Academia.edu no longer supports Internet Explorer.
To browse Academia.edu and the wider internet faster and more securely, please take a few seconds to upgrade your browser .
Enter the email address you signed up with and we'll email you a reset link.
- We're Hiring!
- Help Center
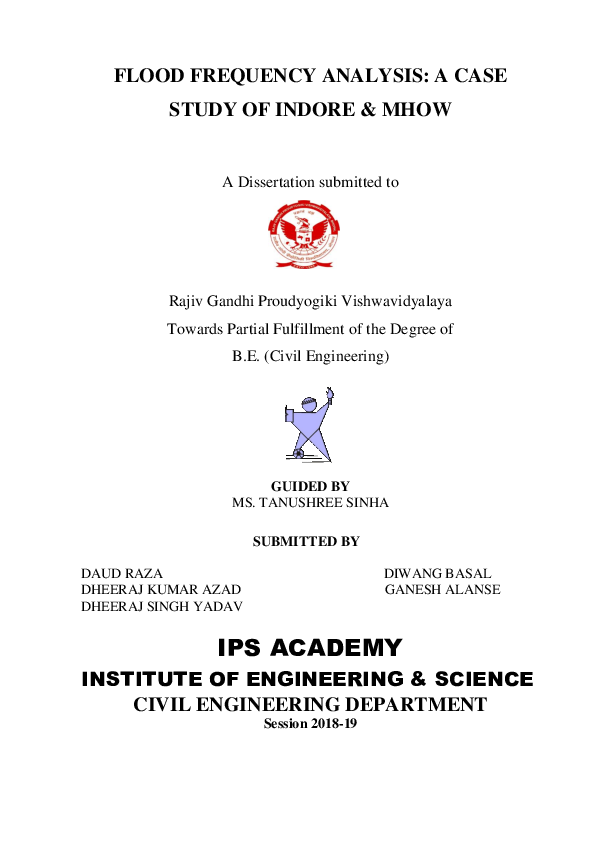

Flood Frequency Analysis: A case study of Indore and Mhow

Flood frequency analysis is a technique used by hydrologists to predict flow values corresponding to specific return periods or probabilities along a river. Flood frequency analysis is the most important statistical technique in understanding the nature and magnitude of high discharge in a river. The objective of frequency analysis is to relate the magnitude of events to their frequency of occurrence through probability distribution. Flood frequency plays a vital role in providing estimates of recurrence of floods which is used in designing structures such as dams, bridges, culverts, levees, highways, sewage disposal plants, waterworks and industrial buildings . In order to evaluate the optimum design specification for hydraulic structures, and to prevent over-designing or under designing, it is imperative to apply statistical tools to create flood frequency estimates. These estimates are useful in providing a measurement parameter to analyze the damage corresponding to specific flows during floods. The application of statistical frequency curves to floods was first introduced by Gumbel. Using annual peak flow data that is available for a number of years, flood frequency analysis is used to calculate statistical information such as mean, standard deviation and Skewness which is further used to create frequency distribution graphs. The best frequency distribution is chosen from the existing statistical distributions such as Gumbel, Normal, Log-normal, Ex-ponential, Weibul, Pearson and Log-Pearson. After choosing the probability distribution that best fits the annual maxima data, flood frequency curves are plotted. These graphs are then used to estimate the design flow values corresponding to specific return periods which can be used for hydrologic planning purposes. The goal of this study is to determine the best-fit probability distributions in the case of maximum annual rainfall using 48 years of data (1971–2018) of Indore and Mhow tehsils by using different statistical analysis and distribution types. Commonly used frequency distributions were applied. The expected frequency for normal, log normal & Gumbel has been calculated and probability plots have been plotted. The goodness of fit criterion has been tested by the Chi-Square test.
Related Papers
Hydrological Processes
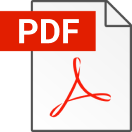
Advances in Urban Flood Management
Demetris Koutsoyiannis
Civil Engineering Journal (CivileJournal.org)
Of all natural disasters, floods have been considered to have the greatest potential damage. The magnitude of economic damages and number of people affected by flooding have recently increased globally due to climate change. This study was based on the establishment of a stochastic model for reducing economic floods risk in Yewa sub-basin, by fitting maximum annual instantaneous discharge into four probability distributions. Daily discharge of River Yewa gauged at Ijaka-Oke was used to establish a rating curve for the sub-basin, while return periods of instantaneous peak floods were computed using the Hazen plotting position. Flood magnitudes were found to increase with return periods based on Hazen plotting position. In order to ascertain the most suitable probability distribution for predicting design floods, the performance evaluation of the models using root mean square error was employed. In addition, the four probability models were subjected to goodness of fit test besed on Anderson-Darling (A 2) and Kolmogorov-Smirnov (KS). As a result of the diagnostics test the Weibul probability distribution was confirmed to fit well with the empirical data of the study area. The stochastic model generated from the Weibul probability distribution, could be used to enhance sustainable development by reducing economic flood damages in the sub-basin.
Hydrological Sciences Journal
Dr. Shakirudeen Odunuga , Adekunle S Raji
Abstract- Flood frequency analysis and inundation mapping of lower Ogun River basin was implemented using the Gumbel probability distribution method. This method was however tested with Log Pearson Type III to ascertain the best fitting statistical measure for hydrological fluxes using Chi Square. Basin delineation data was extracted from the topographic maps of Ilaro SE 1, 2, 3 & 4 which covers the study area while gauge height data at Adiyan intake was used as the principal data for flood frequency assessment and inundation modelling based on (Tr) return periods 2, 5, 10, 25, 50 and 100 years. The results showed modelled water level values of 2.22 metres, 2.24 metres, 2.28 metres, 2.38 metres, 2.55 metres, and 2.90 metres for each of the return periods. Consequent upon this, the inundated area is projected to increase to almost 30% of the area from an initial 23% for most of the scenarios. These findings provide clearer picture for the pattern of hydrological fluxes of the lower Ogun River Basin. Sustainable planning and developmental measures that consider the modelled pattern of hydrological fluxes of the study area were recommended for decision making on urban areas. Keywords- Flood estimation; Flood modelling, Floodplain; Gumbel distribution; Log Pearson Type III and Return period.
kalkidan mekonen
European Scientific Journal ESJ
In large watersheds of Pakistan, a proper flood intensity assessment is a most important concern for many water management applications. The present study investigates the possible best-fit probability distributions technique of uttermost flood events using more than 30 years data (except Mastuj Bridge station) from 15 stream flow stations in northern Pakistan, operated and maintained by Surface Water Hydrology Project (SWHP), WAPDA. Different statistical distribution model, were used to estimate design floods for risk planning and the design of important civil infrastructure. Method of moments was used for estimation of parameters of selective distributions. Four goodness-of-fit statistics test K, A2, χ2 and Root mean square error (RMSE) were applied to evaluate the most appropriate probability distribution. P3, LP3, GEV, and LN were determined best fit distribution model. Among the eight-probability distribution's P3 fitted 33.3% of the stations, LP3 also fitted 33.3% of the stations, GEV fitted 26.7% of the stations and LN fitted 6.7% of the stations. The more practical result for peak flood were calculated for recurrence intervals of 5-year, 25-year, 50-year, 100-year, 500-year and 1000-years respectively. In future before planning and designing any project such as Hydropower, irrigation systems, Dike, flood disaster assessment and management at Indus and adjacent rivers, P3, LP3, GEV and LN distributions results should be considered more reliable on these locations.
Muhammad Faisal , Tanveer Abbas
Floods are the number-one natural disaster in most of the countries of the world in terms of number of lives lost and property damage. They play overwhelming destruction and at some point caused untold torments like death toll, disturbance of human exercises, and harm to property, rural yields and health dangers. Flood frequency analysis of Nullah Deg was employed using the Gumbel distribution which is one of the probability distribution used to model stream flow. This was required by the need to secure lives and property at the downstream of the catchment zone. This distribution was however verified with Log-Pearson III distribution to establish the best fitting statistical measure for hydrological fluctuations using chi square test. Peak flow data from 1982-2014 of Nullah Deg was used for frequency analysis based on return periods of 2, 5, 10, 25, 50, 100, 200 and 1000 years. Gumbel probability distribution gave best result according to chi square test. From the trend line equation, R2 gives a value of 0.573 which shows that Gumbel’s distribution is suitable for predicting expected flow in the Nullah. For the given return periods following were the estimated flows using Gumbel distribution 403 m3/s, 1026 m3/s, 1344 m 3 /s, 1591 m3/s, 1824 m3/s, 2056 m3/s and 2602 m3/s respectively. These values are useful for storm management in the area. Flow trends of Nullah Deg were checked using the discharge data of Monsoon seasons of high flow years from 2010-2014. Flow trend graphs showed that august was the highest peak flows month of Monsoon seasons. This study concluded that in Nullah Deg flood danger is always in the Monsoon season and month of august is more danger for floods. Finally frequency analysis and trend analysis were estimated and conclusions were commented.
Efi Foufoula-georgiou
Loading Preview
Sorry, preview is currently unavailable. You can download the paper by clicking the button above.
RELATED PAPERS
nor eliza alias
Samiul Islam
Journal of Hydrologic Engineering
Veronica Webster
Richard Vogel , Ellen Douglas
Lunamoni Das
anil shirgire
Journal of Climate
Barry D. Keim
Uttam Puri Goswami
Gülay Onuşluel Gül
Gerardo Benito
Deriving Design Flood Hydrographs Based on Copula Function: A Case Study in Pakistan
muhammad rizwan
nigatu sugamo
Juan Piedra Espinosa
Geomorphology
Paul Carling
risorseidriche.dica.unict.it
Blanca Botero
eSAT Journals
etd.aau.edu.et
Aydagne Zelleke
Recep Yurtal
Evaluation of Various Probability Distributions for Deriving Design Flood Featuring Right-Tail Events in Pakistan
Journal of science, Engineering and Technology (Zimbabwe Institution of Engineers)
Lovemore Mamombe , Tumbare, Michael James
Murat Çobaner
Prof. Asit K . Biswas
Advances in Water Resources
Maria Cristina Rulli
Water Resources Management
Poulomi Ganguli
Nicolas Dalezios
MD SHAHID LATIF
Joel A C Baum
Éditions Quae
Theoretical and Applied Climatology
Marcin Wdowikowski
Lina Castro
Mohamed Salem
krishna Rijal
Nature Scientific Reports
Romy Schlögel
Natural Hazards
Ousmane Seidou
International Journal of Constructive Research in Civil Engineering (IJCRCE)
Kaveh Ostad-Ali-Askari
International Journal of Engineering Research and Technology (IJERT)
IJERT Journal
RELATED TOPICS
- We're Hiring!
- Help Center
- Find new research papers in:
- Health Sciences
- Earth Sciences
- Cognitive Science
- Mathematics
- Computer Science
- Academia ©2024
- DOI: 10.11648/J.WROS.20170604.11
- Corpus ID: 55585031
Flood Frequency Analysis Using Gumbel's Distribution Method: A Case Study of Lower Mahi Basin, India
- Published 4 July 2017
- Environmental Science, Engineering
Figures and Tables from this paper
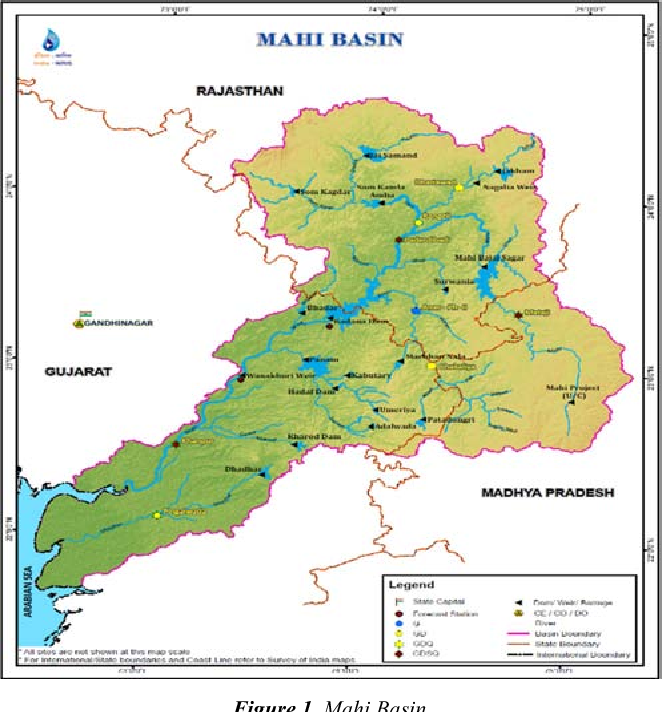
51 Citations
Flood frequency analysis of jamsholaghat sub- basin using three methods and its comparison, comparative analysis of flood estimation using log-pearson type iii and gumbel max models in the cauvery river, india, determination of river design discharge (tar river case study), inundation mapping and flood frequency analysis using hec-ras hydraulic model and easyfit software, at site flood frequency analysis of baitarani river at champua watershed, odisha, evaluating flood frequency analysis techniques: performance analysis of log pearson type iii, gumbel's, and log normal methods for stream discharge estimation, flood frequency analysis using gumbel distribution equation in part of port harcourt metropolis, flood frequency analysis for burhi gandak river basin, flood frequency analysis of jiadhal river basin, india using log pearson type iii distribution method, assessment of flood frequency using statistical and hybrid neural network method: mahanadi river basin, india, 11 references, flood frequency analysis of osse river using gumbel’s distribution, flood frequency analysis using the gumbel distribution, flood frequency analysis of ikpoba river catchment at benin city using log pearson type iii distribution, flood frequency analysis of annual maximum stream flows using l-moments and tl-moments approach, flood-frequency prediction methods for unregulated streams of tennessee, 2000, mitigating floods in southern africa, statistical methods in hydrology, hydrology in practice, theoretical probability distributions for flood peaks, frequency analysis of extreme events, related papers.
Showing 1 through 3 of 0 Related Papers
Thank you for visiting nature.com. You are using a browser version with limited support for CSS. To obtain the best experience, we recommend you use a more up to date browser (or turn off compatibility mode in Internet Explorer). In the meantime, to ensure continued support, we are displaying the site without styles and JavaScript.
- View all journals
- Explore content
- About the journal
- Publish with us
- Sign up for alerts
- Open access
- Published: 02 September 2024
Analysis of the frequency–response stability and reliability of a tower-column unstable rock mass on a high and steep slope
- Fuchuan Zhou 1 , 2 ,
- Xinrong Liu 1 ,
- Hongmei Tang 3 ,
- Mengqi Miao 4 ,
- Bin Xu 5 &
- Xueyan Guo 1
Scientific Reports volume 14 , Article number: 20308 ( 2024 ) Cite this article
Metrics details
- Civil engineering
- Natural hazards
The first-order second-moment checking point method was introduced to judge the instability probability and evaluate the stability of the TCURM. The frequency–response stability calculation and the reliability results were compared, and a frequency–response stability and reliability analysis method was proposed. Taking the Zengziyan W12 # unstable rock mass in Nanchuan, Chongqing, China, as an example, the calculation shows that the dynamic indexes and geological indexes decrease as the stiffness of the deterioration area decreases. According to the statistical data of the laboratory test and the field investigation, reliability theory is used to evaluate the stability of the TCURM, and the failure probabilities are 80.3% and 96.27% under natural and saturated conditions, which correspond to states of poor stability and instability, respectively. The reliability evaluation results are consistent with the conclusion of the frequency–response stability analysis. The new method can provide a theoretical basis for developing the dynamic monitoring and early warning indicators of the TCURM and disaster prevention and mitigation in mountainous areas.
Similar content being viewed by others
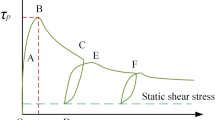
Dynamic stability analysis method of anchored rocky slope considering seismic deterioration effect
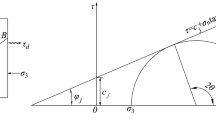
Experimental and constitutive model study on the mechanical properties of a structural plane of a rock mass under dynamic disturbance
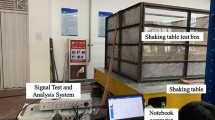
Shaking table test on damage mechanism of bedrock and overburden layer slope based on the time–frequency analysis method
Introduction.
Rock mass collapse usually occurs suddenly. The slope's surface displacement occurs after the formation of the internal fracture of the rock mass. However, monitoring points distributed on the slope's surface or geophysical exploration inside the slope are restricted by the landform, hydrogeological conditions, drilling risk, and cost, resulting in the difficulty of short-term or real-time prediction of collapse disasters. Therefore, it is urgent to study the dynamic parameters that reflect the current characteristics of unstable rock mass 1 . Using vibration parameters to characterize the precursor of collapse is an effective means to identify and monitor unstable rock masses 2 , 3 ; it has apparent advantages in rock mass stability analysis, damage identification, and strength calculation 4 . The natural frequency of the unstable rock mass is an inherent attribute. Various damage mechanisms can reduce the stiffness, natural frequency, and stability of unstable rock masses 5 . Therefore, we can effectively avoid the negative impact of collapse disasters on all kinds of residential buildings and infrastructure by monitoring the natural vibration frequency index, which can be applied to analyze the real-time stability of a tower-column unstable rock mass (TCURM), dynamically predict the instability time, and issue early warning information in a timely manner.
The structure's mass and stiffness influence the natural modal parameters such as the vibration mode and frequency. The deterioration of the structure leads to a decrease in stiffness and then changes the modal parameters 6 . The natural vibration frequency used to predict rock mass collapse has been reported for decades. For example, Levy 7 used seismometers and extensometers to detect the first resonance frequency of the limestone column at the Chamousset site and compared it with finite element model results; Moore 8 , 9 employed ambient resonance measurements of a freestanding rock to measure the elastic modulus of in situ rock masses and identified the first resonant modes of a 120-m high freestanding rock monolith. Valentin 3 found the rock column slenderness influences the resonance frequency because the slenderness will change the mass and rigidity of the rock column.
Contact monitoring methods for obtaining the natural vibration frequency of structures require external force excitation and pulsation. They require a considerable input excitation energy or high instrument accuracy to capture frequency response signals, so their application in large rock slopes is limited. In recent years, laser Doppler vibrometry (LDV) has used optical principles and noncontact nondestructive monitoring modes to obtain the natural vibration frequency of unstable rock masses. It gradually showed advantages in the dynamic monitoring of high-level and large-scale high and steep rock slopes 10 . For example, Siringoringo and Fujino 11 introduced the principle and algorithm of LDV to obtain modal parameters. It has significant advantages over the traditional artificial excitation method in any working conditions, including all weather conditions.
Unstable rock masses are primarily developed with cliff landforms, controlled by a deep and sizeable structural plane with a thin, high shape and free surface. Suppose the size of a rock mass in the vertical Z direction is much larger than that in the two horizontal X and Y directions (10 times or more) and the size ratio in the X and Y directions is less than or equal to 4. Such a rock mass can be defined as a tower-column unstable rock mass 12 . The stiffness of a TCURM has spatial variability because of the nonuniform deterioration of the rock mass. Key blocks control the instability mode, but dynamic models and theoretical research have not been reported 13 . In addition, the uniaxial compressive strength criterion is usually used to analyze the stability of a tower-column unstable rock mass. The compressive strength of the rock mass is related to the integrity of the rock mass, and the acoustic velocity measurement obtains the integrity coefficient of the rock mass. With this method, water is injected into the borehole, and the vibration signal in the adjacent borehole is captured by applying excitation on the surface. However, the cross-hole acoustic velocity measurement test has application limitations in the TCURM on a cliff 14 . Therefore, a new method for obtaining the compressive strength of the TCURM on steep and high slopes can be explored in combination with the self-resonance frequency monitoring index to reduce exploration risks and economic costs.
