023 , 13 , 10239. https://doi.org/10.3390/app131810239
Tagliabue F, Marini E, De Bernardi A, Vischetti C, Casucci C. A Systematic Review on Earthworms in Soil Bioremediation. Applied Sciences . 2023; 13(18):10239. https://doi.org/10.3390/app131810239
Tagliabue, Francesca, Enrica Marini, Arianna De Bernardi, Costantino Vischetti, and Cristiano Casucci. 2023. "A Systematic Review on Earthworms in Soil Bioremediation" Applied Sciences 13, no. 18: 10239. https://doi.org/10.3390/app131810239
Article Metrics
Article access statistics, further information, mdpi initiatives, follow mdpi.
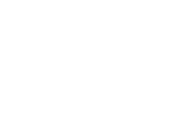
Subscribe to receive issue release notifications and newsletters from MDPI journals
Thank you for visiting nature.com. You are using a browser version with limited support for CSS. To obtain the best experience, we recommend you use a more up to date browser (or turn off compatibility mode in Internet Explorer). In the meantime, to ensure continued support, we are displaying the site without styles and JavaScript.
- View all journals
- Explore content
- About the journal
- Publish with us
- Sign up for alerts
- Review Article
- Published: 03 February 2013
Greenhouse-gas emissions from soils increased by earthworms
- Ingrid M. Lubbers 1 ,
- Kees Jan van Groenigen 2 ,
- Steven J. Fonte 3 ,
- Johan Six 4 ,
- Lijbert Brussaard 1 &
- Jan Willem van Groenigen 1
Nature Climate Change volume 3 , pages 187–194 ( 2013 ) Cite this article
8501 Accesses
280 Citations
212 Altmetric
Metrics details
- Biogeochemistry
- Climate sciences
Earthworms play an essential part in determining the greenhouse-gas balance of soils worldwide, and their influence is expected to grow over the next decades. They are thought to stimulate carbon sequestration in soil aggregates, but also to increase emissions of the main greenhouse gases carbon dioxide and nitrous oxide. Hence, it remains highly controversial whether earthworms predominantly affect soils to act as a net source or sink of greenhouse gases. Here, we provide a quantitative review of the overall effect of earthworms on the soil greenhouse-gas balance. Our results suggest that although earthworms are largely beneficial to soil fertility, they increase net soil greenhouse-gas emissions.
This is a preview of subscription content, access via your institution
Access options
Subscribe to this journal
Receive 12 print issues and online access
195,33 € per year
only 16,28 € per issue
Buy this article
- Purchase on SpringerLink
- Instant access to full article PDF
Prices may be subject to local taxes which are calculated during checkout
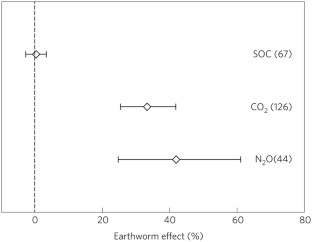
Similar content being viewed by others
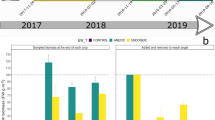
Earthworms do not increase greenhouse gas emissions (CO 2 and N 2 O) in an ecotron experiment simulating a three-crop rotation system
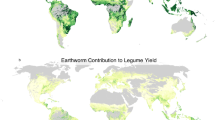
Earthworms contribute significantly to global food production
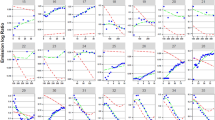
Changes in soil carbon mineralization related to earthworm activity depend on the time since inoculation and their density in soil
Rastogi, M., Singh, S. & Pathak, H. Emission of carbon dioxide from soil. Curr. Sci. 82 , 510–518 (2002).
CAS Google Scholar
Smith, K. A. et al. Exchange of greenhouse gases between soil and atmosphere: Interactions of soil physical factors and biological processes. Eur. J. Soil Sci. 54 , 779–791 (2003).
Article Google Scholar
Le Mer, J. & Roger, P. Production, oxidation, emission and consumption of methane by soils: A review. Eur. J. Soil Biol. 37 , 25–50 (2001).
Article CAS Google Scholar
Wrage, N., Velthof, G. L., van Beusichem, M. L. & Oenema, O. Role of nitrifier denitrification in the production of nitrous oxide. Soil Biol. Biochem. 33 , 1723–1732 (2001).
Kool, D. M. et al. Nitrifier denitrification can be a source of N2O from soil: A revised approach to the dual-isotope labelling method. Eur. J. Soil Sci. 61 , 759–772 (2010).
Brown, G. G., Barois, I. & Lavelle, P. Regulation of soil organic matter dynamics and microbial activityin the drilosphere and the role of interactions with other edaphic functional domains. Eur. J. Soil Biol. 36 , 177–198 (2000).
Lavelle, P. et al. Soil function in a changing world: The role of invertebrate ecosystem engineers. Eur. J. Soil Biol. 33 , 159–193 (1997).
Edwards, C. A. Earthworm Ecology 2nd edn (CRC, 2004).
Book Google Scholar
Drake, H. L. & Horn, M. A. Earthworms as a transient heaven for terrestrial denitrifying microbes: A review. Eng. Life Sci. 6 , 261–265 (2006). Emission of nitrogenous gases (N 2 O and N 2 ) appears to be primarily produced by soil-derived denitrifying bacteria when they are subjected to the unique in situ conditions of the earthworm gut; the particular microenvironment of the earthworm gut might also affect the fitness and diversity of certain members of the soil microbial biome.
Elliott, P. W., Knight, D. & Anderson, J. M. Variables controlling denitrification from earthworm casts and soil in permanent pastures. Biol. Fert. Soils 11 , 24–29 (1991).
Chapuis-Lardy, L. et al. Effect of the endogeic earthworm Pontoscolex corethrurus on the microbial structure and activity related to CO2 and N2O fluxes from a tropical soil (Madagascar). Appl. Soil Ecol. 45 , 201–208 (2010). The presence of P. corethrurus induced a significant increase in CO 2 emissions, but did not affect N 2 O fluxes when measured at mesocosm level, thereby showing opposing effects of earthworms on CO 2 and N 2 O emissions within the same study.
Giannopoulos, G., Pulleman, M. M. & van Groenigen, J. W. Interactions between residue placement and earthworm ecological strategy affect aggregate turnover and N2O dynamics in agricultural soil. Soil Biol. Biochem. 42 , 618–625 (2010). Earthworm-induced N 2 O emissions reflect earthworm feeding strategies: epigeic earthworms can increase N 2 O emissions when residue is applied on the surface; endogeic earthworms when residue is incorporated into the soil by humans (tillage) or by other earthworm species. The relationships between (functional) soil biodiversity and the soil greenhouse-gas balance are important but intricate.
Lubbers, I. M., Brussaard, L., Otten, W. & van Groenigen, J. W. Earthworm-induced N mineralization in fertilized grassland increases both N2O emission and crop-N uptake. Eur. J. Soil Sci. 62 , 152–161 (2011).
Rizhiya, E. et al. Earthworm activity as a determinant for N2O emission from crop residue. Soil Biol. Biochem. 39 , 2058–2069 (2007).
Lavelle, P. et al. in Earthworm Ecology (ed. Edwards, C. A.) 145–160 (CRC, 2004).
Google Scholar
Bossuyt, H., Six, J. & Hendrix, P. F. Protection of soil carbon by microaggregates within earthworm casts. Soil Biol. Biochem. 37 , 251–258 (2005).
Pulleman, M. M., Six, J., van Breemen, N. & Jongmans, A. G. Soil organic matter distribution and microaggregate characteristics as affected by agricultural management and earthworm activity. Eur. J. Soil Sci. 56 , 453–467 (2005).
Six, J., Bossuyt, H., Degryze, S. & Denef, K. A history of research on the link between (micro)aggregates, soil biota, and soil organic matter dynamics. Soil Till. Res. 79 , 7–31 (2004). The quantification of aggregate formation and SOM stabilization can greatly benefit from viewing aggregates as dynamic rather than static entities; the activity of earthworms has a decisive role in the formation of macro- and microaggregates.
Hendrix, P. F. & Bohlen, P. J. Exotic earthworm invasions in North America: Ecological and policy implications. Bioscience 52 , 801–811 (2002). Invasions of exotic earthworms in North America can have negative implications for soil processes, other animals and plant species. More basic knowledge is needed of the natural history and ecology of invasive earthworms in their native habitats, and in ecosystems where they have invaded and had significant impacts.
Norse, D. & Tschirley, J. in World Agriculture: Towards 2015/2030. An FAO Perspective (ed. Bruinsma, J.) 331–355 (Food and Agriculture Organization, 2003).
Hobbs, P. R., Sayre, K. & Gupta, R. The role of conservation agriculture in sustainable agriculture. Phil. Trans. R. Soc. Lond. B 363 , 543–555 (2008).
Chan, K. Y. An overview of some tillage impacts on earthworm population abundance and diversity — Implications for functioning of soils. Soil Till. Res. 57 , 179–191 (2001).
Decaëns, T. & Jiménez, J. J. Earthworm communities under an agricultural intensification gradient in Colombia. Plant Soil 240 , 133–143 (2002).
Bradley, R. L., Chroňáková, A., Elhottová, D. & Šimek, M. Interactions between land-use history and earthworms control gross rates of soil methane production in an overwintering pasture. Soil Biol. Biochem. 53 , 64–71 (2012).
Contreras-Ramos, S. M., Alvarez-Bernal, D., Montes-Molina, J. A., van Cleemput, O. & Dendooven, L. Emission of nitrous oxide from hydrocarbon contaminated soil amended with waste water sludge and earthworms. Appl. Soil Ecol. 41 , 69–76 (2009).
Speratti, A. B. & Whalen, J. K. Carbon dioxide and nitrous oxide fluxes from soil as influenced by anecic and endogeic earthworms. Appl. Soil Ecol. 38 , 27–33 (2008). After an experimental period of 28 days, earthworms were responsible for 7–58% of the total CO 2 flux from soil, compared to the control (no earthworms), but did not affect the N 2 O flux. Species-specific stimulation of nitrifiers and denitrifiers may be related to unique structures (casts, burrows) produced by L. terrestris and A. caliginosa , but this remains to be confirm ed.
Binet, F. et al. Significance of earthworms in stimulating soil microbial activity. Biol. Fert. Soils 27 , 79–84 (1998).
Butenschoen, O., Ji, R., Schaffer, A. & Scheu, S. The fate of catechol in soil as affected by earthworms and clay. Soil Biol. Biochem. 41 , 330–339 (2009).
Hedde, M., Bureau, F., Akpa-Vinceslas, M., Aubert, M. & Decaëns, T. Beech leaf degradation in laboratory experiments: Effects of eight detritivorous invertebrate species. Appl. Soil Ecol. 35 , 291–301 (2007).
Martin, A. Short- and long-term effects of the endogeic earthworm Millsonia anomala (Omodeo) (Megascolecidæ, Oligochæta) of tropical savannas, on soil organic matter. Biol. Fert. Soils 11 , 234–238 (1991).
Fragoso, C. et al. Agricultural intensification, soil biodiversity and agroecosystem function in the tropics: The role of earthworms. Appl. Soil Ecol. 6 , 17–35 (1997).
Velthof, G., Kuikman, P. & Oenema, O. Nitrous oxide emission from soils amended with crop residues. Nutr. Cycl. Agroecosyst. 62 , 249–261 (2002).
Nebert, L. D., Bloem, J., Lubbers, I. M. & van Groenigen, J. W. Association of earthworm-denitrifier interactions with increased emissions of nitrous oxide from soil mesocosms amended with crop residue. Appl. Environ. Microbiol. 77 , 4097–4104 (2011).
Fonte, S. J. & Six, J. Earthworms and litter management contributions to ecosystem services in a tropical agroforestry system. Ecol. Appl. 20 , 1061–1073 (2010).
Cortez, J. & Bouche, M. B. Do earthworms eat living roots? Soil Biol. Biochem. 24 , 913–915 (1992).
Scheu, S. Effects of earthworms on plant growth: Patterns and perspectives: The 7th international symposium on earthworm ecology. Pedobiologia 47 , 846–856 (2003).
Brown, G. et al. in Earthworm Management in Tropical Agroecosystems (eds Lavelle, P., Brussaard, L. & Hendrix, P. F.) 87–147 (CAB International, 1999).
Eisenhauer, N. & Scheu, S. Earthworms as drivers of the competition between grasses and legumes. Soil Biol. Biochem. 40 , 2650–2659 (2008).
Laossi, K-R. et al. Effects of an endogeic and an anecic earthworm on the competition between four annual plants and their relative fecundity. Soil Biol. Biochem. 41 , 1668–1673 (2009).
Barot, S., Ugolini, A. & Brikci, F. B. Nutrient cycling efficiency explains the long-term effect of ecosystem engineers on primary production. Funct. Ecol. 21 , 1–10 (2007).
Laossi, K.-R., Noguera, D. C., Decäens, T. & Barot, S. The effects of earthworms on the demography of annual plant assemblages in a long-term mesocosm experiment. Pedobiologia 54 , 127–132 (2011).
Burtelow, A. E., Bohlen, P. J. & Groffman, P. M. Influence of exotic earthworm invasion on soil organic matter, microbial biomass and denitrification potential in forest soils of the northeastern United States. Appl. Soil Ecol. 9 , 197–202 (1998).
Marhan, S., Langel, R., Kandeler, E. & Scheu, S. Use of stable isotopes ( 13 C) for studying the mobilisation of old soil organic carbon by endogeic earthworms (Lumbricidae). Eur. J. Soil Biol. 43 , S201–S208 (2007).
Curry, J. P. & Schmidt, O. The feeding ecology of earthworms — A review. Pedobiologia 50 , 463–477 (2007).
Frelich, L. E. et al. Earthworm invasion into previously earthworm-free temperate and boreal forests. Biol. Invasions 8 , 1235–1245 (2006).
Crooks, J. A. Characterizing ecosystem-level consequences of biological invasions: The role of ecosystem engineers. Oikos 97 , 153–166 (2002).
Hale, C., Frelich, L., Reich, P. & Pastor, J. Effects of European earthworm invasion on soil characteristics in northern hardwood forests of Minnesota, USA. Ecosystems 8 , 911–927 (2005).
Tylianakis, J. M., Didham, R. K., Bascompte, J. & Wardle, D. A. Global change and species interactions in terrestrial ecosystems. Ecol. Lett. 11 , 1351–1363 (2008).
Timmerman, A., Bos, D., Ouwehand, J. & de Goede, R. G. M. Long-term effects of fertilisation regime on earthworm abundance in a semi-natural grassland area. Pedobiologia 50 , 427–432 (2006).
Borken, W., Grundel, S. & Beese, F. Potential contribution of Lumbricus terrestris L. to carbon dioxide, methane and nitrous oxide fluxes from a forest soil. Biol. Fert. Soils 32 , 142–148 (2000).
Hedges, L. V. & Olkin, I. Statistical Methods for Meta-analysis (Academic, 1985).
Rosenberg, M. S. B., Adams, D. C. & Gurevitch, J. Metawin: Statistical Software for Meta-Analysis Version 2.0. (Sinauer Associates, 2000).
Tianxiang, L., Huixin, L., Tong, W. & Feng, H. Influence of nematodes and earthworms on the emissions of soil trace gases (CO2, N2O). Acta Ecol. Sin. 28 , 993–999 (2008).
Speratti, A., Whalen, J. & Rochette, P. Earthworm influence on carbon dioxide and nitrous oxide fluxes from an unfertilized corn agroecosystem. Biol. Fert. Soils 44 , 405–409 (2007).
Marhan, S., Rempt, F., Hogy, P., Fangmeier, A. & Kandeler, E. Effects of Aporrectodea caliginosa (Savigny) on nitrogen mobilization and decomposition of elevated CO2 Charlock mustard litter. J. Plant Nutr. Soil Sci. 173 , 861–868 (2010).
Bertora, C., van Vliet, P. C. J., Hummelink, E. W. J. & van Groenigen, J. W. Do earthworms increase N2O emissions in ploughed grassland? Soil Biol. Biochem. 39 , 632–640 (2007).
Janzen, H. H. The soil carbon dilemma: Shall we hoard it or use it? Soil Biol. Biochem. 38 , 419–424 (2006). In his 'Points of view', Janzen considers ways to increase C inputs to soil; seeking to optimize the timing of decay; and to gain a better understanding — from an ecosystem perspective — of the flows of C, rather than just the stocks. He suggests that now, when we aim to regain some of the C lost, we may need new ways of thinking about soil C dynamics, and tuning them for the services expected of our ecosystems.
Barois, I., Villemin, G., Lavelle, P. & Toutain, F. Transformation of the soil structure through Pontoscolex corethrurus (Oligochaeta) intestinal tract. Geoderma 56 , 57–66 (1993).
Jongmans, A. G., Pulleman, M. M. & Marinissen, J. C. Y. Soil structure and earthworm activity in a marine silt loam under pasture versus arable land. Biol. Fert. Soils 33 , 279–285 (2001).
Pulleman, M. M., Six, J., Uyl, A., Marinissen, J. C. Y. & Jongmans, A. G. Earthworms and management affect organic matter incorporation and microaggregate formation in agricultural soils. Appl. Soil Ecol. 29 , 1–15 (2005).
Ruz-Jerez, B. E., Ball, P. R. & Tillman, R. W. Laboratory assessment of nutrient release from a pasture soil receiving grass or clover residues, in the presence or absence of Lumbricus rubellus or Eisenia fetida . Soil Biol. Biochem. 24 , 1529–1534 (1992).
Bouché, M. B. Strategies lombriciennes. Ecol. Bull. 25 , 122–132 (1977).
Didden, W. A. M. Earthworm communities in grasslands and horticultural soils. Biol. Fert. Soils 33 , 111–117 (2001).
Hodge, A., Robinson, D. & Fitter, A. Are microorganisms more effective than plants at competing for nitrogen? Trends Plant Sci. 5 , 304–308 (2000).
Download references
Acknowledgements
This study was supported by a personal VIDI grant from the Netherlands Organization for Scientific Research/Earth and Life Sciences (NWO-ALW) to Jan Willem van Groenigen. We thank Olaf Butenschoen and Bruce A. Snyder for providing standard deviations of their published data for our meta-analysis. We are grateful to Gerlinde De Deyn for giving helpful comments on a previous version of the manuscript.
Author information
Authors and affiliations.
Department of Soil Quality, Wageningen University, PO Box 47, Wageningen, 6700AA, the Netherlands
Ingrid M. Lubbers, Lijbert Brussaard & Jan Willem van Groenigen
Department of Biological Sciences, Northern Arizona University, Flagstaff, 86011, Arizona, USA
Kees Jan van Groenigen
Tropical Soil Biology and Fertility Program (Latin American and Caribbean Region) International Center for Tropical Agriculture (CIAT), Unidad Suelos, Apartado Aereo, Cali, 6713, Colombia
Steven J. Fonte
Department of Plant Sciences, University of California, Davis, 95616, California, USA
You can also search for this author in PubMed Google Scholar
Contributions
IML and JWVG conceived and designed the study, with suggestions and input from SJF and JS; IML extracted the data from the literature and constructed the database; IML and KJVG performed the statistical analysis; IML, JWVG, KJVG, SJF, JS and LB interpreted and discussed the results; IML, JWVG and KJVG wrote the paper, with substantial contributions from all co-authors; JWVG had the overall supervision of the project.
Corresponding author
Correspondence to Ingrid M. Lubbers .
Ethics declarations
Competing interests.
The authors declare no competing financial interests.
Supplementary information
Supplementary information.
Supplementary Information (PDF 442 kb)
Rights and permissions
Reprints and permissions
About this article
Cite this article.
Lubbers, I., van Groenigen, K., Fonte, S. et al. Greenhouse-gas emissions from soils increased by earthworms. Nature Clim Change 3 , 187–194 (2013). https://doi.org/10.1038/nclimate1692
Download citation
Received : 12 April 2012
Accepted : 23 August 2012
Published : 03 February 2013
Issue Date : March 2013
DOI : https://doi.org/10.1038/nclimate1692
Share this article
Anyone you share the following link with will be able to read this content:
Sorry, a shareable link is not currently available for this article.
Provided by the Springer Nature SharedIt content-sharing initiative
This article is cited by
The diversity and abundance of soil macrofauna under different agroforestry practices in the drylands of southern ethiopia.
- Nebiyou Masebo
- Emiru Birhane
- Agena Anjulo
Agroforestry Systems (2024)
Short-Term Effects of Earthworms (Eisenia andrei) on Soil CO2 Emission during Autumn in a Temperate Deciduous Forest
Journal of Soil Science and Plant Nutrition (2024)
Optimizing biochar addition for vermicomposting: a comprehensive evaluation of earthworms’ activity, N2O emissions and compost quality
Biochar (2023)
Earthworms enhance the inhibition efficiency of 3,4-dimethylpyrazole phosphate on soil nitrification by altering soil AOB communities and gut denitrifier communities
- Bing-Jie Jin
- Xi-Peng Liu
- Xian-Yong Lin
Biology and Fertility of Soils (2023)
Deciphering waste bound nitrogen by employing psychrophillic Aporrectodea caliginosa and priming of coprolites by associated heterotrophic nitrifiers under high altitude Himalayas
- Tahir Sheikh
- Zahoor Baba
- Mohammad Javed Ansari
Scientific Reports (2022)
Quick links
- Explore articles by subject
- Guide to authors
- Editorial policies
Sign up for the Nature Briefing: Microbiology newsletter — what matters in microbiology research, free to your inbox weekly.


