
An official website of the United States government
The .gov means it’s official. Federal government websites often end in .gov or .mil. Before sharing sensitive information, make sure you’re on a federal government site.
The site is secure. The https:// ensures that you are connecting to the official website and that any information you provide is encrypted and transmitted securely.
- Publications
- Account settings
- My Bibliography
- Collections
- Citation manager

Save citation to file
Email citation, add to collections.
- Create a new collection
- Add to an existing collection
Add to My Bibliography
Your saved search, create a file for external citation management software, your rss feed, tay-sachs disease, affiliations.
- 1 University of Arkansas Medical Science Center
- 2 The Children Hospital at Westmead Clinical School, Sydney Medical school, The faculty of Medicine and Health, The University of Sydney, NSW, Australia
- PMID: 33232090
- Bookshelf ID: NBK564432
Tay Sachs disease (TSD) is a progressive, lethal neurodegenerative disorder caused by a deficiency of enzyme hexosaminidase-A resulting in the accumulation of GM2 gangliosides. Based on the presentation age, the disease is classified into infantile, juvenile, and adult forms. Early diagnosis of Tay Sachs is clinically challenging because of subtle clinical features and nonspecific biochemical findings. Accurate diagnosis is important both for proper management and to reduce complications associated with the disease.
Copyright © 2024, StatPearls Publishing LLC.
PubMed Disclaimer
Conflict of interest statement
Disclosure: Praveen Kumar Ramani declares no relevant financial relationships with ineligible companies.
Disclosure: Bindu Parayil Sankaran declares no relevant financial relationships with ineligible companies.
- Continuing Education Activity
- Introduction
- Epidemiology
- Pathophysiology
- Histopathology
- History and Physical
- Treatment / Management
- Differential Diagnosis
- Complications
- Consultations
- Deterrence and Patient Education
- Enhancing Healthcare Team Outcomes
- Review Questions
Similar articles
- HEXA Disorders. Toro C, Shirvan L, Tifft C. Toro C, et al. 1999 Mar 11 [updated 2020 Oct 1]. In: Adam MP, Feldman J, Mirzaa GM, Pagon RA, Wallace SE, Bean LJH, Gripp KW, Amemiya A, editors. GeneReviews ® [Internet]. Seattle (WA): University of Washington, Seattle; 1993–2024. 1999 Mar 11 [updated 2020 Oct 1]. In: Adam MP, Feldman J, Mirzaa GM, Pagon RA, Wallace SE, Bean LJH, Gripp KW, Amemiya A, editors. GeneReviews ® [Internet]. Seattle (WA): University of Washington, Seattle; 1993–2024. PMID: 20301397 Free Books & Documents. Review.
- GM2 ganglioside accumulation causes neuroinflammation and behavioral alterations in a mouse model of early onset Tay-Sachs disease. Demir SA, Timur ZK, Ateş N, Martínez LA, Seyrantepe V. Demir SA, et al. J Neuroinflammation. 2020 Sep 20;17(1):277. doi: 10.1186/s12974-020-01947-6. J Neuroinflammation. 2020. PMID: 32951593 Free PMC article.
- Progranulin associates with hexosaminidase A and ameliorates GM2 ganglioside accumulation and lysosomal storage in Tay-Sachs disease. Chen Y, Jian J, Hettinghouse A, Zhao X, Setchell KDR, Sun Y, Liu CJ. Chen Y, et al. J Mol Med (Berl). 2018 Dec;96(12):1359-1373. doi: 10.1007/s00109-018-1703-0. Epub 2018 Oct 20. J Mol Med (Berl). 2018. PMID: 30341570 Free PMC article.
- Infantile Monosialoganglioside2 (GM2) Gangliosidosis With Concurrent Bronchopneumonia: An Extraordinary Case of Tay-Sachs Disease. Grezenko H, Al-Deir SS, Eshete FD, Faran N, Mimms CS, Ibrahim M. Grezenko H, et al. Cureus. 2024 Jan 7;16(1):e51797. doi: 10.7759/cureus.51797. eCollection 2024 Jan. Cureus. 2024. PMID: 38322066 Free PMC article.
- The natural history of juvenile or subacute GM2 gangliosidosis: 21 new cases and literature review of 134 previously reported. Maegawa GH, Stockley T, Tropak M, Banwell B, Blaser S, Kok F, Giugliani R, Mahuran D, Clarke JT. Maegawa GH, et al. Pediatrics. 2006 Nov;118(5):e1550-62. doi: 10.1542/peds.2006-0588. Epub 2006 Oct 2. Pediatrics. 2006. PMID: 17015493 Free PMC article. Review.
- Gravel RA, Triggs-Raine BL, Mahuran DJ. Biochemistry and genetics of Tay-Sachs disease. Can J Neurol Sci. 1991 Aug;18(3 Suppl):419-23. - PubMed
- Lew RM, Burnett L, Proos AL, Delatycki MB. Tay-Sachs disease: current perspectives from Australia. Appl Clin Genet. 2015;8:19-25. - PMC - PubMed
- Rozenberg R, Pereira Lda V. The frequency of Tay-Sachs disease causing mutations in the Brazilian Jewish population justifies a carrier screening program. Sao Paulo Med J. 2001 Jul 05;119(4):146-9. - PMC - PubMed
- Solovyeva VV, Shaimardanova AA, Chulpanova DS, Kitaeva KV, Chakrabarti L, Rizvanov AA. New Approaches to Tay-Sachs Disease Therapy. Front Physiol. 2018;9:1663. - PMC - PubMed
- Dersh D, Iwamoto Y, Argon Y. Tay-Sachs disease mutations in HEXA target the α chain of hexosaminidase A to endoplasmic reticulum-associated degradation. Mol Biol Cell. 2016 Dec 01;27(24):3813-3827. - PMC - PubMed
Publication types
- Search in PubMed
- Search in MeSH
- Add to Search
Related information
- Cited in Books
LinkOut - more resources
Full text sources.
- NCBI Bookshelf

- Citation Manager
NCBI Literature Resources
MeSH PMC Bookshelf Disclaimer
The PubMed wordmark and PubMed logo are registered trademarks of the U.S. Department of Health and Human Services (HHS). Unauthorized use of these marks is strictly prohibited.
Tay-Sachs disease
- Revista de la Facultad de Medicina, Universidad Nacional de Colombia 67(3):323-329
- 67(3):323-329

- Universidad Pedagógica y Tecnológica de Colombia
- This person is not on ResearchGate, or hasn't claimed this research yet.
Abstract and Figures

Discover the world's research
- 25+ million members
- 160+ million publication pages
- 2.3+ billion citations
- Curr Pediatr Rev

- L.J. Posso Gomez
- Verónica Botero

- Atsushi Kato
- Izumi Nakagome
- Shinpei Nakagawa

- ARCH MED RES

- Diogo Ribeiro

- Am Jew Year Bk

- M.C. Patterson
- HUM GENE THER

- Ashley N. Randle
- Stacy A. Maitland
- Douglas R. Martin
- J Christ Nurs
- Tricia Clayton

- Recruit researchers
- Join for free
- Login Email Tip: Most researchers use their institutional email address as their ResearchGate login Password Forgot password? Keep me logged in Log in or Continue with Google Welcome back! Please log in. Email · Hint Tip: Most researchers use their institutional email address as their ResearchGate login Password Forgot password? Keep me logged in Log in or Continue with Google No account? Sign up
Thank you for visiting nature.com. You are using a browser version with limited support for CSS. To obtain the best experience, we recommend you use a more up to date browser (or turn off compatibility mode in Internet Explorer). In the meantime, to ensure continued support, we are displaying the site without styles and JavaScript.
- View all journals
- Explore content
- About the journal
- Publish with us
- Sign up for alerts
- Correspondence
- Open access
- Published: 06 July 2021
Efficient and precise generation of Tay–Sachs disease model in rabbit by prime editing system
- Yuqiang Qian 1 na1 ,
- Ding Zhao 1 na1 ,
- Tingting Sui 1 na1 ,
- Mao Chen 1 ,
- Zhiquan Liu 1 ,
- Hongmei Liu 1 ,
- Tao Zhang 1 ,
- Siyu Chen 1 ,
- Liangxue Lai 1 , 2 &
- Zhanjun Li 1
Cell Discovery volume 7 , Article number: 50 ( 2021 ) Cite this article
5476 Accesses
22 Citations
1 Altmetric
Metrics details
- Biological techniques
- Metabolomics
Dear Editor,
Tay–Sachs disease (TSD) is a progressive neurodegenerative disorder due to an autosomal recessively inherited deficiency of β-hexosaminidase A (HexA) 1 . The four-bases (TATC) insertion in exon 11 of the HEXA ( HEXA ins TATC) accounts for 80% of Tay–Sachs disease from the Ashkenazi Jewish population 2 . However, no typical clinical phenotypes, such as neurological abnormalities, the restricted pattern of distribution of GM2-ganglioside and membranous cytoplasmic bodies in the brain, were observed in HEXA −/− mouse models, due to the difference in the ganglioside degradation pathways in mice and human 3 . Thus, it is desired to generate an ideal animal model to accurately mimic HEXA ins TATC in TSD patients. CRISPR–Cas9 system-mediated HDR 4 has been used to generate the mutation of HEXA ins TATC, however, low efficiency and high indels impede its application.
Recently Anzalone et al. 5 described a “search-and-replace” genome editing technology named prime editing (PE) that mediates 12 possible base-to-base conversions, without requiring DSBs or donor DNA templates in human cells. In addition, a previous study showed that, compared to mice, the late onset of TSD in adult rabbits 6 shared more similarities with human regarding physiology, anatomy, and genetics 7 . Thus, we generated a novel TSD rabbit model using the PE system, and characterized the typical phenotype of muscle weakness, ataxia, and mental disorders in the HEXA ins TATC rabbit model.
We first validated the editing efficiencies of PEs (PE2, PE3, PE3b) in HEK293FT cells at fifteen loci: five loci for base insertion, eight loci for base substitutions, and two loci for base deletion (Supplementary Table S1 ). Sanger sequencing results showed that the base insertion at a frequency from 4% to 22% (Fig. 1a and Supplementary Fig. S2 ), the base substitutions at a frequency from 4% to 36%, and the base deletion at a frequency from 7% to 12% were determined using PEs (Supplementary Figs. S1 and S2 ), respectively. These results indicate that PEs were effective in generating base insertion, substitution, and deletion in HEK293FT cells.
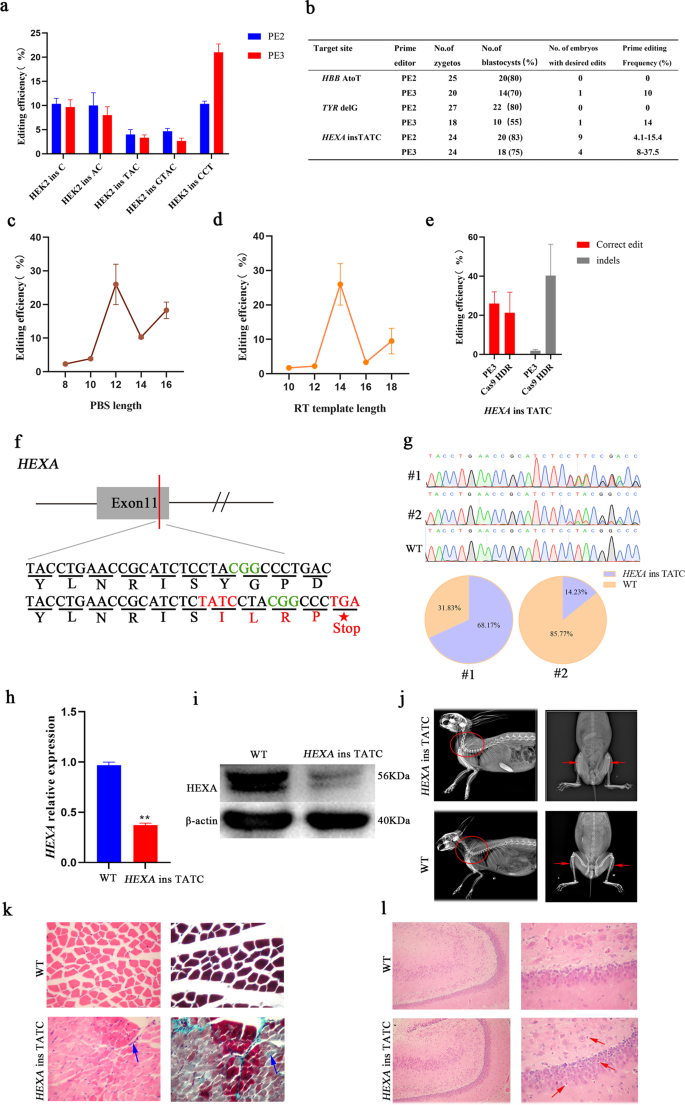
a The base insertion efficiency of PE system in HEK293FT cells. b PE induced efficient and precise gene editing in rabbit embryos. c Editing frequency ( HEXA ins TATC) of PegRNA screening with PBS length (8–16 nt) in rabbit embryos. d Editing frequency ( HEXA ins TATC) of PegRNA screening with RT template length (10–18 nt) in rabbit embryos. e Editing frequency ( HEXA ins TATC) of CRISPR–Cas9 system-mediated HDR compare with PE3. f The target sequence at HEXA locus by PE system. The PAM and sgRNA target sequences are shown in green and black, target mutation (red), frameshift mutation leads to PTC mutation (red and red star). g Editing frequency determination of HEXA ins TATC rabbit by deep sequencing. h Expression of HEXA gene was determined by qRT-PCR. i HEXA protein was determined by western blot. j X-ray radiography of WT and HEXA ins TATC rabbits. Red circle, increased cervical lordosis; Red arrows, clasping of the limbs. k Masson’s trichrome staining of gastrocnemius from WT and HEXA ins TATC rabbits. Blue arrow highlights the myopathy with fibrosis and inflammatory cell infiltration. l HE staining of hippocampus from WT and HEXA heterozygous rabbits. The red arrow highlights the enlargement of perineural space.
Next, we tested the efficiency of the PE system in rabbit embryos at three gene loci of HEXA , HBB , and TYR , which are associated with clinical diseases in ClinVar data 8 (Supplementary Table S2 ). Sanger sequencing results showed that 9 of 20 desired HEXA ins TATC were determined using PE2 with the efficiency of 4.1%–15.4%, while the efficiency is 8%–37.5% using PE3. In addition, 1 of 14 desired HBB with an efficiency of 10% and 1 of 10 desired TYR with an efficiency of 14% were generated using PE3, while there is no desired mutation was detected for these two sites using PE2 (Fig. 1b and Supplementary Fig. S3 ).
We then targeted the HEXA ins TATC to test the efficiency of the PegRNA PBS length (8–16 nt) and RT template length (10–18 nt) in rabbit embryos. TIDE analyzing 9 revealed significantly higher editing efficiencies by using PegRNA with 12 nt PBS and 14 nt RT template (Fig. 1c, d and Supplementary Table S3 ). Additionally, the significantly increased undesired indels were determined by using CRISPR–Cas9 system-mediated HDR (Fig. 1e and Supplementary Tables S3 , S8 ), which is consistent with the previous study 4 . Thus, PE3 with 12 nt PBS and 14 nt RT template was used for the generation of HEXA ins TATC rabbits in the following study.
The HEXA ins TATC introduces a premature termination codon (PTC) in exon 11, which leads to deficient activity of the hexosaminidase A (HexA) 10 (Fig. 1f ). In this study, 2 of 4 HEXA ins TATC rabbits were determined using Sanger sequencing and targeted deep sequencing, with the 68.17% and 14.23% mutation efficiency for #1 and #2 pups, respectively (Fig. 1g ). Furthermore, no sgRNA sequence-depended off-target mutations in HEXA ins TATC rabbits were found by deep sequencing (Supplementary Fig. S4a, b ), suggesting the accuracy of PE system-mediated HEXA ins TATC mutations in rabbits.
Furthermore, the heritability of HEXA ins TATC in rabbits was determined by Sanger sequencing (Supplementary Fig. S5 ), qRT-PCR (Fig. 1h ), and western blot (Fig. 1i ). The results showed a significantly reduced HEXA in HEXA ins TATC rabbits compared with WT controls. The typical phenotypes of the increasingly frequent of head raising, convulsions (Supplementary Fig. S6a and Movies S1 , S2 ), abnormal gait with decreased sway length (Supplementary Fig. S6b and Movies S1 , S2 ), clasping of the limbs, and increased cervical lordosis (Fig. 1j ), muscle fibrosis (Fig. 1k ) and enlargement of perineural space (Fig. 1l ) were also determined in HEXA ins TATC rabbits when compared with WT controls. These phenotypes were similar with late-onset or chronic adult gangliosiderosis in TSD patient exhibiting as limb-girdle weakness, followed by the development of ataxia and progressive neuromuscular weakness 11 .
In summary, this study for the first time verified the feasibility of PE system-mediated base insertions, deletions, and conversions in rabbit. This ideal and novel HEXA ins TATC rabbit model would be beneficial for the pathogenic mechanism study and drug screening to treat TSD in the future.
Kolodny, E. H. Molecular genetics of the beta-hexosaminidase isoenzymes: an introduction. Adv. Genet. 44 , 101–126 (2001).
Article CAS Google Scholar
Frisch, A. et al. Origin and spread of the 1278insTATC mutation causing Tay-Sachs disease in Ashkenazi Jews: genetic drift as a robust and parsimonious hypothesis. Hum. Genet. 114 , 366–376 (2004).
Article Google Scholar
Phaneuf, D. et al. Dramatically different phenotypes in mouse models of human Tay-Sachs and Sandhoff diseases. Hum. Mol. Genet. 5 , 1–14 (1996).
Jasin, M. & Haber, J. E. The democratization of gene editing: Insights from site-specific cleavage and double-strand break repair. DNA Repair 44 , 6–16 (2016).
Anzalone, A. V. et al. Search-and-replace genome editing without double-strand breaks or donor DNA. Nature 576 , 149–157 (2019).
Rickmeyer, T. et al. GM2 gangliosidosis in an adult pet rabbit. J. Comp. Pathol. 148 , 243–247 (2013).
Wang, Y. et al. Generation of knockout rabbits using transcription activator-like effector nucleases. Cell Regen. 3 , 3 (2014).
Article PubMed PubMed Central Google Scholar
Landrum, M. J. et al. ClinVar: public archive of interpretations of clinically relevant variants. Nucleic Acids Res. 44 , D862–D868 (2016).
Brinkman, E. K., Chen, T., Amendola, M. & van Steensel, B. Easy quantitative assessment of genome editing by sequence trace decomposition. Nucleic Acids Res. 42 , e168 (2014).
Myerowitz, R. & Costigan, F. C. The major defect in Ashkenazi Jews with Tay-Sachs disease is an insertion in the gene for the alpha-chain of beta-hexosaminidase. J. Biol. Chem. 263 , 18587–18589 (1988).
Jeyakumar, M. et al. An inducible mouse model of late onset Tay-Sachs disease. Neurobiol. Dis. 10 , 201–210 (2002).
Download references
Acknowledgements
We thank Peiran Hu and Nannan Li for assistance at the Embryo Engineering Center for critical technical assistance. This study was financially supported by the National Key Research and Development Program of China Stem Cell and Translational Research (2019YFA0110700). The Strategic Priority Research Program of the Chinese Academy of Sciences (XDA16030501, XDA16030503), Key Research & Development Program of Guangzhou Regenerative Medicine, and Health Guangdong Laboratory (2018GZR110104004).
Author information
These authors contributed equally: Yuqiang Qian, Ding Zhao, Tingting Sui
Authors and Affiliations
Key Laboratory of Zoonosis Research, Ministry of Education, College of Animal Science, Jilin University, Changchun, Jilin, China
Yuqiang Qian, Ding Zhao, Tingting Sui, Mao Chen, Zhiquan Liu, Hongmei Liu, Tao Zhang, Siyu Chen, Liangxue Lai & Zhanjun Li
CAS Key Laboratory of Regenerative Biology, Guangdong Provincial Key Laboratory of Stem Cell and Regenerative Medicine, South China Institute for Stem Cell Biology and Regenerative Medicine, Guangzhou Institutes of Biomedicine and Health, Chinese Academy of Sciences, Guangzhou, Guang Dong, China
Liangxue Lai
You can also search for this author in PubMed Google Scholar
Contributions
Y.Q., L.L., and Z.Li. conceived and designed the experiments. Y.Q., D.Z., and T.Z. performed the experiments. Z.Liu., M.C., S.C. and Y.Q. analyzed the data. M.C., H.L., and T.S. contributed reagents/materials/analysis tools. Y.Q. and D.Z. wrote the paper. All authors have read and approved the final manuscript.
Corresponding authors
Correspondence to Liangxue Lai or Zhanjun Li .
Ethics declarations
Conflict of interest.
The authors declare no competing interests.
Additional information
Publisher’s note Springer Nature remains neutral with regard to jurisdictional claims in published maps and institutional affiliations.
Supplementary information
Supplymentary data, supplementary movie s1, supplementary movie s2, rights and permissions.
Open Access This article is licensed under a Creative Commons Attribution 4.0 International License, which permits use, sharing, adaptation, distribution and reproduction in any medium or format, as long as you give appropriate credit to the original author(s) and the source, provide a link to the Creative Commons license, and indicate if changes were made. The images or other third party material in this article are included in the article’s Creative Commons license, unless indicated otherwise in a credit line to the material. If material is not included in the article’s Creative Commons license and your intended use is not permitted by statutory regulation or exceeds the permitted use, you will need to obtain permission directly from the copyright holder. To view a copy of this license, visit http://creativecommons.org/licenses/by/4.0/ .
Reprints and permissions
About this article
Cite this article.
Qian, Y., Zhao, D., Sui, T. et al. Efficient and precise generation of Tay–Sachs disease model in rabbit by prime editing system. Cell Discov 7 , 50 (2021). https://doi.org/10.1038/s41421-021-00276-z
Download citation
Received : 25 November 2020
Accepted : 22 April 2021
Published : 06 July 2021
DOI : https://doi.org/10.1038/s41421-021-00276-z
Share this article
Anyone you share the following link with will be able to read this content:
Sorry, a shareable link is not currently available for this article.
Provided by the Springer Nature SharedIt content-sharing initiative
Quick links
- Explore articles by subject
- Guide to authors
- Editorial policies