There are many uncertainties in the geotechnical parameters, such as geological model, frequency, strength, and persistence of the influential factors used for the unstable rock slope stability analysis, so the traditional deterministic analysis method has some limitations 15 . Some scholars have introduced the reliability analysis methods to calculate the instability probability of unstable rock collapse and slope instability, such as a plain failure mode in the jointed rock mass 17 , 18 , a rock wedge stability analysis 19 , 20 , a toppling failure mode in an unstable rock mass 21 . However, the reliability analysis method has not been used to evaluate the instability probability of the fracturing-induced failure mode of a TCURM. Additionally, the reliability analysis is different from the traditional deterministic stability analysis method, so these approaches can mutually verify the unstable rock stability evaluation results.
In conclusion, due to the quality difference in rock mass between the top and the bottom of a TCURM, a TCURM can be divided into the incomplete deterioration area in the middle and upper parts of the rock mass and the deterioration area at the bottom. Then, a two-degree-of-freedom vibration mechanics model can be established. The natural vibration frequency (fundamental frequency) used for stability analysis, instability prediction and early warning of the unstable rock mass is explored. A new method for obtaining the compressive strength of a rock mass suitable for a limestone area is proposed. At the same time, the optimized first-order second-moment method (FOSM) is proposed to analyze the instability probability of the TCURM. The FOSM can be used to verify the rationality of the frequency–response stability analysis method (FRSA). Furthermore, we creatively combine the FOSM and the FRSA to obtain a frequency–response reliability stability analysis method (FRRSA) which can provide a theoretical basis for the dynamic monitoring and early warning indicators for the TCURM disaster prevention and mitigation.
Geomorphic evolution and dynamic monitoring stage
From formation (birth) to collapse and accumulation (death), a TCURM, with a gentle dip, has a geomorphic evolution process with periodic characteristics 22 , 23 . Figure 1 shows the process: (a) Initial formation stage: Due to the intermittent motion and uplift of the Earth's crust, the river exposes soft-hard interbedded strata, which forms a steep and high slope. The tensile stress concentrates on the crest of the slope, resulting in unloading tensile cracks. The soft-hard interbedded strata at the toe of the slope undergo differential unloading rebound deformation and bear the weight of the upper rock mass, resulting in both the unloading tensile cracks and the compression-induced tensile cracks (like the vertical tensile cracks formed under the axial load in the unconfined compressive strength test) near the base interface. (b) The formation stage of the control fissure: The unloading crack at the crest of the slope extends downward, and the compression-induced tensile crack at the toe of the slope propagates upward. (c) Differential deterioration stage of the rock mass: The control fissure separates the unstable rock mass from the parent rock. Under the freeze‒thaw action, dissolution, water-induced weakening, and a self-weight load of the upper part of the unstable rock mass, with the further development of dissolution holes and fissures, the compression-induced tensile cracks continue to develop, and the rock mass strength decreases continuously. (d) Deterioration aggravation stage: When the rock mass quality in the deterioration area at the bottom of the TCURM decreases to a threshold value, sudden fracturing and ejection of the detached rock will occur, and the TCURM behavior transforms from static instability to a dynamic process. (e) Slumping fragmentation stage: The incomplete deterioration area in the upper part of the TCURM impacts the base at a specific speed and disintegrates continuously under the strong shock wave in the slumping period. (f) Accumulation stage: The broken stones move along the valley and accumulate along the route.
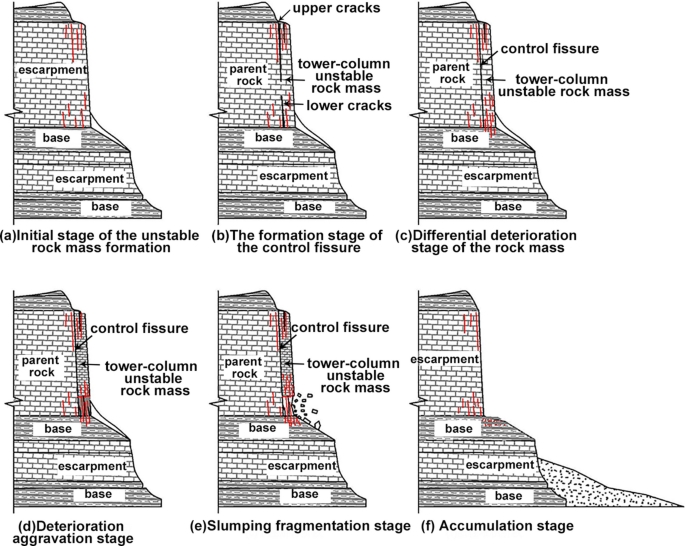
Evolution process of the tower-column unstable rock mass.
As the deterioration aggravation stage corresponds to the critical instability state, dynamic monitoring instruments can obtain data with high efficiency and high frequency; engineers can analyze and obtain feedback in time, and the management department can effectively save monitoring costs and improve decision-making accuracy. Therefore, the dynamic monitoring and early warning period should be performed in the deterioration aggravation stage in Fig. 1 d. According to the on-site geological experience and the theoretical damage-catastrophe calculation results in the deterioration area, we can judge whether critical instability starts at the deterioration aggravation stage.
At present, relevant dynamic monitoring instruments have appeared on the market. However, the vibration mechanics model and the relevant theory still need improvement. Therefore, we discuss the vibration mechanics model below.
Frequency–response stability analysis method
A two-degree-of-freedom vibration mechanical model.
The TCURM, with a triangular prism shape and a gentle stratum dip, is located on the second Zengziyan cliff in the Nanchuan District of Chongqing. The second cliff consists of limestone from Groups 3–5 of the lower Permian Maokou Formation (P 1 m 3+4+5 ) and shale with limestone in Group 2 of the lower Permian Maokou Formation (P 1 m 2 ). The first cliff comprises limestone of Group 1 of the lower Permian Maokou Formation (P 1 m 1 ) and the lower Permian Qixia Formation (P 1 q). Three groups of control fissures are developed in the unstable rock mass, of which two groups are vertical and orthogonal unloading fractures, which propagate from top to bottom, with an extended length of approximately 200 m and a fracture width of 3 ~ 10 m. The other group is in the near-horizontal orientation (Fig. 2 ).
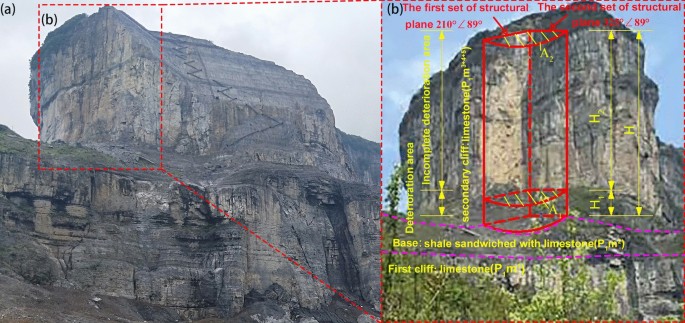
Photographs of the TCURM after collapse of the Zengziyan cliff; The submap ( a ) is the status of the Zengziyan cliff. The submap ( b ) is the restored TCURM which shows the geometric information and the strata.
As usual, the free-standing column or slab has a toppling failure mode, but the Zengziyan cliff rarely shows a special collapse mode. The TCURM collapses vertically, accompanied by fragmentation during the slumping process. The survey results demonstrate that the bottom deterioration area is a water accumulation and circulation area. The stress concentrates, and the rock mass strength decreases most due to a significant dissolution phenomenon. Under the self-weight load of the rock mass in the upper incomplete deterioration area, the rock mass in the deterioration area appears to fracture, which corresponds to a compression-induced instability initiation mechanism at the bottom of the unstable rock mass 24 .
Two groups of vertical unloading fissures cut and separate the TCURM from the parent rock, as shown in the photographs in Fig. 3 a,b. The damage and deterioration degree at the bottom of the unstable rock mass becomes worse when undergoing various geological forces, such as self-weight, weathering, and dissolution. Thus, the TCURM consists of an upper incomplete deterioration area and a lower complete deterioration area from top to bottom. The binary system in Fig. 3 b is selected as the research object.

The generalized process of mechanical model of the TCURM; The submap ( a ) is the generalized model of the cliff; The submap ( b ) is the tower column unstable rock mass; The submap ( c ) is the two-degree-of-freedom vibration mechanical model of the TCURM; The submap ( d ) is the mechanics analysis of the tower column unstable rock mass.
The following is the purpose and procedure for constructing a two-degree-of-freedom vibration mechanics model. The first step is to construct a two DOF model representing the TCURM. Secondly, it’s easy to get the theoretical dynamic response parameters such as the natural frequency which can reflect the deterioration degree of the rock in the bottom of the TCURM. Thirdly, it points out where and which parameter to measure according to the analysis of the two DOF model of the TCURM. So the two DOF model in Fig. 3 is the theoretical foundation for analysis of the TCURM. Furthermore, the reduction of the natural frequency response will reflect the stability state of the TCURM.
Based on the purpose, A two-degree-of-freedom vibration mechanics model is obtained by applying the mass concentration method of vibration mechanics (Fig. 3 c). The upper incomplete deterioration area is simplified as particle m 2 , whose vibration displacement under dead weight load m 2 g is u 2 . The lower deterioration area is simplified as particle m 1 , whose vibration displacement under dead weight load m 1 g is u 1 . Spring k 2 connects the two particles, and spring k 1 connects particle m 1 with the base. The force on the two particles is shown in Fig. 3 d.
We assume that (1) the geometric parameters such as the height H 1 and horizontal cross-sectional area A 1 of the deterioration area and the height H 2 and horizontal cross-sectional area A 2 of the incomplete deterioration area remain unchanged (Fig. 2 b). (2) In a simple harmonic vibration process, the mass of the two particles, m 1 and m 2 , is constant, and the system meets the deformation synchronization principle. (3) The rock at the bottom is deteriorating, therefore, the stiffness coefficient k 1 of the bottom should be a decreasing function of time and the variation of k 1 would cause the motion of the system. The rock in the upper portion is a non-deterioration area where the stiffness coefficient k 2 should keep the same in a long time.
According to Fig. 3 d, the motion equation in matrix form is established for the two-degree-of-freedom undamped vibration system by using the D'Alembert principle, as shown in Eq. ( 1 ):
where \(\mathop {u_{1} }\limits^{..}\) is the acceleration of particle m 1 (m/s 2 ) and \(\mathop {u_{2} }\limits^{..}\) is the acceleration of particle m 2 (m/s 2 ). The meanings of the other symbols are the same as before.
where k i is the stiffness coefficient of the i th spring (N/m), and the subscript i is either 1 or 2; E i , A i , and H i are the elastic modulus ( MPa), the sectional area (m 2 ), and the height (m) of the i th rock mass, respectively; i = 1 represents the simplified particle in the bottom deterioration area of the rock mass; i = 2 represents the simplified particle in the incomplete deterioration area in the middle and upper part of the rock mass.
Suppose that Eq. ( 1 ) has a simple harmonic solution of displacement, as shown in Eq. ( 3 ):
where φ 1 and φ 2 are the particle amplitudes (m), ω i s the circular frequency (Hz), and θ i s the initial phase.
Substituting Eq. ( 3 ) into Eq. ( 1 ), the homogeneous characteristic equation can be obtained, as shown in Eq. ( 4 ):
The necessary and sufficient condition for the existence of a nonzero solution of the vibration displacement in the two-degree-of-freedom system is that the coefficient determinant of Eq. ( 4 ) is zero, that is,
By solving the frequency Eq. ( 5 ), the frequencies of each order of the two-degree-of-freedom system can be obtained, as shown in Eqs. ( 6 ) and ( 7 ):
where ω 1 is the first-order circular frequency (Hz) and ω 2 is the second-order circular frequency (Hz). The meanings of the other symbols are the same as before.
ω 1 and ω 2 are related to the mass distribution and the stiffness of the system and have nothing to do with the cause of vibration, so they are the system’s inherent attributes. Equations ( 8 ) and ( 9 ) show the corresponding first-order natural frequency f 1t and the second-order natural frequency f 2t :
In theory, a laser Doppler vibrometer can monitor the natural frequency of a TCURM, and the change in the natural frequency can reflect the deterioration degree of the binary system. With the decrease in stiffness, the natural frequency of the unstable rock mass decreases, and the rock mass’s deterioration degree worsens.
Frequency–response reduction coefficient
At the initial formation stage of the unstable rock mass, there is no apparent difference in the physical and mechanical indices of the rock mass with the same lithology, and the dynamic characteristics are almost the same. With the effects of unloading rebounding, weathering, root splitting, self-weight loading, and other factors, the different parts of the TCURM show different deterioration trends, the deterioration degree of the bottom is much worse than that of the top, and the dynamic parameters decrease significantly. The first-order natural frequency, which is a primary vibration frequency–response index, is the fundamental frequency, with the lowest vibration frequency and the most prolonged vibration period. Therefore, the fundamental frequency (the first-order natural frequency) is chosen as the primary vibration monitoring index of the TCURM. Obviously, over time, the rock mass strength deteriorates continuously, and the fundamental frequency f 1 , which is a function of time t, decreases accordingly. Based on this, the frequency–response reduction coefficient D t of the TCURM at any time t is defined, as shown in Eq. ( 10 ) 4 :
where f 1t is the fundamental frequency monitoring index at time t , (Hz), and f 1 ( t 0 ) is the fundamental frequency at the initial time of t 0 , (Hz). At the initial formation time of the unstable rock mass, the difference in the rock mass’s physical and mechanical indices in different parts is non-significant. The elastic modulus E i can be obtained from the slightly weathered rock when the fundamental frequency at the initial time is calculated by applying Eqs. ( 2 ) and ( 8 ), (MPa).
The rock mass damage factor D e1 which reflects the proportion of defects in a particular state is introduced to represent the damage and deterioration degree of the rock mass through the decrease in the fundamental frequency, as shown in Eq. ( 11 ) 22 :
Frequency–response stability coefficient
The mechanical model of the TCURM is similar to a large-scale uniaxial compression test 24 , 25 , and the rock mass stability can be approximately judged according to the uniaxial compressive strength criterion. The stability coefficient is shown in Eq. ( 12 ) 26 :
where [ σ b ] is the uniaxial compressive strength (UCS) of the rock mass (MPa). σ is the compressive stress on the top surface of the rock mass deterioration area (MPa).
The UCS [ σ b ] of the rock mass in the limestone area is generally obtained by the uniaxial compressive strength of the indoor rock test, which is reduced according to engineering experience. The reduction coefficient is related to the lithology, complexity, structural plane development, and engineering experience. It lacks a theoretical basis and appears to have apparent subjectivity. The quantitative equivalent acquisition method of the rock mass compressive strength is introduced in detail below.
The combination of the rock mass basic quality (BQ) classification, the rock mass rating (RMR) classification, and the geological strength index (GSI) system can provide a theoretical method for quantitative reduction of the rock mass compressive strength, such as Eqs. ( 13 )–( 17 ) 14 :
where K v is the integrity coefficient of the rock mass, which is obtained by the acoustic velocity measurement method; R c is the uniaxial compressive strength (UCS) of the rock (MPa); GSI is the geological intensity index 27 in the Hoek‒Brown criterion (H-B criterion); η is the disturbance factor between [0,1], and 0 is taken when there is no apparent artificial disturbance; m i is the material constant of the intact rock, and the limestone is taken as 7; m b, s and a are the modified generalized Hoek‒Brown parameters; and σ 3 is the minor principal stress (MPa), which is 0 under uniaxial stress. The meanings of other parameters are the same as before.
Equation ( 17 ) fits the gentle slope terrain where drilling rigs are easily erected, such as the slope of the reservoir bank. When there is a reliable water source, an exploration test can be carried out to obtain the rock integrity coefficient K v and calculate the GSI and the UCS. However, for the TCURM on a large steep and high slope, the toe of a cliff is the crucial part that controls the overall stability of the unstable rock mass. Conventional drilling methods have disadvantages, such as deep working depths, numerous drilling holes, high capital costs, low work efficiency, potential limestone leakage, and drilling damage. Therefore, obtaining the integrity coefficient K v by the acoustic velocity measurement method is difficult.
In order to solve the above problem, the dynamic index (the integrity coefficient K v ) should be replaced equivalently. As we know, the better the rock mass integrity is, the faster the acoustic wave's transmission speed (the acoustic velocity) is and the higher the fundamental frequency is. Therefore, it is assumed that for the same rock mass, the above two dynamic indices (the integrity coefficient and the fundamental frequency) may describe the deterioration degree of the rock mass, both of which must have a specific scientific internal relationship.
The literature 14 defines the deterioration degree of the rock mass as a damage factor D e2 = 1- kV . Theoretically, the deterioration degree of the same rock mass at the same time is an objective attribute, and the damage factor obtained by different methods should be the same; that is, the damage factor D e2 obtained by the acoustic velocity measurement method and the damage factor D e1 obtained by the fundamental frequency monitoring method should be equal. Combined with Eq. ( 11 ), let D e1 = D e2 ; then, K v = D t .
Therefore, the integrity coefficient K v of the rock mass in Eq. ( 17 ) can be replaced by the frequency–response reduction coefficient D ( t ) of Eq. ( 10 ), as shown in Eq. ( 18 ):
The frequency–response stability coefficient of the rock mass can be obtained by the following steps: First, we can obtain the frequency–response reduction coefficient D t according to Eq. ( 10 ) by obtaining the fundamental frequency of the TCURM measured at different times. Second, the frequency response reduction coefficient D t is inserted into Eq. ( 18 ) to obtain the geological strength index (GSI). Third, it's easy to get the compressive strength [ σ b ] based on Eqs. ( 13 )–( 16 ) and ( 18 ). Fourth, by combining the compressive strength [ σ b ] (Eq. ( 13 )) with the stability coefficient F s (Eq. ( 12 )), we can obtain the frequency response stability coefficient F s d . Fifth, the variation of the frequency–response stability coefficient with time can be revealed to estimate the instability of the TCURM by measuring the fundamental frequency of the TCURM.
In addition, due to the lack of a stability classification standard for the compression-induced instability mode of the TCURM at present, the instability analysis can be implemented by referring to the relevant provisions on the evaluation and prediction of the unstable rock mass collapse in the ‘Exploration Code for Geological Disaster Prevention and Control Engineering (DB50/T 143–2018)’.
The frequency–response stability analysis method proposed above is a deterministic analysis method. Due to many uncertainties, such as the geomechanical model, which is difficult to reflect the complexity in nature, and the significant spatial variation of physical and mechanical parameters, reliability theory can verify the rationality of the proposed deterministic analysis method 1 , 4 , 5 . Meanwhile, by combining the deterministic analysis method with the reliability theory, a non-deterministic frequency–response stability reliability analysis method of the TCURM can be obtained. Therefore, the reliability theory is introduced below.
Reliability analysis method
The first-order reliability method (FORM) is one of the most commonly used calculation methods in rock slope stability analysis and includes the first-order second-moment method (FOSM) and the advanced second-moment method (ASMM) 28 . This study intends to combine the frequency–response stability calculation method with the reliability method. First, the performance function (Eq. ( 19 )) can be constructed according to the frequency–response stability coefficient of the TCURM (Eq. ( 12 )). Second, the advanced second-moment method (ASMM) is used to evaluate the reliability of the TCURM stability.