An official website of the United States government
The .gov means it’s official. Federal government websites often end in .gov or .mil. Before sharing sensitive information, make sure you’re on a federal government site.
The site is secure. The https:// ensures that you are connecting to the official website and that any information you provide is encrypted and transmitted securely.
- Publications
- Account settings
Preview improvements coming to the PMC website in October 2024. Learn More or Try it out now .
- Advanced Search
- Journal List
- Scientific Reports

Earthworms increase plant production: a meta-analysis

Jan Willem van Groenigen
1 Department of Soil Quality, Wageningen University, PO Box 47, 6700AA Wageningen, The Netherlands
Ingrid M. Lubbers
Hannah m. j. vos, george g. brown.
2 CNPq fellow, Embrapa Forestry, CEP 83411-000, Colombo, PR, Brazil
Gerlinde B. De Deyn
Kees jan van groenigen.
3 Center for Ecosystem Science and Society, Northern Arizona University, Flagstaff, Arizona 86011, USA
Associated Data
To meet the challenge of feeding a growing world population with minimal environmental impact, we need comprehensive and quantitative knowledge of ecological factors affecting crop production. Earthworms are among the most important soil dwelling invertebrates. Their activity affects both biotic and abiotic soil properties, in turn affecting plant growth. Yet, studies on the effect of earthworm presence on crop yields have not been quantitatively synthesized. Here we show, using meta-analysis, that on average earthworm presence in agroecosystems leads to a 25% increase in crop yield and a 23% increase in aboveground biomass. The magnitude of these effects depends on presence of crop residue, earthworm density and type and rate of fertilization. The positive effects of earthworms become larger when more residue is returned to the soil, but disappear when soil nitrogen availability is high. This suggests that earthworms stimulate plant growth predominantly through releasing nitrogen locked away in residue and soil organic matter. Our results therefore imply that earthworms are of crucial importance to decrease the yield gap of farmers who can't -or won't- use nitrogen fertilizer.
Our global food production system faces the unprecedented challenge of feeding a rapidly increasing world population while simultaneously reducing its environmental footprint 1 . It is still far from clear whether such a “sustainable intensification” 2 can be achieved. In particular, the question of what determines the yield gap between more sustainable forms of agriculture and those of conventional agriculture, and how to close that gap, is still widely debated 3 , 4 . Earthworms are generally thought to be essential to sustainable agroecosystems. They rank among the most important soil fauna, and as ‘ecosystem engineers’ they are instrumental to several ecosystem services the soil provides, such as nutrient cycling, drainage, and regulating greenhouse gas emissions 5 , 6 . However, it is their supposed ability to stimulate crop growth that might be of foremost relevance to agriculture. This ability was already suggested in an age before artificial fertilizers and mechanization provided a short-cut towards higher crop production 7 , 8 .
Although positive effects of earthworms on plant growth have been repeatedly described 9 , 10 , quantitative proof has remained elusive, and mechanisms through which it might be exerted have never been satisfactorily established. Yet, this information is essential to determine whether earthworms can help to fill the yield gap between sustainable and conventional agriculture. Such an effort has previously been hampered by the wide variety of conditions (climate, soil fertility, crop types, earthworm species and farm management) under which the effects of earthworms have been studied, making it difficult to determine the global effect of earthworms response from individual observations. A quantitative synthesis of results across multiple studies can overcome this problem. Here, for the first time, we use meta-analysis to summarize the effect of earthworms on plant production across the globe (see Methods). We collected 462 data points from 58 studies that were published between 1910–2013 ( Supplementary Table 1 ). Studies include the three main global staple crops (maize, rice and wheat), pastures, as well as many other food crops and were conducted on all continents except Antarctica.
We assessed the generality of the effect of earthworm presence on six key plant response variables: (1) crop yield; (2) aboveground biomass; (3) belowground biomass; (4) total biomass; (5) shoot/root ratio (as a proxy for carbon allocation towards harvestable products); and (6) nitrogen (N) concentration in aboveground biomass (as a proxy for crop quality) ( Supplementary Tables 2–5 ). Earthworm presence significantly increased crop yield by +25%, aboveground biomass by +23%, belowground biomass by +20% and total biomass by +21% ( Fig. 1 ). Shoot/root ratio was not significantly different from zero, indicating no proof of altered carbon allocation towards aboveground plant parts 11 . Nitrogen concentration in aboveground biomass was also unaffected by earthworm presence ( Fig. 1 ), indicating that crop quality was maintained.

Effect sizes in all meta-analyses were weighted by the inverse of the pooled variance. The number of observations in each class is shown between parentheses; error bars denote the 95% confidence range.
Because previous studies suggested that the effect of earthworms differs between crop types 9 , we tested the effect of earthworm presence on aboveground biomass of the major grain crops and ryegrass. Aboveground biomass was significantly increased in all crops ( Fig. 2a ), averaging +31% across all grain crops, and +24% across all pasture grasses ( Fig. 2b ).

Influence of crop species (a), type of crop/grasses (b) and type of pasture (c) on the effect of earthworm presence. Effect sizes in all meta-analyses were weighted by the inverse of the pooled variance. The number of observations in each class is shown between parentheses; error bars denote the 95% confidence range.
How do earthworms stimulate plant production? Brown et al . 12 proposed 5 possible pathways through which earthworm can positively affect plant growth: (i) biocontrol of pests and diseases; (ii) stimulation of microbial plant symbionts; (iii) production of plant-growth regulating substances; (iv) soil structure changes; and (v) increased nutrient availability. The last two mechanisms were the most consistently mentioned in early literature 7 . More recent studies suggested earthworm-induced regulation of plant defence mechanisms as additional pathways 13 , 14 , 15 .
However, our results suggest that increased N availability is a dominant pathway by which earthworms stimulate plant growth. We tested this by splitting our data according to fertilizer N application rates ( Fig. 3a ). When application rates exceeded 30 kg N ha −1 yr −1 , the earthworm effect decreased from +19% to +9% and was not significantly different from zero anymore, suggesting that earthworms stimulate plant growth by increasing N mineralization. The effect of earthworms on plant growth in studies applying organic (N) fertilizer (+34%) was significantly stronger than in studies applying inorganic fertilizer (+10%) or no fertilizer (+20%), further implicating increased N mineralization as a major pathway ( Fig. 4 ). Our results are consistent with studies showing the potential of earthworms to increase N mineralization from crop residues and soil organic matter, depending on earthworm community and environmental conditions 16 , 17 .

Influence of fertilizer N rate (a) and crop residue application rate (b) on the effect of earthworm presence. N fertilization rates include both chemical and organic (manure) fertilizer. Effect sizes in all meta-analyses were weighted by the inverse of the pooled variance. The number of observations in each class is shown between parentheses; error bars denote the 95% confidence range.

The number of observations in each class is shown between parentheses; error bars denote the 95% confidence range.
If N mineralization is the main pathway, the positive effect of earthworms should be smaller for plants capable of symbiotic N 2 fixation. Indeed, for the legume crops in our dataset the earthworm effect ceased to be significant ( Fig. 2b ). Furthermore, when legumes were present in pastures, the effect of earthworms on pasture productivity disappeared altogether ( Fig. 2c ).
It is still unclear whether there is any effect of earthworms on nutrients other than N. Although it has been suggested that earthworms increase P availability in their casts 18 , 19 , this has not yet been shown to affect plant growth in experimental studies. Legumes, despite their larger need for P than grasses, did not show a positive effect of earthworm presence ( Fig. 2b & 2c ), which is consistent with a minor role for earthworms on P mobilization.
Both soil organic matter and plant residues can potentially serve as substrates for N mineralization facilitated by earthworms 17 . To distinguish between the two, we subdivided our dataset in different residue application rate classes ( Fig. 3b ). Although the earthworm effect on aboveground biomass peaked with +50% at the highest residue application rate, the effect stabilized around +21% at nil and very low residue application rates. This indicates that both soil organic matter and plant residue are sources for earthworm-induced mineralization. Because it has been often suggested that decaying earthworm tissues may be responsible for the observed increased plant N uptake 20 , we tested for the effect of earthworm survival ( Fig. 5a ). Earthworm presence did not significantly increase crop yield in experiments with survival rates lower than 50%; therefore the N effect is not an artefact related to decomposing earthworm tissue. This is in line with calculations on the contributions of N released from decaying earthworm tissue in previous experimental studies 21 , as well as with studies conducted with control treatments receiving dead earthworms 22 .

Influence of earthworm survival during experiment (a), earthworm density (b) earthworm ecological group (c) on the effect of earthworm presence. The number of observations in each class is shown between parentheses; error bars denote the 95% confidence range.
Although earthworm density had a highly significant effect on aboveground plant biomass, only the highest densities (>400 individuals m −2 ) differed significantly from lower densities ( Fig. 5b ). The effect under realistic earthworm densities varied between +10 and +21%. The positive effect was present for all three ecological categories of earthworms that are traditionally distinguished ( Fig. 5c ).
In experiments where soil was disturbed (that is, homogenized and repacked) prior to the start of the experiment, the earthworm effect on aboveground biomass was almost twice as high as in undisturbed soils ( Fig. 4 ). This result may reflect a beneficial effect of earthworms on restoring the demolished soil structure. As soil structure will be restored after some time, a positive effect of earthworms on plant growth through their effect on soil structure is likely to be a transient effect after soil tillage operations ( Fig. 4 ). Although some studies reported an additional effect of earthworms on plant growth through improving soil structure in undisturbed soil 22 , 23 , it generally was difficult to distinguish this effect from increased nutrient availability 21 . The fact that all three ecological earthworm categories (anecic, epigeic and endogeic), each with distinct burrowing and casting behaviour 23 , 24 , had a positive effect on plant growth also argues against soil structure improvement as a major pathway, and in favour of enhanced nutrient mineralisation.
Significant positive earthworm effects occur across a range of climate regions, soil textures, soil organic matter contents and soil C/N ratios ( Fig. 4 ). No significant differences were detected between categories of soil organic matter content, possibly because soil organic matter quality was not taken into account. In higher pH soils, the earthworm effect is significantly smaller than in lower pH soils. This may be a confounding effect, since the high pH soils were often linked to systems with low or nil application of residues or organic manure. Earthworm effects were strongest in soils with clay texture and not significant in sandy soils ( Fig. 4 ). This is in marked contrast with an earlier review 9 where largest beneficial earthworm effects in tropical regions were achieved on soils with sandy texture.
Which cropping systems would benefit most from earthworms? Because improving N supply in N-limited systems is the main pathway through which earthworms increase plant growth, earthworms are likely to be most beneficial in infertile soils. However, this raises a paradox, because earthworms thrive best in fertile soils with high soil organic matter levels. The paradox disappears in the case of relatively poor soils that depend on crop residue application to maintain soil fertility levels. In those soils, crop residues can serve as food for earthworms and earthworms can increase crop production through increasing N mineralization. This combination of poor soils and reliance on crop residue is particularly found in low-input farming systems in the tropics, and to a lesser extent in organic farming systems in the developed world 25 .
Yet, these systems vary dramatically in terms of habitat quality for earthworms 26 . In low-input systems in the tropics, low residue quality and residue supply are most likely to be the constraining factor for reaching the full potential of earthworm activity 27 . Research in these systems should therefore be aimed at judicious use of the limited residue resources available 28 . Organic farming systems, on the other hand, typically have large application rates of organic manure or high-quality crop residues, providing excellent conditions for earthworm activity 29 . In those systems, earthworm activity might be crucial in closing the yield gap with conventional agriculture 4 . Future research in these systems should therefore focus on management strategies to increase earthworm populations. More generally speaking, management practices aimed at sustainable intensification of agriculture should take into account their effect on the earthworm population, because their presence stimulates crop production and will help to bridge the yield gap with conventional agriculture.
Data compilation
A literature search of peer-reviewed publications published before June 2013 reporting results on the influence of earthworms on plant growth was performed using the ISI-Web of Science research database. We investigated the effect of earthworms on six main response variables concerning plant growth: crop yield, aboveground biomass, belowground biomass, total biomass, shoot/root ratio and N concentration of the aboveground biomass. We used the following search term:
earthworm$ AND (plant biomass OR plant yield OR plant production OR plant growth) AND (crop$ OR grassland$)
We selected the timespan ‘all years’. When we found references in these papers to peer-reviewed publications that were too old to be included in the ISI database, we included them as well when they fitted our selection criteria. We included primary studies in agroecosystems in either temperate/continental or tropical/subtropical climate zones. For annual plants we included studies that reported plant harvest data after a clearly defined experimental period; in the case of multiple harvests over a longer time span of one or more years, we estimated the experimental period for each harvest separately. For perennial plants we included studies that reported harvest data after an explicitly reported experimental period; in the case of an experimental period of multiple years, we expressed harvest data as annual yield.
After carefully checking all of the query results, a total of 58 papers published between 1910 and 2013 fitted our selection criteria for the meta-analysis ( Supplementary Table 1 ). The resulting database covered 130 side by side comparisons of soils with and without earthworms (observations) from 16 papers for crop yield, 378 comparisons from 52 papers for aboveground biomass, 201 comparisons from 35 papers for belowground biomass, 169 comparisons from 27 papers for total biomass, 173 comparisons from 29 papers for shoot/root ratio, and 71 comparisons from 12 papers for the N concentration of the aboveground biomass.
For each observation within every study we collected the means of the control treatment (that is, without earthworm presence) and the experimental treatment (that is, with earthworm presence), as well as their standard deviation (SD) and replicate numbers (n). Field studies that had earthworms excluded from their control treatment (for instance, by electro-shocking) were only included when explicitly reported earthworm numbers from these control treatments did not exceed 10% of the earthworm densities in experimental treatments. For studies that did not report SD or SE (standard error; SD = SE × √n) we calculated the average coefficient of variation (CV) for control treatments and experimental treatments, and then approximated the missing SD by multiplying the reported mean by the average CV 30 , 31 . When data in the original publication were presented graphically, we estimated values from manually digitized figures. Unidentified error bars were, again conservatively, assumed to denote SE rather than SD. In a few cases, we contacted the authors to obtain unpublished SDs (see Acknowledgements).
For the earthworm effect on plant growth, we considered three groups of controlling factors: plant factors, earthworm factors, and experimental factors. Table 1 lists the three groups of controlling factors, as well as the subgroups we identified for our analysis that were based on these factors. Factors such as land management strategy, soil moisture content or phosphorus uptake by the plants were also considered, but the range of these parameters published across studies was too narrow to be included in our meta-analysis. For the plant factors we distinguished between most commonly studied crops (that is, ryegrass, barley, maize, wheat, rice), as well as groups of crops (that is, grasses, grain crops, legumes). In order to discern the effect of symbiotic N fixation, pastures were divided into pastures with or without legumes. For the earthworm factors we distinguished between the three earthworm ecological categories (that is, anecic, epigeic and endogeic) that are typically distinguished 32 , and a fourth subgroup encompassing studies on mixtures of these categories.
Controlling factors | Unit | Subgroups | |||
---|---|---|---|---|---|
Individual crops/grasses | Ryegrass | Barley | Maize | Wheat Rice | |
Groups of crops/grasses | Grasses | Grain crops | Legumes | ||
Pasture types | Without legumes | With legumes | |||
Ecological group | Epigeic | Endogeic | Anecic | Mixture | |
Density | # m | <100 | 100–200 | 200–400 | >400 |
Survival | % | >90 | 50–90 | <50 | |
Climate | Temperate/Continental | Tropical/Subtropical | |||
Soil texture | Sandy | Loamy | Clayey | ||
Soil organic C content | g C kg soil | <15 | 15–30 | >30 | |
Soil C/N ratio | <12.5 | > = 12.5 | |||
Soil pH | <5.6 | 5.6–7.0 | >7.0 | ||
Soil pre-treatment | Disturbed | Undisturbed | |||
N fertilizer type | Inorganic | Organic | Both | None | |
Fertilizer application rate | kg N ha yr | < = 30 | >30 | ||
Residue application rate | kg C ha yr | <1000 | 1000–2999 | 3000–6000 | >6000 |
Earthworm densities were divided into four subgroups, representing low, intermediate, high and very high densities. These were based on the range of densities that can be found in agroecosystems throughout the world, including arable fields and pastures in tropical and subtropical 33 and temperate regions 34 , as well as artificial densities generally only employed in experiments.
We also categorized studies according to earthworm survival rate, distinguishing between <50%, 50–90% and >90% survival. For experimental factors we distinguished between temperate/continental and tropical/subtropical climates. Soil texture of the bulk soil used in the included studies was categorized in three subgroups (sandy, loamy, clayey) according to textural classes as defined by the USDA 35 . We divided studies into three subgroups based on soil organic carbon content (<15 g C kg −1 , 15–30 g C kg −1 , >30 g C kg −1 ). Studies were split into two subgroups according to critical soil C/N ratios within the context of N mineralization and immobilization, as described by Hodge et al. 36 (<12.5 and ≥12.5). Studies were categorized in three subgroups of soil pH (<5.6, 5.6–7.0, >7.0) based on earlier work on the effect of earthworms on plant growth 9 . Soil pre-treatment was taken into account by dividing the studies in two subgroups: disturbed (re-packed soil) and undisturbed (intact soil columns or field plots). Within undisturbed soil we further distinguished between experiments where treatments were installed by applying earthworms to earthworm treatments (indicating an absence of a significant native earthworm population) and those where treatments were installed by reducing earthworm numbers in control treatments (indicating a significant earthworm population that might have affected soil properties prior to the experiment). We distinguished between four types of N fertilizer application (inorganic, organic, both and none) and two fertilizer application rates (≤30 kg N ha −1 yr −1 and >30 kg N ha −1 yr −1 ), the cut-off value being determined by maximum atmospheric N depositions in the United States and most of the European Union, following Van Groenigen et al . 37 . Finally, we divided studies into four subgroups of residue application rates (0 kg C ha −1 yr −1 , 0–2999 kg C ha −1 yr −1 , 3000–5999 kg C ha −1 yr −1 , ≥6000 kg C ha −1 yr −1 ). These represent the lower and upper spectrum of residue application rates in agroecosystems, ranging from systems where most of the residues are removed ( e.g. for biofuel production or construction), via relatively low residue application rates typical of low input systems in developing countries, and high residue application rates typical for high input systems in the developed world, to crops producing large amounts of residues (for instance, sugarcane) 38 .
Most studies comprised several treatments with and without the presence of earthworms, resulting in more than one observation per study. Not all studies provided information on each controlling factor and therefore the number of observations per controlling factor was not always identical to the total number of observations. Results from subgroups of the controlling factors were considered suitable for meta-analysis when a minimum of 10 observations out of at least two independent studies were available.
Meta-analysis
The magnitude of the earthworm-induced effect on the six main response variables in each study was calculated as the natural logarithm of the response ratio (R) 39 :