An official website of the United States government
The .gov means it’s official. Federal government websites often end in .gov or .mil. Before sharing sensitive information, make sure you’re on a federal government site.
The site is secure. The https:// ensures that you are connecting to the official website and that any information you provide is encrypted and transmitted securely.
- Publications
- Account settings
Preview improvements coming to the PMC website in October 2024. Learn More or Try it out now .
- Advanced Search
- Journal List
- BMJ Case Rep

Case Report
Tay-sachs disease: a novel mutation from india, daisy khera.
1 Department of Pediatrics, All India Institute of Medical Sciences, Jodhpur, Jodhpur, Rajasthan, India
Joseph John
2 Department of Pediatrics, All India Institute of Medical Sciences Bhubaneswar, Bhubaneswar, Odisha, India
Kuldeep Singh
Mohammed faruq.
3 Genomics and Molecular Medicine, CSIR-Institute of Genomics and Integrative Biology, New Delhi, India
Lysosomal storage disorders or lipidoses are a wide spectrum of inherited diseases caused by deficiency of a specific lysosomal hydrolase. About 134 mutations have been described so far and this number is gradually increasing with newer mutations being reported. We report a 28-month-old child who presented to us with neurodevelopment regression, seizures and cherry red spot in both eyes. His hexosaminidase A enzyme activity was reduced and genetic testing revealed a homozygous novel variation in HEXA (hexosaminidase A) gene in the DNA sample of the patient.
Lysosomal storage disorders (LSDs) are caused by deficiency of a specific lysosomal hydrolase. One of these LSDs is Tay-Sachs disease (TSD) (MIM ID # 272800) which has an autosomal recessive inheritance caused by a genetic mutation in the HEXA gene on human chromosome 15q23–q24. TSD is the second most common lipid storage disorder among the reported lipidoses in India. These reported mutations have included single base pair deletion or multiple base pair insertions, splice site mutations, missense mutations and nonsense mutations. We report a previously unreported missense mutation c.G619C:p.D207H in the HEXA gene in a case of TSD.
Case presentation
A 28-month-old male child, Muslim by religion, presented to us with delayed attainment of developmental milestones and seizures since the latter half of infancy. He was born to second degree consanguineous parents. The mother of the child had history of one spontaneous abortion at 4 months of gestation. This child was born to a 21-year-old, third gravida mother at term gestation, was small for gestational age and weighed 2.2 kg. He required routine care at birth and had normal APGAR scores. His elder sibling was a girl child who was alive and healthy. All seemed well until about 9 months of age after which, a delay in the attainment of newer milestones was noticed by the parents. They were hopeful that he would improve on his own, when at 15 months of age, he had generalised seizures, which made them seek medical attention. After this episode of seizure, he was noticed to have neuroregression in all domains of development. Subsequently, he was on antiepileptic drugs (AEDs) and was on irregular follow-up at various centres but with no significant improvement.
He was brought to our outpatient department at the age of 26 months for evaluation. His vitals were stable and he had severe acute malnutrition on anthropometric examination. His weight was 8.5 kg (less than −3 z score), height was 85 cm (at −2 z score) and weight for height was less than −3 z score. His occipitofrontal circumference was 52 cm which was more than 2 z score for his age suggestive of macrocephaly. He had severe global developmental delay with a developmental age of about 3–4 months. He had no dysmorphism or neurocutaneous stigmata. He was conscious, not focusing or following objects. He had an exaggerated startle response to sound and had no organomegaly. He had symmetrically decreased bulk of muscles, power of 3/5 in all limbs, hypertonia of all limbs with exaggerated deep tendon reflexes and extensor plantar response. His cranial nerve examination was normal. Rest of the systemic examination was unremarkable. His fundus examination revealed bilateral optic atrophy and cherry red spots. His investigations are detailed in table 1 . MRI of the brain showed symmetrical hypomyelination along the internal capsule and bihemispheric white matter ( figure 1 ).
Haematological, biochemical and genetic investigations
Hb (11.5–14 g/dL) | 11.6 |
TLC (6000–14000/mm ) | 7650 |
N (22%–48%) | 41% |
PLT (1.5–4.0 lakhs) | 3.3 |
ALT (13–45 U/L) | 21 |
AST (9–80 U/L) | 30 |
Urea (5–18 mg/dL) | 21 |
Creatinine (0.2–0.4 mg/dL) | 0.28 |
Sodium (130–145 mEq/L) | 139 |
Potassium (4.1–5.3 mEq/L) | 4.3 |
Calcium (9–11 mg/dL) | 9.8 |
Phosphorus (4–6.5 mg/dL) | 4.7 |
ALP (150–420 U/L) | 157 |
Total hexosaminidase enzyme with fluorometric assay using 4-methylumbelliferone (800–1600 nmol/hour/mL) | 1650 nmol/hour/mL |
Hexosaminidase A enzyme assay (464–1088 nmol/hour/mL) | 26 nmol/hour/mL |
Hexosaminidase B enzyme assay (336–512 nmol/hour/mL) | 1624 nmol/hour/mL |
Genetic testing (Sanger sequencing) | Homozygous missense variation in exon 6 of the HEXA (hexosaminidase A) gene (chr15: 72643527C>G; c.619G>C; p.D207H) |
ALT, alanine transaminase; ALP, alkaline phosphatase; AST, aspartate transaminase; Hb, haemoglobin; N, neutrophils; PLT, platelet count; TLC, total leucocyte count.