We make two assumptions: (1) The confining pressure σ 3 in the Hoek‒Brown criterion and the blasting disturbance factor D are not considered under the uniaxial compression condition. (2) The rock uniaxial compressive strength R c , the GSI, and the H-B parameters ( s and a ) follow the standard normal distribution.
Based on the above hypothesis, the instability performance function g ( X ) and the state criterion of the TCURM are established, as shown in Eq. ( 19 ):
where X = [ X 1 , X 2 , …, X n ] represents the random variable, and the random variable of the TCURM based on the H-B criterion is X = [ R c , s , a ].
According to the ASMM, the mean value μ z , the standard deviation σ z , the reliability index β , the sensitivity coefficient α X i , the extreme point x i *, and the failure probability P f of the performance function are obtained, as shown in Eqs. ( 20 )-( 25 ) 29 :
where X i is a vector including the random variables R c , s , and a ; x i * represents the extreme point of the random variable when the performance function is in a limit state; Φ(·) is the standard normal distribution function; μ x i represents the mean value of the random variable x i ; and σ Xi represents the standard deviation of the random variable x i .
After obtaining the unstable rock mass’s failure probability P f , we can evaluate the stability state of the TCURM based on the stability evaluation criteria (Table 1 ) provided by the literature 21 .
It should be noted that the stability reliability of the TCURM, calculated with engineering test parameters and field investigation data, is not limited to verifying the frequency–response stability analysis method proposed in this study. Obviously, combining the deterministic analysis method and the nondeterministic stability analysis method to obtain the TCURM frequency–response stability reliability analysis method has a more significant engineering application value than each method alone. The specific steps are shown in.
The geometric and physical parameters are determined from the literature 12 and the "Construction drawing of the unstable rock mass numbered W29 # and W23 # treatment project of Jinfoshan, Nanchuan District, Chongqing" report. The height H 1 of the deterioration area of the W12 # unstable rock mass is 48 m, the height H 2 of the incomplete deterioration area is 192 m, the cross-sectional area is approximately 804.25 m 2 , and the limestone unit weight is 26.5 kN/m 3 . The elastic modulus of the moderately weathered limestone is 43.68 GPa, the elastic modulus of the slightly weathered limestone is 62.4 GPa, the natural uniaxial compressive strength of the rock is 51.17 MPa, the saturated uniaxial compressive strength of the rock is 42.07 MPa, and the compressive stress σ on the deterioration area's top surface is 5.088 MPa (Fig. 4 ).

The flowchart of the frequency–response reliability stability method.
The deterioration law of the stiffness coefficient by the time
A large number of limestone rocks in the deterioration area of the W12 # unstable rock mass were taken by us to conduct some indoor experiments to see how the strength of the rock varies with time in the study (Fig. 5 ) 30 . We analyzed and obtained the stiffness coefficient results in Fig. 6 .
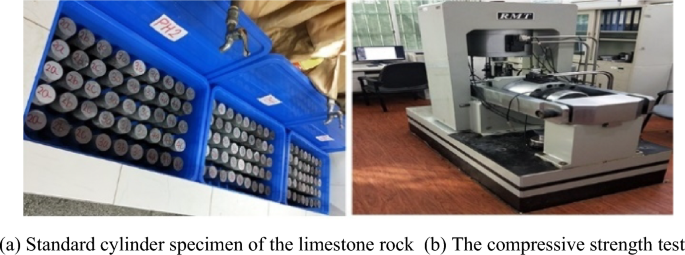
The compressive strength test in different acidity and erosion time. ( a ) Standard cylinder specimen of the limestone rock ( b ) The compressive strength test.
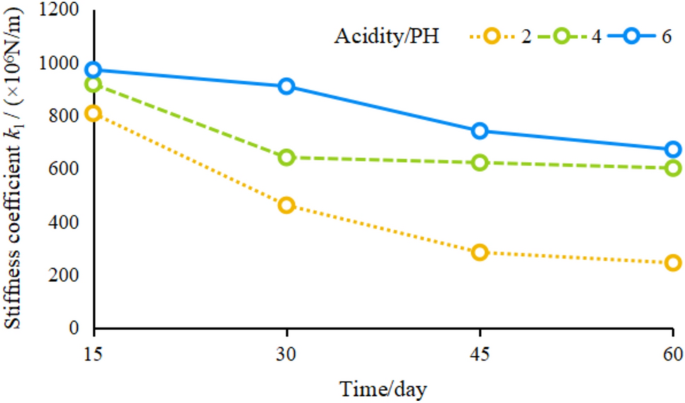
The deterioration law of the stiffness coefficient by the time in different acid condition.
It shows that the stiffness coefficient decreases with time in any acidic environment. The stiffness coefficient decreases from 971.29 × 10 6 N/m to 671.2 × 10 6 N/m when the PH is 6, and the stiffness coefficient decreases dramatically from 807.5 × 10 6 N/m to 244.24 × 10 6 N/m when the PH is 2. Therefore, it can be proved that the assumption that the stiffness coefficient decreases with time in “A two-degree-of-freedom vibration mechanical model” is justified.
Sensitivity analysis of the physical parameters
Since the physical properties of the rock mass in the deterioration area are weaker than those in the incomplete deterioration area, the elastic modulus of the moderately weathered limestone is assigned to the deterioration area's rock mass to calculate the stiffness coefficient k 1 ; the elastic modulus of the slightly weathered limestone is assigned to the incomplete deterioration area to calculate the stiffness coefficient k 2 . The following section studies the sensitivity of each physical parameter to the decrease in the stiffness coefficient k 1 of the deterioration area.
According to Eqs. ( 2 ), ( 8 ), ( 10 ), ( 12 ), ( 13 ), and ( 18 ), the deterioration law of each physical parameter with the deterioration area's stiffness coefficient k 1 is obtained by MATLAB programming, as shown in Fig. 7 . For example, the stiff coefficient k 1 and k 2 will be obtained by the basic data of the case. Then suppose the stiff coefficient k 2 which reflects the condition of the incomplete deterioration area will not change and the stiff coefficient k 1 which represents the condition of the complete deterioration area will decrease significantly with time. If the stiff coefficient k 1 reaches 3 × 10 11 N/m, which shall be put into Eq. ( 1 ), the fundamental frequency f 1 will be 2.85Hz.
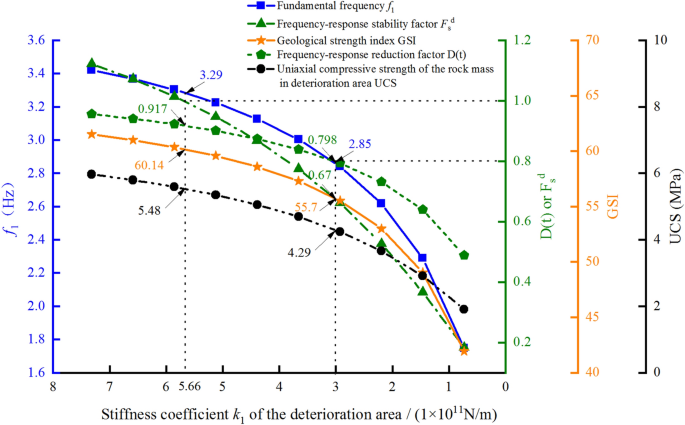
The variation in physical parameters by the change in the deterioration stiffness.
It can be seen from Fig. 7 that (1) the fundamental frequency f 1 decreases with decreasing stiffness coefficient k 1 in the deterioration area. The fundamental frequency monitoring data can reflect the rock mass’s internal deterioration. The fundamental frequency decreases from 3.42 Hz to 1.75 Hz, with an absolute decrease of 1.67 Hz and a relative decrease of 48.8%. When the fundamental frequency is larger than 3.29 Hz, the TCURM does not reach the instability stage.
(2) The frequency–response stability coefficient ( F s d ), the geological strength index (GSI), the frequency–response reduction coefficient ( D t ), and the uniaxial compressive strength [ σ b ] of the deterioration area's rock mass all show a nonlinear decreasing trend as the deterioration area's stiffness coefficient decreases, and the rate of decline accelerates. When the stiffness coefficient k 1 of the deterioration area is larger than 3 × 10 11 N/m, each parameter curve changes approximately linearly. When the stiffness coefficient k 1 of the deterioration area is less than 3 × 10 11 N/m, each curve has a steep drop. When the stiffness coefficient k 1 of the deterioration area is 3 × 10 11 N/m, the TCURM reaches the mutation point of compression-induced instability. The GSI at the mutation point is 55.7, the fundamental frequency f 1 is 2.85 Hz, the frequency–response reduction coefficient D t is 0.798 Hz, and the UCS [ σ b ] of the rock mass in the deterioration area is 4.29 MPa.
(3) When the frequency–response stability coefficient F s d is reduced to the critical state value of 1.0, the deterioration area stiffness coefficient is reduced to 5.66 × 10 11 N/m, GSI is 60.14, fundamental frequency f 1 is 3.29 Hz, frequency–response reduction coefficient D t is 0.917, and [ σ b ] of the rock mass in the deterioration area is 5.48 MPa.
According to the acoustic emission test data of the Zengziyan limestone specimen in the literature 31 , when the damage degree reaches 0.2, the total damage degree curve increases sharply to approximately 0.7, and the peak strength of the rock specimen decreases sharply from approximately 126 MPa to a residual strength of approximately 40 MPa (Fig. 8 ). In Fig. 8 , when the frequency–response reduction coefficient D t is 0.798, the corresponding rock mass’s damage factor D e1 is 0.202 in combination with Eq. ( 11 ), which is the same as the damage degree in the above literature. The frequency–response reduction coefficient and the damage factor based on the acoustic emission theory can characterize the damage degree of the rock mass.
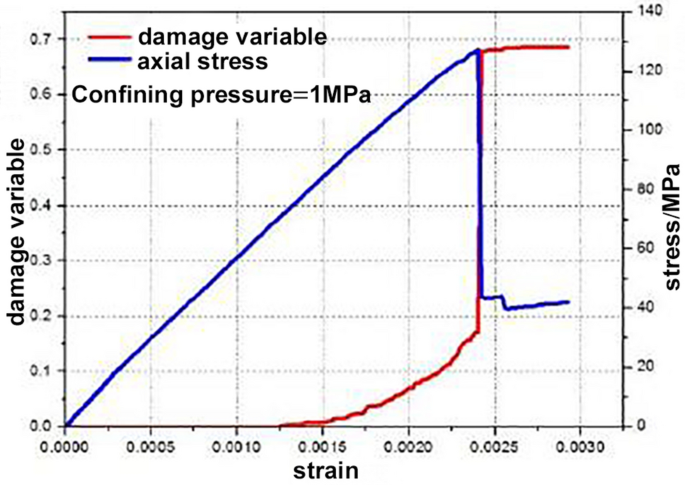
Constitutive curves and damage degree evolution of the rock specimen under the compressive acoustic emission test 31 .
We combine the GSI value of 55.7 at the mutation point in Fig. 4 with Eqs. ( 13 )–( 16 ), and the peak compressive strength data of rock samples presented in the literature 31 are used to determine a rock mass compressive strength of approximately 8.83 MPa, which is different from the rock mass compressive strength of 4.29 MPa under uniaxial compression calculated in this study. The reason for this difference is that the confining pressure corresponding to the peak compressive strength in the literature 31 is 1 MPa, which makes the modified compressive strength of the rock mass higher than the uniaxial compressive strength of the rock mass in the deterioration area calculated theoretically in this study. On-site, the TCURM is separated from the parent rock, resulting in no confining pressure. Therefore, using the rock's uniaxial compressive strength is more convincing to modify the rock mass's compressive strength.
We collected rock samples at the W12 # unstable rock mass collapse site and performed rock uniaxial compression testing with an RMT150C-type rock and concrete pressure machine (Fig. 9 ). The rock's uniaxial compressive strength of 72.25 MPa was modified to the rock mass's uniaxial compressive strength of 5.06 MPa in the deterioration area, combined with the GSI value of 55.7 selected from the mutation point in Fig. 7 and Eqs. ( 13 )–( 16 ) of the H-B criterion. This is 0.77 MPa larger than the UCS of 4.29 MPa calculated from the engineering test data (the rock's natural UCS is 51.17 MPa). The reason is that when engineers plan for engineering reinforcement design, the rock test data are first reduced according to engineering experience and then revised again according to the H-B guidelines in this study, which means a double reduction: artificially and theoretically. This study used the indoor rock test data, which were only modified according to the H-B criterion, so the compressive strength of the rock and the modified rock mass are slightly larger. From the engineering design perspective, the double reduction in the rock mass in the deterioration area is conservative and meets the engineering requirements. However, from the collapse disaster prediction view, overly conservative data may increase monitoring time and cost and even result in erroneous results 32 .
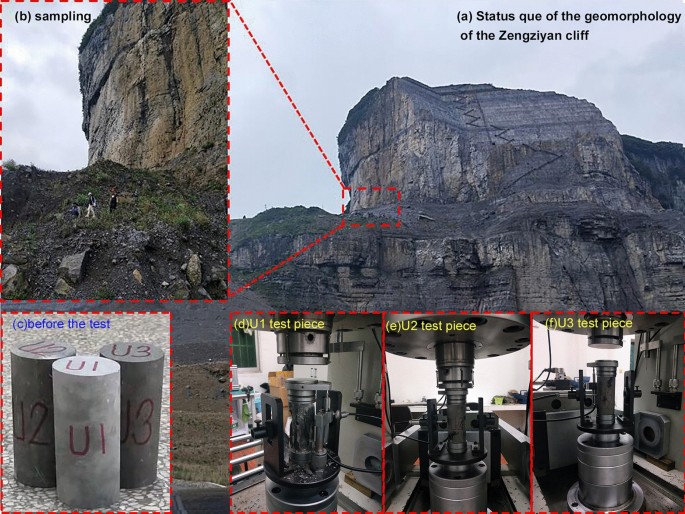
The overall process of the uniaxial compressive strength test of the rock; Submaps ( a ) and ( b ) are the collapsed place of the Zengziyan cliff where we climbed to collect the original rock blocks. Submaps ( c ) and ( d ) are the standard cylindrical specimens drilled from the original rock blocks; the diameter is 50 mm, and the height is 100 mm.
If not considering the rock mass strength attenuation in the deterioration area, the compressive stress induced by the upper incomplete deterioration area is much smaller than the rock mass compressive strength in the deterioration area. Thus, the deterioration area will be stable based on the theory of material mechanics. However, compression-induced instability may occur considering the in situ rock mass deterioration shown in the uniaxial compressive strength curve. This indicates that the rock mass deterioration effect can quantitatively explain the compression-induced instability mode of the TCURM collapse initiation mechanism.
In summary, the frequency–response stability coefficient can be obtained to judge the stability of the TCURM, with the fundamental frequency monitoring data obtained by the laser Doppler vibrometer in the field, combined with the theoretical calculation results (Fig. 7 ). Although there are no two similar TCURMs in the field, but the analysis methods and ideas proposed in this study can be used for reference.
It should also be noted that the unit of the stiffness coefficient in “The deterioration law of the stiffness coefficient by the time” is 10 6 N/m, which is different from the stiffness coefficient in “Sensitivity analysis of the physical parameters”, which is 10 9 N/m. The reason is that the height of the standard cylinder in “The deterioration law of the stiffness coefficient by the time” is 100mm and the height of the tower-column shaped unstable rock mass in “Sensitivity analysis of the physical parameters” is 240m, so the similar scale is nearly 2400 times. If we multiply the stiffness coefficient in “The deterioration law of the stiffness coefficient by the time” by 2400, the stiffness coefficient in “The deterioration law of the stiffness coefficient by the time” and “Sensitivity analysis of the physical parameters” are of the same unit order of magnitude.
The validation of the TCURM stability based on reliability theory
To further validate the frequency–response stability analysis method proposed in this study, a comparative analysis is carried out based on a geological field survey and reliability theory. First, the test parameters of the physical and mechanical indices of the Zengziyan rock mass are statistically analyzed to obtain its statistical indices. Second, the site investigation and statistics are carried out in the deterioration area at the bottom of the second cliff of the collapsed relic of the Zengziyan rock mass, and the statistics of the geological strength index GSI are obtained. The H-B parameters ( s and a ) are calculated in combination with Eqs. ( 15 )–( 16 ). Finally, according to the advanced first-order second-moment method (Eqs. ( 20 )–( 25 )), the reliability index and failure probability under saturated and natural conditions are calculated with MATLAB programming. The results are shown in Table 2 .
Conclusions can be made from the results in Table 2 and the assessment criteria in Table 1 : (1) The instability probability under saturated conditions is 96.27%, which is unstable. The instability probability under natural conditions is 80.3%, which is a poor stability, indicating that the UCS of the rock mass has a significant influence on the stability of the compression-induced instability mode of the TCURM. (2) The average GSI from the field investigation is 53.57. According to the deterioration of the GSI and other physical parameters given in Fig. 4 , the deterioration area stiffness is approximately 2.36 × 10 11 N/m, the fundamental frequency f 1 is 2.66 Hz, the frequency–response reduction coefficient D t is 0.745, the UCS [ σ b ] of the rock mass is 3.8 MPa, and the frequency–response stability coefficient F S d is 0.56 (instability). The results of the reliability theory analysis are consistent with the frequency–response stability analysis results. Calculating the stability of the TCURM according to the fundamental frequency monitoring data is reasonable and feasible.
Conclusions
The deterioration degree of a TCURM has a significant spatial variability, and the deterioration area with poor quality at the bottom controls its instability mode. A TCURM is composed of an incomplete deterioration area and a deterioration area. A two-degree-of-freedom vibration mechanics model is established according to the mass concentration method. The fundamental frequency used for the stability analysis, instability prediction and early warning of the unstable rock mass is explored. By literature comparison, laboratory tests, field investigations, and the ASMM of reliability theory, the rationality and feasibility of this method are verified, providing a new idea for the development of noncontact, nondestructive dynamic monitoring and early warning indicators of TCURMs. The main conclusions are as follows:
A two-degree-of-freedom vibration mechanics model of a TCURM is established. The frequency–response reduction coefficient is calculated by using the fundamental frequency. The GSI is obtained equivalently according to the damage theory. The formulas for the compressive strength of the rock mass in the deterioration area and the expression of the frequency–response stability coefficient are obtained based on the generalized Hoek‒Brown criterion.
The ASMM of the reliability theory is introduced. The performance function of the TCURM compression-induced instability model is established, which is mutually tested with the frequency–response stability analysis method and used to construct a frequency–response stability reliability analysis method.
Taking the W12 # TCURM on the Zengziyan cliff of Nanchuan District in Chongqing municipality as an example, a sensitivity analysis of parameters such as the fundamental frequency, GSI, and frequency–response stability coefficient is carried out. When the fundamental frequency is larger than 3.29 Hz, the TCURM does not reach the instability stage. The calculation results of the frequency–response stability analysis method are discussed by employing the engineering test parameters, indoor testing, and a literature comparison. The engineering test parameters and the GSI of the field investigation are statistically analyzed. These results, combined with the ASMM, are used to determine the failure probabilities under natural and saturated conditions of 80.3% and 96.27%, respectively, which reflect poor stability and instability. The evaluation results are consistent with the results of the frequency–response stability analysis.
Furthermore, an indoor test model of a TCURM is established, and the laser Doppler vibrometer is used to verify the physical model. According to different geological conditions and unstable rock mass styles, the layout and number of natural vibration frequency monitoring points need further development.
Data availability
No datasets were generated or analysed during the current study.
Du, Y. et al. Safety monitoring experiment of unstable rock based on natural vibration frequency. Rock Soil Mech. 37 (10), 3035–3040 (2016).