where E and C are the means of experimental and control groups, respectively. Response ratios that were either more than five standard deviations above or below the mean were considered outliers and not included in further calculations.
Because the results of a meta-analysis may depend on how individual studies are weighted 40 , we used one parametric and three different non-parametric weighting functions in our analyses. For every observation, weights were calculated by using the following functions:

In the parametric meta-analysis (that is, using weight #1), each individual observation was weighted by the reciprocal of the mixed-model variance, which was the sum of the variance of the natural log of the response ratio and the pooled within-class variance. We calculated 95% confidence intervals (CIs) of the mean effect sizes according to Hedges and Olkin 41 . To test whether controlling factors altered the effect of earthworm presence, the data were divided into subgroups as described above. To test whether mean effect sizes differed between subgroups, we used the approach by Curtis and Wang 42 . Briefly, the total heterogeneity (Qt) was partitioned into within-class heterogeneity (Qw) and between class heterogeneity (Qb). Data were then subdivided according to levels of those categorical variables revealing significant Qb values ( Supplementary Tables 2–5 ).
For the non-parametric analyses (that is, weights #2–4), we generated mean effect sizes and 95% CIs by running a bootstrapping procedure with 5000 iterations. The results for the analyses on ln R (mean effects and CIs) were back-transformed and reported as percentage change of the earthworm effect ([ R -1]*100) to ease interpretation. For both the non-parametric and the parametric analyses, the mean earthworm effect was considered significant when the 95% confidence interval did not overlap with 0. Mean earthworm effects for different subgroups were considered to be significantly different from one another if their 95% confidence intervals did not overlap. For the parametric analyses, both the heterogeneity test had to indicate significance and the 95% CIs of study categories had to show no overlap for us to conclude that a categorical variable had a significant impact on the earthworm effect.
The potential for publication bias in this quantitative review was examined through a normal quantile plot. Normal quantile plots were produced for two main response variables, crop yield and aboveground biomass, because the results of this review are mainly based on analyses of these two response variables ( Supplementary Fig. 1 & 2 ). Additionally, Rosenthal's method for calculation of a fail-safe number was added to the plots, indicating the number of non-significant, unpublished, or missing studies that would need to be added to the meta-analysis to change the results from significant to nonsignificant 43 . Both normal quantile plots as well as the fail-safe numbers suggest that our observed results can be treated as a reliable estimate of the true effect. All analyses were performed in METAWIN 2.1 44 .
Supplementary Material
Acknowledgments.
We thank Michael Bonkowski, Nico Eisenhauer, Patrick Lavelle, Olaf Schmidt, and Nicole Schon for providing us with extra data on their published experiments. We are grateful to Ron de Goede for help in collecting the data, and to Lijbert Brussaard for commenting on an earlier version of this manuscript. This study was funded through a personal VIDI grant from the Netherlands Organization for Scientific Research/Earth and Life Sciences (NWO-ALW) to J.W.v.G. (project number 864.07.004). K.J.v.G. receives financial support from the Office of Science (BER), U.S. Department of Energy. Additional funding was provided by CPNq to G.G.B. and through a VIDI grant from NWO-ALW to G.B.D.D. (project number 864.11.003).
The authors declare no competing financial interests.
Author Contributions J.W.v.G., I.M.L. and K.J.v.G. conceived the study. H.M.J.V. and J.W.v.G. extracted the data from the literature. I.M.L. performed the meta-analysis. G.G.B. and G.B.D.D. contributed to analysis and interpretation of the data. J.W.v.G., I.M.L. and K.J.v.G. wrote the manuscript. All authors critically reviewed the manuscript. J.W.v.G. was overall project leader.
- Godfray H. C. J. et al. Food security: the challenge of feeding 9 billion people . Science 327 , 812–818 (2010). [ PubMed ] [ Google Scholar ]
- Royal Society of London. Reaping the benefits: Science and the sustainable intensification of global agriculture. (Royal Society, London, 2009). [ Google Scholar ]
- Hobbs P. R., Sayre K. & Gupta R. The role of conservation agriculture in sustainable agriculture . Philos. Trans. R. Soc. B-Biol. Sci. 363 , 543–555 (2008). [ PMC free article ] [ PubMed ] [ Google Scholar ]
- Seufert V., Ramankutty N. & Foley J. A. Comparing the yields of organic and conventional agriculture . Nature 485 , 229–U113 (2012). [ PubMed ] [ Google Scholar ]
- Lubbers I. M. et al. Greenhouse-gas emissions from soils increased by earthworms . Nature Clim. Change 3 , 187–194 (2013). [ Google Scholar ]
- Blouin M. et al. A review of earthworm impact on soil function and ecosystem services . Eur. J. Soil Sci. 64 , 161–182 (2013). [ Google Scholar ]
- White G. in The life and letters of Gilbert White of Selborne Vol. 1 (ed Holt-White R.) (John Murray, 1901). [ Google Scholar ]
- Darwin C. The formation of vegetable mould through the action of worms with observations on their habits. (Murray, 1881). [ Google Scholar ]
- Brown G. G. et al. in Earthworm management in tropical agroecosystems (eds Lavelle P., Brussaard L. & Hendrix P. F.), 87–147 (CABI Publishing, 1999). [ Google Scholar ]
- Scheu S. Effects of earthworms on plant growth: patterns and perspectives . Pedobiologia 47 , 846–856 (2003). [ Google Scholar ]
- Poorter H. & Nagel O. The role of biomass allocation in the growth response of plants to different levels of light, CO 2 , nutrients and water: a quantitative review . Aust. J. Plant Physiol. 27 , 595–607 (2000). [ Google Scholar ]
- Brown G. G., Edwards C. A. & Brussaard L. in Earthworm ecology. 2 nd Edition. (ed Edwards C. A.) Ch. 2, 441 (CRC Press, 2004). [ Google Scholar ]
- Jana U. et al. Earthworms influence the production of above- and belowground biomass and the expression of genes involved in cell proliferation and stress responses in Arabidopsis thaliana . Soil Biol. Biochem. 42 , 244–252 (2010). [ Google Scholar ]
- Blouin M. et al. Belowground organism activities affect plant aboveground phenotype, inducing plant tolerance to parasites . Ecol. Lett. 8 , 202–208 (2005). [ Google Scholar ]
- Puga-Freitas R., Barot S., Taconnat L., Renou J. P. & Blouin M. Signal Molecules Mediate the Impact of the Earthworm Aporrectodea caliginosa on Growth, Development and Defence of the Plant Arabidopsis thaliana . PLoS One 7 , 10 (2012). [ PMC free article ] [ PubMed ] [ Google Scholar ]
- Blair J. M., Parmelee R. W., Allen M. F., McCartney D. A. & Stinner B. R. Changes in soil N pools in response to earthworm population manipulations in agroecosystems with different N sources . Soil Biol. Biochem. 29 , 361–367 (1997). [ Google Scholar ]
- Postma-Blaauw M. B. et al. Earthworm species composition affects the soil bacterial community and net nitrogen mineralization . Pedobiologia 50 , 243–256 (2006). [ Google Scholar ]
- Kuczak C. N., Fernandes E. C. M., Lehmann J., Rondon M. A. & Luizão F. J. Inorganic and organic phosphorus pools in earthworm casts (Glossoscolecidae) and a Brazilian rainforest Oxisol . Soil Biol. Biochem. 38 , 553–560 (2006). [ Google Scholar ]
- Le Bayon R. C. & Milleret R. Effects of earthworms on Phosphorus dynamics - a review . Dyn. Soil Dyn. Plant 3 , 21–27 (2009). [ Google Scholar ]
- Whalen J. K., Parmelee R., McCartney D. A. & Vanarsdale J. L. Movement of N from decomposing earthworm tissue to soil, microbial and plant N pools . Soil Biol. Biochem. 31 , 487–492 (1999). [ Google Scholar ]
- Edwards C. A. & Lofty J. R. Effects of earthworm inoculation upon the root-growth of direct drilled cereals . J. Appl. Ecol. 17 , 533–543 (1980). [ Google Scholar ]
- Hopp H. & Slater C. S. Influence of earthworms on soil productivity . Soil Sci. 66 , 421–428 (1948). [ Google Scholar ]
- Spurgeon D. J., Keith A. M., Schmidt O., Lammertsma D. R. & Faber J. H. Land-use and land-management change: relationships with earthworm and fungi communities and soil structural properties . BMC Ecol. 13 , 46 (2013). [ PMC free article ] [ PubMed ] [ Google Scholar ]
- Lavelle P. et al. Soil invertebrates and ecosystem services . Eur. J. Soil Biol. 42 , S3–S15 (2006). [ Google Scholar ]
- Feller C., Blanchart E., Bernoux M., Lal R. & Manlay R. Soil fertility concepts over the past two centuries: the importance attributed to soil organic matter in developed and developing countries . Arch. Agron. Soil Sci. 58 , S3–S21 (2012). [ Google Scholar ]
- Lavelle P., Barois I., Martin A., Zaidi Z. & Schaefer R. in Ecology of arable land: perspectives and challenges (eds Clarholm M. & Bergstrom L.), 109–122 (Kluwer Academic Press, 1989). [ Google Scholar ]
- Lavelle P. et al. SOM management in the tropics: Why feeding the soil macrofauna? Nutr. Cycl. Agroecosyst. 61 , 53–61 (2001). [ Google Scholar ]
- Palm C. A., Gachengo C. N., Delve R. J., Cadisch G. & Giller K. E. Organic inputs for soil fertility management in tropical agroecosystems: application of an organic resource database . Agric. Ecosyst. Environ. 83 , 27–42 (2001). [ Google Scholar ]
- Chan K. Y. An overview of some tillage impacts on earthworm population abundance and diversity - implications for functioning in soils . Soil Till. Res. 57 , 179–191 (2001). [ Google Scholar ]
- Bai E. et al. A meta-analysis of experimental warming effects on terrestrial nitrogen pools and dynamics . New Phytol. 199 , 441–451 (2013). [ PubMed ] [ Google Scholar ]
- Fu R. et al. Handling continuous outcomes in quantitative synthesis. Methods guie for comparative effectiveness reviews. AHRQ Publication No.13-EHC103-EF. (Agency for Healthcare Research and Quality, 2013). [ PubMed ] [ Google Scholar ]
- Bouché M. B. in Soil organisms as components of ecosystems Ecological Bulletins (eds Lohm U. & Persson E.), 122–132 (Swedish Natural Science Research Council, 1977). [ Google Scholar ]
- Fragoso C. et al. in Earthworm management in tropical agroecosystems (eds Lavelle P., Brussaard L. & Hendrix P. F.), 27–55 (CABI, 1999). [ Google Scholar ]
- Didden W. A. M. Earthworm communities in grasslands and horticultural soils . Biol. Fertil. Soils 33 , 111–117 (2001). [ Google Scholar ]
- Soil Survey Staff. Keys to Soil Taxonomy. 8th edn, (United States Department of Agriculture, 1998). [ Google Scholar ]
- Hodge A., Robinson D. & Fitter A. Are miroorganisms more effective than plants at competing for nitrogen? Trends in Plant Science 5 , 304–308 (2000). [ PubMed ] [ Google Scholar ]
- Van Groenigen K. J. et al. Element interactions limit soil carbon storage . Proc. Natl. Acad. Sci. U.S.A. 103 , 6571–6574 (2006). [ PMC free article ] [ PubMed ] [ Google Scholar ]
- Lal R. The role of residues management in sustainable agricultural systems . J. Sustain. Agric. 5 , 51–78 (1995). [ Google Scholar ]
- Hedges L. V., Gurevitch J. & Curtis P. S. The meta-analysis of response ratios in experimental ecology . Ecology 80 , 1150–1156 (1999). [ Google Scholar ]
- Hungate B. A. et al. Assessing the effect of elevated carbon dioxide on soil carbon: A comparison of four meta-analyses . Glob. Change Biol. 15 , 2020–2034 (2009). [ Google Scholar ]
- Hedges L. V. & Olkin I. Statistical Methods for Meta-analysis. (Academic Press, 1985). [ Google Scholar ]
- Curtis P. S. & Wang X. A meta-analysis of elevated CO 2 effects on woody plant mass, form and physiology . Oecologia 113 , 299–313 (1998). [ PubMed ] [ Google Scholar ]
- Rosenthal R. The file drawer problem and tolerance for null results . Psychol. Bull. 86 , 638–641 (1979). [ Google Scholar ]
- Rosenberg M. S. B., Adams D. C. & Gurevitch J. Metawin: Statistical Software for Meta-Analysis, Version 2.0. (Sinauer Associates, 2000). [ Google Scholar ]
Giant 'gurgling' earthworms living under Gippsland farms the focus of new research
By Anne Simmons
ABC Gippsland
Topic: Animal Science
The head of a giant Gippsland earthworm. ( Supplied: Beverley Van Praagh )
A new project will map colonies of endangered giant Gippsland earthworms on farms.
The worms, among the biggest in the world, grow to one metre long and are rarely seen above ground.
What's next?
Researchers want to know more about soil conditions for the species to better protect it.
Under the steep rolling hills of eastern Victoria are "gurgling" creatures farmers can hear but rarely lay eyes on.
"It's kind of a strange little thing, that we're passionate about something we never see,” farm manager Burke Brandon said.
"But still, we know they're there.
"They're kind of like our hidden allies."
The south Gippsland organic beef and lamb farmer is talking about a species of annelid worm called the giant Gippsland earthworm ( Megascolides australis ).
It earns the name "giant" because an average adult is a metre long — making it one of the biggest earthworms in the world.
An adult giant Gippsland earthworm is on average a metre long. ( Supplied: Beverley Van Praagh )
Mr Brandon had heard people talk about them but it was not until a mudslide about 10 years ago that he found them on his property.
"I actually saw a part of a giant earthworm, which was quite a surprise when you actually see how big they are in real life," he said.
"And we have seen cases where there's been accidental erosion or a landslip ... and you can see in the soil profile these hundreds of little holes in the soil the size of your finger."
Part of a giant earthworm Burke Brandon found on his property more than 10 years ago. ( Supplied: Burke Brandon )
Stomp around and listen
The farmer is getting to know where these elusive creatures live on his property at Moyarra, 125 kilometres south-east of Melbourne, as they don’t travel far.
"You know they'll always be roughly in the same area," Mr Brandon said.
Stomping the ground is known as a good way to tell if giant earthworms are around - the ground can gurgle in response. ( ABC News: Anne Simmons )
"The more we stomp around on the surface, the more you hear them moving," Mr Brandon said.
"They feel the vibrations of you walking around, that's when they scurry back into their holes.
"It makes a gurgling sound, like you've let the water out of the bathtub."
Mr Brandon now wants to understand more about protecting the species listed as endangered in Victoria.
He is one of several farmers taking part in a project mapping the sites of local colonies.
Not too wet, not too dry
The species is endemic to west and south Gippsland and is fussy about its underground home.
Dr Beverley Van Praagh has been studying the worms for more than 30 years and describes them as the "Goldilocks of the earthworm world".
"They don’t like it too wet, they don't like it too dry. It has to be just right for them to be able to survive in those clay soils," Dr Van Praagh said.
A burrow of a giant Gippsland earthworm. ( Supplied: Beverley Van Praagh )
The biggest threats to the species are changes in soil conditions, which could come from new housing estates, damming a creek or redirecting a waterway, she said.
Another less obvious threat could be planting trees on top of them with the best intentions.
"When people plant dense vegetation on top of earthworm habitat, it sucks the water out of the soil," she said.
The worms prefer specific soil conditions and are often found in the banks of small creeks. ( Supplied: Beverley Van Praagh )
An idyllic rural home
Most giant Gippsland earthworms are found on private farming land.
A telltale clue the species is lurking under the soil is terracing across hill sides.
Dr Van Praagh said the worms are often found along the banks of small creeks or tributaries or on south or west-facing hill slopes.
Terraces on Mr Brandon's property -- a sign earthworms could live beneath. ( ABC News: Anne Simmons )
But when it comes to the exact soil conditions they like, there are knowledge gaps.
Gippsland Threatened Species Action Group and the Bass Coast and South Gippsland Landcare Networks are leading a new project to better understand these parameters.
It has federal funding under the Saving Native Species Program and will involve mapping colonies on private land and studying the hydrology with probes.
South Gippsland, where the species lives, is known for its picturesque rolling pastures. ( ABC News: Anne Simmons )
Co-existing with worms
The project aims to help farmers understand how to coexist with giant earthworms and still have productive farmland.
Researchers will track soil hydrology over time, looking at changes in moisture, temperature and oxygen.
Mr Brandon hopes to help preserve the species on his property for years.
"Some areas we may fence off so we know we can manage that area differently with grazing," he said.
"There is the potential for those areas to be revegetated with plants that coexist well with the earthworms, so grasses and ferns and sedges and small shrubs, but certainly not eucalyptus trees or deep-rooted trees.
"It's about being aware of how we can incorporate those [earthworm] communities in our whole farm plan, into our long-term future."
Pharmaceutical metabolite identification in lettuce ( Lactuca sativa ) and earthworms ( Eisenia fetida ) using liquid chromatography coupled to high-resolution mass spectrometry and in silico spectral library
- Research Paper
- Open access
- Published: 10 September 2024
Cite this article
You have full access to this open access article
- Jan Fučík ORCID: orcid.org/0000-0002-3408-4383 1 ,
- Stanislav Fučík 2 ,
- Sascha Rexroth 3 ,
- Marian Sedlář 4 ,
- Helena Zlámalová Gargošová 1 &
- Ludmila Mravcová 1
Pharmaceuticals released into the aquatic and soil environments can be absorbed by plants and soil organisms, potentially leading to the formation of unknown metabolites that may negatively affect these organisms or contaminate the food chain. The aim of this study was to identify pharmaceutical metabolites through a triplet approach for metabolite structure prediction (software-based predictions, literature review, and known common metabolic pathways), followed by generating in silico mass spectral libraries and applying various mass spectrometry modes for untargeted LC-qTOF analysis. Therefore, Eisenia fetida and Lactuca sativa were exposed to a pharmaceutical mixture (atenolol, enrofloxacin, erythromycin, ketoprofen, sulfametoxazole, tetracycline) under hydroponic and soil conditions at environmentally relevant concentrations. Samples collected at different time points were extracted using QuEChERS and analyzed with LC-qTOF in data-dependent (DDA) and data-independent (DIA) acquisition modes, applying both positive and negative electrospray ionization. The triplet approach for metabolite structure prediction yielded a total of 3762 pharmaceutical metabolites, and an in silico mass spectral library was created based on these predicted metabolites. This approach resulted in the identification of 26 statistically significant metabolites ( p < 0.05), with DDA + and DDA − outperforming DIA modes by successfully detecting 56/67 sample type:metabolite combinations. Lettuce roots had the highest metabolite count (26), followed by leaves (6) and earthworms (2). Despite the lower metabolite count, earthworms showed the highest peak intensities, closely followed by roots, with leaves displaying the lowest intensities. Common metabolic reactions observed included hydroxylation, decarboxylation, acetylation, and glucosidation, with ketoprofen-related metabolites being the most prevalent, totaling 12 distinct metabolites. In conclusion, we developed a high-throughput workflow combining open-source software with LC-HRMS for identifying unknown metabolites across various sample types.
Graphical Abstract
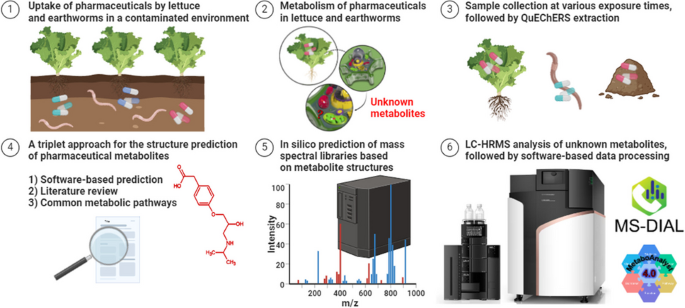
Explore related subjects
- Environmental Chemistry
Avoid common mistakes on your manuscript.
Introduction
Pharmaceuticals play a crucial role in human healthcare and animal husbandry; however, a significant challenge arises as up to 90% of the drug dosage can be excreted in feces or urine. Unfortunately, conventional wastewater treatment plants are not entirely effective in removing these organic micropollutants, leading to their presence in the effluent or sewage sludge. Consequently, these residues can enter the terrestrial environment through various routes, such as irrigation with treated wastewater, application of inadequately treated animal manure, or the use of biosolids in agriculture within the circular economy [ 1 , 2 , 3 ]. The dispersion of these emerging organic contaminants has far-reaching consequences, including adverse effects on soil microbial communities, the emergence of antimicrobial resistance, contamination of the food chain through crop uptake, negative impacts on crop yields (seed germination, biomass growth), and potential degradation of soil quality owing to detrimental effects on soil organisms. Additionally, these xenobiotics may undergo transformation into metabolites within plants or soil organisms. Given the limited understanding of these metabolic pathways, not all drug-related metabolites have been identified [ 2 , 3 , 4 , 5 , 6 , 7 ].
Past studies on xenobiotics have revealed that plants and soil organisms possess the capability to metabolize man-made chemicals through phases I and II. During phase I reactions, the parent compound (or its metabolite) undergoes transformative processes, such as hydrolysis, reduction, or oxidation, enhancing its reactivity and polarity. This enhancement involves the addition of functional groups such as -OH, -NH 2 , or -SH [ 1 ]. During phase II metabolism, xenobiotics undergo conjugation with endogenous molecules, such as acetyl, methyl, and sulfate groups, glucuronic acid, glucose, glutathione, glucopyranosyloxy and malonyl groups, pterin, methyl salicylate, leucyl, glutamic acid, glutamine, and various sugars and amino acids [ 4 , 7 , 8 , 9 , 10 , 11 ]. Moreover, in plants, the occurrence of phase III metabolism, often referred to as the “green liver concept,” is notable. During this phase, conjugates have the ability to bind to insoluble components, such as lignin and polysaccharides, or undergo storage within cell vacuoles, a process known as sequestration [ 1 , 4 , 7 ]. Sequestration of pharmaceutical conjugates may offer an additional mechanism of bioaccumulation, extending beyond passive partitioning into lipids and other phases [ 1 ]. Consequently, the metabolism of plants and soil organisms holds the potential for an “endless” number of drug-related metabolites. This is due to the fact that these reactions, their combinations, and the resulting metabolites of parent substances remain inadequately investigated [ 11 ]. Furthermore, studies emphasize the imperative to identify drug-related metabolites and quantify them alongside parent substances in the environment. This is crucial for a comprehensive assessment of the environmental and potential health risks associated with the dissemination of pharmaceuticals across different environmental compartments [ 1 , 10 ]. Pharmaceutical phytotoxic effects can lead to lower germination rates, reduced root and shoot mass, decreased reproductive rates, chlorosis, oxidative stress, tissue deformation, and altered enzymatic activity. The specific symptoms depend on factors such as contamination concentration, the type of pharmaceutical or metabolite, and their therapeutic class [ 12 , 13 ]. The potential health risks to humans from environmental pharmaceutical residues arise from the unknown adverse effects of long-term exposure to trace amounts of these substances through the contaminated food chain [ 14 ]. While some studies [ 15 , 16 ] have reported minimal health risks from consuming contaminated crops, the research on human risk and exposure remains premature due to a lack of comprehensive studies. Most PhAC metabolites have not been identified or included in these risk assessments. Additionally, these residues can contribute to the spread of antimicrobial resistance within both environmental ecosystems and human populations [ 17 ].
Recent studies [ 4 , 7 , 8 , 9 , 11 , 18 , 19 , 20 , 21 , 22 ] addressing the identification of metabolites (Met-ID) typically employ cell cultures or already pregrown plants for uptake experiments under hydroponic conditions. However, this approach fails to fully mimic real growing conditions. Lettuce ( Lactuca sativa ) is the most commonly used plant in these experiments, although other plant species such as tomato, cucumber, black pepper, onion, maize, amaranth, rice, and pea have also been used. In these experiments, plants were exposed to non-relevant concentrations, ranging from 50 to 1000 times higher than the environmental concentrations, to facilitate the elucidation of the metabolite structure. Consequently, a substantial number of pharmaceutical metabolites are frequently detected. It is noteworthy that these elevated concentrations may induce the formation of metabolites not present at environmentally relevant levels due to their influence on plant metabolism. Commonly tested substances include ibuprofen, diclofenac, trimethoprim, naproxen, triclocarban, and carbamazepine, with contamination typically performed using a single substance per exposure experiment. Plant samples, both contaminated and non-contaminated, were collected at varying exposure intervals, determined by the experimental setup or the plant’s growth rate, spanning from hours to weeks. Root and leaf samples are extracted separately, and liquid chromatography coupled to high-resolution mass spectrometry is the prevailing analytical method. In the initial stages, a literature search for known metabolites is usually conducted, followed by the utilization of commercially available software from mass spectrometry vendors for data processing and identification of these metabolites [ 4 , 7 , 8 , 9 , 11 , 18 , 19 , 20 , 21 , 22 ].
The primary objective of this study was to identify drug-related metabolites in earthworms and lettuce cultivated in both soil and a hydroponic environment after exposure to pharmaceutical contamination. The novelty of this study lies in the conduct of exposure experiments with a mixture of pharmaceuticals at environmentally more relevant concentrations than those in other studies. To achieve this, earthworms and lettuces were subjected to a mixture of six commonly used pharmaceuticals, namely atenolol, enrofloxacin, erythromycin, ketoprofen, sulfamethoxazole, and tetracycline — each representing a distinct pharmaceutical class. Moreover, the design of the study ensured that lettuce was exposed to contamination for almost the entire growth period. A novel study workflow for Met-ID was introduced, as described in detail in the “ Met-ID study workflow ” section of the manuscript. In contrast to certain vendor software, our study employed freely available software with available algorithms, enabling scientific scrutiny and validation of the process: (1) metabolite prediction, (2) in silico spectral library prediction, (3) data processing, and (4) statistical data evaluation. Specifically, our workflow integrated a triplet approach for metabolite structure prediction (combining software prediction, literature search, and common metabolic pathways) with the in silico prediction of MS 2 spectra, a methodology not previously explored in the literature. Additionally, LC-qTOF measurements were conducted in both ESI + and ESI − modes, utilizing both data-dependent acquisition (DDA) and data-independent acquisition (DIA) modes to compare their efficiency in Met-ID.
Materials and methods
Ammonium nitrate (> 99.5%), calcium nitrate tetrahydrate (> 99%), citric acid monohydrate (≥ 99%), disodium hydrogen phosphate dodecahydrate (≥ 99%), ethylenediamine tetraacetic acid (EDTA, ≥ 99%), ferrous sulfate heptahydrate (> 99.7%), hydrochloric acid (35%), potassium nitrate (> 99.9%), sodium sulfate anhydrous (≥ 95%), and sulfuric acid (≥ 96%) were purchased from Lach:ner (Czech Republic). Acetonitrile (LC–MS grade), diethylenetriaminepentaacetic acid (≥ 99%), and methanol (LC–MS grade) were purchased from VWR (Czech Republic). Copper sulfate pentahydrate (> 98%), formic acid (LC–MS grade), manganese sulfate monohydrate (> 99%), sodium chloride (> 99%), sodium molybdate dihydrate (> 99.5%), and zinc sulfate heptahydrate (> 99%) were purchased from Sigma-Aldrich (Germany). Sodium hydroxide (> 98%) and potassium hydroxide (> 98%) were purchased from Penta Chemicals (Czech Republic). Boric acid (> 99.5%), magnesium sulfate heptahydrate (> 99%), and potassium phosphate monobasic (> 99.5%) were purchased from Lachema n.p. (Czech Republic).
The following pharmaceuticals (physicochemical properties in Table S1 ) were purchased from Sigma-Aldrich (Germany): atenolol (≥ 98%), enrofloxacin (≥ 99%), erythromycin (≥ 97%), ketoprofen (≥ 98%), sulfamethoxazole (≥ 98%), and tetracycline (≥ 98%). Individual stock standard solutions for the target pharmaceuticals were prepared in methanol at a concentration of 1 mg∙mL −1 . A standard solution mixture containing all the target compounds was prepared by diluting the individual stock solutions in methanol to achieve a final concentration of 100 μg∙mL −1 . This mixture was subsequently used for spiking in the uptake experiments and for LC–MS standard preparation.
Nylon syringe filters (13 mm, 0.22 μm) were purchased from Chromservis (Czech Republic). For QuEChERS, dispersive SPE (dSPE): DSC-18 SPE and PSA SPE were purchased from Sigma-Aldrich (Germany).
Met-ID study workflow
The outlined workflow (Fig. 1 ) was carefully devised and adhered to throughout this study. Initially, earthworm ( Eisenia fetida ) and lettuce ( Lactuca sativa ) uptake experiments were conducted, followed by QuEChERS extractions. Subsequent LC-ESI-qTOF analyses were performed in both positive and negative ionization modes, utilizing both DDA and DIA modes to assess their efficacy in feature detection. Prior to data processing in MS-DIAL 4.0 software, potential metabolites were predicted through a threefold approach: (1) literature search [ 20 , 23 , 24 , 25 , 26 , 27 , 28 , 29 , 30 , 31 , 32 , 33 , 34 , 35 , 36 , 37 , 38 , 39 , 40 , 41 , 42 , 43 , 44 , 45 , 46 , 47 , 48 ]; (2) software prediction using BioTransformer 3.0 [ 49 ], GLORYx [ 50 ], and enviPath [ 51 ]; and (3) common metabolic pathways [ 4 , 8 , 9 , 18 , 20 , 21 , 36 , 52 , 53 , 54 , 55 , 56 , 57 , 58 , 59 ]. Following this, an in silico MS/MS library for these compounds was generated using CFM-ID 4.0 [ 60 ] for both ESI + and ESI − . These libraries played a crucial role in the subsequent data processing stages within MS-DIAL 4.0 [ 61 ]. Finally, the acquired data were exported and subjected to statistical evaluation using MetaboAnalyst 4.0 [ 62 ] and subsequently reported.
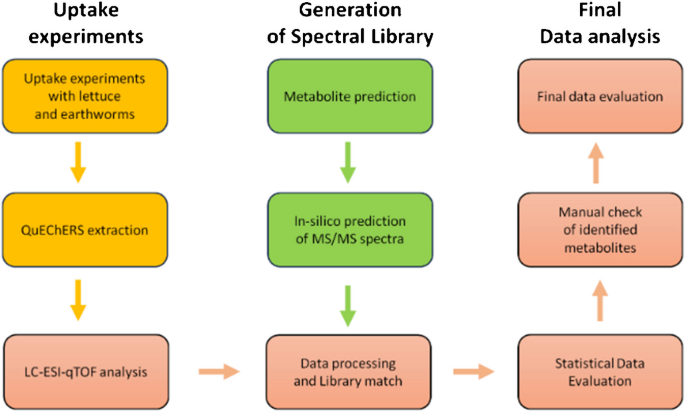
Description of uptake experiments
Lettuce was cultivated and grown under both hydroponic conditions and in soil environments. In both scenarios, the aquatic or soil environment was contaminated from the start of the experiment with a mixture of six pharmaceuticals (atenolol, enrofloxacin, erythromycin, ketoprofen, sulfamethoxazole, and tetracycline), each at a concentration of 10 μg∙L −1 (in hydroponics) or 10 μg∙g −1 (in soil). Concurrently, earthworms were exposed to the same pharmaceutical contamination in the soil environment as the lettuce. Detailed procedures for these uptake experiments are provided in the Supplementary Information—General, Appendix 1 .
QuEChERS sample extraction
Both the lettuce leaves, lettuce roots, and earthworms were extracted separately using our own previously validated and published method [ 63 ], detailed in the Supplementary Information—General, Appendix 2 .
Untargeted analysis using LC-ESI-qTOF
QuEChERS extracts were analyzed by ultra-high-performance liquid chromatography (Shimadzu Nexera X3, Japan) in tandem with quadrupole time of flight (Shimadzu LCMS-9050, Japan) with electrospray ionization (ESI). Chromatographic separation was achieved using a Shim-pack Velox C18 (100 × 2.1 mm, 1.8 µm) column at a flow rate 0.4 mL∙min −1 and temperature of 40 °C. The mobile phases consisted of (A) 0.01% formic acid (FA) in Milli-Q water and (B) MeOH. The elution gradient was 0–1 min, 95% A; 13–14 min, 0% A; and 14–18 min, 95% A. The injection volume was 4 µL for all samples. The qTOF operating parameters were set as follows: interface voltage, 1500 V; interface temperature, 300 °C; desolvation temperature, 250 °C; DL temperature, 250 °C; heat block temperature, 400 °C; nebulizing gas flow, 3 L∙min −1 ; heating gas flow, 10 L∙min −1 ; and drying gas flow, 10 L∙min −1 . For data-dependent analysis (DDA), full scan MS (centroid mode, m/z 60–800, 50 ms scan time) were acquired followed by MS/MS mass scan (20 of dependent events, 20 ms scan time, CE spread 30 ± 25 eV) using precursor priority list containing predicted drug-related metabolites with a total of 0.45 s cycle time. For data-independent analysis (DIA), full scan MS (centroid mode, m/z 60–800, 50 ms scan time) were acquired followed by MS/MS mass scans (23 DIA MS/MS scans with a precursor isolation width of 32 Da, 22 ms scan time, CE spread 30 ± 25 eV) resulting in 0.56 s cycle time. For untargeted analysis, DDA + , DDA − , DIA + , and DIA − modes were employed to analyze the QuEChERS extracts. Mass accuracy correction was achieved using reference masses (m/z 118.086255; 322.048121; 622.028960) after each LC–MS run as external standard, aiming for a measurement accuracy of < 5 ppm. Data stability, reliability, and high quality were ensured through the injection of pooled quality control (pooled QC) at the beginning of the sequence (8 × times), after every fifth sample during the sequence, and samples were analyzed in a random order. Pooled QC was prepared by combining aliquots of the tested samples (both treated and untreated), following common practices in metabolomics studies.
Prediction of pharmaceutical metabolites
To obtain the highest possible number of potential metabolites, the prediction of pharmaceutical metabolites involved the integration of various methods to generate an extensive list of potential drug-related metabolites. Three approaches were employed: (1) literature search, (2) software prediction, and (3) common metabolic pathways (reactions). To compile a comprehensive list of potential drug-related metabolites, a meticulous literature search was conducted. Metabolites from the following publications [ 20 , 23 , 24 , 25 , 26 , 27 , 28 , 29 , 30 , 31 , 32 , 33 , 34 , 35 , 36 , 37 , 38 , 39 , 40 , 41 , 42 , 43 , 44 , 45 , 46 , 47 , 48 ] were extracted and documented in the compounds list within Supplementary Information – List of Predicted Metabolites – Sheet literature search. To predict potential metabolites, three distinct software tools were employed: BioTransformer 3.0 [ 49 ], GLORYx [ 50 ], and enviPath [ 51 ]. This comprehensive software suite was utilized to predict metabolites based on the chemical structures of parent drugs, using their SMILES codes. The resulting lists of potential metabolites generated by each software tool can be found in Supplementary Information – List of Predicted Metabolites – Sheet software prediction. Additionally, a comprehensive set of common metabolic pathways (reactions), as mentioned in previous studies [ 4 , 8 , 9 , 18 , 20 , 21 , 36 , 52 , 53 , 54 , 55 , 56 , 57 , 58 , 59 ] or their combinations, was integrated to create a comprehensive list of potential metabolites. This systematic approach, detailed in Supplementary Information – List of Predicted Metabolites – Sheet List of common metabolic pathways, involved the application of a total of 144 different reaction pathways for each pharmaceutical.
In silico prediction of MS/MS spectra
In the application of methods 1 (literature search) and 2 (software prediction) to generate compound lists, the known structures of metabolites facilitated the retrieval of their chemical formulas, molecular weights, and SMILES codes. However, when employing method 3 (common metabolic pathways/reactions), only the chemical formula and molecular weight were obtained. Because of the inability to predict the site of metabolism (SOM), generating precise structures, and subsequently SMILES codes, was not feasible because of the lack of freely available software solutions. For compounds with available precise structures (SMILES codes), a custom script was employed (Supplementary Information – Script for MS2 in silico prediction). CFM-ID 4.0 [ 60 ] was utilized along with the script to generate MS/MS spectra in both positive and negative ionization modes. CFM-ID produced product spectra at CE values of 10, 20, and 40 eV. The script processed the compound table input (Supplementary Information– chemical_list for All of the Metabolites and Supplementary Information – metabolites_R for metabolites predicted using common metabolic pathways) to create a spectral library, combining CE values of 10 and 20 eV to simulate the use of CE spread during DIA and DDA analyses. CE 40 eV was omitted to avoid the presence of low molecular fragments that could lead to false positives during library matching, lacking specific structural information. In the case of common metabolic pathways, the lack of precise chemical structures (SMILES codes) was effectively addressed as the script generated an MS/MS spectral library for the parent substance, including precursor ions [M + H] + or [M-H] − based on the monoisotopic molecular weight of the metabolite. To illustrate this process, consider the conjugation of sulfamethoxazole with glucose (Sulfamethoxazole-R63). The product ions predicted in silico for sulfamethoxazole are of m/z: 92.04948, 94.06513, and 156.01138, with the precursor ion [M + H] + 254.05994. Consequently, the predicted MS 2 spectrum for Sulfamethoxazole-R63 is 92.04948, 94.06513, 156.01138, and 254.09539, respectively, with the precursor ion [M + H] + 416.11276 (Δm/z 162.0528). The finalized spectral library for the positive ionization mode can be accessed at Supplementary Information—Library Metabolites ESI + , whereas the negative mode library is available at Supplementary Information—Library Metabolites ESI-.
Data processing in MS-DIAL
Following LC-qTOF analysis, the acquired data were converted into centroid mzML format. The converted data were then imported into MS-DIAL 4.0 [ 61 ], along with an in silico spectral library created in the MSP format. Within MS-DIAL, default settings were predominantly applied, with exceptions on tab identification (accurate mass tolerance in MS 1 , 1 mDa; and accurate mass tolerance in MS 2 , 5 mDa), and tab alignment (retention time tolerance 0.1 min). The processed data were subsequently exported for further analysis.
Statistical analysis
The exported data from MS-DIAL 4.0 underwent pre-filtering based on specific criteria, in accordance with established guidelines [ 64 , 65 , 66 , 67 ]: (1) QC %RSD peak area for a particular feature must vary < 30%; (2) the feature must be present in QCs in at least > 50%; and (3) the feature must be present in any group at least > 70%. Subsequently, the data were imported into MetaboAnalyst 4.0 [ 62 ] for statistical analysis. Principal component analysis (PCA) was carried out to determine whether there were statistically significant differences between treated and control groups within each of the studied categories: earthworms and lettuce (leaves and roots). Volcano plot analysis was performed to identify features responsible for these significant differences ( p < 0.05 and fold change (FC) 3.0). Finally, these statistically significant features were cross-referenced with our predicted spectral library and reported.
Results and discussion
Metabolic impact of pharmaceutical exposure.
Similar to findings in environmental studies [ 68 , 69 , 70 , 71 , 72 ], results of PCA (Figs. S1 – S3 ) illustrate whether statistically significant differences exist between non-contaminated and contaminated samples for both earthworms and lettuce. The most pronounced differences between contaminated and non-contaminated samples are observed in lettuce roots (Fig. S2 ), where the sample groups are distinctly separated. This is followed by lettuce leaves (Fig. S3 ), while in the case of earthworm samples, the groups are not clearly separated (Fig. S1 ); this does not imply the absence of differences; rather, it may be due to the limitations of PCA in capturing subtle variations in this dataset. Notably, most of the significant features underlying the differences between sample groups are linked to alterations in the metabolomes of earthworms and lettuce, involving a diverse range of compounds, including lipids, amino acids, saccharides, and others. Although these changes are pertinent to the overall understanding of the system, they fall outside the scope of this manuscript, which focuses on pharmaceutical metabolites. Furthermore, the assurance of high data quality and reliability in various measuring modes is reinforced by the close proximity and tight clustering of the QC pooled samples. Additionally, the graphical placement of QC samples within the figures, interspersed between different sample types, further contributes to the robustness of the data validation process.
In the process of feature picking, a volcano plot analysis was employed with parameters ( p < 0.05 and positive fold change (FC > 3.0)). The use of positive fold change was deliberate, aligning with the focus on identifying only drug-related metabolites, that should not be in blank samples. As in previous studies [ 7 , 19 ], the FC value was set at 3, mirroring the definition of the limit of detection (LoD), and low concentrations of substances. Subsequently, only features deemed statistically significant and showing a positive fold change were subjected to further evaluation (the quantity of significant features in Table S5 ). These significant features were cross-referenced with our in silico spectral library, which was designed for the matching of pharmaceutical metabolites. A total of 26 drug-related metabolites were successfully identified (Table 1 , respectively Tables S6 – S7 with SMILES codes).
Impact of prediction methods on metabolite identification
The prediction process identified a total of 3762 metabolites using three different approaches: 2704 metabolites were identified through software prediction (SP), 194 metabolites were discovered via literature search (LS), and 864 metabolites were identified using common metabolic pathways (CMP). After conducting the predictions, it became apparent that almost 90% of the predicted metabolites exhibited m/z values lower than 800 (3387 out of 3762). Hence, this threshold was utilized as the upper limit for LC-qTOF analysis to optimize the scan time and enhance the sensitivity of the measurements.
The overlap between the three prediction approaches is evaluated in Fig. 2 using a Venn diagram. Software predictions and literature searches identify exact molecular structures, treating structural isomers as distinct metabolites. In contrast, predictions based on common metabolic pathways provide only tentative structures, without differentiating between structural isomers. To accurately compare the approaches, several structural isomers were often grouped together under a single common metabolic pathway (reaction), as illustrated in Fig. 2 . For example, when all three approaches overlapped, the software prediction identified 68 structures, while the literature search yielded 45 structures. All of these metabolites were accounted for by 25 common reaction pathways, due to the generation of structural isomers. This grouping was necessary to ensure a representative and meaningful comparison. The prediction of exact structures through SP can simplify metabolite identification, as it allows for the generation of more precise in silico MS 2 spectra, unlike CMP, which only provides tentative structures. Furthermore, the partial overlap between CMP and SP (or also LS) suggests that the available software for metabolite prediction is not limited to simple oxidation, reduction reactions, hydrolysis, or conjugation with amino acids, saccharides, etc. It also predicts the cleavage of parent compounds into smaller molecules, which are not described by the list of common metabolic pathways, although sometimes it can be analytically quite challenging to link these small degradation product to specific small-drug molecules, as these compounds may also naturally occur within the organism. Nevertheless, the software prediction did not encompass all the common metabolic pathways described in the available literature [ 4 , 8 , 9 , 18 , 20 , 21 , 36 , 52 , 53 , 54 , 55 , 56 , 57 , 58 , 59 ], contrary to our expectations.