Axial fluid attenuation inversion recovery images showing symmetrical hypomyelination along the internal capsule and bihemispheric white matter.
Differential diagnosis
A provisional diagnosis of TSD/Sandhoff disease was considered.
He was treated with syrup valproic acid and levetiracetam. He was referred to physical medicine and rehabilitation department for multisensory stimulation, physiotherapy and occupational therapy. Prognosis was explained to parents and option of prenatal diagnosis in future pregnancies was explained.
Outcome and follow-up
The child continued to have recurrent seizures and the doses of AEDs were subsequently increased. The child was admitted with lower respiratory tract infection with septic shock to our paediatric intensive care unit at 3.5 years of age and succumbed to the illness. His mother conceived again and was offered prenatal diagnosis. In the fetus, this variation was detected in heterozygous condition. He is now a healthy infant.
Neuroregression in childhood could either be due to genetic causes with neurometabolic origin or non-genetic causes such as infections and toxins. LSDs are one of the causes of neuroregression in children. 1 LSDs are the heritable group of nearly 40 heterogeneous disorders occurring due to genetic defect in one or more specific lysosomal enzymes, activator protein or membrane protein resulting in deficient enzyme activity. 2 3 GM2 gangliosidosis includes TSD and Sandhoff disease. 4 Both types of GM2 gangliosidoses are inherited autosomal recessively with the incidence being as high as 1 in 2500 to 3900 in Ashkenazi Jews. It is relatively rare in India and the exact incidence is not known. Both types are caused from a deficiency of β-hexosaminidase activity and accumulation of GM2 gangliosides mostly in the nervous system or viscera but can also result from defects involved in lysosomal enzyme trafficking or lysosomal enzyme activator proteins. 5 Children with activator protein deficiency, though phenotypically fit into TSD the levels of enzyme activity of hexosaminidase A and total hexosaminidase enzyme activity in the leucocytes would surprisingly be normal. A high index of suspicion is needed in such cases and activator protein deficiency should be looked for. The pathway of metabolism of sphingolipids in nervous tissue and visceral organs is well described. The age of presentation and clinical manifestations of LSDs depend on the amount and rate of intracellular substrates accumulation, the quantum of remaining enzyme which is functional and presence of any alternative functional pathways. 6 Both TSD and Sandhoff disease have infantile, juvenile and adult-onset types based on the onset of symptoms.
β-hexosaminidase has two subunits: β-hexosaminidase A (one α and one β-subunit) and β-hexosaminidase B (two β-subunits). A mutation in the α-subunit gene causes TSD while that in the β-subunit chain gene causes a deficiency of both β-hexosaminidase A and B causing Sandhoff disease. Thus, assessment of β-hexosaminidase enzyme activity in peripheral leucocytes, cultured fibroblasts or lymphoblasts can identify affected individuals and determines the diagnosis. In TSD, only the β-hexosaminidase A isoenzyme is deficient while in Sandhoff disease β-hexosaminidase A and B both isoenzymes are deficient.
TSD is the second most common lipid storage disorder among the majority of LSDs studied in India. 7 The usual manifestations of TSD presenting in infancy are developmental regression, decreased eye contact, exaggerated startle response, macrocephaly not associated with hydrocephalus, macular pallor and a cherry red retinal spot. By the second year of life, the child usually has refractory seizures, relentless neuroregression and death by the fourth or fifth year. The most common causes of morbidity and mortality in LSDs are due to neurological, visceral (cardiovascular and skeletal) accumulation of glycosphingolipids. 8 9 There is no known cure or treatment at present though carrier detection can help in prevention of disease. Prenatal diagnosis is usually done using specific enzyme assay of the chorionic villus sample or cultured amniocytes. But this may give erroneous results due to problems with regard to technical expertise, sample transportation and maternal tissue contamination. If the mutation is identified in the proband or in the carrier parents, a targeted mutation analysis in the fetal DNA can also help diagnose the disease in the fetus. A combination of molecular genetic testing along with enzyme assay has been found to significantly increase the reliability of the prenatal diagnostic procedure 10 and guide genetic counselling. The limitation with molecular genetic testing is the limited availability of advanced centres for such testing and the high cost.
There are over 130 mutations described in TSD, most being in the infantile variety. 11 The most commonly reported ones are the missense mutations (1) c.532CNT (p.R178C), (2) c.964GNT (p.D322Y), (3) c.1385ANT (p.E462V), (4) c.1277_1278insTATC (5) p.D175A (6) p.G269R; the nonsense mutation p.R510X, splice site mutation c.459+5G>A and deletions (1 bp deletion c.425deIT). 9
Learning points
- All children with a history of neurodevelopment regression should undergo fundus examination for cherry red spots and should be evaluated for abnormal startle response to noise and if present should be investigated for hexosaminidase enzyme activity.
- Genetic testing of such cases is important to establish the diagnosis.
- Genetic counselling and prenatal diagnosis should be offered to the parents of such cases.
Acknowledgments
We would like to acknowledge MLP1601, Genomics and other omics tools for enabling Medical Decision (GOMED) project from CSIR for funding the genetic analysis in this case.
Patient consent for publication: Parental/guardian consent obtained.
Contributors: DK made substantial contributions to the conception of the work, drafting the work, revising it critically for important intellectual content and final approval of the version to be published and agreed to be accountable for all aspects of the work in ensuring that questions related to the accuracy or integrity of any part of the work are appropriately investigated and resolved. JJ made substantial contributions to the conception of the work, drafting the work, revising it critically for important intellectual content and final approval of the version to be published and agreed to be accountable for all aspects of the work in ensuring that questions related to the accuracy or integrity of any part of the work are appropriately investigated and resolved. KS made substantial contributions to the conception of the work, drafting the work, revising it critically for important intellectual content and final approval of the version to be published and agreed to be accountable for all aspects of the work in ensuring that questions related to the accuracy or integrity of any part of the work are appropriately investigated and resolved. MF made substantial contributions to the drafting of the work, revising it critically for important intellectual content and final approval of the version to be published and agreed to be accountable for all aspects of the work in ensuring that questions related to the accuracy or integrity of any part of the work are appropriately investigated and resolved.
Funding: The authors have not declared a specific grant for this research from any funding agency in the public, commercial or not-for-profit sectors.
Competing interests: None declared.
Provenance and peer review: Not commissioned; externally peer reviewed.
- Download PDF
- Share X Facebook Email LinkedIn
- Permissions
Tay-Sachs Disease
Author Affiliations : University Hospitals of Cleveland, Case Western Reserve University School of Medicine, Cleveland, Ohio.
CHRISTOPHER G.GOETZMD
Tay-Sachs disease is an autosomal recessive disease caused by a deficiency of β-hexosaminidase A, the lysosomal enzyme that normally degrades GM2 ganglioside. As a result, GM2 ganglioside accumulates in the lysosomes of nerve cells. The disease is one of a family of lysosomal storage disorders known as GM2 gangliosidoses, each determined by the specific peptide (α and β subunits of β-hexosaminidase A and the GM2 activator protein) that is defective in the degradation of GM2 ganglioside. 1 While Tay-Sachs disease commonly refers to the classic infantile form of this GM2 gangliosidosis (also called type 1 GM2 gangliosidosis), wherein β-hexosaminidase A is virtually absent, juvenile and late-onset forms also occur when there is residual enzymatic activity. The highest carrier rate has been among Ashkenazic Jews, although the incidence has decreased among this population because of widespread carrier screening, while clusters remain among certain French Canadian and Cajun populations. This article in the Seminal Citations series focuses on early descriptions of the disease and key developments in biochemistry, genetics, testing, and treatment.
Read More About
Fernandes Filho JA , Shapiro BE. Tay-Sachs Disease. Arch Neurol. 2004;61(9):1466–1468. doi:10.1001/archneur.61.9.1466
Manage citations:
© 2024
Artificial Intelligence Resource Center
Neurology in JAMA : Read the Latest
Browse and subscribe to JAMA Network podcasts!
Others Also Liked
Select your interests.
Customize your JAMA Network experience by selecting one or more topics from the list below.
- Academic Medicine
- Acid Base, Electrolytes, Fluids
- Allergy and Clinical Immunology
- American Indian or Alaska Natives
- Anesthesiology
- Anticoagulation
- Art and Images in Psychiatry
- Artificial Intelligence
- Assisted Reproduction
- Bleeding and Transfusion
- Caring for the Critically Ill Patient
- Challenges in Clinical Electrocardiography
- Climate and Health
- Climate Change
- Clinical Challenge
- Clinical Decision Support
- Clinical Implications of Basic Neuroscience
- Clinical Pharmacy and Pharmacology
- Complementary and Alternative Medicine
- Consensus Statements
- Coronavirus (COVID-19)
- Critical Care Medicine
- Cultural Competency
- Dental Medicine
- Dermatology
- Diabetes and Endocrinology
- Diagnostic Test Interpretation
- Drug Development
- Electronic Health Records
- Emergency Medicine
- End of Life, Hospice, Palliative Care
- Environmental Health
- Equity, Diversity, and Inclusion
- Facial Plastic Surgery
- Gastroenterology and Hepatology
- Genetics and Genomics
- Genomics and Precision Health
- Global Health
- Guide to Statistics and Methods
- Hair Disorders
- Health Care Delivery Models
- Health Care Economics, Insurance, Payment
- Health Care Quality
- Health Care Reform
- Health Care Safety
- Health Care Workforce
- Health Disparities
- Health Inequities
- Health Policy
- Health Systems Science
- History of Medicine
- Hypertension
- Images in Neurology
- Implementation Science
- Infectious Diseases
- Innovations in Health Care Delivery
- JAMA Infographic
- Law and Medicine
- Leading Change
- Less is More
- LGBTQIA Medicine
- Lifestyle Behaviors
- Medical Coding
- Medical Devices and Equipment
- Medical Education
- Medical Education and Training
- Medical Journals and Publishing
- Mobile Health and Telemedicine
- Narrative Medicine
- Neuroscience and Psychiatry
- Notable Notes
- Nutrition, Obesity, Exercise
- Obstetrics and Gynecology
- Occupational Health
- Ophthalmology
- Orthopedics
- Otolaryngology
- Pain Medicine
- Palliative Care
- Pathology and Laboratory Medicine
- Patient Care
- Patient Information
- Performance Improvement
- Performance Measures
- Perioperative Care and Consultation
- Pharmacoeconomics
- Pharmacoepidemiology
- Pharmacogenetics
- Pharmacy and Clinical Pharmacology
- Physical Medicine and Rehabilitation
- Physical Therapy
- Physician Leadership
- Population Health
- Primary Care
- Professional Well-being
- Professionalism
- Psychiatry and Behavioral Health
- Public Health
- Pulmonary Medicine
- Regulatory Agencies
- Reproductive Health
- Research, Methods, Statistics
- Resuscitation
- Rheumatology
- Risk Management
- Scientific Discovery and the Future of Medicine
- Shared Decision Making and Communication
- Sleep Medicine
- Sports Medicine
- Stem Cell Transplantation
- Substance Use and Addiction Medicine
- Surgical Innovation
- Surgical Pearls
- Teachable Moment
- Technology and Finance
- The Art of JAMA
- The Arts and Medicine
- The Rational Clinical Examination
- Tobacco and e-Cigarettes
- Translational Medicine
- Trauma and Injury
- Treatment Adherence
- Ultrasonography
- Users' Guide to the Medical Literature
- Vaccination
- Venous Thromboembolism
- Veterans Health
- Women's Health
- Workflow and Process
- Wound Care, Infection, Healing
- Register for email alerts with links to free full-text articles
- Access PDFs of free articles
- Manage your interests
- Save searches and receive search alerts
Tay-Sachs Disease
- Living reference work entry
- First Online: 17 October 2016
- Cite this living reference work entry
- Harold Chen 2 , 3
764 Accesses
8 Altmetric
Tay-Sachs disease is a hereditary neurodegenerative disorder resulting from excess storage of GM2 ganglioside within the lysosomes of cells, caused by deficiency of hexosaminidase A. The incidence of the disease is estimated to be 1 in 3,600 in Ashkenazi Jews (AJ) with carrier frequency of 1 in 30 and 1 in 360,000 in other population with carrier frequency of 1 in 300. Tay-Sachs disease is the most frequently occurring sphingolipidoses.
This is a preview of subscription content, log in via an institution to check access.
Access this chapter
Institutional subscriptions
Similar content being viewed by others
Rare Diseases in Glycosphingolipid Metabolism
Disorders of Sphingolipid Synthesis, Sphingolipidoses, Niemann-Pick Disease Type C and Neuronal Ceroid Lipofuscinoses
Adachi, M., Schneck, L., & Volk, B. W. (1974). Ultrastructural studies of eight cases of fetal Tay-Sachs disease. Laboratory Investigation, 30 , 102–112.
CAS PubMed Google Scholar
Akli, S., Boue, J., Sandhoff, K., et al. (1993). Collaborative study of the molecular epidemiology of Tay-Sachs disease in Europe. European Journal of Human Genetics, 1 , 229–238.
Argov, Z., & Navon, R. (1984). Clinical and genetic variations in the syndrome of adult GM2 gangliosidosis resulting from hexosaminidase A deficiency. Annals of Neurology, 16 , 14–20.
Article CAS PubMed Google Scholar
Aydin, K., Bakir, B., Tatli, B., et al. (2005). Proton MR spectroscopy in three children with Tay-Sachs disease. Pediatric Radiology, 35 , 1081–1085.
Article PubMed Google Scholar
Bach, G., Tomczak, J., Risch, N., et al. (2001). Tay-Sachs screening in the Jewish Ashkenazi population: DNA testing is the preferred procedure. American Journal of Medical Genetics, 99 (1), 70–75.
Brady, R. O. (2001). Tay-Sachs disease: The search for the enzymatic defect. Advances in Genetics, 44 , 51–60.
Brett, E. M., Ellis, R. B., Haas, L., et al. (1973). Late onset GM2-gangliosidosis. Clinical, pathological, and biochemical studies on 8 patients. Archives of Disease in Childhood, 48 , 775–785.
Article CAS PubMed PubMed Central Google Scholar
Callahan, J. W., Archibald, A., Skomorowski, M. A., et al. (1990). First trimester prenatal diagnosis of Tay-Sachs disease using the sulfated synthetic substrate for hexosaminidase A. Clinical Biochemistry, 23 , 533–536.
Chamoles, N. A., Blanco, M., Gaggioli, D., et al. (2002). Tay-Sachs and Sandhoff diseases: Enzymatic diagnosis in dried blood spots on filter paper: Retrospective diagnoses in newborn-screening cards. Clinica Chimica Acta, 318 , 133–137.
Article CAS Google Scholar
Chen, H., Chan, A. Y., Stone, D. U., et al. (2014). Beyond the cherry-red spot: Ocular manifestations of sphingolipid-mediated neurodegenerative and inflammatory disorders. Survey of Ophthalmology, 59 , 64–76.
Cutz, E., Lowden, J. A., & Conen, P. E. (1974). Ultrastructural demonstration of neuronal storage in fetal Tay-Sachs disease. Journal of Neurological Sciences, 21 , 197–202.
Desnick, R. J., & Goldberg, J. D. (1977). Tay-Sachs disease: Prospects for therapeutic intervention. Progress in Clinical and Biological Research, 18 , 129–141.
Georgiou, T., Christopoulos, G., Anastasiadou, V., et al. (2014). The first family with Tay-Sachs disease in Cyprus: Genetic analysis reveals a nonsense (c.78GNA) and a silent (c.1305CNT) mutation and allows preimplantation genetic diagnosis. Meta Gene, 2 , 200–205.
Article PubMed PubMed Central Google Scholar
Grabowski, G. A., Kruse, J. R., Goldberg, J. D., et al. (1984). First-trimester prenatal diagnosis of Tay-Sachs disease. American Journal of Human Genetics, 36 , 1369–1378.
CAS PubMed PubMed Central Google Scholar
Gravel, R. A., Triggs-Raine, B. L., & Mahuran, D. J. (1991). Biochemistry and genetics of Tay-Sachs disease. Canadian Journal of Neurological Sciences, 18 , 419–423.
Grebner, E. E., & Jackson, L. G. (1985). Prenatal diagnosis for Tay-Sachs disease using chorionic villus sampling. Prenatal Diagnosis, 5 , 313–320.
Güngör, O., Güngör, G., Yurttutan, N., et al. (2016). Thalamic T2 hypointensity: A diagnostic clue for Tay-Sachs disease. Acta Neurologica Belgica, 116 , 195–197.
Hansis, C., & Grifo, J. (2001). Tay-Sachs disease and preimplantation genetic diagnosis. Advances in Genetics, 44 , 311–315.
Hoffman, J. D., Greger, V., Strovel, E. T., et al. (2013). Next-generation DNA sequencing of HEXA: A step in the right direction for carrier screening. Molecular Genetics & Genomic Medicine, 1 , 260–268.
Kaback, M. M., & Desnick, R. J. (2011). Hexosaminidase a deficiency. GeneReviews . Retrieved August 11, 2011. Available at: http://www.ncbi.nlm.nih.gov/books/NBK1218/
Kaback, M. M., Nathan, T. J., & Greenwald, S. (1977). Tay-Sachs disease: Heterozygote screening and prenatal diagnosis-US experience and world perspective. Progress in Clinical and Biological Research, 18 , 13–36.
Kaback, M., Lim-Steele, J., Dabholkar, D., et al. (1993). Tay-Sachs disease-carrier screening, prenatal diagnosis, and the molecular era. An international perspective, 1970 to 1993. The International TSD Data Collection Network. Journal of the American Medical Association, 270 , 2307–2315.
Kivlin, J. D., Sanborn, G. E., & Myers, G. G. (1985). The cherry-red spot in Tay-Sachs and other storage diseases. Annals of Neurology, 17 , 356–360.
Lew, R. M., Burnett, L., Proos, A. L., et al. (2015a). Ashkenazi Jewish population screening for Tay-Sachs disease: The International and Australian experience. Journal of Paediatrics and Child Health, 51 , 271–279.
Lew, R. M., Burnett, L., Proos, A. L., et al. (2015b). Tay-Sachs disease: Current perspectives from Australia. The Application of Clinical Genetics, 8 , 19–25.
MacQueen, G. M., Rosebush, P. I., & Mazurek, M. F. (1998). Neuropsychiatric aspects of the adult variant of Tay-Sachs disease. The Journal of Neuropsychiatry and Clinical Neurosciences, 10 , 10–19.
Maegawa, G. H., Stockley, T., Tropak, M., et al. (2006). Natural history of juvenile or subacute GM2 gangliosidosis: 21 new cases and literature review of 134 previously reported. Pediatrics, 118 , e1550–e1562.
Mahuran, D. J., Triggs-Raine, B. L., Feigenbaum, A. J., et al. (1990). The molecular basis of Tay-Sachs disease: Mutation identification and diagnosis. Clinical Biochemistry, 23 , 409–415.
O’Brien, J. S., Okada, S., Fillerup, D. L., et al. (1971). Tay-Sachs disease: Prenatal diagnosis. Science, 172 , 61–64.
Schneck, L., Adachi, M., & Volk, B. W. (1972). The fetal aspects of Tay-Sachs disease. Pediatrics, 49 , 342–351.
Schweitzer-Miller, L. (2001). Tay-Sachs disease: Psychologic care of carriers and affected families. Advances in Genetics, 44 , 341–347.
Shapiro, B. E., Logigian, E. L., Kolodny, E. H., et al. (2008). Late-onset Tay-Sachs disease: The spectrum of peripheral neuropathy in 30 affected patients. Muscle & Nerve, 38 , 1012–1015.