Google Scholar
Yang, J. W., Bai, R. C., Yu, Y. J. & He, F. Inversion test and numerical verification on natural period of rock slope with weak layers. J. China Coal Soc. 40 (S1), 69–75 (2015).
Valentin, J. et al. The dynamic response of prone-to-fall columns to ambient vibrations: comparison between measurements and numerical modelling. Geophys. J. Int. 2 , 1–41 (2017).
Du, Y., Wu, Z. X., Xie, M. W., Liu, R. C. & Chen, C. Early-warning method of rock collapse and its experimental verification. J. China Coal Soc. 44 (10), 3069–3075 (2019).
Du, Y., Lu, Y. D., Xie, M. W. & Jia, J. L. A new attempt for early warning of unstable rocks based on vibration parameters. Bull. Eng. Geol. Environ. 79 , 4363–4368 (2020).
Article Google Scholar
George, H. & Rene, B. T. Modal analysis for damage detection in structures. J. Struct. Eng. 117 (10), 3042–3061 (1991).
Levy, C., Baillet, L., Jongmans, D., Mourot, P. & Hantz, D. Dynamic response of the Chamousset rock column (Western Alps, France). J. Geophys. Res. 2010 (115), 1–13 (2010).
Moore, J. R., Geimer, P. R., Finnegan, R. & Thorne, M. S. Use of seismic resonance measurements to determine the elastic modulus of freestanding rock masses. Rock Mech. Rock Eng. 51 (12), 3937–3944 (2018).
Article ADS Google Scholar
Moore, J. R., Geimer, P. R., Finnegan, R. & Michel, C. Dynamic Analysis of a Large Freestanding Rock Tower (Castleton Tower, Utah). Bull. Seismol. Soc. Am. 109 (5), 2125–2131 (2019).
Ma, G. C., Sawada, K., Yashima, A. & Saito, H. Experimental study of the applicability of the remotely positioned laser doppler vibrometer to rock-block stability assessment. Rock Mech. Rock Eng. 48 (2), 787–802 (2015).
Siringoringo, D. M. & Fujino, Y. Noncontact operational modal analysis of structural members by laser doppler vibrometer. Comput.-Aided Civ. Inf. 24 (4), 249–265 (2009).
He, K., Yin, Y. P., Li, B. & Chen, C. L. The mechanism of the bottom-crashing rockfall of a massive layered carbonate rock mass at Zengziyan, Chongqing. China. J. Earth. Syst. Sci. 128 (4), 103–116 (2019).
ADS Google Scholar
He, K., Chen, C. L., Feng, Z., Li, B. & Pan, L. B. A review on the collapse hazards of tower-shaped rock. J. Geomech. 22 (3), 714–724 (2016).
Yin, Y. P., Yan, G. Q., Huang, B. L., Dai, Z. W. & Qin, Z. Geological strength index of the slope rock mass deterioration process of the hydro-fluctuation belt in the Three Gorges Reservoir. China. ASCE J. Hydraul. Div. 51 (8), 883–896 (2020).
Chowdhury, R. & Flentje, P. Role of slope reliability analysis in landslide risk management. Bull. Eng. Geol. Environ. 62 (1), 41–46 (2003).
Johari, A., Fazeli, A. & Javadi, A. A. An investigation into application of jointly distributed random variables method in reliability assessment of rock slope stability. Comput. Geotech. 47 , 42–47 (2013).
Duzgun, H. & Bhasin, R. K. Probabilistic stability evaluation of oppstadhornet rock slope, norway. Rock Mech. Rock Eng. 42 (5), 729–749 (2009).
Johari, A. Mehrabani Lari. System probabilistic model of rock slope stability considering correlated failure modes. Comput. Geotech. 81 , 26–38 (2017).
Jimenez-rodriguez, R. & Sitar, N. Rock wedge stability analysis using system reliability methods. Rock Mech. Rock Eng. 40 (4), 419–427 (2007).
Johari, A. & Mehrabani, L. A. System reliability analysis of rock wedge stability considering correlated failure modes using sequential compounding method. Int. J. Rock Mech. Min. Sci. 82 , 61–70 (2016).
Tang, H. M., Shu, Q. J. & Wang, L. F. Reliability analysis of perilous toppling rock considering seismic force direction. Appl. Math. Mech. 41 (3), 319–328 (2020).
Tang, H. M., Zhang, X. C., Wang, L. F., Shen, J. G. Study on the landforms evolution of high cliff in limestone area: Taking zhenziyan cliff of Jinfo mountain in Chongqing city as an example. Journal of Chongqing Normal University(Natural Science) 35 (5):54–62, (2018).
Tang, H. M., Zhou, F. C., Chen, S. & Wang, L. F. Fracture mechanism of unstable rock with double-crack control discontinuity subjected to high earthquake intensities. Applied Math. Mech. 42 (6), 645–655 (2021).
Feng, Z., Li, B., Yin, Y. P. & He, K. Rockslides on limestone cliffs with sub-horizontal bedding in the southwestern calcareous area. China. Nat. Hazard Earth Sys. 14 (9), 2627–2635 (2014).
Zhou, F. C., Tang, H. M., Chen, S., Wang, L. F. & Shang, C. Research on the Damage-catastrophe Mechanism of a Tower-column Unstable Rock Mass with Compressed-fracture Slumping Mode Under Multi-factor Couplings. Geotech. Geol. Eng 41 , 135–151 (2022).
Hu, H. T. Research on the collapse and falling stone. J. Railway Eng. Soc. S1 (1), 387–391 (2005).
Hoek, E. & Brown, E. T. The Hoek-Brown failure criterion and GSI–2018 edition. J. Rock Mech. Geotech. 3 , 1–19 (2018).
Bafghi, A. R. Y. & Verdel, T. The probabilistic key-group method. Int. J. Numer. Anal. Methods Geomech. 28 (9), 899–917 (2004).
Zhang, L. L., Zhang, J., Xu, Y. & Li, X. Reliability theory of geotechnical engineering (Tongji University Press, 2011).
Tang, H. M. et al. Experimental study on the dynamic acid water weakening mechanism of limestone. Chin. J. Rock Mech. Eng. 42 (6), 1312–1324 (2023).
He, K., Li, B., Zhu, S. N. & Gao, Y. Experimental Study on Rock Damage Characteristic of the Key Zone for Collapsed Rock Mass. Chin. J. Underground Space Eng. 14 (6), 1490–1497 (2018).
Xu, Q. Understanding the landslide monitoring and early warning: consideration to practical issues. J. Eng. Geol. 28 (2), 360–374 (2020).
Download references
Acknowledgements
The authors are grateful to the anonymous reviewers and relevant editors for the constructive criticism and suggestions. In addition, the studies in this manuscript were supported by the National Natural Science Foundation of China (Grant Nos. 51378521, 51678097), the Chongqing Natural Science Foundation(Grant No. CSTB2024NSCQ-MSX0006), the China Postdoctoral Science Foundation (Grant No. 2023M730432), the Chongqing Postdoctoral Science Foundation (Grant No. CSTB2023NSCQ-BHX0223), the Science and Technology Research Program of Chongqing Municipal Education Commission (Grant No. KJQN202300744).
This work was financially supported by the National Natural Science Foundation of China (Grant Nos. 51378521, 51678097), the Chongqing Natural Science Foundation(Grant No. CSTB2024NSCQ-MSX0006), the China Postdoctoral Science Foundation (Grant No. 2023M730432), the Chongqing Postdoctoral Science Foundation (Grant No. CSTB2023NSCQ-BHX0223), the Science and Technology Research Program of Chongqing Municipal Education Commission (Grant No. KJQN202300744). The authors have no relevant financial or non-financial interests to disclose, All authors contributed to the study conception and design, and this manuscript is approved by all authors for publication. All authors declare that we consulted the Guide for Authors in preparing the manuscript. And the work described was prepared in compliance with the Ethics in Publishing Policy. Te research work has not been published previously, and not under consideration for publication elsewhere, in whole or in part.
Author information
Authors and affiliations.
School of Civil Engineering, Chongqing University, 174 Shazheng Street, Shapingba District, Chongqing, 400045, China
Fuchuan Zhou, Xinrong Liu & Xueyan Guo
Chongqing Vocational Institute of Engineering, Chongqing, 402260, China
Fuchuan Zhou
Institute of Geotechnical Engineering, Chongqing Jiaotong University, Nan’an District, 66 Xuefu Road, Chongqing, 400074, China
Hongmei Tang
CCCC Third Harbor Engineering Co., Ltd., Shanghai, 200030, China
Mengqi Miao
School of River and Ocean Engineering, Chongqing Jiaotong University, Chongqing, 400074, China
You can also search for this author in PubMed Google Scholar
Contributions
F.Z. wrote the main manuscript text, X.L. and H.T. provided the idea and validated, and. All authors reviewed the manuscript.
Corresponding author
Correspondence to Xinrong Liu .
Ethics declarations
Competing interests.
The authors declare no competing interests.
Additional information
Publisher's note.
Springer Nature remains neutral with regard to jurisdictional claims in published maps and institutional affiliations.
Rights and permissions
Open Access This article is licensed under a Creative Commons Attribution-NonCommercial-NoDerivatives 4.0 International License, which permits any non-commercial use, sharing, distribution and reproduction in any medium or format, as long as you give appropriate credit to the original author(s) and the source, provide a link to the Creative Commons licence, and indicate if you modified the licensed material. You do not have permission under this licence to share adapted material derived from this article or parts of it. The images or other third party material in this article are included in the article’s Creative Commons licence, unless indicated otherwise in a credit line to the material. If material is not included in the article’s Creative Commons licence and your intended use is not permitted by statutory regulation or exceeds the permitted use, you will need to obtain permission directly from the copyright holder. To view a copy of this licence, visit http://creativecommons.org/licenses/by-nc-nd/4.0/ .
Reprints and permissions
About this article
Cite this article.
Zhou, F., Liu, X., Tang, H. et al. Analysis of the frequency–response stability and reliability of a tower-column unstable rock mass on a high and steep slope. Sci Rep 14 , 20308 (2024). https://doi.org/10.1038/s41598-024-70941-2
Download citation
Received : 09 December 2023
Accepted : 22 August 2024
Published : 02 September 2024
DOI : https://doi.org/10.1038/s41598-024-70941-2
Share this article
Anyone you share the following link with will be able to read this content:
Sorry, a shareable link is not currently available for this article.
Provided by the Springer Nature SharedIt content-sharing initiative
By submitting a comment you agree to abide by our Terms and Community Guidelines . If you find something abusive or that does not comply with our terms or guidelines please flag it as inappropriate.
Quick links
- Explore articles by subject
- Guide to authors
- Editorial policies
Sign up for the Nature Briefing newsletter — what matters in science, free to your inbox daily.

- Open access
- Published: 01 September 2024
Downstream impacts of dam breach using HEC-RAS: a case of Budhigandaki concrete arch dam in central Nepal
- Anu Awal 1 ,
- Utsav Bhattarai 2 ,
- Vishnu Prasad Pandey 3 &
- Pawan Kumar Bhattarai 4
Environmental Systems Research volume 13 , Article number: 37 ( 2024 ) Cite this article
Metrics details
Studies on concrete dam breach are limited compared to earthen and other types of dams. With an increase in the construction of concrete dams, particularly in the developing world, it is imperative to have a better understanding of the dam breach phenomena and the identification of the most influential breach parameters. This study aims to contribute to this gap by taking the case of the concrete arch dam proposed for the 1200 MW Budhigandaki Hydropower Project located in central Nepal. This study carries special significance for Nepal, primarily because of the increasing number of under construction and proposed large dams for water resources development in the country. We carry out dam breach analysis of the Budhigandaki dam using HEC-RAS 2D model to calculate the flood discharge peaks, time to peak, water surface elevation and the extent of inundation for two scenarios (with and without probable maximum flood) to estimate the damage on four downstream settlements. We carry out sensitivity analysis of the breach parameters on the flood magnitudes and severity. Results show that all the study locations lie in the high flood hazard zone. Flood peaks can reach as high as 286,000 m 3 s − 1 to 511,000 m 3 s − 1 in the considered settlements. The time to peak ranges from 11.3 to 17 h after the breach at these locations. We estimate that if a breach should happen, it would most likely inundate around 150,000 buildings, impact nearly 672,000 lives and flood 3,500 km of road downstream. Furthermore, dam breach elevation is found to be the most sensitive parameter to downstream floods. Hence, rather than structural measures, it is recommended that non-structural measures are implemented for minimizing the impacts of flood disasters at the study locations. The findings could be a useful reference for future dam projects in Nepal and other areas with similar hydrological and topographical conditions.
Introduction
Dams are storage structures providing beneficial functions such as flood control and water supply for different types of users (for example, domestic water supply, hydropower, irrigation, recreation and water transport). The construction of large dams along with generation of electricity started during the industrial revolution in Europe and America. The early 1900s ushered in an era of “big dam” building in America mostly for hydropower generation as demands for electricity increased, the Hoover Dam being regarded as an engineering marvel. The Asian region includes some of the largest dams in the world today such as Tarbela Dam and Mangla Dam in Pakistan, Nurek Dam in Tajikistan, San Rogue Dam in Phillipines and Three Gorges Dam in China, mostly for hydropower generation.
Despite the benefits, failure of dams can cause tremendous losses by generation of unforeseen flood magnitudes in downstream areas. Unfortunately, the history of dams has been studded with disasters of various types, sometimes of great magnitude, with loss of human lives and destruction of property and infrastructure (Aureli et al. 2021 ). USACE ( 2018 ) lists causes of dam breach as earthquakes, landslides, extreme storms, piping, equipment malfunction, structural damage, foundation failure, and sabotage. Regardless of the reason, almost all failures begin with a breach formation.
Basically, breach is defined as the opening formed in the dam body that leads the dam to fail and this phenomenon causes the stored water behind the dam to propagate rapidly downstream (Dincergok 2007 ). Despite piping or overtopping being the main modes of dam failure, the actual mechanics are still not completely understood for either earthen or concrete dams (USACE 2018 ). Past dam-failure disasters have shown that the majority of dams that have failed are earthen (74 dam breaks out of 7812 earthen dams) and the highest percentage of failure of rockfill dams (17 dam breaks out of 200 rockfill dams) (Fang et al. 2017 ). The world’s worst dam disaster happened in China in 1975 when the Banqiao and Shimantan dams failed killing about 171,000 people while 11 million lost their homes (Vincent et al. 2020 ). In 1979, the 25 m high Machu Dam in India, which stored 100 million m 3 , failed after several hours of over-topping causing about 10,000 deaths, 150,000 people were displaced, and 10,000 habitations were destroyed (Lempérière 2017 ). A recent case of the failure of the Rishiganga dam in Uttarakhand (India) in 2021 due to glacier avalanche caused more than 200 deaths and severely damaged infrastructure (Shugar et al. 2021 ). Similarly, failure of the Edenville dam followed by the Sanford dam downstream on the same day in 2020 due to heavy rain in Michigan USA ( Independent Forensic Team 2022 ), and failure of the Spencer Dam in Nebraska USA in 2019 due to ice run (Ettema et al. 2021 ), demonstrate the devastation that dam breaches can lead to. Thus, identification of the vulnerable areas and being aware of the likely damages are key for minimization of the adverse impacts of dam breach.
Dam breach analysis involves three key sequential steps: predicting the reservoir outflow hydrograph, determining dam breach parameters, and routing the hydrograph downstream. Essentially, the breach flood hydrograph depends on the prediction of breach geometry and breach formation time (Basheer et al. 2017 ). There have been many studies on dam breach analysis around the world from the 1980’s (Leng et al. 2023 ; Singh and Snorrason 1984 ; USACE 2024 ). Dam breach analysis is generally carried out by either numerical/computer models or scaled-down physical models. The United States Department of Interior ( 1988 ), recommends estimating a reasonable maximum breach discharge using four principal methods:
Physically Based Methods: Using erosion models based on principles of hydraulics, sediment transport and soil mechanics, development of breach and resulting breach outflow are estimated;
Parametric Models: Time to failure and ultimate breach geometry are assessed utilizing case studies; breach growth is simulated as a time-dependent linear process and breach outflows are computed using principles of hydraulics;
Predictor Equations: Using data of case studies, peak discharge is estimated from empirical equations and a reasonable shape of outflow hydrograph is assumed; and.
Comparative Analysis: Breach parameters are determined by comparison of dam under consideration and a dam that failed.
There are far fewer studies on the failures of concrete dams compared to earthen dams, especially due to breaches which leads to difficulty in determining the concrete dam breach parameters (Fang et al. 2017 ). Moreover, a study of well documented dam-failure cases showed that empirical formulas provide results closer to reality (Fang et al. 2017 ). For instance, Froehlich( 1995 ) developed a prediction equation for the average breach width based on 63 cases of embankment-dam failures and an equation for the breach-formation time based on 21 cases. Focusing on earthen dams has been driven by their historical prevalence, cost-effectiveness, and adaptability. However, studying concrete arch dams is crucial for advancing engineering practices, improving safety and efficiency in dam construction, supporting hydroelectric power generation, addressing environmental impacts, and preserving significant cultural landmarks. Many federal agencies such as FERC ( 1993 ),Office of the State Engineer( 2020 ) and USACE ( 2014 ) have published guidelines recommending possible ranges of values for breach width, side slopes, and development time for different types of dams. This study aims to investigate the breach characteristics of concrete arch dams, an area with limited existing literature. Several dam breach analysis studies have been carried out in Nepal such as in Kulekhani dam using HEC-RAS (Pandey et al. 2023 ), Kaligandaki landslide dam using BREACH (Bricker et al. 2017 ), Koshi high dam using HEC-RAS (Gyawali, D.R. and Devkota, 2015 ), among others. However, no sensitivity analysis of dam breach parameters has been carried out for the afore-mentioned studies.
The proposed Budhigandaki dam located in the transboundary Budhigandaki Basin, spread over southern China and central Nepal, is taken as a case. The Government of Nepal (GoN) has prioritized hydropower generation as the backbone of economic development to attain the goals to raise the country’s status to middle income country level by 2030 (Government of Nepal 2020 ). As a result, there are currently more than 9 planned and proposed large hydropower dam projects by the state (Nepal Electricity Authority 2022 ). The Budhigandaki Hydropower Project (BGHPP) could be the largest storage project of Nepal, if constructed, which could lead to catastrophic damages downstream in the event of a breach.
Hence, the overarching objective of this study is to assess the flood impacts of the Budhigandaki Dam on the downstream settlements due to possible dam breach scenarios. Specifically, this study intends to quantify the peak discharge, time to peak, and the water surface elevation at the downstream locations due to a dam-breach flood. Further, sensitivity analysis of five different dam breach parameters is conducted to acquire information about extent of influence of each parameter on the dam breach. The analysis is carried out in the widely-used hydraulic model Hydrologic Engineering Center’s - River Analysis System (HEC-RAS) developed by the United States Army Corps of Engineers (USACE). Furthermore, zoning of the downstream settlement areas in Geographic Information System (GIS) based on flood severity provides meaningful information to the project developers as well as planners in the impacted areas.
Materials and methods
The Budhigandaki Hydropower Project (BGHPP) is a 1200 MW storage type proposed project of Nepal located approximately 2 km upstream of the confluence of Budhigandaki River with Trishuli River as shown in Fig. 1 . The Budhigandaki Dam is a 263 m high double curvature concrete arch dam with a reservoir volume of 4.5 billion cubic meters (BCM), out of which the active storage is 2.2 BCM. The dam crest length is 737.4 m and the reservoir Full Supply Level (FSL) is at 540 m above sea level (masl) (Budhigandaki Development Committee, 14a). There are some major settlement areas nearly 110 km downstream which are susceptible to danger in case of dam breach. For this study, four major towns namely, Narayangarh, Baraghare, Divyanagar and Meghauli, have been assessed. Moreover, future risk of impact from the dam failure can be expected to increase as increased in population growth due to improved job opportunities and other economic activities in the area because of the construction of the dam. Therefore, the Budhigandaki Dam has been taken as a case in this study to assess the flooding impacts of the dam on the downstream areas through simulation of a hypothetical dam failure.