Venn diagram illustrating the overlap among three approaches for metabolite structure prediction, highlighting the number of metabolites predicted by each method
This weak overlap may be due to several factors. The software’s prediction algorithms might be optimized for specific types of reactions or tailored to particular organisms, leading to the omission of pathways described in the literature for different organisms. Additionally, the complexity and variability of biological systems could result in metabolites that are not predicted by the software, even though they are reported in the literature. The algorithms might also focus on common metabolic routes, potentially missing less frequent or more complex pathways. Lastly, there may be a lag in updating prediction models with the latest scientific discoveries.
Following successful metabolite identification, a comparison of the different prediction methods for Met-ID success was visualized in a Venn diagram (Fig. 3 and Table S7 ). Breaking down the successful identifications, software prediction identified 11 compounds, literature search identified 12 compounds, and common reaction pathways identified 16 compounds. Notably, common reaction pathways resulted in a slightly higher number of successfully identified metabolites. However, each method produced a unique set of compounds, and only 3 of 26 substances were predicted by all three approaches. Given that no single approach demonstrated superiority, it can be concluded that the strategy employing all three methods for prediction was successful. Meanwhile, studies [ 7 , 11 , 13 , 18 , 19 , 20 , 21 , 22 , 53 , 56 , 74 , 75 ] rely on either literature search, common metabolic pathways, or a simple blank subtraction in mass spectrometry. The comprehensive use of diverse prediction methods enhances the likelihood of capturing a broader spectrum of metabolites, thus contributing to a more thorough and reliable Met-ID process. Moreover, the overlap between the three approaches for successful metabolite identification (Fig. 3 ) is quite low, much like the overlap observed in the number of metabolites predicted by each method (Fig. 2 ). If we examine whether the most intense metabolites were identified by the SP, LS, or CMP approaches, we find that in the case of earthworms (Fig. S43 ), both metabolites (Atenolol-LS1 and Sulfamethoxazole-LS1) were predicted by all three approaches. In contrast, for L. sativa major metabolites (Figs. S44 – S52 ), the major metabolites (such as Ketoprofen-R87, Ketoprofen-R81, Ketoprofen-R63, Sulfamethoxazole-R63, Enrofloxacin-R63) are formed through glucosidation, a process described in common metabolic pathways, but are not predicted by software predictions. Conversely, metabolites identified solely by SP (such as Enrofloxacin-M242, Erythromycin-M46, Erythromycin-M361, Ketoprofen-M648, and Ketoprofen-M835) exhibited lower ion intensities.
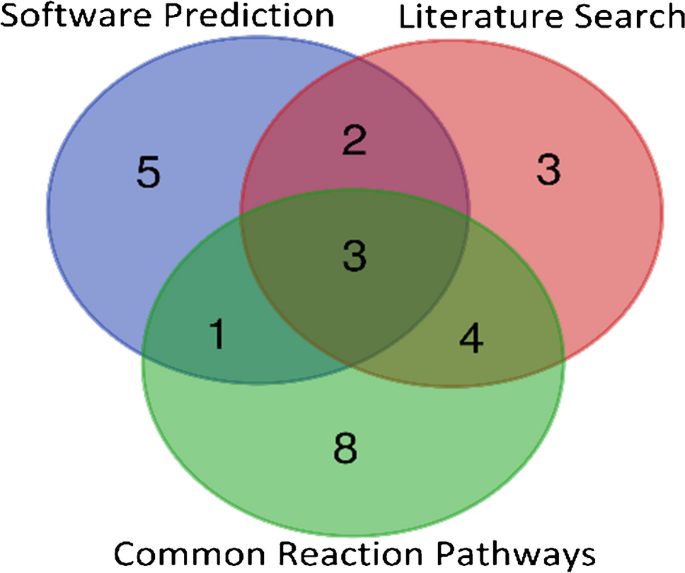
Venn diagram comparing metabolite identification efficiency across different prediction methods, showing the number of metabolites identified by each method
Impact of MS/MS modes on metabolite identification
In this analysis, various MS/MS modes were compared on the basis of the number of library matches, which were visualized using a Venn diagram (Fig. 4 ). Each metabolite:sample type combination was considered as one element, totaling 67 unique elements. ESI + demonstrated slightly more matches (74) than ESI − (55). We postulate that, despite the typical measurement of pharmaceuticals in ESI + , efficient ionization in ESI − was facilitated by conjugation with various endogenous molecules, such as glucose. Both ESI + and ESI − modes yielded both diverse and unique compounds in Met-ID, highlighting the efficiency of utilizing both modes and their complementarity. In ESI + , DDA + (16 unique metabolites) outperformed DIA + (3 unique metabolites), except in the case of earthworm samples, where both MS/MS modes successfully identified 2 pharmaceutical metabolites. This difference may be attributed to the use of a precursor ion list and better MS 2 quality due to the low concentrations in the complex matrix. In ESI − , the difference between DIA − (7) and DDA − (3) was less significant, given the overall lower number of identified metabolites. Consequently, combining DDA + and DDA − resulted in 56 of 67 successful identifications while halving the analysis time. Previous studies on the identification of drug-related metabolites have commonly focused on ESI + [ 11 , 13 , 18 , 19 , 20 , 22 , 55 , 56 , 75 ], whereas in the case of ibuprofen [ 53 ] or triclocarban [ 74 ], ESI − is utilized. Rarely, both ionization modes are employed [ 7 , 21 ]. MS/MS mode studies typically use either DDA [ 7 , 11 , 19 ] or DIA [ 18 , 22 , 74 , 75 ] mode.