Article Google Scholar
Sheth, J., Datar, C., Mistri, M., et al. (2016). GM2 gangliosidosis AB variant: Novel mutation from India – A case report with a review. BMC Pediatrics, 16 , 1–5.
Steiner, K. M., Brenck, J., Goericke, S., et al. (2016). Cerebellar atrophy and muscle weakness: Late-onset Tay-Sachs disease outside Jewish populations. BMJ Case Reports. 2016. March 31 [Epub ahead of print].
Google Scholar
Streifler, J. Y., Gornish, M., Hadar, H., et al. (1993). Brain imaging in late-onset GM2 gangliosidosis. Neurology, 43 , 2055–2058.
Sutton, V. R. (2002). Tay-Sachs disease screening and counseling families at risk for metabolic disease. Obstetrics and Gynecology Clinics of North America, 29 , 287–296.
Suzuki, K. (1994). Saul R. Korey Lecture. Molecular genetics of Tay-Sachs and related disorders: A personal account. Journal of Neuropathology and Experimental Neurology, 53 , 344–350.
Thurmon, T. F. (1993). Tay-Sachs genes in Acadians. American Journal of Human Genetics, 53 , 781–783.
Download references
Author information
Authors and affiliations.
Medical Genetics, Shriners Hospitals for Children, Shreveport, LA, USA
Harold Chen
Perinatal and Clinical Genetics, Department of Pediatrics, LSU Health Sciences Center, 1501 Kings Highway, Shreveport, LA, 71130, USA
You can also search for this author in PubMed Google Scholar
Corresponding author
Correspondence to Harold Chen .
Rights and permissions
Reprints and permissions
Copyright information
© 2016 Springer Science+Business Media LLC
About this entry
Cite this entry.
Chen, H. (2016). Tay-Sachs Disease. In: Atlas of Genetic Diagnosis and Counseling. Springer, New York, NY. https://doi.org/10.1007/978-1-4614-6430-3_225-2
Download citation
DOI : https://doi.org/10.1007/978-1-4614-6430-3_225-2
Received : 31 August 2016
Accepted : 31 August 2016
Published : 17 October 2016
Publisher Name : Springer, New York, NY
Online ISBN : 978-1-4614-6430-3
eBook Packages : Springer Reference Biomedicine and Life Sciences Reference Module Biomedical and Life Sciences
- Publish with us
Policies and ethics
- Find a journal
- Track your research
- Open access
- Published: 17 September 2018
Neural stem cells for disease modeling and evaluation of therapeutics for Tay-Sachs disease
- Mylinh Vu 1 ,
- Rong Li 1 ,
- Amanda Baskfield 1 ,
- Billy Lu 1 ,
- Atena Farkhondeh 1 ,
- Kirill Gorshkov 1 ,
- Omid Motabar 1 ,
- Jeanette Beers 2 ,
- Guokai Chen 2 , 3 ,
- Jizhong Zou 2 ,
- Angela J. Espejo-Mojica 4 ,
- Alexander Rodríguez-López 4 , 5 ,
- Carlos J. Alméciga-Díaz 4 ,
- Luis A. Barrera 4 ,
- Xuntian Jiang 6 ,
- Daniel S. Ory 6 ,
- Juan J. Marugan 1 &
- Wei Zheng ORCID: orcid.org/0000-0003-1034-0757 1
Orphanet Journal of Rare Diseases volume 13 , Article number: 152 ( 2018 ) Cite this article
10k Accesses
31 Citations
13 Altmetric
Metrics details
Tay-Sachs disease (TSD) is a rare neurodegenerative disorder caused by autosomal recessive mutations in the HEXA gene on chromosome 15 that encodes β-hexosaminidase. Deficiency in HEXA results in accumulation of GM2 ganglioside, a glycosphingolipid, in lysosomes. Currently, there is no effective treatment for TSD.
We generated induced pluripotent stem cells (iPSCs) from two TSD patient dermal fibroblast lines and further differentiated them into neural stem cells (NSCs). The TSD neural stem cells exhibited a disease phenotype of lysosomal lipid accumulation. The Tay-Sachs disease NSCs were then used to evaluate the therapeutic effects of enzyme replacement therapy (ERT) with recombinant human Hex A protein and two small molecular compounds: hydroxypropyl-β-cyclodextrin (HPβCD) and δ-tocopherol. Using this disease model, we observed reduction of lipid accumulation by employing enzyme replacement therapy as well as by the use of HPβCD and δ-tocopherol.
Our results demonstrate that the Tay-Sachs disease NSCs possess the characteristic phenotype to serve as a cell-based disease model for study of the disease pathogenesis and evaluation of drug efficacy. The enzyme replacement therapy with recombinant Hex A protein and two small molecules (cyclodextrin and tocopherol) significantly ameliorated lipid accumulation in the Tay-Sachs disease cell model.
Tay-Sachs disease (TSD) is one of three lysosomal storage diseases classified as GM2 gangliosidoses, along with Sandhoff disease and the AB variant. TSD and Sandhoff disease are caused by mutations in the HEXA and HEXB genes, respectively. The AB variant is caused by mutations in the GM2A gene encoding for the GM2 activator for β-hexosaminidase A [ 1 ]. Both TSD and Sandhoff disease are rare neurodegenerative disorders due to a deficiency in the enzyme β-hexosaminidase, which hydrolyzes GM2 ganglioside into GM3 ganglioside. β-Hexosaminidase is a heterodimer that exists in three isoforms: hexosaminidase A (Hex A), hexosaminidase B (Hex B), and hexosaminidase S (Hex S). Hex A is an α/β heterodimer while Hex B and Hex S consist of two β-subunits and two α-subunits, respectively. In TSD patients, mutations in the HEXA gene result in misfolded α-subunits that render Hex A and Hex S non-functional [ 2 ]. Deficiency of Hex A activity in TSD causes accumulation of GM2 ganglioside in lysosomes, which ultimately results in progressive neurodegeneration.
There are three forms of TSD: acute infantile, juvenile, and adult. The variations of TSD are characterized by the age of onset and level of remaining Hex A activity in patient cells [ 3 ]. Acute infantile TSD is the most common and harmful variant which shows progressive decline in muscle strength and loss of motor skills around six months to three years of age. As the disease progresses, the infant’s brain deteriorates which leads to seizures, blindness, loss of cognitive functions, and ultimately death [ 4 ].
Currently, there are no effective treatments for Tay-Sachs disease. The main treatment approach involves managing the symptoms of the disease [ 4 ]. Enzyme replacement therapy (ERT) is available for treatment of several lysosomal storage diseases such as Gaucher, Fabry, and Pompe disease [ 5 ]. Treatment with recombinant human β-hexosaminidase in both human TSD fibroblasts and mouse TSD models decreased lysosomal GM2 accumulation [ 6 , 7 ]. However, an earlier study failed to show the beneficial effect of ERT in Tay-Sachs disease patients [ 8 ]. Cyclodextrin (HPβCD) and δ-tocopherol have been reported to reduce lipid accumulation and decrease the enlarged lysosomes through increasing lysosomal exocytosis [ 9 ]. We have observed the therapeutic effect of HPβCD and δ-tocopherol in the induced pluripotent stem cell (iPSC)-derived neural stem cells (NSCs) in NPC1, NPA, Wolman, and Batten (CLN1 and CLN2) diseases [ 9 , 10 , 11 , 12 , 13 ].
Recent advances in stem cell technology have enabled the generation of disease-specific iPSCs from patient somatic cells. These iPSCs can be differentiated into various types of progenitor cells and mature cells such as neurons, cardiomyocytes, hepatocytes, or retinal pigment epithelial cells for modeling diseases in cell-based assays [ 14 , 15 ]. Due to the availability of large numbers of NSCs derived from patient iPSCs and their disease phenotypes, they have been used as a cell-based model system for evaluating drug efficacy and drug development [ 10 , 11 , 13 ]. In this study, we report the generation of iPSC lines from two TSD patient dermal fibroblast cells. These TSD iPSC lines were further differentiated into NSCs that exhibited a disease phenotype of lipid accumulation and enlarged lysosomes. The treatment of these patient cells with recombinant human Hex A protein dramatically reduced the lipid accumulation in the TSD cells. δ-Tocopherol and hydroxypropyl-beta-cyclodextrin (HPβCD) also ameliorated the lysosomal lipid accumulation and decreased the enlargement of lysosomes in the TSD NSCs. The results demonstrate that the TSD NSCs differentiated from patient iPSCs are a useful disease model for further study of disease pathophysiology and for use as a cell-based model in drug development.
Three human dermal fibroblast cell lines were purchased from the Coriell Cell Repositories (Camden, NJ): a wild type female (catalog no. GM05659), a female TSD patient (GM00515), and a male TSD patient (GM00221). See Table 1 for details. DMEM medium (11965092), TrypLE Express (12605010), penicillin-streptomycin (15140122), sodium pyruvate (11360070), Essential 8 medium (A1517001), PSC Neural Induction Medium kit (A1647801), StemPro NSC SFM kit (A1050901), Human neural stem cell immunocytochemistry kit (A24354), CytoTune-iPS 2.0 Sendai Reprogramming Kit (A16517), GlutaMax (35050), Hoechst 33342 (H3570), and Nile Red (N1142) were obtained from Thermo Fisher Scientific. Matrigel hESC-Qualified Matrix (354277) was obtained from Corning. Rock Inhibitor Y-27632 (1254) was obtained from Tocris Bioscience (Ellisville, MO). Hyclone Fetal Bovine Serum (SH30071.03) was purchased from GE Healthcare. δ-Tocopherol obtained from Sigma Aldrich was further purified by HPLC to gain purity greater than 99% while hydroxypropyl-beta-cyclodextrin (HPβCD, E0163) was obtained from Roquette America (IL, USA). Black, clear bottom, tissue-culture treated 96-well plates (655090) were purchased from Greiner Bio-One (Monroe, NC).
Generation and characterization of induced pluripotent stem cells
Fibroblasts were cultured in DMEM medium with 15% fetal bovine serum (FBS), 1X penicillin-streptomycin, and 1X sodium pyruvate. Induced pluripotent stem cell (iPSC) lines were generated from three fibroblast lines using the non-integrating CytoTune-iPS 2.0 Sendai Reprogramming Kit (Table 1 ). Two iPSC clones were generated from each Tay-Sachs disease cell line. The iPSCs were cultured on Matrigel pre-coated tissue culture plates using Essential 8 medium and passaged using an EDTA-based protocol as previously described [ 16 ]. All iPSC lines were cultured beyond passage 10, to ensure the clearance of Sendai viruses and the stability of the iPSC cell lines.
Short tandem repeat (STR) DNA analysis was performed by Johns Hopkins University’s Genetic Resources Core Facility on each set of TSD patient fibroblast cells and NSC lines to confirm their identity as derivatives of patient fibroblast lines GM00515 and GM00221.
G-banded karyotyping analysis of iPSCs was conducted using standard cytogenetic protocol by WiCell Research Institute (Madison, WI). The harvested iPSCs were incubated with ethidium bromide and colcemid in a hypotonic solution before being fixed. Twenty randomly chosen cells in metaphase from each iPSC clone were stained with Leishman’s stain and analyzed by G-banding. The immunofluorescence staining and flow cytometry were also used to characterize iPSCs generated. Immunocytochemistry assay was performed on fixed iPSCs using the pluripotent cell markers of Sox2, Oct4, Nanog, Tra-1-60, and SSEA4. Cells were imaged using an INCell 2200 imaging system (GE Healthcare) using 20X objective lens and Cy5, FITC, and DAPI filter sets. For the FACS analysis, iPSCs were also harvested and fixed using 4% paraformaldehyde and washed, followed by a 10 min incubation with 0.2% Tween-20 to permeabilize cell membrane. The cells were then stained with anti-Tra-1-60-FITC and anti-Nanog-AlexaFluor 488. FACS was performed on a BD Accuri C6 Flow Cytometry System.
Neural stem cell differentiation and characterization
Tay-Sachs disease iPSCs were differentiated into neural stem cells (NSCs) using the PSC neural Induction Medium kit (Thermo Fisher Scientific) according to the manufacture’s protocol. Once iPSCs reached confluence of 70 to 80%, cells were dislodged from plates using 0.5 mM EDTA buffer and seeded onto Matrigel coated 6-well plates at 3 × 10 5 cells/well in E8 medium with 10 μM of Rock Inhibitor Y-27632. Cells were incubated overnight at 37 °C to attach onto the plate. The culture media was then changed to PSC Neural Induction Medium containing 1X Neural Induction Supplement in Neurobasal Medium (Thermo Fisher Scientific). Media was changed daily for 7 days. On the 7th day of neural induction, the NSCs were dissociated from the plate using Accutase enzyme cell detachment medium (Thermo Fisher Scientific) and seeded into Matrigel pre-coated T75 flasks for further expansion in the Neural Expansion Medium containing 1X Neural Induction Supplement in equal volume of Neurobasal Medium and Advanced DMEM/F-12 (Thermo Fisher Scientific).
A Human Neural Stem Cell ICC kit (Thermo Fisher Scientific) was used to characterize NSCs generated from TSD patients and WT control iPSCs. The anti-PAX6 antibody was replaced with Oct4 to verify that the NSCs were no longer iPSCs. The manufacture’s protocol was followed for the immunofluorescence staining. Cells were then imaged in the INCell 2200 imaging system (GE Healthcare) using a 20X objective lens with Cy5, FITC, and DAPI filter sets. Additionally, Tay-Sachs disease NSCs were sent to the Johns Hopkins University Genetic Resources Core Facility for STR DNA profiling with the FAF’s PowerPlex 16D (Promega) Standard Service Plus Profile Search.
Neuron differentiation and characterization
Tay-Sachs patient NSCs were further differentiated into neurons using STEMdiff™ Neuron Differentiation Kit and STEMdiff™ Neuron Maturation Kit (StemCell Technologies) according to the manufacture’s protocol. After 7 days of differentiation of Tay-Sachs NSCs to neuronal precursors in 6- well plate using STEMdiff™ Neuron Differentiation medium, cells were dislodged and seeded into Poly-L-ornithine (PLO)/laminin coated black, clear bottom, tissue-culture treated 96-well plate in STEMdiff™ Neuron Maturation medium for another 7–14 days before downstream application. Immunofluorescence staining of several neuronal markers MAP2 (Cell signaling), beta-III-tubulin (Cell signaling), Neurofilament-L (Cell signaling) as well as Nestin (neural stem cell protein marker, BD Bioscience) were performed to characterize the neuronal cells derived from TSD patient and WT control NSCs.
Nile red assay
Nile Red dye stains the accumulated lipids and lipid droplets in cells. It has been reported that the yellow-gold fluorescence detects cytoplasmic lipid droplets better than red fluorescence [ 11 , 12 , 17 ]. In this experiment, the Nile Red dye staining was used to evaluate the accumulation of lipids in TSD patient NSCs, as well as neuronal cells. Briefly, cells were seeded onto 96-well plates using the NSC maintenance media from the StemPro NSC SFM kit with 5 μM Rock Inhibitor. Plates with TSD NSCs were incubated overnight at 37 °C and Rock Inhibitor was removed the following day. The assay media was replaced and 10% FBS (Hyclone) was added to the medium followed by overnight incubation at 37 °C to load the lipids into the NSC cells. Assay media was then replaced to remove the FBS, and plates were incubated again overnight at 37 °C for the Nile Red assay to be performed the following day. Nile Red powder was reconstituted in DMSO to 1 mM and stored in the dark at − 20 °C until used. In the Nile Red staining assay, the stock solution was diluted to 1 μM in warmed assay media and incubated with cells for 10 mins in the dark at 37 °C. The cells were then washed twice using Dulbecco’s phosphate-buffered saline (DPBS) and fixed in 4% paraformaldehyde for 30 min at room temperature. Hoechst was also added to the fixing step using a 1:5000 dilution. After washing twice with DPBS, 200 μl/well DPBS was added before analyzing in the INCell 2200 imaging system using a 20X objective lens with TR/TR, FITC/YFP, and DAPI/DAPI filter sets.
LysoTracker Red dye staining
TSD NSCs were seeded and treated with FBS in the same way as described above. For the LysoTracker dye staining, the NSCs were treated with 50 nM LysoTracker Red DND-99 dye (L-7528, Thermo Fisher Scietific) in assay media at 37 °C for 1 h followed by plate washing twice with DPBS. The plates were fixed and stained with Hoechst simultaneously in 4% paraformaldehyde solution with Hoechst dye at a 1:5000 dilution for 30 min at room temperature. Plates were washed twice using DPBS and stored with 100 μl/well DPBS at 4 °C until imaging. Images were acquired using the INCell 2200 imaging system using a 20X objective lens with Texas Red and DAPI filter sets.
Filipin staining
Filipin dye was used to stain unesterified cholesterol in cells [ 18 ]. Cells were seeded at 2000 cells/well in 100 μl of media in black, clear bottom, tissue culture-treated 96-well plates and cultured for 24 h. The test compounds were dissolved in media and then added at 100 μl /well; the cells were returned to incubation for 4 days. The cells were washed twice with DPBS and fixed with 100 μl/well of 4% paraformaldehyde (PFA) solution at room temperature for 30 min. After washing twice with DPBS, the cells were stained with 50 ng/mL Filipin solution (freshly dissolved in DMSO at 10 mg/mL and then diluted in DPBS) at room temperature for 1 h. The plates were stored in 100 μl/well DPBS at 4 °C after washing two times with DPBS before imaging analysis. On the day of the imaging, cell nuclei were stained with 100 μl/well of 4 μM ethidium homodimer (EthD-1) (Thermo Fisher Scientific) in DPBS at room temperature for 30 min. The plates were imaged using the INCell 2200 imaging system with a 20X or 40X objective lens. A DAPI filter set (excitation = 350 ± 50 nm, and emission = 455 ± 50 nm) and Cy3 filter set (excitation = 543 ± 22 nm, and emission = 604 ± 64 nm) were used to visualize Filipin and EthD-1 staining, respectively.
GM2 ganglioside immunofluorescence staining
For immunofluorescence staining of GM2, same FBS treatment protocol was used as for the Nile Red staining experiment prior to the staining. Briefly, 10% FBS was added to the assay medium followed by overnight incubation to load the lipids into the cells. Assay media was then replaced to remove the FBS, and plates were incubated again overnight at 37 °C for the GM2 immunofluorescence staining to be performed the following day. Cells were fixed in 4% paraformaldehyde for 30 min, rinsed with PBS, and permeabilized with 0.3% Triton X-100 for 15 min at 4 °C. Cells were incubated with normal goat serum 10% blocking buffer (Life Technologies) and incubated with mouse anti-GM2 antibody (Amsbio, clone MK1–16) overnight at 4 °C. After washing with PBS, a corresponding secondary antibody conjugated with Alexa Fluor 594 was added. Cells were then stained with Hoechst 33342 for 20 min and imaged using an INCell Analyzer 2200 imaging system (GE Healthcare) with 20X objective lens and Texas Red and DAPI filter sets.
LC-MS/MS analysis of GM2 in TSD NSCs
We further utilized the LC-tandem mass spectrometry (LC-MS/MS) analysis to determine the GM2 content in TSD patient cells following the protocol established previously [ 19 ]. Briefly, TSD patients and WT control neural stem cell pellets were collected and suspended in 50 μL of water. The ganglioside GM2 was extracted from cell suspension with protein precipitation with methanol in the presence of internal standard (d3-GM2 (18:0)). The ganglioside GM2 was separated by column-switching high-performance liquid chromatography (HPLC) and monitored by multiple-reaction monitoring (MRM) detection on an Applied Biosystems Sciex 6500QTRAP+ tandem mass spectrometer (MS/MS) equipped with an electrospray ion source. A quality control (QC) sample was prepared from pooled study samples and injected every 3 study samples to monitor the LC-MS/MS assay performance. All the ganglioside GM2 species in QC injections demonstrate CV < 15%. The protein precipitate from extraction was dissolved in a mixture of 2% CHAPS (100 μL) and 1% SDS (750 μL), and the protein was measured with BCA assay. The relative quantification data were provided as area ratios of GM2 species to internal standard, which were normalized to the protein.