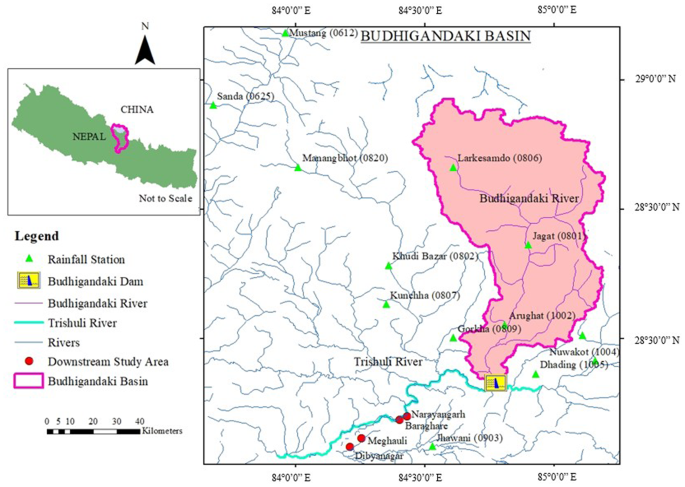
Location of Budhigandaki dam and downstream settlement areas
Methodology
Dam breach analysis of the Budhigandaki dam has been carried out in HEC-RAS using unsteady flow simulation with terrain and land cover as the geometric input data. The upstream boundary condition is the probable maximum flood (PMF) hydrograph which has been generated using an empirical method while the downstream boundary condition is normal depth. Two dam failure scenarios, namely, dam breach at reservoir full condition with PMF (Scenario I: base case) and dam breach at reservoir full condition without PMF (Scenario II), have been modelled in the study. Outputs of the simulation are used for creating flood inundation maps, flood hazard vulnerability maps and flood arrival time maps corresponding to the different scenarios. Sensitivity analysis of the dam breach parameters is also carried out to assess their impacts on the flood conditions downstream of the dam. Figure 2 summarizes the overall research methodology.
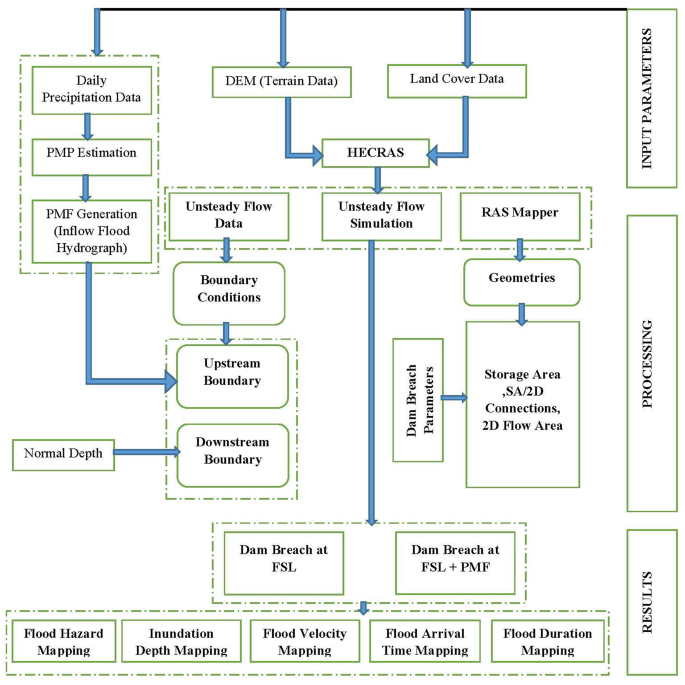
Overall research methodology of this study. DEM: Digital Elevation Model, PMP: Probable Maximum Precipitation, PMF: Probable Maximum Flood, SA: Storage Area, 2D: Two Dimensional, FSL: Full Supply Level
The spatial inputs required to model the dam breach are digital elevation model (DEM), land cover and Manning’s roughness coefficient. Rainfall and discharge are needed for generation of inflow hydrograph as upstream boundary condition to the model. In addition, infrastructure data of the downstream area is required for estimating the impacts of floods. Details of the required data and their sources are presented in Table 1 .
PMP and PMF
The probable maximum precipitation (PMP) is the theoretical maximum precipitation for a given duration under current meteorological conditions (World Meteorological Organization 2009 ). Daily maximum rainfall data of 13 surrounding stations from 1972 to 2014 has been used for the calculation of PMP. The 1-day PMP for all the stations was calculated using Hershfield formula (Hershfield 1965 ) given in Eq. ( 1 ) :
Where, PMP = Probable maximum precipitation.
M = mean of maximum daily rainfall sample S = Standard deviation.
K = Frequency factor = 15 (Hershfield 1965 ).
The calculated 1-day PMP of the point stations was further interpolated using Thiessen Polygon, Kriging, Spline and Inverse Distance Weighing (IDW) methods in GIS to compute the 1-day PMP for the Budhigandaki Basin. In order to model a worst-case scenario, the maximum value of the PMP among these methods was chosen for generating the PMF hydrograph.
Probable Maximum Flood (PMF) is theoretically the flood resulting from a combination of the most severe meteorological and hydrologic conditions that could conceivably occur in a given area (FERC 2001 ). HEC-RAS requires a flood hydrograph to be provided as input for the unsteady flow analysis in the dam breach model. Therefore, a synthetic unit hydrograph was developed using Snyder’s Method (American Geophysical Union 1938 ) using the following equations (Eq. ( 2 ) to Eq. ( 7 ) which was then transposed to generate a direct runoff hydrograph of PMF.
Mathematically,
Dam breach analysis
Dam breach analysis of the Budhigandaki dam has been carried out in HEC-RAS model under two-dimensional dynamic (unsteady-flow) mode. Hypothetical breach of the dam and its propagation downstream has been modelled using 2D Diffusion wave equations (Eq. ( 8 ) to Eq. ( 10 )).
Where, h is the water depth (m), p and q are the specific flow in the x and y directions (m 2 s − 1 ), ζ is the surface elevation (m), g is the acceleration due to gravity (9.8 m s − 2 ), n is the Manning’s coefficient, ρ is the water density (1000 kg m − 3 ), τ xx , τ yy , and τ xy are the components of the effective shear stress along x and y directions (N m − 2 ), and f is the Coriolis (s − 1 ).
Two-dimensional (2D) mesh of size 100 m x 100 m was chosen to represent the downstream land. Comparison of different mesh sizes (100 m and 200 m) indicated no significant difference in model performance. The storage areas and downstream areas are connected using an inline structure (Budhigandaki dam) as shown in Fig. 3 . “Storage Area” refers to upstream reservoir of the dam axis while “Downstream Study Area” represents the four towns (Narayangarh, Baraghare, Divyanagar, and Meghauli) located downstream which are likely to be inundated in case of dam breach (BGHP, 2015). Boundary conditions are required at the upstream and downstream ends of the model for flood routing. The upstream boundary was fixed at the reservoir extent (storage area) and the boundary condition was provided in the form of flood hydrograph generated from PMF. Outlet is the downstream boundary past the settlement areas as shown in Fig. 3 while the boundary condition of normal depth is maintained by providing the river bed-slope obtained from the DEM.
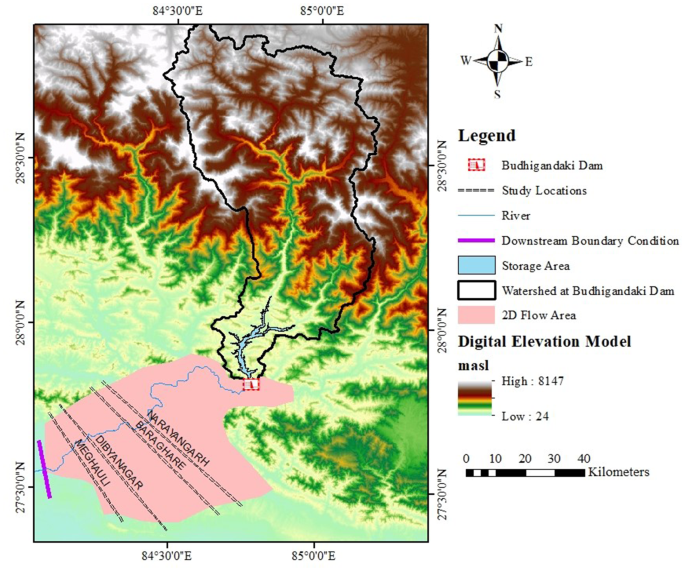
HEC-RAS 2D flow area and model schematic for the flood simulation of Budhigandaki dam breach
Scenarios and sensitivity analysis
In order to quantify the downstream effects of the Budhigandaki dam breach, the following two scenarios have been simulated:
Scenario 1: Dam breach when reservoir is at FSL with PMF. Scenario 2: Dam breach when reservoir is at FSL.
Only overtopping breach mode was analyzed as the dam is made up of concrete and there are less chances of other failure modes (Zhang et al. 2016 ). Moreover, for better understanding the Budhigandaki dam breach mechanism and impacts, sensitivity analysis of the following five important breach parameters as breach bottom elevation, breach bottom width, breach weir coefficient, breach formation time and breach side slope was carried out by varying their values over a reasonable range obtained from literature.
Scenario I have been considered as the base case. Sensitivity of the above-mentioned breach parameters on flood peak discharge, water surface elevation and flood arrival time at the four downstream locations along with inundation area are analyzed considering the base case.
The inputs for the dam break analysis adopted for the base case i.e., Scenario I is listed in the Table 2 . The values of breach parameters have been derived from FERC ( 1993 ), Office of the State Engineer ( 2020 ) and USACE ( 2014 ) specific for concrete dams.
Flood characteristics from 2D simulations
Using RAS Mapper, a series of flood maps were generated based on the outputs of the 2D simulation of the Scenario I dam breach. These maps were helpful in identifying the potentially risky and safe areas. The outputs of the HEC-RAS model were exported to GIS for further analysis and mapping.
Maximum Flood depth map
Using the simulation results, flood inundation maps were prepared illustrating the maximum flood depths across the study area for the different scenarios.
Flood Hazard Vulnerability Map : A flood hazard vulnerability map based on the product of depth and velocity was prepared using the Australian Rainfall-Runoff Guidelines (Australian Rainfall and Runoff 2019 ) which categorize the flood in six zones as: H1 ( D*V ≤ 0.3, D max = 0.3 m, V max = 2.0 m/s, safe for people, vehicles and buildings); H2 ( D*V ≤ 0.6, D max = 0.5 m, V max = 2.0 m/s, unsafe for small vehicles); H3 ( D*V ≤ 0.6, D max = 1.2 m, V max = 2.0 m/s, unsafe for vehicles, children and elderly); H4 ( D*V ≤ 1.0, D max = 2.0 m, V max = 2.0 m/s, unsafe for people and vehicles); H5 ( D*V ≤ 4.0, D max = 4.0 m, V max = 4.0 m/s, unsafe for people and vehicles, buildings vulnerable to structural damage) ; H6 ( D*V > 4.0, unsafe for people and vehicles, all buildings vulnerable to failure) where D and V refer to the flood depth and velocity, respectively while D max and V max refers to the maximum depth and maximum velocity, respectively.
Flood arrival Time Map
Flood arrival time maps represent the computed time (in hours or days) from a specified time in the simulation when the water depth reaches a specified inundation depth. For the case of Budhigandaki dam breach, flood arrival times at the four settlement areas were calculated and mapped.
Estimated values of PMP and PMF
The 1-day PMP value using the 13 precipitation stations was calculated to be 518 mm, 530 mm, 556 mm and 485 mm using Thiessen polygon, Kriging, inverse distance weighted (IDW), and Spline interpolation methods, respectively. As a worst-case scenario, we chose the IDW method, which gave the maximum value of PMP among the four methods, for generating the PMF hydrograph. Using the input data listed in the Appendix 1, ordinates of the synthetic unit hydrograph was computed using Snyder’s method as shown in Fig. 4 .

Synthetic Unit Hydrograph and Probable Maximum Flood Hydrograph for the Budhigandaki dam
From the synthetic unit hydrograph and rainfall intensity duration curve, Direct Runoff Hydrograph was generated. The flood values are generated for a 60-minute interval by linear interpolation between the ordinates of the unit hydrograph. August is the month with the highest flows at the Budhigandaki dam site. Therefore, base flow of 441 m 3 s − 1 which is the mean August flow (during 1964–2012) was added to obtain the final hydrographs (BGHPP Development Committee 2014b ). The final results are plotted in Fig. 4 . I t can be seen that the peak discharge of 11,669 m 3 s − 1 occurs at 33.9 h after the start of rainfall for PMF + base flow.
Flood depth and flood hazard vulnerability
The river valley of 110 km length from Budhigandaki dam to Meghauli was considered for the analysis. The maximum flood depth Fig. 5 shows that the flood depth is as high as 212 m in the upstream area as the river channel is narrow whereas the depth becomes lesser in the downstream river sections where the area is relatively wide and plain. The maximum water depths at Narayangarh is estimated to be 90 m followed by 50.3 m at Baraghare.
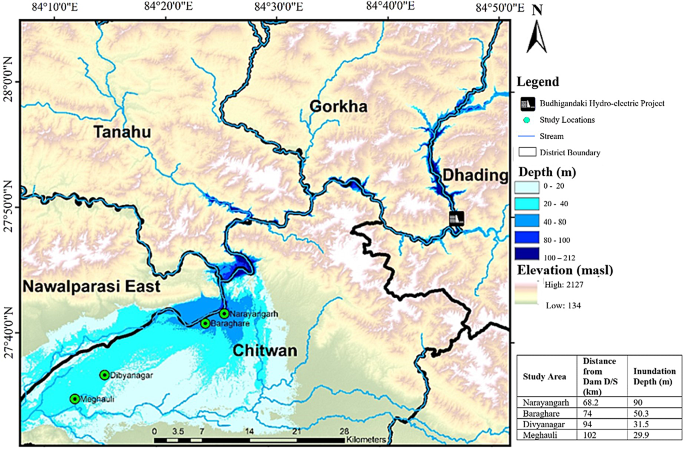
Flood Inundation Map Based on Maximum Depths
Similarly, Flood Hazard Vulnerability Map based on the depth and velocity was prepared as shown in Fig. 6 . It can be identified from the map that all the downstream area lies in H6 zone i.e., unsafe for people and vehicles and all buildings are vulnerable to failure.

Flood Hazard Vulnerability Mapping Based on Depth and Velocity
Flood arrival time
Simulated flood peak arrival times calculated at the four downstream settlement areas are shown in Fig. 7 . It is useful in designing of early warning systems at these locations. It can be seen that the travel times range from 11.3 h (Narayangarh) to 17 h (Meghauli) immediately after the dam breach depending on the proximity from the dam.
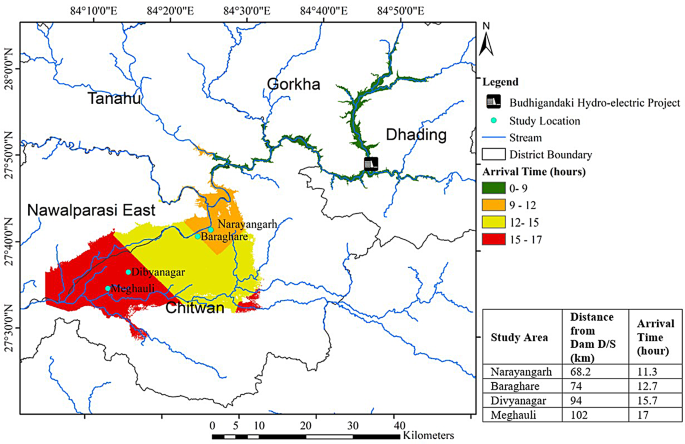
Flood arrival time for the major downstream settlement locations; D/S is downstream
Flood inundation across different land covers
As an impact of dam breach on land cover, it is seen that the inundated type to be most likely inundated is agricultural area (538 km 2 ). Similarly, 239 km 2 of forest is likely to be inundated second in rank. Grassland, water body, barren area, built-up area and shrub land are expected to be inundated with areas of 43 km 2 , 38 km 2 , 25 km 2 , 22 km 2 and 1.5 km 2 respectively as shown in Fig. 8 .
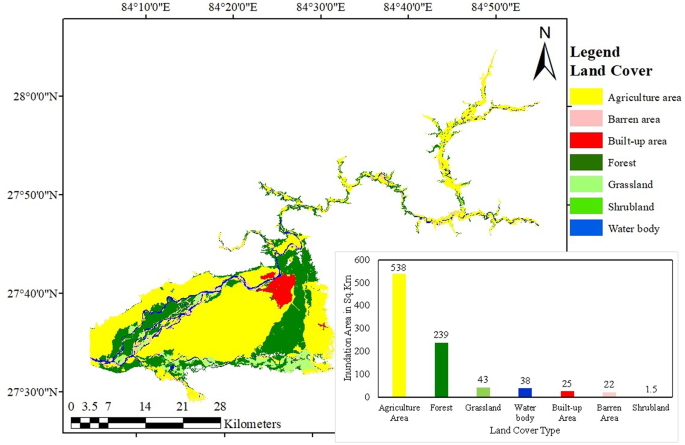
Inundation extent due to dam breach by land cover
Flood Impact on Water Surface Elevation (WSE) and peak discharge
Water surface elevations along the modelled river reach corresponding to the two scenarios are shown in Fig. 9 . It is seen that the water surface is nearly 110 m above the bed level at immediate downstream of the dam site while it is as low as 30 m in the downstream study areas. There is an enormous volume of water flowing down in a very short time because of the breach resulting in such high values of water depths along the river reach. There is very less change in the water surface elevation between Scenario-1 and 2. Also, at the settlement areas, the flow width is large i.e., flat plain area and hence lesser change is seen on the water surface elevation at downstream areas.
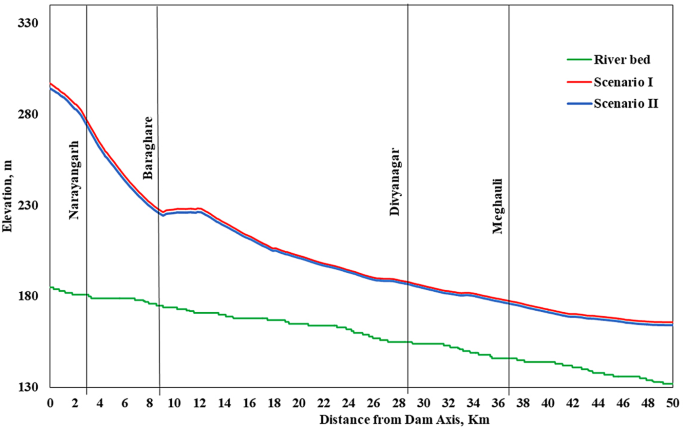
Profile of water surface elevation and river bed for Scenario I and Scenario II. Scenario I: Dam Breach at FSL with PMF and Scenario II: Dam Breach at FSL without PMF
For the two scenarios (Scenario-1 and Scenario-2), the flow hydrographs have been compared at immediate downstream of the dam and at the four major settlement locations as shown in Fig. 10 . It is to be noted that the peak discharge occurs nearly at the same time for both scenarios at all locations. At Narayangarh, peak discharges for Scenarios-1 and 2 are 511,587 m 3 s − 1 and 501,479 m 3 s − 1 respectively i.e., around 2% of difference in the value. Similarly, at Baraghare, the peak discharge for Scenario-1 is 454,267 m 3 s − 1 whereas 441,862 m 3 s − 1 for Scenario-2 and for Divyanagar, the peak discharge for Scenario-1 is 364,697 m 3 s − 1 whereas 357,294 m 3 s − 1 for Scenario II respectively. Lastly for Meghauli, the peak discharge for Scenario-1 is 294,928 m 3 s − 1 whereas 286,813 m 3 s − 1 for Scenario-2. It is obvious that the peak discharge for Scenario-1 is greater than that of Scenario-2, however, the differences in the peak values between the two scenarios are quite small (in the range of 2–3%). This implies that the storage volume of the dam is the major contributor to the flood discharge rather than the PMF.