Venn diagram comparing metabolite identification efficiency across different MS/MS modes, showing the number of metabolites identified by each mode
Identification of pharmaceutical metabolites
The majority of environmental Met-ID studies [ 18 , 19 , 21 , 22 , 55 , 56 , 74 ] typically focus on the identification of metabolites derived from a single parent drug per experiment. To enhance the study throughput, we simultaneously investigated the metabolites of a mixture comprising six pharmaceuticals. In total, 26 drug-related metabolites were successfully identified (Table 1 ). The distribution among parent substances is as follows: atenolol (1), enrofloxacin (5), ketoprofen (12), sulfamethoxazole (3), tetracycline (2), and erythromycin (3). A pivotal study [ 76 ] characterized five identification confidence levels in HRMS for elucidating small molecules and their metabolites. These confidence levels are commonly employed in Met-ID studies [ 8 , 11 , 19 , 22 ]. Some studies even synthesized metabolites after successful structure elucidation to attain level 1 confidence (confirmed structure by reference standard) [ 8 , 19 ]. Within the scope of this study, only pharmaceutical metabolites with identification confidence level 1 (confirmed structure by reference standard), level 2 (probable structure by library spectrum match or experimental data), and level 3 (tentative structures supported with MS 2 experimental data or, as in our case, by a library match with in silico MS/MS predictions) were reported. Metabolites with confidence levels 4 and 5 were excluded because of complex matrix, low concentrations, missing MS 2 spectra, and overall low data reliability. The overall measured MS 2 quality is influenced by the MS/MS measurement mode, with DDA spectral quality being higher than in the case of DIA due to differences in functionality. Additionally, sample matrix and metabolite concentration can negatively impact spectral quality [ 77 ]. In some instances, MS 2 spectra for both parent drugs and metabolites were obtained in both ESI + and ESI − . All MS 2 spectra of the identified metabolites in this study are illustrated in Figs. S4 – S42 , and their corresponding product ions are listed in Table S6 .
For metabolites with known structures, the product ions were found to be in agreement with in silico predictions by CFM-ID 4.0 [ 60 ]. When the structure of a metabolite was already described, the observed product ions were consistent with available literature, such as acetyl-sulfamethoxazole (Fig. S6 with study [ 40 ]), conjugation of sulfamethoxazole with glucose (Fig. S8 with study [ 40 ]), conjugation of sulfamethoxazole with pterin (Fig. S10 with study [ 41 ]), atenolol acid (Fig. S12 with study [ 23 ]), conjugation of ketoprofen with glucose (Fig. S19 with study [ 36 ]), conjugation of ketoprofen with malonic acid and glucose (Fig. S21 with study [ 36 ]), and double conjugation of ketoprofen with glucose (Fig. S22 with study [ 36 ]).
In instances where only the tentative structure was known, derived from the prediction of the metabolite through common metabolic pathways, the product ions typically exhibited alignment with those of the parent substances. This alignment often included the formation of [M + H] + (or [M-H] − ) ions of the parent substances as intense product ions for the metabolite, especially in cases of phase II metabolism involving conjugation with saccharides or amino acids [ 21 ]. Phase III processes may encompass the formation of secondary conjugations, such as malonyl, which serves as a signal for transport from the cytoplasm to the vacuole [ 19 ]. Regarding metabolism phase I reactions (e.g., hydroxylation, methylation), the presence of precursor ion of the parent drug in MS 2 spectra of metabolites is less common due to fragmentation rules, which are dependent on molecular structure. Despite this, the product ions of parent substances are commonly shared with drug-related metabolites.
This observation aligns with a study [ 18 ] that found that diclofenac metabolites tend to cleave off parts of the molecule attached during conjugation metabolism, even at low collision energies. This suggests that the ion is fragmented to yield characteristic fragments while retaining information about the intact precursor. Similar fragmentation patterns were also noted in other studies [ 11 , 18 , 20 , 36 , 53 , 55 , 75 ] for different compounds such as diclofenac, trimethoprim, ibuprofen, clarithromycin, naproxen, and mefenamic acid. From these findings in both ionization modes, specific fragmentation patterns emerge, such as Δm/z 162.0528 for conjugation with glucose [ 18 , 55 ]; Δm/z 176.0320 for conjugation with glucuronic acid [ 18 ]; Δm/z 86.0004 for conjugation with malonic acid; Δm/z 248.0532 for conjugation with glucose and malonic acid [ 36 ]; or several multiples of Δm/z 162.0528 corresponding to the degree of conjugation with glucose. In the study [ 36 ], the glucosidation of mefenamic acid, naproxen, and ketoprofen was frequently observed, with glucosidation events occurring multiple times and accompanied by gradual losses of Δm/z 162.0528 in MS 2 spectra. However, this phenomenon was not observed in this study, likely because of the lower concentrations. Various combinations of these conjugations, including those involving amino acids, and metabolic reactions from phase I can occur. Subsequent mass spectrometric losses resulting from these processes can be explored in detail in Supplementary Information – List of Predicted Metabolites – Sheet List of common metabolic pathways. The identified fragmentation pathways were exploited for the in silico prediction of MS 2 spectra when the precise structure was not known. Additionally, it is important to note that, in the case of exposure involving a mixture of pharmaceuticals, caution should be exercised to avoid combining pharmaceuticals from the same group. This precaution is warranted because many pharmaceuticals within one group share identical product ions, making it challenging to reliably distinguish which metabolites belong to specific parent drugs.
In ESI + mode, atenolol and its metabolite share some of the following precursor and product ions: 267.1703, 250.1438, 249.1597, 225.1234, 208.0968, 190.0863, 178.0863, 134.06, 116.107, 98.0964, 74.0600, 72.0808, in agreement with studies [ 23 , 78 ]. For sulfamethoxazole and its metabolite in ESI + , the common precursor and product ions are 254.0594, 156.0114, 108.04439, and 92.0495, consistent with study [ 41 ]. In the ESI − mode, the following ions were observed: 252.0448, 156.0125, and 92.0506. In ESI + mode, ketoprofen and its metabolite exhibit common precursor and product ions: 255.1016, 209.0961, 105.0335, as reported in article [ 34 ]. Additionally, in ESI − , ions 253.087 and 209.0972 were observed. For enrofloxacin and its metabolite in ESI + , the common precursor and product ions are 360.1718, 342.1612, 316.182, 245.1085, and 231.0928, in agreement with study [ 30 ]. In ESI + mode, tetracycline and its metabolite share the following precursor and product ions: 445.1606, 428.134, 410.1234, as reported in article [ 45 ], whereas in the ESI − mode, 443.146, 426.1194, 273.0768, and 271.0612 were observed. For erythromycin and its metabolite in ESI + , the common precursor and product ions are 734.4685, 716.4579, 576.3742, 558.3636, 540.3531, and 158.1176, as noted in study [ 79 ].
Additionally, during Met-ID, we considered the relative change of retention time (ΔRt). The ΔRt values were evaluated to align with the alterations in compound polarity. For instance, the ΔRt of Sulfamethoxazole-LS1 (acetyl-sulfamethoxazole) transitioned from 5.15 min to a higher Rt of 6.25 min, in agreement with study [ 40 ]. Similarly, the ΔRt of Sulfamethoxazole-R63 (glucose conjugation) shifted from 5.15 min to a lower Rt of 4.45 min, aligning with study [ 40 ]. Additionally, the ΔRt of Atenolol-LS1 (atenolol acid) moved from 2.94 min to a higher Rt of 4.22 min, as supported by study [ 23 ]; other ΔRt can be seen in Table S6 .
Metabolism of pharmaceuticals
The biotransformation of xenobiotics involves two distinct phases. In phase I of our study, processes such as oxidative deamination, de-alkylation, hydroxylation, or hydrolysis occur, leading to the inclusion or exposure of reactive and hydrophilic groups in the xenobiotic structures. Transitioning into phase II, xenobiotics or their phase I metabolites can undergo conjugation reactions with endogenous compounds such as glucose, malonic acid, or pterin [ 55 ]. The identified metabolites in Eisenia fetida and Lactuca sativa underwent a combination of reactions from both metabolism phases I and II, as summarized in Table S6 . The distribution of metabolites among parent substances is as follows: atenolol (1), enrofloxacin (5), ketoprofen (12), sulfamethoxazole (3), tetracycline (2), and erythromycin (3). The overall metabolic fate of pharmaceuticals in plants and soil organisms can be influenced by several factors, including the chemical structure of the compound, soil properties, contamination levels, plant species, cells/whole organism, and environmental conditions [ 11 , 19 , 21 , 55 , 80 ]. The study [ 55 ] highlighted the differences in biotransformation between ribwort cell suspension and whole ribwort regenerants. Although both model systems exhibited similar types of biotransformation reactions, there were significant differences in the spectrum and abundance of the detected metabolites. Overall, existing studies [ 11 , 19 , 21 , 55 , 80 ] have reported a wide range of metabolites, diverse metabolic pathways within the same pharmaceutical class, varying ratios of parent drugs and metabolites, and concentration dependencies across different sampling times. Our findings align with the observation that different metabolic pathways were evident for various parent drugs, even when experiments involved simultaneous exposure to a mixture of these pharmaceuticals at the same concentration under identical experimental conditions. Despite conducting a thorough literature search for metabolites (Supplementary Information – List of Predicted Metabolites – Sheet literature search), our study revealed distinct metabolites, even for parent drugs previously investigated. For example, a study [ 41 ] reported formyl-SMX and methylsalicylate-SMX, which were not identified in our study. Some pharmaceuticals displayed a preference for conjugation with glucose (e.g., ketoprofen — 6 metabolites, enrofloxacin — 1, sulfamethoxazole — 1), whereas others exhibited cleavage reactions of functional groups (tetracycline — 2 metabolites) or saccharide groups (erythromycin — 2 metabolites), as summarized in Table S6 . Notably, ketoprofen showed the highest number of drug-related metabolites (12) in plants, consistent with a previous study [ 36 ]. A noteworthy disparity in metabolism pathways between earthworms and lettuce lies in the considerably broader spectrum of detected metabolites in lettuce (27 metabolites, while only 2 in earthworms). This difference could be attributed to the different enzymes and also presence of phase III metabolism in plants, a process involving the storage of metabolites within cell vacuoles, commonly referred to as sequestration (green liver concept) [ 1 , 4 , 7 ]. Additionally, other studies have identified several intermediates of metabolic reactions [ 41 , 44 ], whereas in our case, we only identified Ketoprofen-LS24 as an intermediate in the transformation to Ketoprofen-LS25, consistent with the findings of the study [ 38 ]. Moreover, analysis of the spatial distribution of both parent drugs and metabolites could shed light on their origin and whether they accumulate in specific parts of the body or organs.
Occurrence of pharmaceuticals and their metabolites in the samples
Table 1 summarizes the presence of parent drugs and their metabolites in earthworm and lettuce samples at various exposure times: for earthworms (1, 3, 7, 14, and 21 days) and lettuce (14, 21, and 28 days). Table 1 also indicates whether the compounds were present in the soil or water environment at the end of the experiment, providing insights into where the metabolites were formed. The number of identified metabolites in different lettuce samples includes lettuce roots — hydroponics (22), lettuce leaves — hydroponics (0), lettuce roots — soil (13), lettuce leaves — soil (6), and earthworm tissue (2).
Among all the parent pharmaceuticals and their metabolites detected in our study, only a subset of erythromycin metabolites was identified in both aqueous and soil environments at the end of the exposure experiments. This contrasts with studies [ 9 , 10 ], which generally report that metabolites of pharmaceuticals commonly form in the environment. The observed discrepancy may be due to differences in experimental design, including variations in the specific pharmaceutical tested (our study focused on atenolol, enrofloxacin, erythromycin, ketoprofen, sulfamethoxazole, and tetracycline), the pharmaceutical concentrations, exposure duration, physicochemical properties of the soil, the volume of soil and water, and environmental conditions such as temperature, humidity, and photoperiod. Additionally, differences in the presence and activity of microorganisms could also influence the formation and detection of metabolites.
Earthworm tissues contained all six parent pharmaceuticals (atenolol — ATE, enrofloxacin — ENR, ketoprofen — KPF, sulfamethoxazole — SMX, tetracycline — TC, and erythromycin — ERY). In contrast, lettuce roots and leaves from both hydroponic and soil environments did not contain all the parent substances. Surprisingly, despite the initial discrepancy, lettuce samples ultimately contained a significantly higher number of metabolites compared to earthworm tissues. Specifically, only two metabolites were detected in earthworm tissues: ATE-LS1 (atenolol acid [ 23 ]) and SMX-LS1 (acetyl-sulfamethoxazole [ 39 , 40 ]). Both compounds, previously described in the literature, were consistently present in earthworm tissues throughout the experiment, spanning from day 1 to day 21, as illustrated by the normalized peak intensity displayed in Fig. S43 .
In the lettuce roots under hydroponic conditions, the following metabolites of parent drugs, along with their normalized intensities, were consistently present across all samples taken on days 14, 21, and 28: atenolol (ATE-LS1; abundance in Fig. S44 B), enrofloxacin (ciprofloxacin, ENR-R2, ENR-R13, ENR-R63, and ENR-M242; abundance in Fig. S48 ), ketoprofen (KPF-R13, KPF-R23, KPF-R63, KPF-R81, KPF-R111, KPF-LS24, KPF-LS25, and KPF-M835; abundance in Fig. S46 ), sulfamethoxazole (SMX-LS1, SMX-LS6, and SMX-R63; abundance in Fig. S47 B), tetracycline (TC-LS2, TC-LS3; abundance in Fig. S49 ), and erythromycin (ERY-M1, ERY-M46, and ERY-M361; abundance in Fig. S50 ). All of these metabolites were present in all samples on days 14, 21, and 28, except for ERY-M1 and ERY-M361, which were only present on day 14. The three ERY metabolites were also detected in the aqueous medium, making it difficult to distinguish whether they were formed in both the aqueous environment and lettuce roots or only in the medium and subsequently taken up. In the lettuce roots under soil conditions, the following metabolites of parent drugs, along with their normalized intensities, were identified: atenolol (ATE-LS1; abundance in Fig. S44 A), ketoprofen (KPF-R13, KPF-R23, KPF-R63, KPF-R81, KPF-R87, KPF-R93, KPF-R96, KPF-LS24, KPF-M648, and KPF-M835; abundance in Fig. S45 ), and sulfamethoxazole (SMX-LS1, and SMX-R63; abundance in Fig. S47 A). Metabolites ATE-LS1, KPF-R13, KPF-R81, KPF-M835, SMX-LS1, and SMX-R63 were consistently present in all samples over the span of 14, 21, and 28 days. Metabolites KPF-R23 to KPF-R63, KPF-R87, KPF-R93, KPF-LS24, and KPF-M835 were in samples from day 21. KPF-R96 was only in samples taken on day 28. As indicated by our results, the formation of certain metabolites occurs later during the exposure experiment, influenced by whether lettuce is cultivated under hydroponic or soil conditions. This difference is notably caused the concentrations of parent drugs in the roots, thereby affecting the kinetic rate of metabolite formation. Additionally, these cultivation conditions can influence the ratios between parent drugs and both major and minor metabolites. In lettuce root samples, a higher number of metabolites (22) were detected under hydroponic conditions compared with the soil environment (13). Remarkably, in the soil environment, roots contained only metabolites of atenolol, ketoprofen, and sulfamethoxazole, excluding enrofloxacin, tetracycline, and erythromycin, despite the detection of their parent compounds in the roots. This discrepancy, even with roots distributed throughout the PET pot, is likely attributable to a combination of the physicochemical properties of pharmaceuticals (Table S1 ) and soil properties (Table S4 ). In agreement with previous studies [ 16 , 81 , 82 ], the lower uptake of these parent drugs can be attributed to significantly higher Kd values in soil (enrofloxacin 260–6310 L∙kg −1 [ 83 ], tetracycline 420–1030 L∙kg −1 [ 83 ], erythromycin 8.3–128 L∙kg −1 [ 83 ]), molecular weight, their pKa values, and soil properties, particularly organic matter content, pH value, and concentrations of divalent cations (such as Ca 2+ , Mg 2+ ) [ 84 , 85 ]. Under hydroponic conditions, where soil factors are excluded, only the physicochemical properties of the substances and plant metabolism influence uptake. This explains why the majority of studies [ 82 , 86 ] employ hydroponic conditions to assess the impact of pharmaceutical properties on uptake.
After the uptake of the parent compound by lettuce roots, translocation to leaves may occur, either as the parent drug with potential subsequent metabolization or the translocation of metabolites from roots to leaves. Previous studies [ 19 , 55 , 74 ] have reported that roots contain significantly higher concentrations of pharmaceuticals than leaves. This finding aligns with the number of identified metabolites in lettuce roots (26) and leaves (6), as well as with the abundances in Figs. S44 – S52 . Furthermore, the concentration of pharmaceuticals in plants depends on the contamination level of the environment. The following metabolites were detected in lettuce leaves grown in a soil environment: atenolol metabolite (ATE-LS1), ketoprofen (KPF-R13, KPF-R63, KPF-M835; abundance in Fig. S51 ), and sulfamethoxazole (SMX-LS1 and SMX-R63; abundance in Fig. S52 ). All of these metabolites were also detected in lettuce roots. Interestingly, these metabolites were present only when lettuce was grown in soil, although the abundance of the parent compound and its metabolites was significantly higher under hydroponic conditions. Similar to lettuce roots, the highest number of metabolites was ketoprofen-related. According to previous studies [ 10 , 19 ], the distribution of compounds in plants appears to be affected by the molecular size, pharmaceutical charge, and other physicochemical properties. However, the pH in different plant organelles (vacuole, xylem, phloem, and cytosol) may differ from each other and from the pH in soil or hydroponic solution. Therefore, compounds taken up easily do not necessarily distribute well within plants. The diverse pattern of metabolites in different vegetables indicates that the uptake and metabolism of micropollutants are plant-specific and difficult to predict.
A novel workflow for high-throughput identification of pharmaceutical metabolites across various matrices, utilizing open-source software and LC-HRMS analyses, was developed and presented. This workflow featured a triplet approach for metabolite structure prediction — integrating software-based predictions, literature review, and known metabolic pathways — which led to the prediction of 3762 metabolites corresponding to six parent compounds: atenolol, enrofloxacin, erythromycin, ketoprofen, sulfamethoxazole, and tetracycline. In silico mass spectral libraries were generated for both positive and negative ionization modes and uploaded to MS-DIAL software for processing DDA and DIA measurements. Ultimately, 26 statistically significant metabolites ( p < 0.05) were identified in E. fetida and L. sativa .
The main advantage of this novel workflow is its capacity for high-throughput data analysis, including statistical evaluation, using only freely available software compatible with instruments from all vendors. Additionally, it can be adapted for other organic micropollutants beyond pharmaceuticals by simply importing different chemical structures. However, the workflow has limitations due to the software’s inability to predict all potential metabolites, given the unique metabolic profiles of different organisms. In this study, these limitations were addressed through literature reviews and the incorporation of common metabolic pathways. Although, discrepancies between in silico mass spectra and experimentally obtained MS 2 spectra may still occur, due to the dependence on available data, future improvements are anticipated. As more data becomes available and software evolves, prediction accuracy and metabolite coverage are expected to improve significantly. This advancement will enhance the estimation of environmental and health risks by enabling more accurate identification of metabolites.
Nevertheless, the implications of this study confirm significant concerns about environmental and health risks, particularly given the number and abundance of pharmaceutical metabolites relative to parent substances. Currently, only parent compounds are quantified in environmental and biological matrices, as these metabolites are often not identified, potentially leading to an underestimation of these risks. Pharmaceutical metabolites could contaminate the food chain, posing health risks from long-term dietary intake of trace concentrations, and may also contribute to the rise of antimicrobial resistance in the environment, potentially impacting human health. In the future, appropriate legislation should be implemented to limit the dissemination of both pharmaceutical and metabolite residues, as well as antibiotic-resistant genes into the soil environment. Since these contaminants can be removed from wastewater, animal manure, and biosolids more easily than from the soil environment using processes such as advanced oxidation and aerobic/anaerobic fermentation.
Data availability
Data not included in the main text or SI can be accessed by contacting the corresponding author.
Miller EL, Nason SL, Karthikeyan KG, Pedersen JA. Root uptake of pharmaceuticals and personal care product ingredients. Environ Sci Technol. 2016;50:525–41.
Article PubMed CAS Google Scholar
Wu C, Spongberg AL, Witter JD, Fang M, Czajkowski KP. Uptake of pharmaceutical and personal care products by soybean plants from soils applied with biosolids and irrigated with contaminated water. Environ Sci Technol. 2010;44:6157–61.
Carter LJ, Williams M, Böttcher C, Kookana RS. Uptake of pharmaceuticals influences plant development and affects nutrient and hormone homeostases. Environ Sci Technol. 2015;49:12509–18.
Riemenschneider C, Seiwert B, Moeder M, Schwarz D, Reemtsma T. Extensive transformation of the pharmaceutical carbamazepine following uptake into intact tomato plants. Environ Sci Technol. 2017;51:6100–9.
Tanoue R, Sato Y, Motoyama M, Nakagawa S, Shinohara R, Nomiyama K. Plant uptake of pharmaceutical chemicals detected in recycled organic manure and reclaimed wastewater. J Agric Food Chem. 2012;60:10203–11.