Recombinant β-hexosaminidases production in Pichia pastoris GS115
Recombinant Hex A was produced in the methylotrophic yeast Pichia pastoris GS115 as previously described [ 20 ]. Briefly, cDNA of α- (GenBank AAH84537) and β- (GenBank AAH17378) subunits of human β-hexosaminidases were codon-optimized for P. pastoris and inserted into a pPICK9k vector. Constructs pPIC9k-alpha or pPIC9k-beta were co-transformed into P. pastoris GS115. Clones were evaluated at a shake flask scale, and that with highest activity was used for production of recombinant human Hex A (rhHexA). Cultures were prepared in a modified FM22 saline media and protein production conditions were performed as previously described [ 20 , 21 ].
Recombinant protein was purified from culture media thanks to the presence of the α-factor secretion signal, and purified by ion exchange chromatography as previously described [ 20 ]. β-Hexosaminidase activity was assayed by using 4-methylumbelliferyl-β-D-acetyl-glucosaminide (MUG, Sigma-Aldrich) or 4-methylumbelliferyl-β-D-acetyl-glucosaminide sulfate (MUGS, Calbiochem) substrates [ 20 , 22 ]. One unit (U) was defined as the amount of enzyme hydrolyzing 1 nmol of substrate per hour. Specific hexosaminidase activity was expressed as U/mg of total protein determined by a Lowry assay.
- Enzyme replacement therapy
TSD NSCs were seeded onto 96-well plates pre-coated with Matrigel using the NSC maintenance media formulated from the StemPro NSC SFM kit containing 5 μM Rock Inhibitor. Plates were incubated at 37 °C overnight and the medium was changed the following day to remove Rock Inhibitor; 100 nM of Hex A recombinant enzyme was incubated with TSD NSCs for 4 h at 37 °C in NSC maintenance media. The media was changed to remove excess recombinant enzymes not taken up by the cells and other components in the buffer of enzyme stock that are not suitable for neural stem cell culture. Because the neural stem cell culture medium does not containing serum and regular lipid components, 10% FBS was added to the medium to facilitate lysosomal storage of lipids. Therefore, we added the enzyme first and wash the cells 4 h later to replace with the medium containing 10% FBS for cell culture overnight. NSCs were cultured in NSC maintenance medium with or without 10% FBS for 24 h at 37 °C. The Nile Red dye staining was performed on the following day.
Treatments with HPβCD and δ-tocopherol
On Day 0, TSD NSCs and wild type NSCs were seeded into 96-well plates pre-coated with Matrigel in 100 μl/well of NSC maintenance media from the StemPro NSC SFM kit along with 5 μM Rock Inhibitor. The plates were incubated overnight at 37 °C. The media was changed the next day (Day 1) to remove Rock Inhibitor. The cells were treated with HPβCD and δ-tocopherol at 37 °C for 24 h. On Day 2, the medium was replaced with NSC maintenance medium containing 10% FBS and HPβCD or δ-tocopherol followed by incubation at 37 °C for another 24 h. HPβCD was dissolved in water while δ-tocopherol was dissolved in DMSO to a 100 mM stock. The Nile Red dye staining was performed on Day 3 of the experiment.
ATP content assay for cell viability
An ATP content assay kit (ATPLite, PerkinElmer) was used to measure cell viability to monitor compound cytotoxicity. Cells were seeded at 2500 cells/well in 100 μl medium in white, solid 96-well plates and incubated for 24 h. Cells were cultured and treated as described above. After 3 days of incubation, 100 μl/well of ATP content reagent mixture (prepared according to the manufacturer’s instruction) was added to the assay plates followed by incubation at room temperature for 2–5 min. The luminescence signal was determined in the luminescence mode of the ViewLux Plate reader (PerkinElmer).
Data analysis and statistics
Image analysis of Nile Red staining, LysoTracker Red staining, and Filipin staining was performed using INCell Analyzer software (GE Healthcare, version 3.7.2). The Multi-Target Analysis protocol was used for quantification of all three assays. Concentration-response curves were analyzed and IC 50 values calculated using the Prism software (GraphPad, Inc., San Diego). Results in the figures were expressed as mean of replicates ± standard error of the mean (SEM). Unless otherwise stated, an unpaired t-test was used to test for significance, with * p < 0.05, ** p < 0.01, and *** p < 0.001.
Generation and characterization of Tay-Sachs iPSCs
Tay-Sachs iPSC lines were generated from two Tay-Sachs patient dermal fibroblasts obtained from Coriell Cell Repositories (GM00221 and GM00515) using the non-integrating Sendai virus reprogramming system that expresses Oct3/4, Sox2, Klf4, and c-Myc factors (Table 1 ). A patient iPSC line HT134A was established from the fibroblast line GM00221, while another patient iPSC line HT151A was generated from GM00515 (Table 1 ). No obvious differences in iPSC morphology (Additional file 1 : Figure S1A) or growth rate were observed in the TSD iPSC lines compared to the wild type (WT) cells. Flow cytometry analysis showed that the iPSCs expressed the pluripotency markers of TRA-1-60 and Nanog (Fig. 1b , Additional file 1 : Figure S1B). Immunofluorescence staining experiments showed positive staining of major pluripotency markers including nuclear markers (SOX2, Oct4, Nanog) and stem cell-specific cell surface markers (TRA-1-60 and SSEA4) (Fig. 1a , Additional file 1 : Figure S1A). Furthermore, G-banding karyotyping analysis confirmed the normal karyotype in the TSD iPSC (Additional file 1 : Figure S2B). The short tandem repeat (STR) profiling analysis confirmed the cell source of each iPSC line as all 11 checked STR loci matched with its parental Tay-Sachs patient fibroblasts (Additional file 1 : Figure S2C). The results demonstrate the successful generation of two TSD iPSC lines.
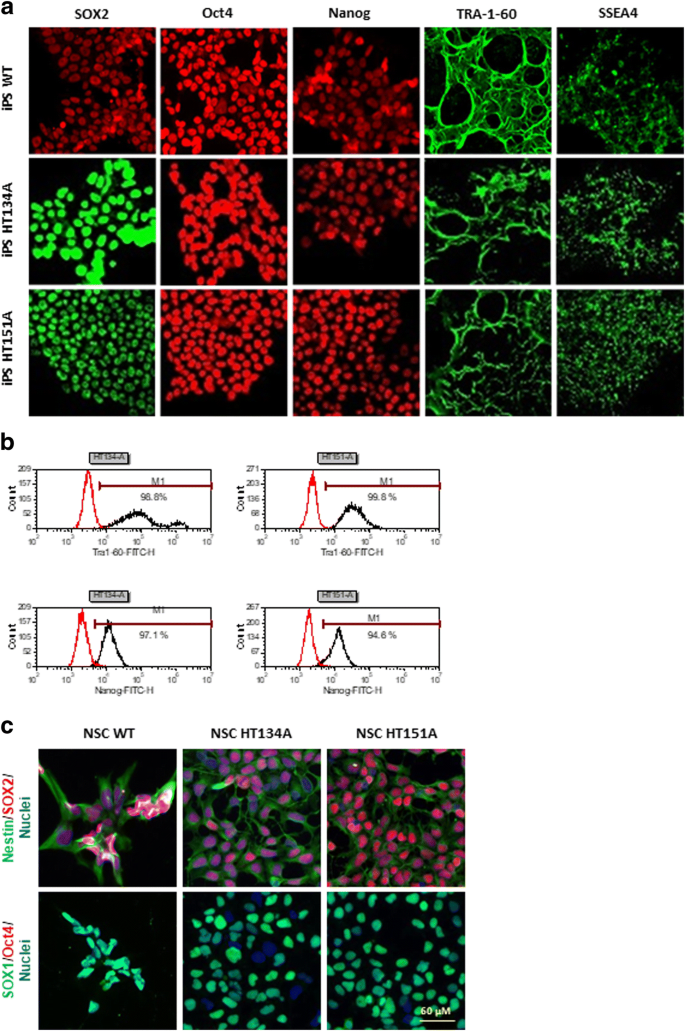
Characterization of Tay-Sachs disease iPSCs and NSCs . a The iPSCs derived from TSD patients and WT control fibroblasts expressed pluripotency protein markers SOX2, Oct4, Nanog, TRA-1-60 and SSEA4. b Flow cytometry analysis of TSD iPSCs, > 94% of iPSCs expressing Nanog and TRA-1-60 markers. c Immunofluorescence staining of TSD and WT NSCs. Nestin, SOX1, and SOX2 are neural stem cell markers while Oct4 is an iPSC marker
Generation and characterization of Tay-Sachs disease neural stem cells
The TSD iPSCs and WT iPSCs were then differentiated into NSCs using the commercial PSC Neural Induction Medium. The NSCs exhibited normal morphology and no significant differences were observed in the differentiation time and cell morphology of patient cells compared to the WT control cells. Immunofluorescence staining of major NSC markers demonstrated that the NSCs differentiated from TSD iPSC lines expressed high level of NSC markers SOX1, SOX2, and Nestin, but not Oct4, a pluripotency marker only expressed in iPSCs (Fig. 1c , Additional file 1 : Figure S1C).
TSD NSCs exhibited lipid accumulation in lysosomes and enlarged lysosomes
To examine the lipid accumulation in lysosomes, a Nile Red dye staining assay was used for visualizing nonpolar lipid in the patient cells [ 9 ]. Both TSD NSC lines (HT134A and HT151A) showed increased Nile Red dye staining, indicating accumulation of lipids in lysosomes after loading with lipids in FBS for 24 h (Fig. 2a , Additional file 1 : Figure S3A and 3B). In comparison to WT NSCs, the Nile Red staining in NSC HT134A and HT151A lines was two-fold. (Fig. 2c ). These results indicated a significant accumulation of nonpolar lipid in both TSD patient iPSC-derived NSCs.
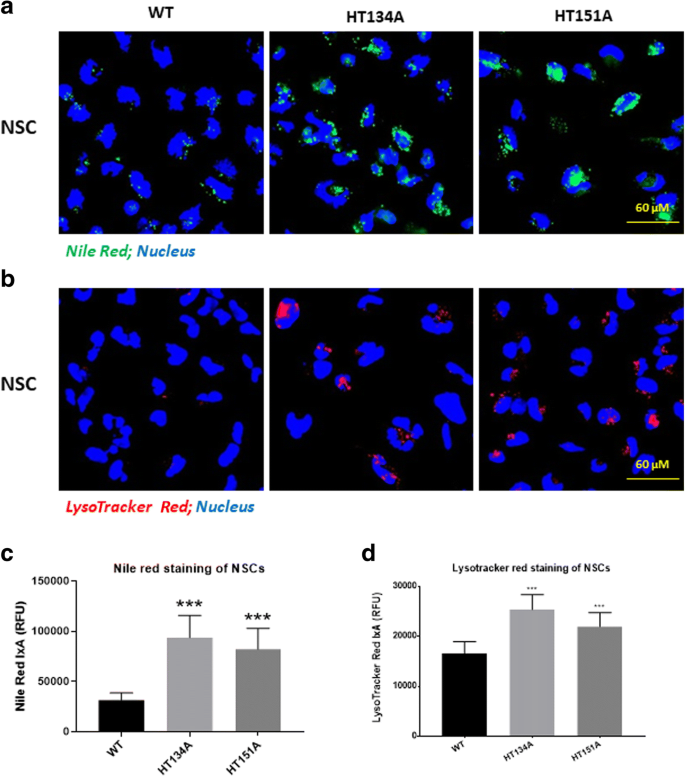
Nile Red and LysoTracker Red staining of TSD NSCs. a Images of increased intensity of Nile Red staining in TSD NSC compared to WT NSCs after 24 h addition of 10% FBS. The yellow/gold fluorescence of Nile Red excites and emits at 450-500 nm and 528 nm, respectively. b LysoTracker Red staining in TSD NSCs. c Intensity of Nile Red staining in TSD NSCs after FBS treatment. d Intensity of LysoTracker Red staining in Tay-Sachs disease NSCs after FBS treatment. Data are displayed as mean ± SD. **** p < 0.0001 compared to WT control. IxA, integrated cell intensity
To determine whether the lysosomes were enlarged in those TSD patient cells due to the accumulation of lipid, LysoTracker Red dye staining was also carried out to visualize enlarged lysosomes. A sight increase of lysotracker staining in TSD patient NSCs compared to the NSCs derived from WT control iPSCs. In contrast, the NSCs derived from Niemann-Pick disease, type C1 (NPC1) iPSCs showed much stronger Lysotracker fluorescence signal (Fig. 2b , Additional file 1 : Figure S3A and 3C), consistent with our previous findings [ 9 , 13 , 23 ].
To evaluate the potential secondary accumulation of other lipids inside the cells, we further carried out the Filipin staining for unesterified cholesterols that accumulates in the lysosomes of many patient cells with lysosomal storage diseases, especially the Niemann Pick disease type C. The Filipin staining was not significantly different in all four TSD patient iPSC-derived NSCs compared to the WT NSCs (Additional file 1 : Figure S5), indicating an absence of lysosomal accumulation of unesterified cholesterol in these TSD patient cells.
Together, these results revealed a disease phenotype of significant increase in lipid accumulation in both TSD NSC lines differentiated from patient iPSCs. Thus, these patient NSCs may serve as a cell-based disease model to study disease pathology to evaluate drug efficacy.
GM2 ganglioside accumulation in TSD NSCs
To examine the accumulation of GM2 gangliosides in TSD patient cells, immunofluorescence staining of GM2 were carried out. No significant difference was observed in both TSD patient NSCs compared to the WT control (Additional file 1 : Figure S4A and 4C).
GM2 profiling was further performed with LC-MS/MS analysis. In contrast to the immunofluorescence staining data, LC-MS/MS analysis showed significant elevation of GM2 levels in both TSD patient cell lines (NSC HT134A and NSC HT151A) compared to the WT control NSCs (Fig. 3 ).
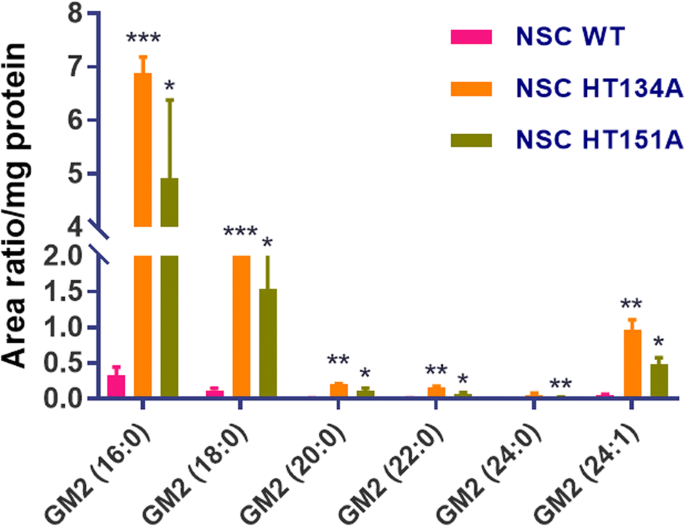
LC-MS/MS analysis of GM2 profile in TSD NSCs. The relative quantification data were provided as area ratios of GM2 species to internal standard, which were normalized to the protein level. Significant elevation of GM2 level was detected in TSN NSCs compared to the WT NSCs. All samples were run in the same batch. Data are displayed as mean ± SD. * p < 0.05, ** p < 0.01, *** p < 0.001, compared to the WT control
Recombinant human Hex A reduced lipid accumulation in TSD NSCs
To further validate this cell-based disease model, we evaluated the effect of recombinant human Hex A protein on rescuing the disease phenotype of TSD NSCs. Treatment of NSCs with 100 nM Hex A significantly reduced Nile Red dye staining in TSD NSC lines (HT134A and HT151A) (Fig. 4 ). Nile Red dye staining in both NSC lines of HT134A and HT151A was reduced to a low level similar to WT cells, indicating significant reduction of lipid accumulation in lysosomes. These results demonstrate that recombinant Hex A treatment rescued the disease phenotype in the TSD NSCs.
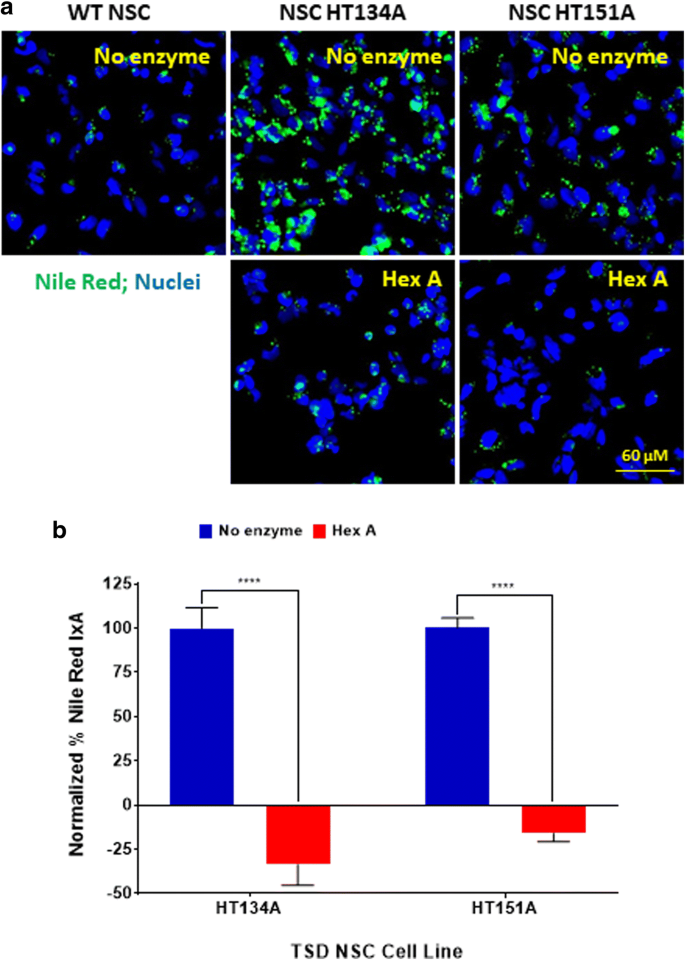
Effect of hexosaminidase A (Hex A) on lipid accumulation in TSD NSCs. a Nile Red staining of NSC HT134A and NSC HT151A after 4 h incubation with Hex A. b Normalized percent Nile Red IxA of TSD NSCs after treatment with Hex A. Nile Red IxA values of TSD NSCs treated with HexA were normalized to untreated TSD NSCs as 100% and Nile Red IxA of WT NSCs as 0%. Data are mean ± SEM ( n = 30), **** p < 0.0001, compared to each other. IxA, integrated cell intensity
δ-Tocopherol and HPβCD reduced lipid accumulation in TSD NSCs
δ-Tocopherol and HPβCD have been reported to significantly reduce lysosomal accumulation of cholesterol and decrease the enlargement of lysosomes in NPC1 and NPA cells [ 10 , 13 , 23 ]. The positive effects of δ-tocopherol were previously observed in the TSD fibroblasts [ 9 ]. Here, we examined the effects of δ-tocopherol and HPβCD on lipids accumulated in TSD NSCs using the Nile Red dye staining. We found that both compounds reduced Nile Red dye staining in a dose-dependent manner (Figs. 5 and 6 ). The half maximal effective concentration (EC 50 ) values of δ-tocopherol were 14.5 μM in HT134A and 10.9 μM in HT151A lines. Treatment with 20 μM δ-tocopherol displayed a 75% and 83% decrease of Nile Red staining compared to the untreated patient cell lines, HT134A and HT151A, respectively (Fig. 5b ). Treatment with 500 μM HPβCD dramatically reduced the Nile Red dye staining to a level similar to WT NSCs, with a 97% reduction in the TSD HT134A line and a 92% decrease in the HT151A line. The EC 50 values for TSD NSCs treated with HPβCD were 183.8 μM in cell line HT134A and 261.1 μM in cell line HT151A (Fig. 6b ). Together, the results demonstrate that δ-tocopherol and HPβCD significantly ameliorated the lipid accumulation in the TSD NSCs.
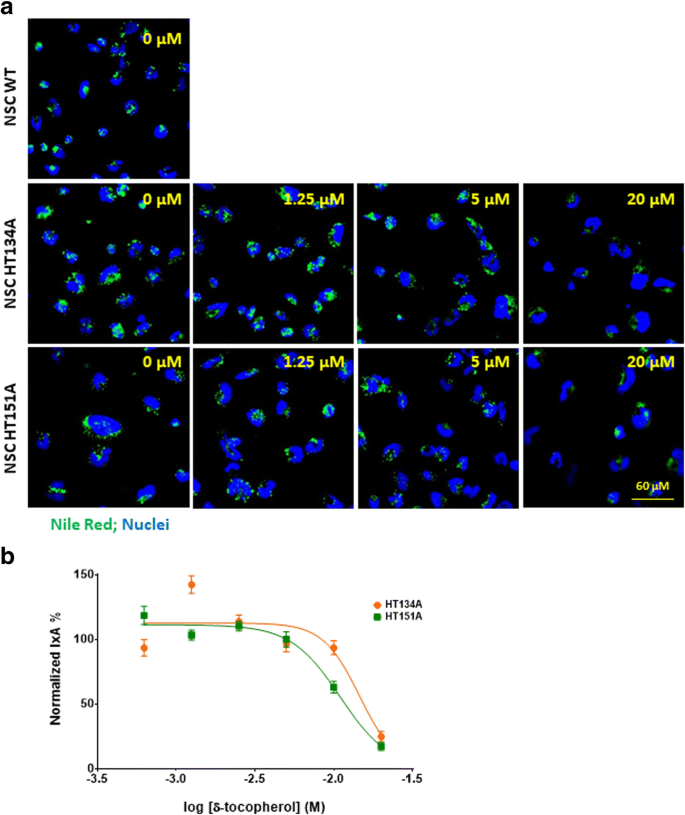
Reduction of lipid accumulation in TSD NSCs after treatment with δ-tocopherol. a Nile Red staining of NSC HT134A and HT151A after 48 h of treatment with δ-tocopherol ranging from 0 to 20 uM. b Normalized percent IxA of TSD NSCs after treatment with δ-tocopherol ( n = 40; SEM). Nile Red IxA of TSD NSCs treated with δ-tocopherol were normalized to IxA of untreated TSD NSCs set as 100% and IxA of WT NSCs set as 0%. IxA, integrated cell intensity
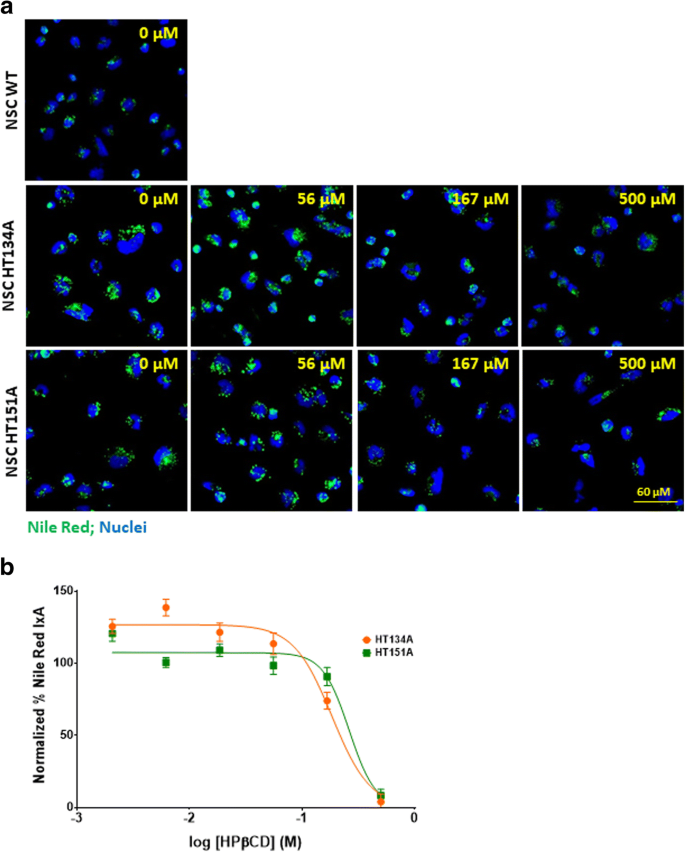
Reduction of lipid accumulation in TSD NSCs after treatment with HPβCD. a Nile Red staining of TSD NSC HT134A and HT151A after 48 h treatment with HPβCD ranging from 0 to 500 uM. b Normalized percent Nile Red IxA of TSD NSCs after treated with HPβCD (n = 40; SEM). Nile Red IxA of HPβCD treated TSD NSCs were normalized to untreated TSD NSCs IxA as 100% and WT NSCs IxA as 0%. IxA, integrated cell intensity
To improve the therapeutic effect and reduce the drug concentrations needed to achieve the maximal response, a combination therapy of both δ-tocopherol and HPβCD was also tested. This combination showed a significantly decrease of lipid accumulation in TSD NSCs (Fig. 7 ). A combination of 50 μM HPβCD and 5 μM δ-tocopherol, both at lower concentrations than either one alone, was effective in reducing lipid accumulation. At these concentrations (50 μM of HPβCD and 5 μM of δ-tocopherol), no significant reduction of lipid accumulation was observed when either compound was used alone. The combination of 5 μM δ-tocopherol and 50 μM HPβCD significantly decreased lipid accumulation to levels comparable to WT cells, suggesting a synergistic effect (Fig. 7a, b ). Additionally, an ATP content assay confirmed the cell viability from different experimental groups (Fig. 7c ).