Comparison of flood hydrographs at major study locations for Scenario I and Scenario II. Scenario I: Dam Breach at FSL with PMF and Scenario II: Dam Breach at FSL without PMF
Flood impact on infrastructure
The possible impact of inundation due to dam breach on buildings and roads was assessed. The total road length includes several types of roads such as highways, feeder roads, district roads and local roads. The inundated highway road length has been computed separately and all other types of roads has been kept as other roads (Table 3 ). It can be seen that Chitwan is the most impacted district with 58.5% of buildings and 2,541 km of road likely to be inundated. Meanwhile, Gorkha is expected to be the least affected district with 2.6% buildings and 132.4 km road inundated. Also, 149,311 numbers of buildings are inundated in total. If the total number of persons on average per household is taken as 4.5 (Cental Bureau of Statisitics 2016 ), a total of about 0.7 million people are likely to be affected by inundation in the case of dam breach. This is about 2.3% of the total population of Nepal.
- Sensitivity analysis
Sensitivity analysis was performed in order to estimate the impact of the breach parameters on the simulated floods in the downstream impacted areas. The values of the input breach parameters were changed within a reasonable range, one at a time, in the dam breach model and the corresponding values of the peak discharge, water surface elevation, flood arrival time and land inundation area were recorded. Breach bottom elevation was varied from 450 masl to 525 masl. Similarly, breach width was varied from 55 m to 150 m and breach weir coefficient was varied from 0.9 to 1.7. Also, breach formation time was varied from 0.05 h to 0.3 h and breach side slope was varied from 0.7:1 to 2.5:1 ( H : V ). Results of the sensitivity analysis have been presented in Table 4 .
Breach bottom elevation
It is seen from Table 4 that as the breach bottom elevation is increased from 450 masl to 525 masl, the value of peak discharge and WSE are significantly decreased at the different downstream locations. It is observed that a 30% increase in breach bottom elevation (450 masl to 475 masl) led to 20–35% decrease in peak discharge, 20–25% decrease in WSE at different downstream locations and nearly 30% decrease in inundation area (893 km 2 to 735 km 2 ). However, the flood peak arrival time is not much altered due to change in breach bottom elevation.
Breach bottom Width
It is seen from Table 4 that an increase in breach width from 55 m to 150 m corresponds to an increase in discharge, WSE and inundation area but the change is not as significant as compared to that of change in breach bottom elevation. A 30% increase in breach width (80 m to 105 m) led to nearly 3% increase in peak discharge at all downstream locations. However, not much change is seen on the WSE, flood arrival time and inundation area due to change in breach bottom width.
Breach weir coefficient
An increase in the breach weir coefficient from 0.9 to 1.7 led to increase in discharge, WSE and inundation area but with a smaller magnitude compared to that of change in breach bottom elevation (Table 4 ). A 20% increase in breach weir coefficient (1.44 to 1.7) led to nearly 3% increase in peak discharge at all downstream locations. Also, no significant change is seen on the WSE, flood arrival time and inundation area due to change in breach weir coefficient.
Breach formation time
Interestingly, there is very insignificant change in peak discharge, WSE, flood arrival time and inundation area due to varying breach formation time (Table 4 ). The values of peak discharge, WSE, flood arrival time and inundation area remain almost unchanged despite the breach formation time is increased up to 200% (0.1 h to 0.3 h).
Breach side slope
A 50% increase in the side slope (1.3:1 to 2:1) led to nearly 2–3% increase in peak discharge as shown in Table 4 . Also, no significant change is seen on the WSE, flood arrival time and inundation area due to change in breach side slope.
Thus, results of the sensitivity analysis varying the values of the breach parameters, namely, dam breach bottom elevation, breach bottom width, breach weir coefficient, breach formation time and breach side slope on the peak discharge, WSE, flood arrival time and downstream inundation area has been summarized in Table 5 . It can be seen that dam breach bottom elevation is the most sensitive parameter with respect to output values such as peak discharge, WSE and downstream inundation area while breach formation time is the least sensitive parameter with respect to all the output parameters.
We have estimated the PMP followed by PMF which is the upstream boundary condition required for the dam breach model in HEC-RAS. The PMP value was chosen as 556 mm from the IDW method. Also, the PMP value as per the detail design report (BGHPP Development Committee 2014b ) is 594 mm. Both the values of PMP are generated using Hershfield formula. However, this slight variation in the PMP values is due to the difference in the values of frequency factor. The value of frequency factor in this study is taken as 15 (Hershfield 1965 ). Subsequently, the PMF value for this study is generated using Snyder’s Unit Hydrograph Method with peak discharge as 11,669 m 3 s − 1 . Besides, by using regional method the PMF was calculated to be 11,479 m 3 s − 1 and regional regression flood analysis method 11,957 m 3 s − 1 (Department of Electricity Development 2006 ). Hence, the PMF values considered in this study are assumed to be reliable.
Impacts of dam breach and sensitivity analysis of dam breach parameters
Simulation results of Scenario I and Scenario II showed that there is a huge peak discharge immediately downstream of the dam breach (Fig. 10 and the difference in discharge values for both scenarios is low. The reason for this is due to the large storage volume of the dam leading to minimum effect of PMF being observed. Also, the downstream tributaries are much smaller compared to the Budhigandaki mainstream river. Hence, their additional impacts on the dam breach flood magnitudes can be considered to be marginal. Additionally, the outputs such as peak discharge, WSE, flood arrival time and inundation area from the dam breach has been estimated as a standalone event. The impact of addition of inflows from the other tributaries (for example, due to localized cloudburst events) to the mainstream river in the downstream settlement area could be areas of further study.
Previous dam breach analysis on Budhigandaki dam has been carried out by Tractebel and jade consult as JV using TELEMAC software (BGHPP Development Committee 2014a ). The output results of the previous study appeared to be quite different from the study carried out using HEC-RAS. There could be various reasons for such discrepancies. The TELEMAC model has considered full dam breach whereas our study does not consider full dam breach. Also, the earlier model has considered high accuracy resolution LiDAR data and other input data (mesh size 30 m*50 m) whereas our study considers 30 m*30 m DEM data and 100 m*100 m mesh size due to model stability issues. However, the pattern of change in peak discharge and WSE at the different study locations are quite similar for both models.
Dam breach analysis has been carried out in different parts of the world using HEC-RAS adopting a methodology similar to ours. For example, simulations of the breach of Batutegi earthen Dam, Indonesia (Wahyudi 2004 ), Mosul earthen Dam, Iraq (Basheer et al. 2017 ) and the results of sensitivity analysis are found out to be quite similar to this study. All these studies showed that dam breach bottom elevation is the most sensitive parameter. Further, the trends in WSE and peak discharge with time and distance from the dam obtained in these studies are also comparable to those of our study. The WSE and peak discharge increased with the increase in the breach parameters as breach bottom elevation, breach bottom width, breach weir coefficient and breach side slope. The peak discharge decreased with increase in breach formation time and negligible change was seen on WSE. Hence, through sensitivity analysis, it is seen that dam breach bottom elevation is the most sensitive parameter while breach formation time is the least sensitive parameter with regards to the floods.
Challenges to flood management
This analysis of a hypothetical dam breach provides insight to the level of possible damage should such a breach occur. Also, it can be deduced from this study that construction of embankments along the river is not a practical mitigation measure because of the extremely high-water depths (nearly 90 m) that these structures need to retain within them. Hence, other non-structural preventive measures such as creating awareness regarding flood risks, community-based flood early warning system (CBFEWS), training and deployment of efficient disaster response teams, zoning of high-risk areas, avoiding construction/settlements in such areas, identification of evacuation centers etc. are recommended. The Yokohama Strategy and Plan of Action (World Conference on Natural Disaster Reduction 1994 ), Hyogo Framework for Action 2005–2015 (International Strategy for Disaster Reduction 2005 ), and the current Sendai Framework for Action 2015–2030 (United Nations 2015 ) highlight the importance of early warning in reducing disaster risk and enhancing the resilience of vulnerable communities. CBFEWS generates and disseminates meaningful and timely flood warnings to vulnerable communities threatened by flood, so they can prepare and act correctly in sufficient time to minimize the possibility of harm. Owing to non-structural measures, the response and adaptation to floods of the vulnerable communities vary widely and are impacted upon by various factors, such as community resilience and susceptibility to flood. Also, the effectiveness of the non-structural measures appears sensitive to the socio-economic changes and governance arrangements (Dawson et al. 2011 ). Nonetheless, non-structural measures provide flexible flood management options for adapting to the ever-changing river basins, socio-economic and climate scenarios, and are in line with the spirit of environment friendly and sustainable development (Shah et al. 2018 ). Also, research on identification of shelter areas and evacuation plan can be an extension of this study using network analysis, buffers and proximity analysis in GIS. Moreover, the sensitivity analysis depicts the most sensitive breach parameters which need to be considered with extreme importance during planning, design, construction and operation of the dam.
Conclusions
This paper simulated the dam breach scenarios of the proposed Budhigandaki dam in central Nepal using HEC-RAS and assessed the impacts on the downstream settlements. Flood peaks, water surface elevations and flood arrival times were calculated for the two scenarios with and without PMF. In addition, sensitivity analysis was carried out to examine the influence of the breach parameters on the flood characteristics.
Results show that the entire downstream area lies in high hazard zone with flood arrival times at Narayangarh, Baraghare, Divyanagar and Meghauli ranges from 11.3 h to 17 h. Moreover, a total of 1,49,311 number of buildings are prone to inundation in the case of dam breach along with 671,900 lives at risk and around 3,500 km stretch of road most likely to be severely damaged. The dam-break flood peak exceeds 650,000 m 3 s − 1 in the immediate downstream of the dam while it attenuates to 511,000 and 286,000 m 3 s − 1 at Narayangarh and Meghauli, respectively. The maximum depth of water ranges from 30 m (in the downstream flat areas) to 212 m (in the upstream steep gorges) clearly discarding the physical and economic feasibility of structural measures for flood management in this case. In addition, 538 km 2 of agricultural land and 25 km 2 of built-up land is at risk of flood inundation. Therefore, it is imperative to implement preventive and non-structural measures such as creating awareness regarding flood risks, developing community-based flood early warning system (CBFEWS), training and deployment of efficient disaster response teams, zoning of high-risk areas, avoiding construction/settlements in such areas, identification of evacuation centers, monitoring and constant auscultation of the structure and developing robust and efficient emergency and alert plans.
Furthermore, the differences in the peak discharges and water surface elevations between the two scenarios are very less at the study locations. This implies that the impact of the huge storage volume of the reservoir on the breach flood characteristics is considerably larger in comparison to the PMF. In addition, change in dam breach bottom elevation was found to be the most sensitive to floods compared to other dam breach parameters.
Additionally, the methodology applied in this study is conveniently replicable of other dams, large or small. However, the simulation run-times may vary depending upon the size of the dam, mesh size, simulation time step and other model complexities. It is to be noted that the case may change for snow fed rivers and glacier lakes. Also, while applying this method to other projects, one should always be careful about the boundary conditions and the initial values of dam breach parameters as they vary depending upon the dam under consideration.
Nepal has currently only one storage dam hydropower project (Kulekhani) in operation. With a greater number of storage projects being planned and under construction, this study could be a useful reference for such future projects. Moreover, this study provides interesting results particularly related to the sensitivity of the breach parameters of concrete arch dams, which could be applicable in study of similar dams in other regions of the world.
Data availability
No datasets were generated or analysed during the current study.
Abbreviations
Catchment Area (km2)
Peak flow coefficient (-)
Lag Coefficient (-)
Coriolis (s − 1 )
acceleration due to gravity (m s − 2 )
water depth (m)
Frequency Factor (-)
main channel length from basin outlet to upstream watershed boundary (km)
main channel length from outlet to a point nearest to centroid of watershed (km)
Mean of Maximum daily rainfall (mm)
Manning’s Coefficient (-)
Specific flow in x-direction (m 2 s − 1 )
Probable maximum precipitation (mm)
Discharge (m 3 s − 1 )
Specific flow in y-direction (m 2 s − 1 )
Unit peak discharge (m 3 s − 1 )
Standard Deviation (mm)
Base time (hours)
Rainfall excess duration time (hours)
Basin Lag time (hours)
Width of unit hydrograph at discharge value exceeded 50% of the peak discharge (hours)
Width of unit hydrograph at discharge value exceeded 75% of the peak discharge (hours)
Surface Elevation (m)
Water Density (kg m − 3 )
Effective Shear Stress (N m − 2 )
Effective Shear Stress along x direction (N m − 2 )
Effective Shear Stress along x and y direction (N m − 2 )
Effective Shear Stress along y direction (N m − 2 )
American Geophysical Union (1938) Reports and Papers, Hydrology
Aureli F, Maranzoni A, Petaccia G (2021) Review of historical dam-break events and laboratory tests on real topography for the validation of numerical models. Water 13(14). https://doi.org/10.3390/w13141968
Australian Rainfall and Runoff (2019) A Guide to Flood Estimation, Book 6 - Flood Hydraulics
Basheer TA, Wayayok A, Yusuf B, Rowshon MK, Basheer TA, Kamal R (2017) Dam breach parameters and their influence on flood hydrographs for Mosul dam. Article J Eng Sci Technol 12(11):2896–2908. https://www.researchgate.net/publication/328475986
Google Scholar
BGHPP Development Committee (2014a) Feasibility Study And Detailed Design of Budhi Gandaki HPP, Vol. 2B: Assessment of Downstream Impacts
BGHPP Development Committee (2014b) Feasibility Study and Detailed Design of Budhigandaki HPP Vol. 2A: Hydrological and Meteorological Analysis
Bricker JD, Schwanghart W, Adhikari BR, Moriguchi S, Roeber V, Giri S (2017) Performance of models for Flash Flood warning and Hazard Assessment: the 2015 Kali Gandaki Landslide Dam Breach in Nepal. Mt Res Dev 37(1):5–15. https://doi.org/10.1659/MRD-JOURNAL-D-16-00043.1
Article Google Scholar
C Froehlich D (1995) Embankment dam breach parameters revisited. Int Water Resour Eng Conf - Proc 1(January 1995):887–891
Cental Bureau of Statisitics (2016) Statistical Pocket Book of Nepal . www.cbs.gov.np
Dawson RJ, Ball T, Werritty J, Werritty A, Hall JW, Roche N (2011) Assessing the effectiveness of non-structural flood management measures in the Thames Estuary under conditions of socio-economic and environmental change. Glob Environ Change 21(2):628–646. https://doi.org/10.1016/j.gloenvcha.2011.01.013
Department of Electricity Development (2006) Design Guidelines for Headworks of Hydropower Projects . https://www.doed.gov.np/pages/guidelines-and-manuals
Dincergok T (2007) The role of dam safety in dam-break induced flood management. International Conference on River Basin Management , 683–691
Ettema R, Baker ME, Teal M, Trojanowski J (2021) Ice-Run Destruction of Spencer Dam on Nebraska’s Niobrara River. CGU HS Committee on River Ice Processes and the Environment, 21st Workshop on the Hydraulics of Ice-Covered Rivers, Saskatoon, Saskatchewan, Canada
Fang CH, Chen J, Duan YH, Xiao K (2017) A new method to quantify breach sizes for the flood risk management of concrete arch dams. J Flood Risk Manag 10(4):511–521. https://doi.org/10.1111/jfr3.12240
Federal Energy Regulatory Commission (FERC) (1993) FERC Engineering Guidelines
FERC (2001) FERC Engineering Guidelines for the Evaluation of Hydropower Projects
Government of Nepal (2020) The Fifteenth Plan (Fiscal Year 2019/20–2023/24) . www.npc.gov.np
Gyawali DR, Devkota LP (2015) Dam Break Analysis using HEC-RAS: A Case Study of Proposed Koshi High Dam. Proceedings of the Seminar on Water and Sustainable Development
Hershfield DM (1965) Method for estimating probable Maximum Precipitation. J Am Waterworks Association 57:965–972
Independent Forensic Team (2022) Final Investigation of Failures of Edenville and Sanford Dams
International Strategy for Disaster Reduction (2005) Hyogo framework for action 2005–2015. World Conference on Disaster Reduction , January 2005 , 508–516. https://doi.org/10.1007/978-1-4020-4399-4_180
Lempérière F (2017) Dams and floods. Elsevier 3(1):144–149. https://doi.org/10.1016/J.ENG.2017.01.018
Leng Q, Zhang M, Zhao G, Mao S, Jiang A (2023) Simulation and Hazard Map of flooding caused by the Break of a concrete gravitational dam. Lecture Notes Civil Eng 264 LNCE:1248–1260. https://doi.org/10.1007/978-981-19-6138-0_109
Nepal Electricity Authority (2022) Nepal Electricity Authority, A Year in Review- Fiscal Year 2021/2022 . www.nea.org.np
Office of the State Engineer (2020) Guidelines for Dam Breach Analysis
Pandey BR, Knoblauch H, Zenz G (2023) Potential dam Breach Flood Hazard Assessment of Kulekhani Reservoir Rock fill dam using 2D diffusion and full dynamic shallow water equation defining Coriolis Effect. Preprints Org. https://doi.org/10.20944/preprints202311.0088.v1
Shah MAR, Rahman A, Chowdhury SH (2018) Challenges for achieving sustainable flood risk management. J Flood Risk Manag 11:S352–S358. https://doi.org/10.1111/jfr3.12211
Shugar DH, Jacquemart M, Shean D, Bhushan S, Upadhyay K, Sattar A, Schwanghart W, McBride S, van Wyk de Vries M, Mergili M, Emmer A, Deschamps-Berger C, McDonnell M, Bhambri R, Allen S, Berthier E, Carrivick JL, Clague JJ, Dokukin M, Westoby MJ (2021) A massive rock and ice avalanche caused the 2021 disaster at Chamoli. Indian Himalaya Sci 373(6552):300–306. https://doi.org/10.1126/science.abh4455
Article CAS Google Scholar
Singh KP, Snorrason A (1984) Sensitivity of outflow peaks and flood stages to the selection of dam breach parameters and simulation models. J Hydrol 68(1–4):295–310. https://doi.org/10.1016/0022-1694(84)90217-8
United Nations (2015) Sendai Framework for Disaster Risk Reduction. In UN World Conference
United States Department of Interior (1988) Downstream Hazard Classification Guidelines. In ACER Technical Memorandum No. 11, Assisstant Commissioner - Engineering and Research, Denver, Colorado
USACE (2014) Using HEC-RAS for Dam Break Studies . www.hec.usace.army.mil
USACE (2018) Hydrologic Engineering Requirements for Reservoirs Engineer Manual
USACE (2024) HEC-RAS River Analysis System HEC-RAS Hydraulic Reference Manual
Vincent E, Emeka OM, Dominic P (2020) Dam and its Failure: A Brief Review of some selected Dams around the World. Adamawa State University Journal of Scientific Research , 8 . http://www.adsujsr.com
Wahyudi E (2004) Dam Break Analysis; A Case Study
World Conference on Natural Disaster Reduction (1994) Yokohama Strategy and Plan of Action for a Safer World: Guidelines for Natural Disaster Prevention, Preparedness and Mitigation. World Conference on Natural Disaster Reduction Yokohama, Japan, 23–27 May 1994 , May 1994 , 23–27
World Meteorological Organization (2009) Manual on estimation of probable maximum precipitation (PMP)
Zhang L, Peng M, Chang D, Xu Y (2016) Statistical Analysis of Failures of Concrete Dams. In Dam Failure Mechanisms and Risk Assessment . https://doi.org/10.1002/9781118558522.ch4
Download references

Acknowledgements
We wish to express my very deepest thanks and gratitude to Mr. Shreeram Shrestha, Civil Engineer, Chilime Hydropower Company Limited, Nepal for his continuous guidance, inspiration and encouragement during the initial preparation of building HEC-RAS model to result interpretation and completion of this study.