Carter LJ, Garman CD, Ryan J, Dowle A, Bergström E, Thomas-Oates J, et al. Fate and uptake of pharmaceuticals in soil-earthworm systems. Environ Sci Technol. 2014;48:5955–63.
Article PubMed PubMed Central CAS Google Scholar
Chuang Y-H, Liu C-H, Hammerschmidt R, Zhang W, Boyd SA, Li H. Metabolic demethylation and oxidation of caffeine during uptake by lettuce. J Agric Food Chem. 2018;66:7907–15.
Fu Q, Zhang J, Borchardt D, Schlenk D, Gan J. Direct conjugation of emerging contaminants in Arabidopsis : indication for an overlooked risk in plants? Environ Sci Technol. 2017;51:6071–81.
He Y, Langenhoff AAM, Sutton NB, Rijnaarts HHM, Blokland MH, Chen F, et al. Metabolism of ibuprofen by Phragmites australis : uptake and phytodegradation. Environ Sci Technol. 2017;51:4576–84.
Riemenschneider C, Al-Raggad M, Moeder M, Seiwert B, Salameh E, Reemtsma T. Pharmaceuticals, their metabolites, and other polar pollutants in field-grown vegetables irrigated with treated municipal wastewater. J Agric Food Chem. 2016;64:5784–92.
Tadić Đ, Gramblicka M, Mistrik R, Bayona JM. Systematic identification of trimethoprim metabolites in lettuce. Anal Bioanal Chem. 2022;414:3121–35.
Article PubMed PubMed Central Google Scholar
Rede D, Santos LHMLM, Ramos S, Oliva-Teles F, Antão C, Sousa SR, et al. Individual and mixture toxicity evaluation of three pharmaceuticals to the germination and growth of Lactuca sativa seeds. Sci Total Environ. 2019;673:102–9.
Akenga P, Gachanja A, Fitzsimons MF, Tappin A, Comber S. Uptake, accumulation and impact of antiretroviral and antiviral pharmaceutical compounds in lettuce. Sci Total Environ. 2021;766:144499.
Ceci L, Cavalera MA, Serrapica F, Di Francia A, Masucci F, Carelli G. Use of reclaimed urban wastewater for the production of hydroponic barley forage: water characteristics, feed quality and effects on health status and production of lactating cows. Front Vet Sci. 2023;10:1274466.
Geng J, Liu X, Wang J, Li S. Accumulation and risk assessment of antibiotics in edible plants grown in contaminated farmlands: A review. Sci Total Environ. 2022;853:158616.
Keerthanan S, Jayasinghe C, Biswas JK, Vithanage M. Pharmaceutical and Personal Care Products (PPCPs) in the environment: Plant uptake, translocation, bioaccumulation, and human health risks. Crit Rev Environ Sci Technol. 2021;51:1221–58.
Article CAS Google Scholar
Srichamnong W, Kalambaheti N, Woskie S, Kongtip P, Sirivarasai J, Matthews KR. Occurrence of antibiotic-resistant bacteria on hydroponically grown butterhead lettuce ( Lactuca sativa var capitata ). Food Sci Nutr. 2021;9:1460–70.
Mlynek F, Himmelsbach M, Buchberger W, Klampfl CW. A new analytical workflow using HPLC with drift-tube ion-mobility quadrupole time-of-flight/mass spectrometry for the detection of drug-related metabolites in plants. Anal Bioanal Chem. 2020;412:1817–24.
Madmon M, Zvuluni Y, Mordehay V, Hindi A, Malchi T, Drug E, et al. Pharmacokinetics of the recalcitrant drug lamotrigine: identification and distribution of metabolites in cucumber plants. Environ Sci Technol. 2023;57:20228–37.
Emhofer L, Himmelsbach M, Buchberger W, Klampfl CW. High-performance liquid chromatography – mass spectrometry analysis of the parent drugs and their metabolites in extracts from cress ( Lepidium sativum ) grown hydroponically in water containing four non-steroidal anti-inflammatory drugs. J Chromatogr A. 2017;1491:137–44.
Marsik P, Sisa M, Lacina O, Motkova K, Langhansova L, Rezek J, et al. Metabolism of ibuprofen in higher plants: A model Arabidopsis thaliana cell suspension culture system. Environ Pollut. 2017;220:383–92.
Martínez-Piernas AB, Nahim-Granados S, Polo-López MI, Fernández-Ibáñez P, Murgolo S, Mascolo G, et al. Identification of transformation products of carbamazepine in lettuce crops irrigated with Ultraviolet-C treated water. Environ Pollut. 2019;247:1009–19.
Article PubMed Google Scholar
Koba O, Golovko O, Kodešová R, Klement A, Grabic R. Transformation of atenolol, metoprolol, and carbamazepine in soils: The identification, quantification, and stability of the transformation products and further implications for the environment. Environ Pollut. 2016;218:574–85.
Xu Y, Wang N, Peng L, Li S, Liang C, Song K, et al. Free nitrous acid inhibits atenolol removal during the sidestream partial nitritation process through regulating microbial-induced metabolic types. Environ Sci Technol. 2022;56:11614–24.
Quaresma AV, Sousa BA, Silva KTS, Silva SQ, Werle AA, Afonso RJCF. Oxidative treatments for atenolol removal in water: Elucidation by mass spectrometry and toxicity evaluation of degradation products. Rapid Commun Mass Spectrom. 2019;33:303–13.
Toolaram AP, Menz J, Rastogi T, Leder C, Kümmerer K, Schneider M. Hazard screening of photo-transformation products from pharmaceuticals: Application to selective β1-blockers atenolol and metoprolol. Sci Total Environ. 2017;579:1769–80.
Xu Y, Radjenovic J, Yuan Z, Ni B-J. Biodegradation of atenolol by an enriched nitrifying sludge: Products and pathways. Chem Eng J. 2017;312:351–9.
Medana C, Calza P, Carbone F, Pelizzetti E, Hidaka H, Baiocchi C. Characterization of atenolol transformation products on light-activated TiO 2 surface by high-performance liquid chromatography/high-resolution mass spectrometry. Rapid Commun Mass Spectrom. 2008;22:301–13.
Rusch M, Spielmeyer A, Zorn H, Hamscher G. Degradation and transformation of fluoroquinolones by microorganisms with special emphasis on ciprofloxacin. Appl Microbiol Biotechnol. 2019;103:6933–48.
Morales-Gutiérrez FJ, Barbosa J, Barrón D. Metabolic study of enrofloxacin and metabolic profile modifications in broiler chicken tissues after drug administration. Food Chem. 2015;172:30–9.
Wetzstein HG, Schmeer N, Karl W. Degradation of the fluoroquinolone enrofloxacin by the brown rot fungus Gloeophyllum striatum: identification of metabolites. Appl Environ Microbiol. 1997;63:4272–81.
Zhao C-Y, Ru S, Cui P, Qi X, Kurade MB, Patil SM, et al. Multiple metabolic pathways of enrofloxacin by Lolium perenne L.: Ecotoxicity, biodegradation, and key driven genes. Water Res. 2021;202:117413.
Chen Q, Zhang L, Han Y, Fang J, Wang H. Degradation and metabolic pathways of sulfamethazine and enrofloxacin in Chlorella vulgaris and Scenedesmus obliquus treatment systems. Environ Sci Pollut Res. 2020;27:28198–208.
Salgado R, Pereira VJ, Carvalho G, Soeiro R, Gaffney V, Almeida C, et al. Photodegradation kinetics and transformation products of ketoprofen, diclofenac and atenolol in pure water and treated wastewater. J Hazard Mater. 2013;244–245:516–27.
Marco-Urrea E, Pérez-Trujillo M, Cruz-Morató C, Caminal G, Vicent T. White-rot fungus-mediated degradation of the analgesic ketoprofen and identification of intermediates by HPLC–DAD–MS and NMR. Chemosphere. 2010;78:474–81.
Mlynek F, Himmelsbach M, Buchberger W, Klampfl CW. A fast-screening approach for the tentative identification of drug-related metabolites from three non-steroidal anti-inflammatory drugs in hydroponically grown edible plants by HPLC-drift-tube-ion-mobility quadrupole time-of-flight mass spectrometry. Electrophoresis. 2021;42:482–9.
Quintana J, Weiss S, Reemtsma T. Pathways and metabolites of microbial degradation of selected acidic pharmaceutical and their occurrence in municipal wastewater treated by a membrane bioreactor. Water Res. 2005;39:2654–64.
Wang L, Zheng Y, Zhou Y, Lu J, Chovelon J-M, Ji Y. Aquatic photolysis of ketoprofen generates products with photosensitizing activity and toxicity. Water Res. 2022;210:117982.
Menacherry SPM, Kodešová R, Švecová H, Klement A, Fér M, Nikodem A, et al. Selective accumulation of pharmaceutical residues from 6 different soils by plants: a comparative study on onion, radish, and spinach. Environ Sci Pollut Res. 2023;30:54160–76.
Wang J, Gardinali PR. Identification of phase II pharmaceutical metabolites in reclaimed water using high resolution benchtop Orbitrap mass spectrometry. Chemosphere. 2014;107:65–73.
Huynh K, Reinhold D. Metabolism of Sulfamethoxazole by the Model Plant Arabidopsis thaliana . Environ Sci Technol. 2019;53:4901–11.
Dudley S, Sun C, Jiang J, Gan J. Metabolism of sulfamethoxazole in Arabidopsis thaliana cells and cucumber seedlings. Environ Pollut. 2018;242:1748–57.
García-Galán MJ, Arashiro L, Santos LHMLM, Insa S, Rodríguez-Mozaz S, Barceló D, et al. Fate of priority pharmaceuticals and their main metabolites and transformation products in microalgae-based wastewater treatment systems. J Hazard Mater. 2020;390:121771.
Kurade MB, Xiong J-Q, Govindwar SP, Roh H-S, Saratale GD, Jeon B-H, et al. Uptake and biodegradation of emerging contaminant sulfamethoxazole from aqueous phase using Ipomoea aquatica. Chemosphere. 2019;225:696–704.
Sengupta A, Sarkar D, Das P, Panja S, Parikh C, Ramanathan D, et al. Tetracycline uptake and metabolism by vetiver grass (Chrysopogon zizanioides L. Nash). Environ Sci Pollut Res. 2016;23:24880–9.
Arslan Topal EI. Uptake of tetracycline and metabolites in Phragmites australis exposed to treated poultry slaughterhouse wastewaters. Ecol Eng. 2015;83:233–8.
Article Google Scholar
Bhatt P, Jeon C-H, Kim W. Tetracycline bioremediation using the novel Serratia marcescens strain WW1 isolated from a wastewater treatment plant. Chemosphere. 2022;298:134344.
Pan M, Lyu T, Zhan L, Matamoros V, Angelidaki I, Cooper M, et al. Mitigating antibiotic pollution using cyanobacteria: Removal efficiency, pathways and metabolism. Water Res. 2021;190:116735.
Wishart DS, Tian S, Allen D, Oler E, Peters H, Lui VW, et al. BioTransformer 3.0—a web server for accurately predicting metabolic transformation products. Nucleic Acids Res. 2022;50:W115-23.
de Bruyn KC, Šícho M, Mazzolari A, Kirchmair J. GLORYx: Prediction of the Metabolites Resulting from Phase 1 and Phase 2 Biotransformations of Xenobiotics. Chem Res Toxicol. 2021;34:286–99.
Wicker J, Lorsbach T, Gütlein M, Schmid E, Latino D, Kramer S, et al. enviPath – The environmental contaminant biotransformation pathway resource. Nucleic Acids Res. 2016;44:D502–8.
Jacobs PL, Ridder L, Ruijken M, Rosing H, Jager NG, Beijnen JH, et al. Identification of drug metabolites in human plasma or serum integrating metabolite prediction, LC–HRMS and untargeted data processing. Bioanalysis. 2013;5:2115–28.
Pietrini F, Di Baccio D, Aceña J, Pérez S, Barceló D, Zacchini M. Ibuprofen exposure in Lemna gibba L.: Evaluation of growth and phytotoxic indicators, detection of ibuprofen and identification of its metabolites in plant and in the medium. J Hazard Mater. 2015;300:189–93.
Lahti M, Brozinski J, Jylhä A, Kronberg L, Oikari A. Uptake from water, biotransformation, and biliary excretion of pharmaceuticals by rainbow trout. Environ Toxicol Chem. 2011;30:1403–11.
Stuchlíková Raisová L, Podlipná R, Szotáková B, Syslová E, Skálová L. Evaluation of drug uptake and deactivation in plant: Fate of albendazole in ribwort plantain (Plantago laceolata) cells and regenerants. Ecotoxicol Environ Saf. 2017;141:37–42.
Sauvêtre A, May R, Harpaintner R, Poschenrieder C, Schröder P. Metabolism of carbamazepine in plant roots and endophytic rhizobacteria isolated from Phragmites australis. J Hazard Mater. 2018;342:85–95.
Huber C, Bartha B, Harpaintner R, Schröder P. Metabolism of acetaminophen (paracetamol) in plants—two independent pathways result in the formation of a glutathione and a glucose conjugate. Environ Sci Pollut Res. 2009;16:206.
Chen F, Huber C, May R, Schröder P. Metabolism of oxybenzone in a hairy root culture: Perspectives for phytoremediation of a widely used sunscreen agent. J Hazard Mater. 2016;306:230–6.
Bartha B, Huber C, Schröder P. Uptake and metabolism of diclofenac in Typha latifolia – How plants cope with human pharmaceutical pollution. Plant Sci. 2014;227:12–20.
Wang F, Allen D, Tian S, Oler E, Gautam V, Greiner R, et al. CFM-ID 4.0 – a web server for accurate MS-based metabolite identification. Nucleic Acids Res. 2022;50:W165-74.
Tsugawa H, Ikeda K, Takahashi M, Satoh A, Mori Y, Uchino H, et al. A lipidome atlas in MS-DIAL 4. Nat Biotechnol. 2020;38:1159–63.
Chong J, Soufan O, Li C, Caraus I, Li S, Bourque G, et al. MetaboAnalyst 4.0: towards more transparent and integrative metabolomics analysis. Nucleic Acids Res. 2018;46:W486-94.
Mravcová L, Amrichová A, Navrkalová J, et al. Optimization and validation of multiresidual extraction methods for pharmaceuticals in soil, lettuce, and earthworms. Environ Sci Pollut Res. 2024;31:33120–40. https://doi.org/10.1007/s11356-024-33492-7 .
Broadhurst D, Goodacre R, Reinke SN, Kuligowski J, Wilson ID, Lewis MR, et al. Guidelines and considerations for the use of system suitability and quality control samples in mass spectrometry assays applied in untargeted clinical metabolomic studies. Metabolomics. 2018;14:72.
Godzien J, Alonso-Herranz V, Barbas C, Armitage EG. Controlling the quality of metabolomics data: new strategies to get the best out of the QC sample. Metabolomics. 2015;11:518–28.
Rodríguez-Coira J, Delgado-Dolset M, Obeso D, Dolores-Hernández M, Quintás G, Angulo S, et al. Troubleshooting in large-scale LC-ToF-MS metabolomics analysis: solving complex issues in big cohorts. Metabolites. 2019;9:247.
Dunn WB, Broadhurst D, Begley P, Zelena E, Francis-McIntyre S, Anderson N, et al. Procedures for large-scale metabolic profiling of serum and plasma using gas chromatography and liquid chromatography coupled to mass spectrometry. Nat Protoc. 2011;6:1060–83.
David A, Lange A, Abdul-Sada A, Tyler CR, Hill EM. Disruption of the prostaglandin metabolome and characterization of the pharmaceutical exposome in fish exposed to wastewater treatment works effluent as revealed by nanoflow-nanospray mass spectrometry-based metabolomics. Environ Sci Technol. 2017;51:616–24.
Fu Q, Scheidegger A, Laczko E, Hollender J. Metabolomic Profiling and Toxicokinetics Modeling to Assess the Effects of the Pharmaceutical Diclofenac in the Aquatic Invertebrate Hyalella azteca . Environ Sci Technol. 2021;55:7920–9.
Wang H, Li Z, Chen H, Jin J, Zhang P, Shen L, et al. Metabolomic analysis reveals the impact of ketoprofen on carbon and nitrogen metabolism in rice (Oryza sativa L.) seedling leaves. Environ Sci Pollut Res. 2022;30:21825–37.
Karpuzcu ME, Fairbairn D, Arnold WA, Barber BL, Kaufenberg E, Koskinen WC, et al. Identifying sources of emerging organic contaminants in a mixed use watershed using principal components analysis. Environ Sci Processes Impacts. 2014;16:2390–9.
Papa E, Fick J, Lindberg R, Johansson M, Gramatica P, Andersson PL. Multivariate chemical mapping of antibiotics and identification of structurally representative substances. Environ Sci Technol. 2007;41:1653–61.
Agius JE, Kimble B, Govendir M, Rose K, Pollard C-L, Phalen DN. Pharmacokinetic profile of enrofloxacin and its metabolite ciprofloxacin in Asian house geckos (Hemidactylus frenatus) after single-dose oral administration of enrofloxacin. Vet Anim Sci. 2020;9:100116.
Huynh K, Banach E, Reinhold D. Transformation, conjugation, and sequestration following the uptake of triclocarban by jalapeno pepper plants. J Agric Food Chem. 2018;66:4032–43.
Tian R, Zhang R, Uddin M, Qiao X, Chen J, Gu G. Uptake and metabolism of clarithromycin and sulfadiazine in lettuce. Environ Pollut. 2019;247:1134–42.
Schymanski EL, Jeon J, Gulde R, Fenner K, Ruff M, Singer HP, et al. Identifying small molecules via high resolution mass spectrometry: communicating confidence. Environ Sci Technol. 2014;48:2097–8.
Guo J, Huan T. Comparison of full-scan, data-dependent, and data-independent acquisition modes in liquid chromatography-mass spectrometry based untargeted metabolomics. Anal Chem. 2020;92:8072–80.
Helbling DE, Hollender J, Kohler H-PE, Singer H, Fenner K. High-throughput identification of microbial transformation products of organic micropollutants. Environ Sci Technol. 2010;44:6621–7.
González de la Huebra MJ, Vincent U. Analysis of macrolide antibiotics by liquid chromatography. J Pharm Biomed Anal. 2005;39:376–98.
Tadić Đ, Gramblicka M, Mistrik R, Flores C, Piña B, Bayona JM. Elucidating biotransformation pathways of ofloxacin in lettuce (Lactuca sativa L). Environ Pollut. 2020;260:114002.
Li Y, Sallach JB, Zhang W, Boyd SA, Li H. Insight into the distribution of pharmaceuticals in soil-water-plant systems. Water Res. 2019;152:38–46.
Bigott Y, Khalaf DM, Schröder P, Schröder PM, Cruzeiro C. Uptake and translocation of pharmaceuticals in plants: principles and data analysis. In: Pérez Solsona S, Montemurro N, Chiron S, Barceló D, editors. Interaction and Fate of Pharmaceuticals in Soil-Crop Systems, vol. 103. The Handbook of Environmental Chemistry. Cham: Springer; 2020. https://doi.org/10.1007/698_2020_622 .
Chapter Google Scholar
Song W, Guo M. Residual Veterinary Pharmaceuticals in Animal Manures and Their Environmental Behaviors in Soils. Applied Manure and Nutrient Chemistry for Sustainable Agriculture and Environment. Dordrecht: Springer; 2014. p. 23–52.
Google Scholar
Kim K-R, Owens G, Kwon S-I, So K-H, Lee D-B, Ok YS. Occurrence and environmental fate of veterinary antibiotics in the terrestrial environment. Water Air Soil Pollut. 2011;214:163–74.
Chee-Sanford JC, Mackie RI, Koike S, Krapac IG, Lin Y, Yannarell AC, et al. Fate and transport of antibiotic residues and antibiotic resistance genes following land application of manure waste. J Environ Qual. 2009;38:1086–108.
Carvalho PN, Basto MCP, Almeida CMR, Brix H. A review of plant–pharmaceutical interactions: from uptake and effects in crop plants to phytoremediation in constructed wetlands. Environ Sci Pollut Res. 2014;21:11729–63.
Download references
Acknowledgements
This work was measured within the Lab4you Shimadzu student program in 2022. Moreover, we would like to thank Mrs. Kleinová and Mr. Klein for safe transportation of samples to Shimadzu Europa in Germany. In addition, we would like to acknowledge that the graphical abstract was created using BioRender.com software.
Open access publishing supported by the National Technical Library in Prague. This study was financially supported by the Ministry of Education, Youth, and Sports of the Czech Republic (Project No. FCH-S-24–8591).
Author information
Authors and affiliations.
Institute of Chemistry and Technology of Environmental Protection, Faculty of Chemistry, Brno University of Technology, Purkyňova 118, 612 00, Brno, Czech Republic
Jan Fučík, Helena Zlámalová Gargošová & Ludmila Mravcová
Faculty of Electrical Engineering and Communication, Brno University of Technology, Technická 3058/10, 616 00, Brno, Czech Republic
Stanislav Fučík
Shimadzu Europa GmbH, Albert-Hahn-Straße 6, 472 69, Duisburg, Germany
Sascha Rexroth
CEITEC Brno University of Technology, Purkyňova 656/123, 612 00, Brno, Czech Republic
Marian Sedlář
You can also search for this author in PubMed Google Scholar
Contributions
J.F. — conceptualization, methodology, formal analysis, investigations, resources, data curation, writing original draft, visualization, project administration, funding acquisition. S.F. — software, data curation, writing — review and editing. S.R. — conceptualization, supervision, writing — review and editing, resources. M.S. — investigation, resources. H.Z.G. — supervision, funding acquisition. L.M. — conceptualization, supervision, writing — review and editing, funding acquisition. All authors contributed to the manuscript and approved the final version for publication.
Corresponding author
Correspondence to Jan Fučík .
Ethics declarations
Ethics approval.
Not applicable.
Consent to participate
Consent for publication.
All authors approved the final manuscript.
Competing interests
The authors declare no competing interests.
Additional information
Publisher's note.
Springer Nature remains neutral with regard to jurisdictional claims in published maps and institutional affiliations.
Supplementary Information
Below is the link to the electronic supplementary material.
216_2024_5515_MOESM1_ESM.docx
Supplementary file1 contains general and detailed information about Uptake experiments, QuEChERS extraction methods, modified Sonneveld’s recipe for the hydroponic solution; physicochemical properties of pharmaceuticals; physicochemical properties of soil; PCA of different samples (earthworms, lettuce roots, lettuce leaves); Overview of identified metabolites and their structure as SMILES code; Overview of the method of prediction of identified metabolites; MS2 spectra of all identified metabolites; and normalised abundances of parent pharmaceuticals and their metabolites. (DOCX 3784 KB)
216_2024_5515_MOESM2_ESM.7z
Supplementary file2 consists of several files. File “List of Predicted Metabolites” contains list of all predicted metabolites from three different prediction methods (software prediction, literature search, common metabolic pathways) and List of common metabolic pathways. File “chemical_list” encompasses all metabolites, including those with precisely known structures with available SMILES and InChI, as well as metabolites predicted by common metabolic pathways where SMILES and InChI are not available. This file explicitly specifies for which metabolites the MS2 spectral library should be predicted and included in the output library. File “metabolites_R” contains metabolites predicted by common metabolic pathways, and its purpose is to generate the MS2 spectral library for these particular metabolites. File “Library Metabolites ESI +” is In silico predicted MS2 Library for pharmaceutical metabolites in ESI+ in msp format. File “Library Metabolites ESI-” is In silico predicted MS2 Library for pharmaceutical metabolites in ESI- in msp format. (7Z 906 KB)
216_2024_5515_MOESM3_ESM.docx
Supplementary file3 presents the script for MS2 in silico prediction. Document outlines instructions for running the script, required input data, output details, and information about the generated MS/MS spectral library. (DOCX 23 KB)
Rights and permissions
Open Access This article is licensed under a Creative Commons Attribution 4.0 International License, which permits use, sharing, adaptation, distribution and reproduction in any medium or format, as long as you give appropriate credit to the original author(s) and the source, provide a link to the Creative Commons licence, and indicate if changes were made. The images or other third party material in this article are included in the article's Creative Commons licence, unless indicated otherwise in a credit line to the material. If material is not included in the article's Creative Commons licence and your intended use is not permitted by statutory regulation or exceeds the permitted use, you will need to obtain permission directly from the copyright holder. To view a copy of this licence, visit http://creativecommons.org/licenses/by/4.0/ .
Reprints and permissions
About this article
Fučík, J., Fučík, S., Rexroth, S. et al. Pharmaceutical metabolite identification in lettuce ( Lactuca sativa ) and earthworms ( Eisenia fetida ) using liquid chromatography coupled to high-resolution mass spectrometry and in silico spectral library. Anal Bioanal Chem (2024). https://doi.org/10.1007/s00216-024-05515-2
Download citation
Received : 18 June 2024
Revised : 20 August 2024
Accepted : 22 August 2024
Published : 10 September 2024
DOI : https://doi.org/10.1007/s00216-024-05515-2
Share this article
Anyone you share the following link with will be able to read this content:
Sorry, a shareable link is not currently available for this article.
Provided by the Springer Nature SharedIt content-sharing initiative
- Pharmaceuticals
- Software prediction
- Metabolite identification in Eisenia fetida and Lactuca sativa
- Liquid chromatography
- High-resolution mass spectrometry
- In silico spectral library
- Find a journal
- Publish with us
- Track your research