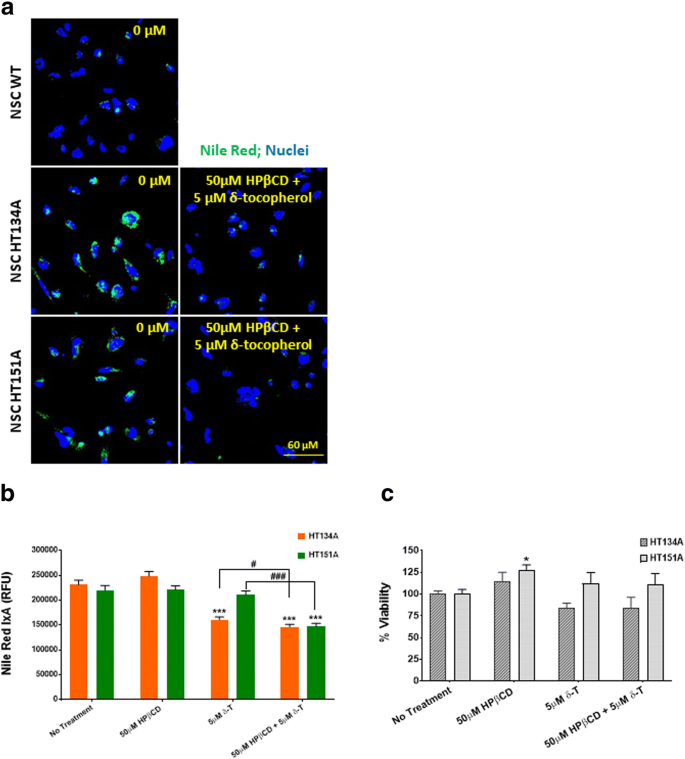
Combination treatment of HPβCD and δ-tocopherol reduces lipid accumulation in TSD NSCs. a Nile Red staining images of TSD NSCs compared to WT NSCs following compound combination treatment. b Nile Red IxA of NSC HT134A and HT151A after 48 h incubation with HPβCD and δ-tocopherol. c Cell viability of TSD NSCs after compound combination treatment. * p < 0.5, *** p < 0.001 compared to untreated control. # p < 0.05, ### p < 0.001 compared to each other. IxA, integrated cell intensity
Neuronal cell differentiation from Tay-Sachs iPS cells
We further differentiated TSD NSCs and WT NSCs into neuronal cells. Immunofluorescence staining of major neuronal cell makers demonstrated that the neuronal cells differentiated from TSD and WT NSCs lines expressed high levels of neuronal cell protein markers MAP2, ß-III-Tubulin, Neurofilament-L, but not Nestin (a protein marker of neural stem cells). (Fig. 8a ). The results provide evidence for the generation of TSD neurons from patient NSCs cells.
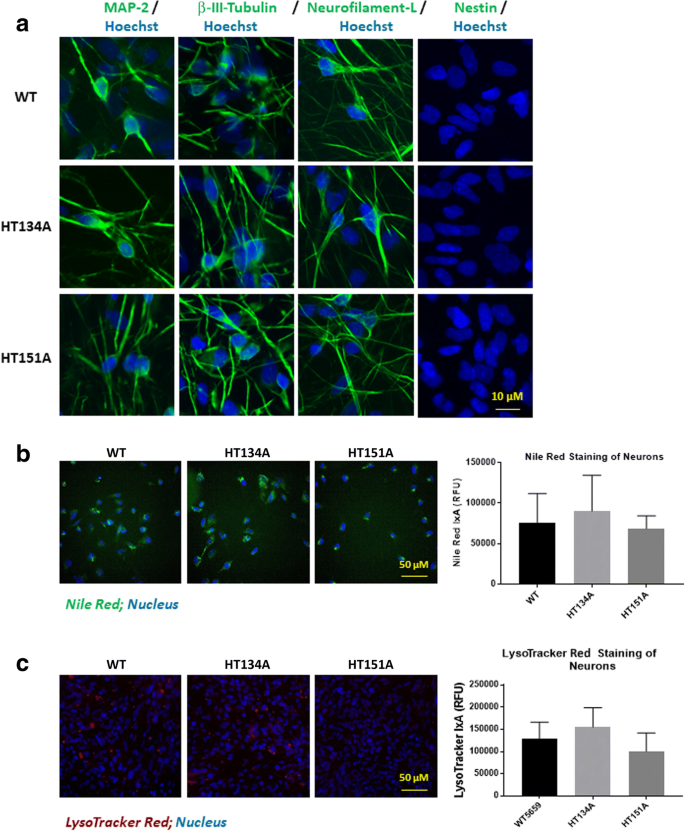
Neuronal cell differentiation from Tay-Sachs iPSCs. a Immunofluorescence staining of TSD and WT neurons. MAP2, β-III-tubulin and Neurofilament-L are neuronal cell protein markers while Nestin is a neural stem cell marker, serving as the negative control. b Nile red staining on TSD and WT neurons. c LysoTracker red staining on TSD and WT neurons. No significant increase of Nile red staining or LysoTracker red staining was observed in TSD neurons compared to the WT control cells. Data are displayed as mean ± SD. * p < 0.05, compared to the WT control. IxA, integrated cell intensity
Nile Red staining and LysoTracker Red staining were carried out to evaluate the lipid accumulation and lysosome enlargement in TSD neurons. However, we only observed a small, but not significant increase of both Nile Red fluorescence signal (Fig. 8b ) and lysoTracker Red staining signal (Fig. 8c ) in one of the patient cell lines, HT131A, but not in HT151 patient line compared to the WT control neurons.
δ-Tocopherol and HPβCD had been reported to significantly reduce lysosomal accumulation of cholesterol and decrease the enlargement of lysosomes in NPC1 and NPA cells [ 10 , 13 , 23 ].
Recent advances in iPSC technology have enabled the generation of patient iPSC lines from skin fibroblasts and other cells such as peripheral blood mononuclear cells (PBMCs). These patient-derived iPSC are self-renewable and can be differentiated to many cell types previously hard to obtain including neuronal cells, cardiomyocytes, and hepatocytes [ 13 , 24 , 25 , 26 , 27 , 28 ]. Neural stem cells can self-renew and also exhibit the characteristic disease phenotype of several lysosomal diseases including Niemann Pick disease type C (NPC), NPA and Wolman disease [ 10 , 11 , 13 ]. Other groups have also employed patient iPSC-derived NSCs or neurons to study disease pathogenesis and phenotypes such as neuropsychiatric diseases [ 27 ]. Those patient-derived cells have also been used as cell-based disease models to evaluate drug efficacy and phenotypic high throughput screening (HTS) to identify new lead compounds for drug discovery [ 10 , 11 , 13 ] [ 29 ].
In this study, we have successfully generated two Tay-Sachs disease patient iPSC lines and further differentiated them into NSCs that exhibit a characteristic disease phenotype of lysosomal lipid accumulation. GM2 is the primary lipid accumulated in the Tay-Sachs’ patient cells [ 2 ]. However, we found that the immunostaining with anti-GM2 antibodies did not reveal difference between the patient cells and wild type control cells. This might be due to the issue with specificity of commercially available GM2 antibodies that are not sensitive enough for the immunostaining experiment. Further study using MS method confirmed the accumulation of GM2 lipid in the patient cells. However, the GM2 measurement using MS method requires a significant amount of resources and cells without the high-throughput necessary for compound screening.
In addition to GM2 ganglioside buildup, secondary accumulation of other lipids including phospholipids, cerebrosides, sphingomyelin, and cholesterol have been reported [ 30 ]. Nile Red is a hydrophobic and metachromatic dye, which yields fluorescence varying from deep red to strong yellow-gold depending on the hydrophobicity of the lipid environment [ 17 ]. In this study, we found that the increased Nile Red dye staining in TSD patient NSCs could be rescued by recombinant human Hex A protein. Thus, these patient cells can be used as a cell-based disease model and Nile Red staining offers a valid method for evaluating drug efficacy and compound screening. The significant lipid accumulation was not observed in the patient iPSC-derived neurons in our experiments. It could be due to a lack of neuronal maturation. The images of LysoTracker Red and Nile Red staining in neurons also exhibited high variability in individual cells, indicating that the current method may not be sensitive enough for neuronal detection. Currently, production of large amount of neurons is still a bottleneck for compound screening assays. The reproducibility of iPSC-differentiated neurons from batch to batch can not be easily controlled. Therefore, we have used the NSCs [ 9 , 10 , 11 , 12 ] as a model system for evaluation of compound efficacy and compound screening because NSCs can be produced in large quantities with good reproducibility.
Previously, ERT for TSD was tested in clinical trials through intraventricular delivery and injections into the spinal canal. However, no reduction of GM2 ganglioside accumulation was detected in patients due to the limited amount of the enzyme that was able to reach neural cells [ 8 ]. In this study, we found that patient NSCs treated with Hex A exhibited decreased lipid accumulation (Fig. 4 ). It has been reported that just 10% of normal activity of Hex A is required for patients to be characterized as WT [ 31 ]. Therefore, only a small amount of Hex A activity is needed to ameliorate the disease phenotype.
Even though ERT has proven to be an effective treatment in several other lysosomal storage disorders like Gaucher disease, and Pompe diseases, ERT is not feasible for TSD [ 5 ] because recombinant Hex A cannot cross the blood-brain barrier (BBB) and therefore cannot be effective for neurological symptoms [ 8 ]. The recombinant enzyme may be directly administrated into brain via intrathecal or intraparenchymal injection. But the procedure is invasive and usually not suggested as the best route of treatment due to its high safety risks and costs [ 32 , 33 ]. Furthermore, ERT for lysosomal storage diseases generally requires frequent administration [ 34 ].
Nonetheless, ERT should be further investigated and developed for patients with TSD to ameliorate symptoms in the peripheral body such as muscle weakness, spasm, and paralysis. New technologies and methods are needed for efficient and safe delivery of recombinant Hex A protein into the brain. One of the methods currently under study is using ultrasound to temporarily increase the permeability of the BBB, as well as decreasing the efflux of transporters to allow more passive diffusion of hydrophobic compounds [ 35 ]. Additionally, liposomes and their polymer analog, polymerosomes, have been used in conjugation with ligands or polymer chains that allow for specific drug delivery across the BBB [ 33 , 35 ] and enable ERT for lysosomal storage diseases with neurological effects.
We also evaluated two small molecules, HPβCD and δ-tocopherol, using the TSD patient NSCs. Both compounds effectively reduced lipid accumulation in TSD NSCs. However, a much higher concentration of HPβCD is needed to reduced lipid accumulation in TSD cells compared to NPC1 NSCs [ 13 ]. The concentration of HPβCD can be significantly reduced when it was used in combination with δ-tocopherol. It has been reported that both δ-tocopherol and HPβCD decrease cholesterol accumulation in NPC1 cells by inducing lysosomal exocytosis [ 9 , 36 ]. Although the exact mechanisms of action for both δ-tocopherol and HPβCD in TSD NSCs are unclear, it is possible that there is a synergistic effect when the two compounds are used as a combination therapy.
Conclusions
Two lines of iPSCs have been generated from TSD patient fibroblasts, and the NSCs derived from these TSD iPSCs exhibit the disease phenotype of lipid accumulation in lysosomes. ERT with recombinant Hex A protein rescues the disease phenotype in the TSD NSCs. HPβCD and δ-tocopherol also reduce the lysosomal lipid accumulation in patient-derived NSCs. Overall, our data demonstrate that the patient-derived NSCs can be used as a cell-based disease model to study disease pathology and perform compound screening for drug development.
Mahuran DJ. Biochemical consequences of mutations causing the GM2 gangliosidoses. Biochim Biophys Acta. 1999;1455(2–3):105–38.
Article PubMed CAS Google Scholar
Patterson MC. Gangliosidoses. Handb Clin Neurol. 2013;113:1707–8.
Article PubMed Google Scholar
Clarke JT, Mahuran DJ, Sathe S, Kolodny EH, Rigat BA, Raiman JA, Tropak MB. An open-label phase I/II clinical trial of pyrimethamine for the treatment of patients affected with chronic GM2 gangliosidosis (Tay-Sachs or Sandhoff variants). Mol Genet Metab. 2011;102(1):6–12.
Colaianni A, Chandrasekharan S, Cook-Deegan R. Impact of gene patents and licensing practices on access to genetic testing and carrier screening for Tay-Sachs and Canavan disease. Genet Med. 2010;12(4 Suppl):S5–S14.
Article PubMed PubMed Central Google Scholar
Baldo BA. Enzymes approved for human therapy: indications, mechanisms and adverse effects. BioDrugs. 2015;29(1):31–55.
Akeboshi H, Chiba Y, Kasahara Y, Takashiba M, Takaoka Y, Ohsawa M, Tajima Y, Kawashima I, Tsuji D, Itoh K, et al. Production of recombinant beta-hexosaminidase a, a potential enzyme for replacement therapy for Tay-Sachs and Sandhoff diseases, in the methylotrophic yeast Ogataea minuta. Appl Environ Microbiol. 2007;73(15):4805–12.
Article PubMed PubMed Central CAS Google Scholar
Ohsawa M, Kotani M, Tajima Y, Tsuji D, Ishibashi Y, Kuroki A, Itoh K, Watabe K, Sango K, Yamanaka S, et al. Establishment of immortalized Schwann cells from Sandhoff mice and corrective effect of recombinant human beta-hexosaminidase a on the accumulated GM2 ganglioside. J Hum Genet. 2005;50(9):460–7.
von Specht BU, Geiger B, Arnon R, Passwell J, Keren G, Goldman B, Padeh B. Enzyme replacement in Tay-Sachs disease. Neurology. 1979;29(6):848–54.
Xu M, Liu K, Swaroop M, Porter FD, Sidhu R, Firnkes S, Ory DS, Marugan JJ, Xiao J, Southall N, et al. delta-tocopherol reduces lipid accumulation in Niemann-pick type C1 and Wolman cholesterol storage disorders. J Biol Chem. 2012;287(47):39349–60.
Long Y, Xu M, Li R, Dai S, Beers J, Chen G, Soheilian F, Baxa U, Wang M, Marugan JJ, et al. Induced pluripotent stem cells for disease modeling and evaluation of therapeutics for Niemann-pick disease type a. Stem Cells Transl Med. 2016;5(12):1644–55.
Aguisanda F, Yeh CD, Chen CZ, Li R, Beers J, Zou J, Thorne N, Zheng W. Neural stem cells for disease modeling of Wolman disease and evaluation of therapeutics. Orphanet J Rare Dis. 2017;12(1):120.
Sima N, Li R, Huang W, Xu M, Beers J, Zou J, Titus S, Ottinger EA, Marugan JJ, Xie X, et al. Neural stem cells for disease modeling and evaluation of therapeutics for infantile (CLN1/PPT1) and late infantile (CLN2/TPP1) neuronal ceroid lipofuscinoses. Orphanet J Rare Dis. 2018;13(1):54.
Yu D, Swaroop M, Wang M, Baxa U, Yang R, Yan Y, Coksaygan T, DeTolla L, Marugan JJ, Austin CP, et al. Niemann-pick disease type C: induced pluripotent stem cell-derived neuronal cells for modeling neural disease and evaluating drug efficacy. J Biomol Screen. 2014;19(8):1164–73.
Park IH, Arora N, Huo H, Maherali N, Ahfeldt T, Shimamura A, Lensch MW, Cowan C, Hochedlinger K, Daley GQ. Disease-specific induced pluripotent stem cells. Cell. 2008;134(5):877–86.
Avior Y, Sagi I, Benvenisty N. Pluripotent stem cells in disease modelling and drug discovery. Nat Rev Mol Cell Biol. 2016;17(3):170–82.
Beers J, Gulbranson DR, George N, Siniscalchi LI, Jones J, Thomson JA, Chen G. Passaging and colony expansion of human pluripotent stem cells by enzyme-free dissociation in chemically defined culture conditions. Nat Protoc. 2012;7(11):2029–40.
Greenspan P, Mayer EP, Fowler SD. Nile red: a selective fluorescent stain for intracellular lipid droplets. J Cell Biol. 1985;100(3):965–73.
Kruth HS. Localization of unesterified cholesterol in human atherosclerotic lesions. Demonstration of filipin-positive, oil-red-O-negative particles. Am J Pathol. 1984;114(2):201–8.
PubMed PubMed Central CAS Google Scholar
Gray-Edwards HL, Jiang X, Randle AN, Taylor AR, Voss TL, Johnson AK, McCurdy VJ, Sena-Esteves M, Ory DS, Martin DR. Lipidomic evaluation of feline neurologic disease after AAV gene therapy. Mol Ther Methods Clin Dev. 2017;6:135–42.
Espejo-Mojica AJ, Mosquera A, Rodríguez-López A, Díaz D, Beltrán L, Hernandez FL, Alméciga-Díaz CJ, Barrera LA. Characterization of recombinant human lysosomal beta-hexosaminidases produced in the methylotrophic yeast Pichia pastoris. Universitas Scientarium . 2016;21(3):195–217.
Rodriguez-Lopez A, Almeciga-Diaz CJ, Sanchez J, Moreno J, Beltran L, Diaz D, Pardo A, Ramirez AM, Espejo-Mojica AJ, Pimentel L, et al. Recombinant human N-acetylgalactosamine-6-sulfate sulfatase (GALNS) produced in the methylotrophic yeast Pichia pastoris. Sci Rep. 2016;6:29329.
Shapira E, Blitzer MB, Africk DK, Miller JB: Biochemical genetics: a laboratory manual 1st edition edn: Oxford University press; 1989.
Li R, Hao J, Fujiwara H, Xu M, Yang S, Dai S, Long Y, Swaroop M, Li C, Vu M, et al. Analytical characterization of methyl-beta-Cyclodextrin for pharmacological activity to reduce lysosomal cholesterol accumulation in Niemann-pick disease type C1 cells. Assay Drug Dev Technol. 2017;15(4):154–66.
Lin Y, Linask KL, Mallon B, Johnson K, Klein M, Beers J, Xie W, Du Y, Liu C, Lai Y, et al. Heparin promotes cardiac differentiation of human pluripotent stem cells in chemically defined albumin-free medium, enabling consistent manufacture of cardiomyocytes. Stem Cells Transl Med. 2017;6(2):527–38.
Lian X, Zhang J, Azarin SM, Zhu K, Hazeltine LB, Bao X, Hsiao C, Kamp TJ, Palecek SP. Directed cardiomyocyte differentiation from human pluripotent stem cells by modulating Wnt/beta-catenin signaling under fully defined conditions. Nat Protoc. 2013;8(1):162–75.