This research received no specific grant from any funding agency in the public, commercial, or not-for-profit sectors.
Author information
Authors and affiliations.
Nepal Engineering College, Pokhara University, Bhaktapur, Nepal
Institute for Life Sciences and the Environment, University of Southern Queensland, Toowoomba, Australia
Utsav Bhattarai
Centre for Water Resources Studies, Institute of Engineering, Tribhuvan University, Lalitpur, Nepal
Vishnu Prasad Pandey
Department of Civil Engineering, Pulchowk Campus, Institute of Engineering, Tribhuvan University, Lalitpur, Nepal
Pawan Kumar Bhattarai
You can also search for this author in PubMed Google Scholar
Contributions
A.A. and P.K.B. devised the project, the main conceptual ideas, and the proof outline. A.A. worked out almost all of the technical details, prepared figures, and performed the model analysis for the suggested topics. A.A., P.K.B, and U.B. verified the numerical results. A.A. and V.P.P. interpreted the Results. A.A. with the help of U.B., P.K.B., and V.P.P. wrote the manuscript. U.B., P.K.B., and V.P.P. worked on the discussion of results and commented on the manuscript. A.A. finalizes the manuscript after all the edits.
Corresponding author
Correspondence to Pawan Kumar Bhattarai .
Ethics declarations
Competing interests.
The authors declare no competing interests.
Additional information
Publisher’s note.
Springer Nature remains neutral with regard to jurisdictional claims in published maps and institutional affiliations.
Rights and permissions
Open Access This article is licensed under a Creative Commons Attribution-NonCommercial-NoDerivatives 4.0 International License, which permits any non-commercial use, sharing, distribution and reproduction in any medium or format, as long as you give appropriate credit to the original author(s) and the source, provide a link to the Creative Commons licence, and indicate if you modified the licensed material. You do not have permission under this licence to share adapted material derived from this article or parts of it. The images or other third party material in this article are included in the article’s Creative Commons licence, unless indicated otherwise in a credit line to the material. If material is not included in the article’s Creative Commons licence and your intended use is not permitted by statutory regulation or exceeds the permitted use, you will need to obtain permission directly from the copyright holder. To view a copy of this licence, visit http://creativecommons.org/licenses/by-nc-nd/4.0/ .
Reprints and permissions
About this article
Cite this article.
Awal, A., Bhattarai, U., Pandey, V.P. et al. Downstream impacts of dam breach using HEC-RAS: a case of Budhigandaki concrete arch dam in central Nepal. Environ Syst Res 13 , 37 (2024). https://doi.org/10.1186/s40068-024-00358-3
Download citation
Received : 31 March 2024
Accepted : 16 July 2024
Published : 01 September 2024
DOI : https://doi.org/10.1186/s40068-024-00358-3
Share this article
Anyone you share the following link with will be able to read this content:
Sorry, a shareable link is not currently available for this article.
Provided by the Springer Nature SharedIt content-sharing initiative
- Downstream impacts
- Budhigandaki dam
Advertisement
Environmental dynamics of rainfall patterns: a comparative analysis of intensity-duration-frequency curves of metropolitan cities in Pakistan
- Published: 06 September 2024
Cite this article
- Safeera Zaineb 1 &
- Muzaffar Bashir 1
The analysis of extreme rainfall parameters, particularly rainfall intensities, plays a serious role in the protection, productivity, and resilience of hydrological systems against storms and floods. This is especially important in arid and semi-arid regions like Pakistan, where inclusive long-term rainfall data with short aggregation periods is limited. Addressing this need, the current study develops intensity-duration-frequency (IDF) curves using rainfall data from four cities across different elevations and geographical regions within Pakistan. By statistically fitting the Gumbel distribution to observed data at different durations (1 h, 6 h, 12 h, and 24 h), the study originates rainfall intensities for distinct return periods. The analysis discloses an average annual rainfall of 25.42 mm, 9.62 mm, 9.25 mm, and 28.02 mm, with standard deviations of 6.45 mm, 9.67 mm, 7.50 mm, and 11.96 mm for Lahore, Karachi, Quetta, and Peshawar, respectively, based on data from 2001 to 2022. Notably, the assessed rainfall intensities for various return periods (2, 5, 10, and 25 years) are higher in mountainous regions compared to interior and coastal regions. Additionally, the study develops empirical parameters for the IDF formula for each city through a linear regression technique, allowing the prediction of rainfall intensities based on desired return periods. Finally, contour maps for all the parameters were created, which can be used to determine IDF relationships for un-gauged locations. These outcomes underscore the vulnerability of mountainous regions to extreme rainfall events, focus the necessity for updated infrastructure and robust flood management strategies. The derived IDF curves and empirical parameters offer valuable tools for policymakers and urban planners to plan effective interventions aimed at mitigating the adverse impacts of extreme rainfall in Pakistan.
This is a preview of subscription content, log in via an institution to check access.
Access this article
Subscribe and save.
- Get 10 units per month
- Download Article/Chapter or eBook
- 1 Unit = 1 Article or 1 Chapter
- Cancel anytime
Price includes VAT (Russian Federation)
Instant access to the full article PDF.
Rent this article via DeepDyve
Institutional subscriptions
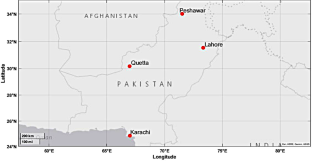
Data availability
The data that support the findings of this study are openly available at https://power.larc.nasa.gov/data-access-viewer/ .
Agakpe MD, Nyatuame M, Ampiaw F (2024) Development of intensity–duration–frequency (IDF) curves using combined rain gauge (RG) and remote sense (TRMM) datasets for Weta Traditional Area in Ghana. HydroResearch 7:109–121
Article Google Scholar
Ahmed R, Ali S (2016) Modelling of intensity-duration-frequency curves for Lahore City using frequency analysis. 725–733. https://doi.org/10.2495/SDP160611
AL-Areeq A, Al-Zahrani M, Chowdhury S (2021) Rainfall intensity–duration–frequency (IDF) curves: effects of uncertainty on Flood Protection and Runoff quantification in Southwestern Saudi Arabia. Arab J Sci Eng 46(11):10993–11007. https://doi.org/10.1007/s13369-021-06142-0
Article CAS Google Scholar
AlHassoun SA (2011) Developing an empirical formulae to estimate rainfall intensity in Riyadh region. J King Saud university-engineering Sci 23(2):81–88
Alzahrani F, Seidou O, Alodah A (2023) Assessing the performance of daily to subdaily temporal disaggregation methods for the IDF curve generation under climate change. J Water Clim Change 14(4):1339–1357. https://doi.org/10.2166/wcc.2023.507
Ashok Kumar K, Sudheer KVS, Pavani K et al (2023) Extreme Rainfall Analysis for Development of Rainfall Intensity Duration Frequency Curves for Semiarid Region of Andhra Pradesh in India. Natl Acad Sci Lett
Basumatary V, Sil BS (2018) Generation of rainfall intensity-duration-frequency curves for the Barak River Basin. Meteorology Hydrology and Water Management. Research and Operational Applications , 6
Bell FC (1969) Generalized rainfall - duration - frequency relationships. J Hydraulics Div 95(1):311–328. https://doi.org/10.1061/JYCEAJ.0001942
Bernard MM (1932) Formulas for rainfall intensities of long duration. Trans Am Soc Civ Eng 96(1):592–606. https://doi.org/10.1061/TACEAT.0004323
Cheng L, AghaKouchak A (2014) Nonstationary Precipitation intensity-duration-frequency curves for infrastructure design in a changing climate. Sci Rep 4(1):7093. https://doi.org/10.1038/srep07093
Chitrakar P, Sana A, Hamood N, Almalki S (2023) Regional distribution of intensity–duration–frequency (IDF) relationships in Sultanate of Oman. J King Saud Univ - Sci 35(7):102804. https://doi.org/10.1016/j.jksus.2023.102804
Chow VT (1953) Frequency analysis of hydrologic data, with a special application to rainfall intensities. Illinois University of Illinois Engineering Experiment Station, Urbana
Google Scholar
Chow VT, Maidment DR, Mays LW (1988) Applied Hydrology. McGraw-Hill, New York
Cook LM, McGinnis S, Samaras C (2020) The effect of modeling choices on updating intensity-duration-frequency curves and stormwater infrastructure designs for climate change. Clim Change 159(2):289–308. https://doi.org/10.1007/s10584-019-02649-6
Creaco E (2024) Scaling models of intensity–duration–frequency (IDF) curves based on adjusted design event durations. J Hydrol, 130847
de Bodas Terassi PM, Pontes PRM, Xavier ACF, Cavalcante RBL, de Oliveira Serrão EA, Sobral BS, Baratto J (2023) A comprehensive analysis of regional disaggregation coefficients and intensity-duration-frequency curves for the Itacaiúnas watershed in the eastern Brazilian Amazon. Theoret Appl Climatol 154(3):863–880
Gumbel EJ (1958) Statistics of extremes. Columbia University. https://doi.org/10.7312/gumb92958
Gunawardhan LN, Al-Rawas GA (2016) A comparison of trends in Extreme Rainfall using 20-Year Data in three Major cities in Oman. J Eng Res [TJER] 13(2):137. https://doi.org/10.24200/tjer.vol13iss2pp137-148
Haddad K, Rahman A (2011) Selection of the best fit flood frequency distribution and parameter estimation procedure: a case study for Tasmania in Australia. Stoch Env Res Risk Assess 25(3):415–428
Kourtis IM, Nalbantis I, Tsakiris G, Psiloglou BΕ, Tsihrintzis VA (2023) Updating IDF curves under Climate Change: impact on Rainfall-Induced Runoff in Urban basins. Water Resour Manage 37(6–7):2403–2428. https://doi.org/10.1007/s11269-022-03252-8
Mahmoudi MR, Eslamian S, Soltani S, Tahanian M (2023) Regionalization of rainfall intensity–duration–frequency (IDF) curves with L-moments method using neural gas networks. Theoret Appl Climatol 151(1):1–11
Mirhosseini G, Srivastava P, Fang X (2016) Developing rainfall intensity-duration-frequency (IDF) curves for Alabama under future climate scenarios using regional climate models. Hydrol Earth Syst Sci 20(2):477–492
Mohamadi S, Bagheri R (2022) Hydrological response of a paired watershed to rainfall storm events in arid region: a study in Dehgin of Hormozgan province, Iran. Environ Sci Pollut Res 29(53):80831–80848. https://doi.org/10.1007/s11356-022-21543-w
Noor M, Ismail T, Shahid S, Asaduzzaman M, Dewan A (2021) Evaluating intensity-duration-frequency (IDF) curves of satellite-based precipitation datasets in Peninsular Malaysia. Atmos Res 248:105203
Noor M, Ismail T, Shahid S, Asaduzzaman M, Dewan A (2022) Projection of rainfall intensity-duration-frequency curves at ungauged location under climate change scenarios. Sustainable Cities Soc 83:103951. https://doi.org/10.1016/j.scs.2022.103951
Onarun T, Thepprasit C, Sittichok K (2023) Development of statistical downscaling methods for the assessment of rainfall characteristics under climate change scenarios. J Water Clim Change. https://doi.org/10.2166/wcc.2023.490
Rasool U, Yin X, Xu Z, Padulano R, Rasool MA, Siddique MA, Hassan MA, Senapathi V (2023) Rainfall-driven machine learning models for accurate flood inundation mapping in Karachi, Pakistan. Urban Clim 49:101573. https://doi.org/10.1016/j.uclim.2023.101573
Sathish S, Chanu S, Sadath R, Elango L (2022) Impacts of regional climate model projected rainfall, sea level rise, and urbanization on a coastal aquifer. Environ Sci Pollut Res 29(22):33305–33322. https://doi.org/10.1007/s11356-021-18213-8
Siamaki M, Safavi HR, Klaho MH (2024) Extraction of intensity-duration for short-term extreme rainfalls from daily and yearly extreme rainfalls using copula functions. Theoret Appl Climatol, 1–19
Takeleb AM, Fajriani QR, Ximenes MA (2022) Determination of Rainfall Intensity Formula and Intensity Duration frequency (IDF) curve at the Quelicai Administrative Post, Timor Leste. Timor-Leste J Eng Sci 3:1–10
Tfwala CM, Van Rensburg LD, Schall R, Mosia SM, Dlamini P (2017) Precipitation intensity-duration-frequency curves and their uncertainties for Ghaap plateau. Clim Risk Manage 16:1–9
Willems P (2013) Revision of urban drainage design rules after assessment of climate change impacts https//. https://doi.org/10.2166/wcc.2023.430skkkkkkkkk
Yamoat N, Hanchoowong R, Sriboonlue S, Kangrang A (2022) Temporal change of extreme precipitation intensity–duration–frequency relationships in Thailand. J Water Clim Change 13(2):839–853
Yamoat N, Hanchoowong R, Yamoad O, Chaimoon N, Kangrang A (2023) Estimation of regional intensity–duration–frequency relationships of extreme rainfall by simple scaling in Thailand. J Water Clim Change 14(3):796–810
Yan H, Moradkhani H, Zhao S (2017) Hydrologic uncertainty Assessment using a bayesian multivariate distribution. Water Resour Res 53(12):10982–11006
Download references
Acknowledgements
We are thankful to PU, Lahore, Pakistan. Many thanks go to Atif Mahmood and Ahsan Javed for their support.
This study has no funding information to declare.
Author information
Authors and affiliations.
Smart Computing and Applied Sciences group, Department of Physics, University of the Punjab, Lahore, Pakistan
Safeera Zaineb & Muzaffar Bashir
You can also search for this author in PubMed Google Scholar
Contributions
All authors contributed equally.
Corresponding author
Correspondence to Muzaffar Bashir .
Ethics declarations
Ethical approval.
Not required.
Consent to Participate
All authors declare consent of participation in this research.
Consent to Publish
All authors declare consent to publish this research.
Competing interests
The authors declare no competing interests.
Additional information
Publisher’s note.
Springer Nature remains neutral with regard to jurisdictional claims in published maps and institutional affiliations.
Rights and permissions
Springer Nature or its licensor (e.g. a society or other partner) holds exclusive rights to this article under a publishing agreement with the author(s) or other rightsholder(s); author self-archiving of the accepted manuscript version of this article is solely governed by the terms of such publishing agreement and applicable law.
Reprints and permissions
About this article
Zaineb, S., Bashir, M. Environmental dynamics of rainfall patterns: a comparative analysis of intensity-duration-frequency curves of metropolitan cities in Pakistan. Theor Appl Climatol (2024). https://doi.org/10.1007/s00704-024-05166-4
Download citation
Received : 02 July 2024
Accepted : 20 August 2024
Published : 06 September 2024
DOI : https://doi.org/10.1007/s00704-024-05166-4
Share this article
Anyone you share the following link with will be able to read this content:
Sorry, a shareable link is not currently available for this article.
Provided by the Springer Nature SharedIt content-sharing initiative
- Find a journal
- Publish with us
- Track your research
Information
- Author Services
Initiatives
You are accessing a machine-readable page. In order to be human-readable, please install an RSS reader.
All articles published by MDPI are made immediately available worldwide under an open access license. No special permission is required to reuse all or part of the article published by MDPI, including figures and tables. For articles published under an open access Creative Common CC BY license, any part of the article may be reused without permission provided that the original article is clearly cited. For more information, please refer to https://www.mdpi.com/openaccess .
Feature papers represent the most advanced research with significant potential for high impact in the field. A Feature Paper should be a substantial original Article that involves several techniques or approaches, provides an outlook for future research directions and describes possible research applications.
Feature papers are submitted upon individual invitation or recommendation by the scientific editors and must receive positive feedback from the reviewers.
Editor’s Choice articles are based on recommendations by the scientific editors of MDPI journals from around the world. Editors select a small number of articles recently published in the journal that they believe will be particularly interesting to readers, or important in the respective research area. The aim is to provide a snapshot of some of the most exciting work published in the various research areas of the journal.
Original Submission Date Received: .
- Active Journals
- Find a Journal
- Proceedings Series
- For Authors
- For Reviewers
- For Editors
- For Librarians
- For Publishers
- For Societies
- For Conference Organizers
- Open Access Policy
- Institutional Open Access Program
- Special Issues Guidelines
- Editorial Process
- Research and Publication Ethics
- Article Processing Charges
- Testimonials
- Preprints.org
- SciProfiles
- Encyclopedia
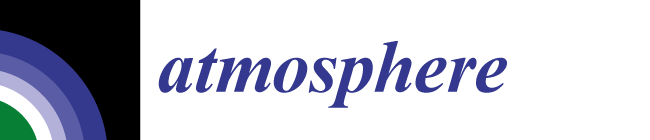
Article Menu

- Subscribe SciFeed
- Recommended Articles
- Google Scholar
- on Google Scholar
- Table of Contents
Find support for a specific problem in the support section of our website.
Please let us know what you think of our products and services.
Visit our dedicated information section to learn more about MDPI.
JSmol Viewer
Deriving tropical cyclone-associated flood hazard information using clustered gpm-imerg rainfall signatures: case study in dominica.
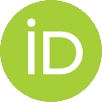
1. Introduction
2. materials and methods, 2.1. data description, 2.2. spatial partitional clustering using k-means, 2.3. temporal alignment and quantile analysis, 2.4. flood modelling, 2.5. case study, 3.1. results of the spatial partitional clustering, 3.2. cluster representative precipitation signals, 3.3. results for the flooding simulations, 3.3.1. rpss from k = 5, 3.3.2. rpss from k = 4 and k = 3, 3.4. selection of final rainfall scenarios for ts erika, 3.5. comparison, 4. discussion: final rainfall scenarios for ts erika, 5. conclusions, author contributions, institutional review board statement, informed consent statement, data availability statement, conflicts of interest.