U.S. Government Accountability Office
Small Business Research Programs: Opportunities Exist for SBA and Agencies to Reduce Vulnerabilities to Fraud, Waste, and Abuse
Federal agencies awarded small businesses more than $4.4 billion in FY 2022 for research and development and to commercialize technologies. The Small Business Administration oversees the programs making these awards, which are carried out by 11 agencies.
We analyzed past award data to identify awardees' foreign ownership, business size, and other indicators of fraud, waste, or abuse. We found, for example:
Almost 8% of awardees in our review had four or more indicators
$445 million worth of awards may have funded duplicative work
Our recommendations to SBA and 2 other agencies are to help improve their fraud risk management and more.
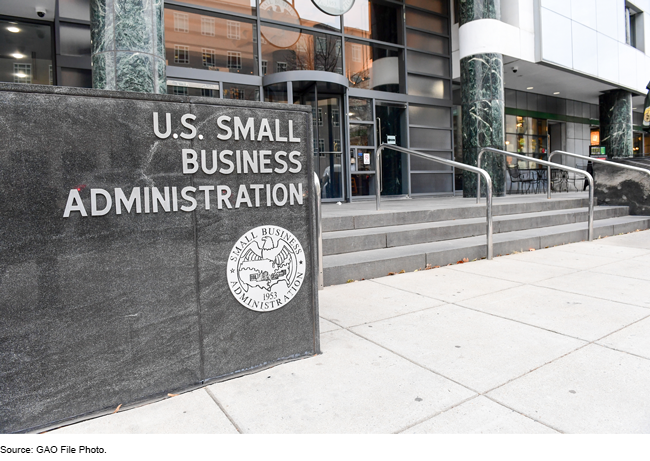
What GAO Found
GAO's analysis of 37 fraud schemes targeting the Small Business Innovation Research (SBIR) and Small Business Technology Transfer (STTR) programs demonstrates control vulnerabilities and fraud risks with a range of financial and other impacts. These schemes often involved multiple participating agencies and programs. For example, 25 schemes involved awards from more than one agency, and 14 involved both SBIR and STTR awards. GAO identified approximately $34.7 million in civil settlements associated with these schemes. Fraudsters' diversion of funds affects the programs' economic stimulus goals and makes funds unavailable to eligible businesses. It can also result in prison time, financial penalties, and loss of employment for those involved in the schemes.
In addition to its Policy Directive guidance, the U.S. Small Business Administration (SBA) uses several tools, including its monthly program manager meetings, annual survey to participating agencies, and listing of fraud convictions and civil liabilities on SBIR.gov, to monitor and support agencies' fraud, waste, and abuse prevention efforts. However, GAO identified opportunities for SBA to better leverage these tools. For example, some agencies were unaware of the requirement to report fraud convictions and civil liabilities for listing on SBIR.gov, limiting the site's usefulness as an information source and fraud deterrent.
Most agencies did not conduct SBIR/STTR fraud risk assessments in alignment with GAO's leading practices and identified lack of guidance, training, and resources as related challenges. Through its guidance and other tools, SBA is in a position to reinforce fraud risk assessment requirements for agencies, in support of Policy Directive goals for fraud, waste, and abuse prevention.
GAO's analysis of SBIR.gov award data from fiscal years 2016 through 2021 identified thousands of awardees with one or more fraud, waste, or abuse risk indicator. Among the 10,570 awardees in this period, 842 were associated with four or more such indicators. GAO designed 27 analytic tests for (1) applicant eligibility, including foreign ownership, business size, essentially equivalent work, research facility address; and (2) other fraud, waste, or abuse risks, such as having prior criminal or civil actions. Data quality issues in SBIR.gov, such as incomplete project summaries, may impede agencies' full use of analytics for managing these risks. By improving the data through guidance and verification, SBA can support agencies' risk management activities.
Awardees by Number of Fraud, Waste, or Abuse Risk Indicators Identified in Analytic Tests