Burridge PW, Matsa E, Shukla P, Lin ZC, Churko JM, Ebert AD, Lan F, Diecke S, Huber B, Mordwinkin NM, et al. Chemically defined generation of human cardiomyocytes. Nat Methods. 2014;11(8):855–60.
Wen Z, Nguyen HN, Guo Z, Lalli MA, Wang X, Su Y, Kim NS, Yoon KJ, Shin J, Zhang C, et al. Synaptic dysregulation in a human iPS cell model of mental disorders. Nature. 2014;515(7527):414–8.
Hannan NR, Segeritz CP, Touboul T, Vallier L. Production of hepatocyte-like cells from human pluripotent stem cells. Nat Protoc. 2013;8(2):430–7.
Wainger BJ, Kiskinis E, Mellin C, Wiskow O, Han SS, Sandoe J, Perez NP, Williams LA, Lee S, Boulting G, et al. Intrinsic membrane hyperexcitability of amyotrophic lateral sclerosis patient-derived motor neurons. Cell Rep. 2014;7(1):1–11.
Walkley SU, Vanier MT. Secondary lipid accumulation in lysosomal disease. Biochim Biophys Acta. 2009;1793(4):726–36.
Mahuran DJ. The biochemistry of HEXA and HEXB gene mutations causing GM2 gangliosidosis. Biochim Biophys Acta. 1991;1096(2):87–94.
Desnick RJ. Enzyme replacement and enhancement therapies for lysosomal diseases. J Inherit Metab Dis. 2004;27(3):385–410.
Muro S. Strategies for delivery of therapeutics into the central nervous system for treatment of lysosomal storage disorders. Drug Deliv Transl Res. 2012;2(3):169–86.
Matsuoka K, Tamura T, Tsuji D, Dohzono Y, Kitakaze K, Ohno K, Saito S, Sakuraba H, Itoh K. Therapeutic potential of intracerebroventricular replacement of modified human beta-hexosaminidase B for GM2 gangliosidosis. Mol Ther. 2011;19(6):1017–24.
Bicker J, Alves G, Fortuna A, Falcao A. Blood-brain barrier models and their relevance for a successful development of CNS drug delivery systems: a review. Eur J Pharm Biopharm. 2014;87(3):409–32.
Chen FW, Li C, Ioannou YA. Cyclodextrin induces calcium-dependent lysosomal exocytosis. PLoS One. 2010;5(11):e15054.
Download references
Acknowledgements
The authors would like to thank Miao Xu and Shu Yang for WT NSC induction, the compound management group at NCATS, NIH for their professional support and Dr. DeeAnn Visk for editing this manuscript.
This work was supported by the Intramural Research Program of the Therapeutics for Rare and Neglected Diseases, National Center for Advancing Translational Sciences, National Institutes of Health. This work was also supported by the doctoral scholarship from the Administrative Department of Science, Technology and Innovation COLCIENCIAS, Colombia, and the Pontificia Universidad Javeriana (to AJEM and ARL), by the grants from the Pontificia Universidad Javeriana (Grant ID 7204 to CJAD, Grant ID 3637 to LAB) and COLCIENCIAS (Grant No. 120356933205 and 120356933427 to CJAD, Grant ID No. 120352128575 to LAB).
Availability of data and materials
The datasets used and/or analyzed during the current study are available from the corresponding author on reasonable request.
Author information
Authors and affiliations.
National Center for Advancing Translational Sciences, National Institutes of Health, Bethesda, MD, USA
Mylinh Vu, Rong Li, Amanda Baskfield, Billy Lu, Atena Farkhondeh, Kirill Gorshkov, Omid Motabar, Juan J. Marugan & Wei Zheng
Center for Molecular Medicine, National Heart, Lung, and Blood Institute, National Institutes of Health, Bethesda, MD, USA
Jeanette Beers, Guokai Chen & Jizhong Zou
Faculty of Health Sciences, University of Macau, Macau, People’s Republic of China
Guokai Chen
Institute for the Study of Inborn Errors of Metabolism, Faculty of Sciences, Pontificia Universidad Javeriana, Bogotá, Colombia
Angela J. Espejo-Mojica, Alexander Rodríguez-López, Carlos J. Alméciga-Díaz & Luis A. Barrera
Chemistry Department, Faculty of Science, Pontificia Universidad Javeriana, Bogotá, Colombia
Alexander Rodríguez-López
Diabetic Cardiovascular Disease Center, Washington University School of Medicine, St. Louis, MO, USA
Xuntian Jiang & Daniel S. Ory
You can also search for this author in PubMed Google Scholar
Contributions
WZ, RL and MV designed the study. JJM, DO aided study design. MV, RL, AB, BL, AF, KG and OM performed the experiments and analyzed the data. JB, GC and JZ generated iPSCs. AJEM, ARL, CJAD and LAB produced recombinant rhHexA protein. XJ performed the mass spectrometry analysis. MV, RL and WZ wrote the manuscript. All authors discussed the results and commented on the manuscript. All authors read and approved the final manuscript.
Corresponding author
Correspondence to Wei Zheng .
Ethics declarations
Ethics approval and consent to participate.
Not applicable.
Consent for publication
Competing interests.
The authors declared no potential conflicts of interest with respect to the research, authorship, and/or publication of this article.
Publisher’s Note
Springer Nature remains neutral with regard to jurisdictional claims in published maps and institutional affiliations.
Additional file
Additional file 1:.
Figure S1. Tay-Sachs disease induced pluripotent stem cells (iPSCs) generation and neuronal stem cells (NSCs) differentiation. Figure S2. Characterization of Tay-Sachs disease iPSCs. Figure S3. Tay-Sachs disease NSCs express increased lipid accumulation and lysosomal size compared to WT NSCs. Figure S4. Immunofluorescence staining of GM2 in TSD patient NSCs and neurons.
Rights and permissions
Open Access This article is distributed under the terms of the Creative Commons Attribution 4.0 International License ( http://creativecommons.org/licenses/by/4.0/ ), which permits unrestricted use, distribution, and reproduction in any medium, provided you give appropriate credit to the original author(s) and the source, provide a link to the Creative Commons license, and indicate if changes were made. The Creative Commons Public Domain Dedication waiver ( http://creativecommons.org/publicdomain/zero/1.0/ ) applies to the data made available in this article, unless otherwise stated.
Reprints and permissions
About this article
Cite this article.
Vu, M., Li, R., Baskfield, A. et al. Neural stem cells for disease modeling and evaluation of therapeutics for Tay-Sachs disease. Orphanet J Rare Dis 13 , 152 (2018). https://doi.org/10.1186/s13023-018-0886-3
Download citation
Received : 21 November 2017
Accepted : 06 August 2018
Published : 17 September 2018
DOI : https://doi.org/10.1186/s13023-018-0886-3
Share this article
Anyone you share the following link with will be able to read this content:
Sorry, a shareable link is not currently available for this article.
Provided by the Springer Nature SharedIt content-sharing initiative
- Tay-Sachs disease
- Induced pluripotent stem cells
- Neural stem cells
- Cyclodextrin
- δ-tocopherol
- Hexosaminidase A
- GM2 gangliosidosis
- High throughput screening
- Drug discovery
Orphanet Journal of Rare Diseases
ISSN: 1750-1172
- Submission enquiries: Access here and click Contact Us
- General enquiries: [email protected]
Tay Sachs Disease
Tay-Sachs disease (abbreviated TSD, also known as GM2 gangliosidosis or Hexosaminidase A deficiency) is an autosomal recessive genetic disorder. In its most common variant known as infantile Tay-Sachs disease it presents with a relentless deterioration of mental and physical abilities which commences at 6 months of age and usually results in death by the age of four.
Tay-Sachs Disease Pathophysiology
Tay-sachs disease diagnosis, tay-sachs disease prevention, feature articles, tay-sachs disease society and culture, tay-sachs disease research, tay-sachs disease symptoms, latest tay sachs disease news and research.
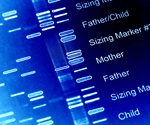
Quick genetic test offers hope for sick, undiagnosed kids. but few insurers offer to pay.
Just 48 hours after her birth in a Seattle-area hospital in 2021, Layla Babayev was undergoing surgery for a bowel obstruction.
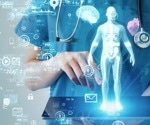
ChatGPT: A diagnostic sidekick for doctors? Caution advised for non-professionals
Research evaluates the diagnostic accuracy of ChatGPT, an AI language model, in providing medical advice online. It concludes that ChatGPT can be a useful tool for assisting human doctors in diagnosing difficult cases, but non-professionals should use it with caution.
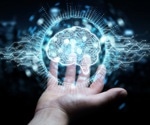
AI tool promises to accelerate the development of gene therapies on a large scale
An artificial intelligence (AI) program may enable the first simple production of customizable proteins called zinc fingers to treat diseases by turning genes on and off.
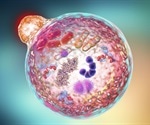
U-M researchers reveal how a mutated protein acts as risk factor in Parkinson’s disease
In about a fifth of the cases of Parkinson’s disease, look to a small, malfunctioning protein in the lysosome as a risk factor, say University of Michigan researchers.
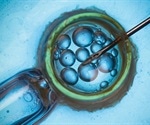
Using genetic screening for selecting the 'best' embryo in IVF
Each year, tens of thousands of babies are born in the United States as the result of IVF (in vitro fertilization) – where several eggs are fertilized outside the mother's body and then an embryo is selected for transfer into her womb.
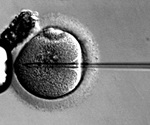
A new combination therapy for treating rare, fatal Sanfilippo syndrome in children
A study aiming to develop a new therapeutic technique could bring a revolution in our approach to treating rare, fatal Sanfilippo syndrome, a disorder that affects children as young as 2 years old and leads to childhood dementia and premature death.
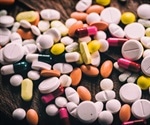
Study underscores the need for more precise rare disease definitions
Some diseases are like black swans. They occur so rarely that many physicians never encounter them in their clinical practice, complicating efforts to treat them.
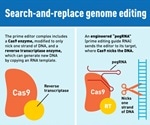
New CRISPR genome “prime editing” system
Gene editing to correct genetic faults and removing the risk of hereditary diseases may soon become reality. Researchers have come up with a new gene editing technology that could help patients with sickle cell anaemia and up 89 percent of other genetic defects.
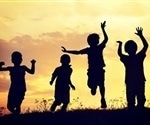
$28 million grant supports Cincinnati Children's role as coordinating center for Rare Diseases Clinical Research Network
The federal agency that oversees a large network of research centers seeking cures for hundreds of rare, disabling and often fatal diseases has named Cincinnati Children's as its new data management and coordinating center.
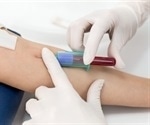
New blood test detects genetic disorders in fetuses
Tel Aviv University researchers have developed a new blood test for genetic disorders that may allow parents to learn about the health of their baby as early as 11 weeks into pregnancy.
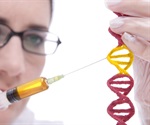
Gene therapy is the best stratagem to impact the course of GM2 gangliosidosis
Since its clinical description in 1881, Tay-Sachs disease has had almost totemic significance as a cruel and relentless genetic condition that destroys the developing brain of babies and young children.
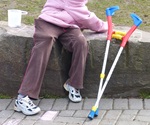
Actress Kiruna Stamell argues about gene editing with Dr Christopher Gyngell
Two papers published today by the Journal of the Royal Society of Medicine, debate gene editing and the health of future generations.
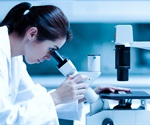
New gene therapy shows promising results for treating neurodegenerative disorders
A new gene therapy approach designed to replace the enzyme that is deficient in patients with the inherited neurodegenerative disorders Tay-Sachs and Sandhoff diseases successfully delivered the therapeutic gene to the brains of treated mice, restored enzyme function, and extended survival by about 2.5-fold.
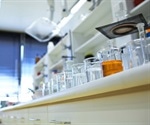
Mutation that increases sphingolipid levels can lead to neurodegeneration
A mutation that increases the level of a special class of sphingolipids--molecules important to cell structure and signaling--can lead to neurodegeneration due to problems with neuronal membranes, reports a research team led by Jackson Laboratory Research Scientist Lihong Zhao, Ph.D. and Professor Patsy Nishina, Ph.D.
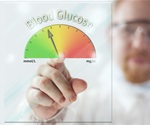
Researchers find genetic mutation that causes glycogen storage disease type IIIa in Inuit
A team of Canadian and Japanese researchers has identified the genetic mutation responsible for glycogen storage disease type IIIa in Inuit in northern Quebec, Canada, in a study published in CMAJ (Canadian Medical Association Journal).
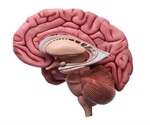
Genetic basis of hereditary disease causes severe brain atrophy in Jews of Moroccan ancestry
Ben-Gurion University of the Negev (BGU) researchers have unraveled the genetic basis of a hereditary disease that causes severe brain atrophy, mental retardation and epilepsy in Jews of Moroccan ancestry, according to a study published this week online in the Journal of Medical Genetics.
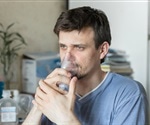
LUHS offers highly accurate prenatal test to determine chromosomal abnormalities in fetus
Loyola University Health System (LUHS) now offers chorionic villus sampling (CVS), a highly accurate prenatal test for chromosomal abnormalities in the fetus. Loyola is one of the few centers in the Chicago area to offer CVS.
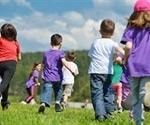
Available drugs may have a dramatic impact on children stricken with Tay-Sachs disease
A team of researchers has made a significant discovery which may have a dramatic impact on children stricken with Tay-Sachs disease, a degenerative and fatal neurological condition that often strikes in the early months of life.
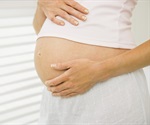
Counsyl, ARUP partner to expand pregnancy genetic test offering for prospective parents
Counsyl has partnered with ARUP Laboratories, a nonprofit enterprise of the University of Utah, to expand its pre-pregnancy and early pregnancy genetic test offering for prospective parents. ARUP's molecular genetic testing laboratory already provides a comprehensive set of testing options for a broad range of inherited disorders.
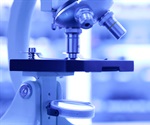
Statistical method uses evolutionary information to enhance identification of disease-associated alleles
A book containing misprints may cause annoyance for the reader, but typos in an individual's genetic blueprint (DNA) can mean serious disease or even death. The search for genetic correlates for the wide range of diseases plaguing humankind has inspired a wealth of research falling under the heading of genome-wide association studies (GWAS).
- Trending Stories
- Latest Interviews
- Top Health Articles

How can microdialysis benefit drug development
Ilona Vuist
In this interview, discover how Charles River uses the power of microdialysis for drug development as well as CNS therapeutics.

Global and Local Efforts to Take Action Against Hepatitis
Lindsey Hiebert and James Amugsi
In this interview, we explore global and local efforts to combat viral hepatitis with Lindsey Hiebert, Deputy Director of the Coalition for Global Hepatitis Elimination (CGHE), and James Amugsi, a Mandela Washington Fellow and Physician Assistant at Sandema Hospital in Ghana. Together, they provide valuable insights into the challenges, successes, and the importance of partnerships in the fight against hepatitis.

Addressing Important Cardiac Biology Questions with Shotgun Top-Down Proteomics
In this interview conducted at Pittcon 2024, we spoke to Professor John Yates about capturing cardiomyocyte cell-to-cell heterogeneity via shotgun top-down proteomics.

Newsletters you may be interested in

Your AI Powered Scientific Assistant
Hi, I'm Azthena, you can trust me to find commercial scientific answers from News-Medical.net.
A few things you need to know before we start. Please read and accept to continue.
- Use of “Azthena” is subject to the terms and conditions of use as set out by OpenAI .
- Content provided on any AZoNetwork sites are subject to the site Terms & Conditions and Privacy Policy .
- Large Language Models can make mistakes. Consider checking important information.
Great. Ask your question.
Azthena may occasionally provide inaccurate responses. Read the full terms .
While we only use edited and approved content for Azthena answers, it may on occasions provide incorrect responses. Please confirm any data provided with the related suppliers or authors. We do not provide medical advice, if you search for medical information you must always consult a medical professional before acting on any information provided.
Your questions, but not your email details will be shared with OpenAI and retained for 30 days in accordance with their privacy principles.
Please do not ask questions that use sensitive or confidential information.
Read the full Terms & Conditions .
Provide Feedback
- Corpus ID: 21056950
Tay-Sachs disease: from clinical description to molecular defect.
- M. Kaback , R. Desnick
- Published in Advances in Genetics 2001
- Biology, Medicine
18 Citations
New approaches to correcting metabolic errors in tay-sachs, tay-sachs disease, tay-sachs disease., assessing the potential success of cystic fibrosis carrier screening: lessons learned from tay-sachs disease and β-thalassemia, juvenile gm2 gangliosidosis: a model for investigation of small-molecule therapies for lysosomal storage diseases.
- Highly Influenced
Impact of gene patents and licensing practices on access to genetic testing and carrier screening for Tay-Sachs and Canavan disease
New developments in tay-sachs disease, boy with 47,xxy,del(15)(q11.2q13) karyotype and prader–willi syndrome: a new case and review of the literature, john s. o’brien, prader-willi and klinefelter syndrome: a coincidence or not, related papers.
Showing 1 through 3 of 0 Related Papers

COMMENTS
Abstract. Tay-Sachs disease belongs to the group of autosomal-recessive lysosomal storage metabolic disorders. This disease is caused by β-hexosaminidase A (HexA) enzyme deficiency due to various mutations in α-subunit gene of this enzyme, resulting in GM2 ganglioside accumulation predominantly in lysosomes of nerve cells.
Tay Sachs disease (TSD) is a progressive, lethal neurodegenerative disorder caused by a deficiency of enzyme hexosaminidase-A resulting in the accumulation of GM2 gangliosides. Based on the presentation age, the disease is classified into infantile, juvenile, and adult forms. Early diagnosis of Tay Sachs is clinically challenging because of subtle clinical features and nonspecific biochemical ...
Tay Sachs disease (TSD) is a progressive, lethal neurodegenerative disorder caused by a deficiency of enzyme hexosaminidase-A resulting in the accumulation of GM2 gangliosides. Based on the presentation age, the disease is classified into infantile, juvenile, and adult forms. Early diagnosis of Tay …
Tay-Sachs disease is considered a prototype disease for tar geted ethnic. evaluations. (5) TSD occurs most often in children with intellectual disability, skill. regression, dementia, paralysis ...
Tay-Sachs disease (TSD) is an inherited neurological disorder caused by deficiency of hexosaminidase A (HexA). ... Source data are provided with this paper. References. Bley, A. E. et al. Natural ...
Tay-Sachs disease is an autosomal recessive disease caused by HEXA mutations. It is mainly characterized by neurodegenerative clinical manifestations in patients. While there is a wide gradient of signs and symptoms, the age of onset generally indicates disease severity and patient outcome. ... While this review focused on research and ...
Introduction. Tay-Sachs disease (TSD) or GM2 gangliosidosis variant B (MIM 272800) is an autosomal recessive neurodegenerative disorder due to β-N-acetyl-hexosaminidase A (Hex A) deficiency (Gravel et al., 2001).Hex A is a heterodimer of α and β subunits encoded by the HEXA and HEXB genes respectively. TSD is due to mutations in the HEXA gene localized on chromosome 15 (Myerowitz et al ...
Tay-Sachs disease is a rare inherited neurodegenerative disorder that destroys neurons in the brain and spinal cord in a progressive manner ( Genetics and Rare Diseases Information Center, 2018 ). TSD was named after Warren Tay and Bernard Sachs. Tay, an ophthalmologist, was the first person to describe a cherry-red spot on the retina of a patient.
Tay-Sachs disease (TSD) is an inherited condition caused by mutations in the genes encoding β-hexosaminidase A (HexA), an enzyme responsible for the recycling of GM2 gangliosides ...
Tay-Sachs disease (TSD) is a rare neurodegenerative disorder caused by mutations in the HEXA gene, which encodes the ɑ subunit of the enzyme β-hexosaminidase A. Lacking this key enzyme in GM2 ganglioside catabolism, individuals who are homozygous for HEXA mutations suffer from abnormal accumulation of GM2 ganglioside in brain and nerve cells, ultimately resulting in the progressive ...
Please use one of the following formats to cite this article in your essay, paper or report: APA. Smith, Yolanda. (2021, May 17). Tay-Sachs Disease Research.
Tay-Sachs disease, a heritable metabolic disorder commonly associated with Ashkenazi Jews, has also been found in the French Canadians of Southeastern Quebec, the Cajuns of Southwest Louisiana, and other populations throughout the world. The severity of expression and the age at onset of Tay-Sachs varies from infantile and juvenile forms that exhibit paralysis, dementia, blindness and early ...
individuals with Tay-Sachs disease have enabled a highly sensitive, straightforward, and inexpensive method for prenatal diagnosis and identification of heterozygous individuals through enzymatic testing11. Within the general population, about one in 300 individuals is a carrier, and one in 360,000 individuals is born with Tay-Sachs1. Among the ...
Myerowitz, R. & Costigan, F. C. The major defect in Ashkenazi Jews with Tay-Sachs disease is an insertion in the gene for the alpha-chain of beta-hexosaminidase. J. Biol. Chem. 263, 18587-18589 ...
Background. Lysosomal storage disorders (LSDs) are caused by deficiency of a specific lysosomal hydrolase. One of these LSDs is Tay-Sachs disease (TSD) (MIM ID # 272800) which has an autosomal recessive inheritance caused by a genetic mutation in the HEXA gene on human chromosome 15q23-q24. TSD is the second most common lipid storage disorder ...
A review of the state of the art on TSD describing its definition, epidemiology, etiology, physiopathology, clinical manifestations and news in diagnosis and treatment found it to be an autosomal recessive neurodegenerative disorder. Introduction: Lysosomal storage disease is caused by the deficiency of a single hydrolase (lysosomal enzymes). GM2 gangliosidoses are autosomal recessive ...
this article. Tay-Sachs disease is an autosomal recessive disease caused by a deficiency of β-hexosaminidase A, the lysosomal enzyme that normally degrades GM2 ganglioside. As a result, GM2 ganglioside accumulates in the lysosomes of nerve cells. The disease is one of a family of lysosomal storage disorders known as GM2 gangliosidoses, each ...
Abstract. Tay-Sachs disease is a hereditary neurodegenerative disorder resulting from excess storage of GM2 ganglioside within the lysosomes of cells, caused by deficiency of hexosaminidase A. The incidence of the disease is estimated to be 1 in 3,600 in Ashkenazi Jews (AJ) with carrier frequency of 1 in 30 and 1 in 360,000 in other population ...
Tay-Sachs disease. Tay-Sachs disease (TSD), a fatal condition, is a neurodegenerative lysosomal sphingolipid storage disorder. TSD is caused by mutations of HEXA (MIM *606869, gene map locus 15q23-q24). The HEXA gene product was identified in 1969 as the α-subunit of β-hexosaminidase enzyme (HEXA). The normal function of HEXA is to degrade GM2 gangliosides in central nervous system cell ...
Background Tay-Sachs disease (TSD) is a rare neurodegenerative disorder caused by autosomal recessive mutations in the HEXA gene on chromosome 15 that encodes β-hexosaminidase. Deficiency in HEXA results in accumulation of GM2 ganglioside, a glycosphingolipid, in lysosomes. Currently, there is no effective treatment for TSD. Results We generated induced pluripotent stem cells (iPSCs) from two ...
Tay-Sachs disease (abbreviated TSD, also known as GM2 gangliosidosis or Hexosaminidase A deficiency) is an autosomal recessive genetic disorder. In its most common variant known as infantile Tay ...
Tay-Sachs disease is a prototypic neurodegenerative disease. Lysosomal storage of GM2 ganglioside in Tay-Sachs and the related disorder, Sandhoff disease, is caused by deficiency of β-hexosaminidase A, a heterodimeric protein. Tay-Sachs-related diseases (GM2 gangliosidoses) are incurable, but gene therapy has the potential for widespread ...
In 1881 British ophthalmologist Warren Tay reported a cherry-red spot on the retina of a oneyear-old patient, a patient who was also showing signs of progressive degeneration of the central nervous system as manifested in the child's physical and mental retardation, leading to the lysosomal storage disorder known as Tay-Sachs Disease. Expand ...