Why GAO Did This Study
Since the inception of the programs, federal agencies have invested over $68 billion in SBIR/STTR awards for research and development and to commercialize technologies. SBA oversees the programs, which are carried out by 11 participating agencies. In response to the Small Business Act, as amended, the SBA established 10 minimum requirements for participating agencies to prevent fraud, waste, and abuse. The act also includes a provision that GAO report to Congress every 4 years on agencies' and their Offices of Inspector General (OIG) efforts related to fraud, waste, and abuse in the programs.
This GAO report, its fourth, assesses (1) SBIR/STTR fraud schemes from fiscal years 2016 through 2023 and participants and impacts; (2) SBA and agency antifraud activities against fraud, waste, and abuse requirements; (3) agency fraud risk assessments against leading practices; and (4) applicant and award data to identify fraud, waste, and abuse vulnerabilities.
GAO reviewed documentation from SBA and the 11 participating agencies and OIGs; analyzed criminal, civil, and administrative actions; compared SBA and agencies' processes against leading practices; conducted data matching to identify potentially ineligible awardees for fiscal years 2016 through 2021; and interviewed SBA, agency, and OIG officials.
Recommendations
GAO is making eight recommendations, including six to SBA to provide agencies with guidance to support their fraud risk management. The agencies generally agreed with the recommendations.
Recommendations for Executive Action
Recommendation | ||
---|---|---|
Small Business Administration | The Administrator of SBA should ensure that the Associate Administrator for the Office of Investment and Innovation expands the methods and sources used to identify fraud-related convictions and findings of civil liability to list in the SBA's database, such as through alerts from legal research resources. (Recommendation 1) | |
Small Business Administration | The Administrator of SBA should ensure that the Associate Administrator for the Office of Investment and Innovation leverages its oversight mechanisms to identify, share, and report fraud-related convictions and findings of civil liability to SBIR.gov and address participating agencies' challenges in understanding and meeting the 15-day reporting requirement. (Recommendation 2) | |
Department of Agriculture | The Secretary of the U.S. Department of Agriculture should ensure that the Director of the National Institute of Food and Agriculture ensures that USDA SBIR/STTR applicants receive fraud, waste, and abuse training. (Recommendation 3) | |
Department of Defense | The Secretary of Defense should ensure that the DOD Office of the Under Secretary of Defense for Research and Engineering SBIR/STTR Program ensures that DOD SBIR/STTR subcomponent program officials receive fraud, waste, and abuse training. (Recommendation 4) | |
Small Business Administration | The Administrator of SBA should ensure that the Associate Administrator for the Office of Investment and Innovation leverages its existing oversight mechanisms to ensure the accuracy of agencies' survey responses to required fraud, waste, and abuse training and, to the full extent of the SBA's legal authority, shares SBIR/STTR fraud risk information and resources for conducting fraud risk assessments. (Recommendation 5) | |
Small Business Administration | The Administrator of SBA should ensure that the Associate Administrator for the Office of Investment and Innovation, to the full extent of the SBA's legal authority, provides guidance to participating agencies to conduct comprehensive SBIR/STTR program fraud risk assessments, including all key elements, in support of the Policy Directive's fraud, waste, and abuse prevention requirements and consistent with Fraud Risk Framework leading practices. (Recommendation 6) | |
Small Business Administration | The Administrator of SBA should ensure that the Associate Administrator for the Office of Investment and Innovation improves SBIR.gov data quality by updating guidance to require that abstracts are sufficiently complete and that applicant and awardee addresses are verified to support program eligibility determinations. (Recommendation 7) | |
Small Business Administration | The Administrator of SBA should ensure that the Associate Administrator for the Office of Investment and Innovation validates existing information in the SBIR/STTR databases, specifically the Company Registry and SBIR.gov, to identify and correct deficiencies, as appropriate. (Recommendation 8) |
Full Report
Gao contacts.
Rebecca Shea Director [email protected] (202) 512-6722
Office of Public Affairs
Sarah Kaczmarek Acting Managing Director [email protected] (202) 512-4800
Role of earthworm biodiversity in soil fertility and crop productivity improvement
- October 2021
- In book: Biological Diversity: Current Status and Conservation Policies (pp.230-241)

- Gurukula Kangri Vishwavidyalaya

Abstract and Figures

Discover the world's research
- 25+ million members
- 160+ million publication pages
- 2.3+ billion citations
- Ghana S. Al-Hadithi
- Hassan A. A. Al-Saady

- Deshans Chaudhary
- K I M Perera

- Alix Delhal

- A. Antho-Nyreddy

- Rajiv K. Sinha

- Recruit researchers
- Join for free
- Login Email Tip: Most researchers use their institutional email address as their ResearchGate login Password Forgot password? Keep me logged in Log in or Continue with Google Welcome back! Please log in. Email · Hint Tip: Most researchers use their institutional email address as their ResearchGate login Password Forgot password? Keep me logged in Log in or Continue with Google No account? Sign up

IMAGES
VIDEO
COMMENTS
Global data on earthworm abundance, biomass, diversity ...
Earthworms contribute significantly to global food production
Earthworms, as ecosystem engineers by nature (), influence the structure and functioning of terrestrial ecosystems.Through their burrowing activities, earthworms promote the stabilization of soil particles into aggregates, increase soil porosity, and elevate the rates at which water infiltrates soil during rainfall; reduce erosion of surface soils from hillslopes; and accelerate the movement ...
Fig. 1 Global distribution of earthworm diversity. (A) Black dots represent the center of a "study" used in at least one of the three models (species richness, total abundance, and total biomass). The size of the dot corresponds to the number of sites within the study. Opaqueness is for visualization purposes only.
Although there has been much research into the role of earthworms in soil ecology, this review demonstrates substantial gaps in our knowledge related in particular to difficulties in identifying the effects of species, land use and climate. The review aims to assist people involved in all aspects of land management, including conservation ...
Global earthworm distribution and activity windows based ...
Explore the latest full-text research PDFs, articles, conference papers, preprints and more on EARTHWORMS. Find methods information, sources, references or conduct a literature review on EARTHWORMS
Applied aspects of earthworm research can also be achieved through use of simple techniques to enhance population development and even population dynamics can be directly addressed with use of relatively inexpensive, effective marking techniques. ... Many avenues of research are available and this article could very easily seek to review and ...
Over the past 30 years, 1310 original research articles on earthworms in croplands have been published. A steady increase was observed until 2006, and since approximately 2015, there has been an exponential upward trend, reaching more than 60 publications annually (Fig. 1), which indicates that the number of publications continues to increase ...
The complexity of the soil system, and the earthworms' interactions with it, means that determining field variability is likely to remain a research challenge until novel methods for rapid assessment are developed into practical tools, such as the identification of species from earthworm cast or genetic material [44], [51]; however, these ...
Earthworm Study Opens New Dimension in Climate Science. October 24, 2019. Research & Discovery. As the world waxes warmer, scientists are scrambling to prioritize conservation efforts. A new global study of earthworm diversity suggests looking at soil, as climate change could seriously affect earthworms and the many functions they provide.
In agro-ecosystems, earthworm communities are generally very sensitive to physico-chemical properties of the soil which directly or indirectly influence the availability of resources for earthworm survival [].The soil structure and its pore size also play a key role in the distribution of the earthworms within the soil and a minute changes in the same can adversely affect the earthworm's ...
animal biomass and are commonly termed 'ecosystem engineers'. This review considers the contribution. of earthworms to ecosystem services through pedogenesis, development of soil structure ...
To assess the ecological risk of microplastics (MPs) in agricultural systems, it is critical to simultaneously focus on MP-mediated single-organism response and different trophic-level organism interaction. Herein, we placed earthworms in soils contaminated with different concentrations (0.02% and 0.2% w/w) of polyethylene (PE) and polypropylene (PP) MPs to investigate the effect of earthworms ...
Among the research articles, the most used earthworm species involved in the PTEs vermiremediation is Eisenia fetida. E. fetida is an epigeic earthworm employed in terrestrial ecotoxicology as the standard test organism for the following reasons: it is easy to breed in laboratory, has short generation times and is susceptible to chemicals [79,80].
Earthworms increase plant production: a meta-analysis
The aim of this review is to generate awareness and understand the importance of earthworms in sustainable agriculture and effect of pesticides on their action. The natural resources are finite and highly prone to degradation by the misuse of land and mismanagement of soil. The world is in utter need of a healthy ecosystem that provides with fertile soil, clean water, food and other natural ...
Prof Olaf Schmidt (Associate Editor, University College Dublin) and Dr Felicity Crotty (Associate Editor, Royal Agricultural University) To meet the recent resurgence in interest in earthworm research, this Virtual Issue provides Free Access for a limited period to selected papers that have explored the role of earthworms in soils and soil processes in the European Journal of Soil Science and ...
Earthworms also possess a strong impact on the soil microbiome. It is present in the soil rhizosphere and significantly adds to about 80% of the biomass in the soil macrofauna (Yasmin and D'Souza ...
Effects of Three Pesticides on the Earthworm Lumbricus ...
Earthworm effects on GHG emissions and SOC. Our meta-analysis strongly suggests that earthworms increase net soil GHG emissions. Earthworm presence increased soil N 2 O emissions by 42% and soil ...
Here we show, using meta-analysis, that on average earthworm presence in agroecosystems leads to a 25% increase in crop yield and a 23% increase in aboveground biomass. The magnitude of these effects depends on presence of crop residue, earthworm density and type and rate of fertilization. The positive effects of earthworms become larger when ...
A new project will map colonies of endangered giant Gippsland earthworms on farms. The worms, among the biggest in the world, grow to one metre long and are rarely seen above ground. Under the ...
use of articles in any medium, reproduction and distribution by providing adequate credit to the author (s) and the source of publication. quali ties (Deswal et al ., 2020; Singh and F atim a, 2022) .
Pharmaceuticals released into the aquatic and soil environments can be absorbed by plants and soil organisms, potentially leading to the formation of unknown metabolites that may negatively affect these organisms or contaminate the food chain. The aim of this study was to identify pharmaceutical metabolites through a triplet approach for metabolite structure prediction (software-based ...
Among the 10,570 awardees in this period, 842 were associated with four or more such indicators. GAO designed 27 analytic tests for (1) applicant eligibility, including foreign ownership, business size, essentially equivalent work, research facility address; and (2) other fraud, waste, or abuse risks, such as having prior criminal or civil actions.
The earthworms are the biological indicator of the soil ecosystem as they indicate the health and fertility of the soil for proper cropping. Systematic of earthworm distribution based on the ...