= 5 | ||||||
Cluster | T1 | T2 | T3 | T4 | T5 | |
Cluster size | 132 | 798 | 433 | 399 | 149 | |
% | 6.9 | 41.8 | 22.7 | 20.9 | 7.8 | |
Maximum intensity (mm/h) | min | 55 | 0.4 | 36.4 | 18.2 | 32 |
max | 120 | 50.2 | 120 | 111 | 120 | |
Total rainfall (mm) | min | 451.5 | 1.4 | 201.1 | 47.8 | 121 |
max | 767.9 | 112.4 | 571.5 | 275.9 | 603.2 | |
mean | 583.9 | 29.4 | 344.2 | 167.5 | 331.4 | |
= 4 | ||||||
Cluster | T1 | T2 | T3 | T4 | ||
Cluster size | 146 | 818 | 513 | 434 | ||
% | 7.6 | 42.8 | 26.8 | 22.7 | ||
Maximum intensity (mm/h) | min | 55 | 0.4 | 42 | 18.8 | |
max | 120 | 50.2 | 120 | 111 | ||
Total rainfall (mm) | min | 433.7 | 1.4 | 202.2 | 47.8 | |
max | 767.9 | 132.1 | 571.5 | 275.9 | ||
mean | 574.3 | 31.1 | 347.6 | 180.1 | ||
= 3 | ||||||
Cluster | T1 | T2 | T3 | |||
Cluster size | 212 | 937 | 762 | |||
% | 11.1 | 49.0 | 39.9 | |||
Maximum intensity (mm/h) | min | 55 | 0.4 | 26 | ||
max | 120 | 68.6 | 120 | |||
Total rainfall (mm) | min | 352.5 | 1.4 | 83.6 | ||
max | 767.9 | 171.9 | 489.2 | |||
mean | 530.8 | 42.7 | 279.8 |
RPS | ||||
Flood extent (km ) | ||||
Q | 3.98 | 3.12 | 1.02 | 1.40 |
Q | 4.84 | 3.76 | 2.19 | 3.12 |
Flood depth (m) | ||||
Q | 3.88 | 2.79 | 1.26 | 1.32 |
Q | 4.21 | 3.70 | 1.69 | 2.85 |
Flood volume (million m ) | ||||
Q | 1.67 | 1.10 | 0.42 | 0.56 |
Q | 2.28 | 1.50 | 0.77 | 1.12 |
Runoff ratio | ||||
Q | 0.80 | 0.71 | 0.33 | 0.43 |
Q | 0.85 | 0.77 | 0.59 | 0.74 |
Infiltration (mm) | ||||
Q | 92.14 | 75.31 | 60.83 | 77.82 |
Q | 97.42 | 86.61 | 73.03 | 98.50 |
Flood duration (h) | ||||
Q | 18.43 | 15.71 | 16.68 | 23.64 |
Q | 27.24 | 18.85 | 22.25 | 18.60 |
Click here to enlarge figure
- Acevedo, S. Gone with the Wind: Estimating Hurricane and Climate Change Costs in the Caribbean ; Working Paper No. 2016/199; International Monetary Fund: Washington, DC, USA, 2016. [ Google Scholar ]
- Nurse, L.A.; McLean, R.F.; Agard Trinidad, J.; Pascal Briguglio, L.; Duvat-Magnan, V.; Pelesikoti, N.; Tompkins, E.; Webb, A. Small Islands. In Climate Change 2014: Impacts, Adaptation, and Vulnerability. Part B: Regional Aspects. Contribution of Working Group II to the Fifth Assessment Report of the Intergovernmental Panel on Climate Change ; Barros, V.R., Field, C., Dokken, D.J., Mastrandrea, M.D., Dokken, D.J., Mach, K.J., Bilir, T.E., Chatterjee, M., Ebi, K.L., Estrada, Y.O., et al., Eds.; Cambridge University Press: Cambridge, UK; New York, NY, USA, 2014; pp. 1613–1654. [ Google Scholar ]
- Wilkinson, E.; Arvis, B.; Mendler de Suarez, J.; Weingärtner, L.; Jaime, C.; Grainger, N.; Simonet, C.; Bazo, J.; Kruczkiewicz, A. Preparing for Extreme Weather in the Eastern Caribbean ; Working Paper 603; Overseas Development Institute: London, UK, 2021. [ Google Scholar ]
- Nugent, A.D.; Rios-Berrios, R. Factors Leading to Extreme Precipitation on Dominica from Tropical Storm Erika (2015). Mon. Weather. Rev. 2018 , 146 , 525–541. [ Google Scholar ] [ CrossRef ]
- Pasch, R.J.; Penny, A.B.; Berg, R. Hurricane Maria (AL152017) ; National Oceanic and Atmospheric Administration: Miami, FL, USA, 2017. [ Google Scholar ]
- Pasch, R.J.; Penny, A.B. Tropical Storm Erika (AL052015) ; National Oceanic and Atmospheric Administration: Miami, FL, USA, 2015. [ Google Scholar ]
- NOAA. How Do Hurricanes Form? National Oceanic and Atmospheric Adminicstration: Miami, FL, USA, 2024. [ Google Scholar ]
- Lin, Y.; Zhao, M.; Zhang, M. Tropical Cyclone Rainfall Area Controlled by Relative Sea Surface Temperature. Nat. Commun. 2015 , 6 , 6591. [ Google Scholar ] [ CrossRef ]
- Lonfat, M.; Rogers, R.; Marchok, T.; Marks, F.D. A Parametric Model for Predicting Hurricane Rainfall. Mon. Weather. Rev. 2007 , 135 , 3086–3097. [ Google Scholar ] [ CrossRef ]
- Ybañez, R. Understanding Rainfall Return Periods Understanding Rainfall Return Periods ; University of the Philippines: Diliman, Quezon City, Philippines, 2013; Volume 1. [ Google Scholar ]
- De Risi, R.; Jalayer, F.; De Paola, F.; Carozza, S.; Yonas, N.; Giugni, M.; Gasparini, P. From Flood Risk Mapping toward Reducing Vulnerability: The Case of Addis Ababa. Nat. Hazards 2020 , 100 , 387–415. [ Google Scholar ] [ CrossRef ]
- Jetten, V.G. CHaRIM Project Dominica National Flood Hazard Map Methodology and Validation Report ; University of Twente: Enschede, The Netherlands, 2016. [ Google Scholar ]
- DMS. Climatology for Canefield Airport 1982–2011 ; Dominica Meteorological Service: Canefield, Dominica, 2012. [ Google Scholar ]
- Smith, R.B.; Schafer, P.; Kirshbaum, D.J.; Regina, E. Orographic Precipitation in the Tropics: Experiments in Dominica. J. Atmos. Sci. 2009 , 66 , 1698–1716. [ Google Scholar ] [ CrossRef ]
- Pollock, M.D.; O’Donnell, G.; Quinn, P.; Dutton, M.; Black, A.; Wilkinson, M.E.; Colli, M.; Stagnaro, M.; Lanza, L.G.; Lewis, E.; et al. Quantifying and Mitigating Wind-Induced Undercatch in Rainfall Measurements. Water Resour. Res. 2018 , 54 , 3863–3875. [ Google Scholar ] [ CrossRef ]
- Veneziano, D.; Villani, P. Best Linear Unbiased Design Hyetograph. Water Resour. Res. 1999 , 35 , 2725–2738. [ Google Scholar ] [ CrossRef ]
- Bezak, N.; Šraj, M.; Rusjan, S.; Mikoš, M. Impact of the Rainfall Duration and Temporal Rainfall Distribution Defined Using the Huff Curves on the Hydraulic Flood Modelling Results. Geosciences 2018 , 8 , 69. [ Google Scholar ] [ CrossRef ]
- Balbastre-Soldevila, R.; García-Bartual, R.; Andrés-Doménech, I. A Comparison of Design Storms for Urban Drainage System Applications. Water 2019 , 11 , 757. [ Google Scholar ] [ CrossRef ]
- Chow, V.T.; Maidment, D.R.; Mays, L.W. Applied Hydrology ; McGraw-Hill: New York, NY, USA, 1998. [ Google Scholar ]
- Lumbroso, D.M.; Boyce, S.; Bast, H.; Walmsley, N. The Challenges of Developing Rainfall Intensity-Duration-Frequency Curves and National Flood Hazard Maps for the Caribbean. J. Flood Risk Manag. 2011 , 4 , 42–52. [ Google Scholar ] [ CrossRef ]
- Richard, C.Y.L.; Zhou, W.; Lee, T.C. Climatological Characteristics and Observed Trends of Tropical Cyclone–Induced Rainfall and Their Influences on Long-Term Rainfall Variations in Hong Kong. Mon. Weather. Rev. 2015 , 143 , 2192–2206. [ Google Scholar ]
- Huffman, G.J.; Bolvin, D.T.; Braithwaite, D.; Hsu, K.L.; Joyce, R.J.; Kidd, C.; Nelkin, E.J.; Sorooshian, S.; Stocker, E.F.; Tan, J.; et al. Integrated Multi-Satellite Retrievals for the Global Precipitation Measurement (GPM) Mission (IMERG). Adv. Glob. Chang. Res. 2020 , 67 , 343–353. [ Google Scholar ]
- Adhikari, A.; Liu, C.; Hayden, L. Uncertainties of GPM Microwave Imager Precipitation Estimates Related to Precipitation System Size and Intensity. J. Hydrometeorol. 2019 , 20 , 1907–1923. [ Google Scholar ] [ CrossRef ]
- Wang, F. Temporal Pattern Analysis of Local Rainstorm Events in China During the Flood Season Based on Time Series Clustering. Water 2020 , 12 , 725. [ Google Scholar ] [ CrossRef ]
- Cristiano, E.; ten Veldhuis, M.-C.; Gaitan, S.; Ochoa Rodriguez, S.; van de Giesen, N. Critical Scales to Explain Urban Hydrological Response: An Application in Cranbrook, London. Hydrol. Earth Syst. Sci. 2018 , 22 , 2425–2447. [ Google Scholar ] [ CrossRef ]
- Santos, C.A.G.; Neto, R.M.B.; da Silva, R.M.; Costa, S.G.F. Cluster Analysis Applied to Spatiotemporal Variability of Monthly Precipitation over Paraíba State Using Tropical Rainfall Measuring Mission (TRMM) Data. Remote Sens. 2019 , 11 , 637. [ Google Scholar ] [ CrossRef ]
- Ogden, F.L. Evidence of Equilibrium Peak Runoff Rates in Steep Tropical Terrain on the Island of Dominica during Tropical Storm Erika, August 27, 2015. J. Hydrol. 2016 , 542 , 35–46. [ Google Scholar ] [ CrossRef ]
- DMS. Rainfall Data on Tropical Storm, 26th to 27th August, 2015 ; Dominica Meteorological Service: Canefield, Dominica, 2015. [ Google Scholar ]
- GoCD. Rapid Damage and Impact Assessment Tropical Storm Erika-August 27, 2015 ; Government of the Commonwealth of Dominica: Roseau, Dominica, 2015. [ Google Scholar ]
- EM-DAT. Inventorying Hazards & Disasters Worldwide Since 1988 ; EM-DAT: Brussels, Belgium, 2024. [ Google Scholar ]
- Le, M.H.; Lakshmi, V.; Bolten, J.; Bui, D.D. Adequacy of Satellite-Derived Precipitation Estimate for Hydrological Modeling in Vietnam Basins. J. Hydrol. 2020 , 586 , 124820. [ Google Scholar ] [ CrossRef ]
- Wang, Z.; Zhong, R.; Lai, C.; Chen, J. Evaluation of the GPM IMERG Satellite-Based Precipitation Products and the Hydrological Utility. Atmos. Res. 2017 , 196 , 151–163. [ Google Scholar ] [ CrossRef ]
- Mekonnen, K.; Melesse, A.M.; Woldesenbet, T.A. Spatial Evaluation of Satellite-Retrieved Extreme Rainfall Rates in the Upper Awash River Basin, Ethiopia. Atmos. Res. 2021 , 249 , 105297. [ Google Scholar ] [ CrossRef ]
- Cui, W.; Dong, X.; Xi, B.; Feng, Z.; Fan, J. Can the GPM IMERG Final Product Accurately Represent MCSs’ Precipitation Characteristics over the Central and Eastern United States? J. Hydrometeorol. 2020 , 21 , 39–57. [ Google Scholar ] [ CrossRef ]
- Tang, S.; Li, R.; He, J.; Wang, H.; Fan, X.; Yao, S. Comparative Evaluation of the GPM IMERG Early, Late, and Final Hourly Precipitation Products Using the CMPA Data over Sichuan Basin of China. Water 2020 , 12 , 554. [ Google Scholar ] [ CrossRef ]
- Qi, W.; Yong, B.; Gourley, J.J. Monitoring the Super Typhoon Lekima by GPM-Based Near-Real-Time Satellite Precipitation Estimates. J. Hydrol. 2021 , 603 , 126968. [ Google Scholar ] [ CrossRef ]
- NASA. NASA Finds Heavy Rain in New Tropical Cyclone Hola near Vanuatu ; Goddard Space Flight Center: Greenbelt, MD, USA, 2018. [ Google Scholar ]
- Omranian, E.; Sharif, H.O.; Tavakoly, A.A. How Well Can Global Precipitation Measurement (GPM) Capture Hurricanes? Case Study: Hurricane Harvey. Remote Sens. 2018 , 10 , 1150. [ Google Scholar ] [ CrossRef ]
- NASA. IMERG: Integrated Multi-satellitE Retrievals for GPM | NASA Global Precipitation Measurement Mission ; Goddard Space Flight Canter: Greenbelt, MD, USA, 2023. [ Google Scholar ]
- Landsea, C.W.; Franklin, J.L. Atlantic Hurricane Database Uncertainty and Presentation of a New Database Format. Mon. Weather. Rev. 2013 , 141 , 3576–3592. [ Google Scholar ] [ CrossRef ]
- Kisilevich, S.; Mansmann, F.; Nanni, M.; Rinzivillo, S. Spatio-Temporal Clustering. In Data Mining and Knowledge Discovery Handbook ; Springer: Boston, MA, USA, 2009; pp. 855–874. [ Google Scholar ]
- Wu, X.; Cheng, C.; Zurita-Milla, R.; Song, C. An Overview of Clustering Methods for Geo-Referenced Time Series: From One-Way Clustering to Co- and Tri-Clustering. Int. J. Geogr. Inf. Sci. 2020 , 34 , 1822–1848. [ Google Scholar ] [ CrossRef ]
- Machiwal, D.; Dayal, D.; Kumar, S. Long-Term Rainfall Trends and Change Points in Hot and Cold Arid Regions of India. Hydrol. Sci. J. 2017 , 62 , 1050–1066. [ Google Scholar ] [ CrossRef ]
- Hadi, A.F.; Yudistira, I.; Anggraeni, D.; Hasan, M. The Geographical Clustering of The Rainfall Stations on Seasonal GSTAR Modeling for Rainfall Forecasting. J. Phys. Conf. Ser. 2018 , 1028 , 012238. [ Google Scholar ] [ CrossRef ]
- Alam, M.S.; Paul, S. A Comparative Analysis of Clustering Algorithms to Identify the Homogeneous Rainfall Gauge Stations of Bangladesh. J. Appl. Stat. 2019 , 47 , 1460–1481. [ Google Scholar ] [ CrossRef ]
- Naranjo-Fernández, N.; Guardiola-Albert, C.; Aguilera, H.; Serrano-Hidalgo, C.; Montero-González, E. Clustering Groundwater Level Time Series of the Exploited Almonte-Marismas Aquifer in Southwest Spain. Water 2020 , 12 , 1063. [ Google Scholar ] [ CrossRef ]
- Syakur, M.A.; Khotimah, B.K.; Rochman, E.M.S.; Satoto, B.D. Integration K-Means Clustering Method and Elbow Method for Identification of The Best Customer Profile Cluster. IOP Conf. Ser. Mater. Sci. Eng. 2018 , 336 , 012017. [ Google Scholar ] [ CrossRef ]
- Kodinariya, T.M.; Makwana, P.R. Review on Determining of Cluster in K-Means Clustering. Int. J. Adv. Res. Comput. Sci. Manag. Stud. 2013 , 1 , 90–95. [ Google Scholar ]
- Ketchen, D.J.; Shook, C.L. The Application of Cluster Analysis in Strategic Management Research: An Analysis and Critique. Strateg. Manag. J. 1996 , 17 , 441–458. [ Google Scholar ] [ CrossRef ]
- Martin, J.E.; Sinclair, R.R. A Typology of the Part-Time Workforce: Differences on Job Attitudes and Turnover. J. Occup. Organ. Psychol. 2007 , 80 , 301–319. [ Google Scholar ] [ CrossRef ]
- Feldmann, M.; Emanuel, K.; Zhu, L.; Lohmann, U. Estimation of Atlantic Tropical Cyclone Rainfall Frequency in the United States. J. Appl. Meteorol. Climatol. 2019 , 58 , 1853–1866. [ Google Scholar ] [ CrossRef ]
- van Westen, C.J.; Alkema, D.; Damen, M.C.J.; Kerle, N.; Kingma, N.C. Multi-Hazard Risk Assessment Distance Education Course Guide Book ; University of Twente: Enschede, The Netherlands, 2011. [ Google Scholar ]
- van der Knijff, J.M.; Younis, J.; De Roo, A.P.J. LISFLOOD: A GIS-Based Distributed Model for River Basin Scale Water Balance and Flood Simulation LISFLOOD: A GIS-Based Distributed Model for River Basin Scale Water Balance and Flood Simulation. Int. J. Geogr. Inf. Sci. 2008 , 24 , 189–212. [ Google Scholar ] [ CrossRef ]
- Mester, B.; Willner, S.N.; Frieler, K.; Schewe, J. Evaluation of River Flood Extent Simulated with Multiple Global Hydrological Models and Climate Forcings. Environ. Res. Lett. 2021 , 16 , 094010. [ Google Scholar ] [ CrossRef ]
- Leijnse, T.; van Ormondt, M.; Nederhoff, K.; van Dongeren, A. Modeling Compound Flooding in Coastal Systems Using a Computationally Efficient Reduced-Physics Solver: Including Fluvial, Pluvial, Tidal, Wind- and Wave-Driven Processes. Coast. Eng. 2021 , 163 , 103796. [ Google Scholar ] [ CrossRef ]
- van den Bout, B.; Jetten, V.G. The Validity of Flow Approximations When Simulating Catchment-Integrated Flash Floods. J. Hydrol. 2018 , 556 , 674–688. [ Google Scholar ] [ CrossRef ]
- Delestre, O.; Cordier, S.; Darboux, F.; Du, M.; James, F.; Laguerre, C.; Lucas, C.; Planchon, O.; Cordier, S.; James, Á.F.; et al. FullSWOF: A Software for Overland Flow Simulation. In Advances in Hydroinformatics ; Springer Hydrogeology: Singapore, 2013; pp. 221–231. [ Google Scholar ]
- Umer, Y.M.; Jetten, V.G.; Ettema, J. Sensitivity of Flood Dynamics to Different Soil Information Sources in Urbanized Areas. J. Hydrol. 2019 , 577 , 123945. [ Google Scholar ] [ CrossRef ]
- Umer, Y.M.; Jetten, V.G.; Ettema, J.; Lombardo, L. Application of the WRF Model Rainfall Product for the Localized Flood Hazard Modeling in a Data-Scarce Environment. Nat. Hazards 2022 , 111 , 1813–1844. [ Google Scholar ] [ CrossRef ]
- van den Bout, B.; Jetten, V.G. Catchment-Scale Multi-Process Modeling with Local Time Stepping. Environ. Earth Sci. 2020 , 79 , 184. [ Google Scholar ] [ CrossRef ]
- Baartman, J.E.M.; Jetten, V.G.; Ritsema, C.J.; de Vente, J. Exploring Effects of Rainfall Intensity and Duration on Soil Erosion at the Catchment Scale Using OpenLISEM: Prado Catchment, SE Spain. Hydrol. Process 2012 , 26 , 1034–1049. [ Google Scholar ] [ CrossRef ]
- Starkloff, T.; Stolte, J.; Hessel, R.; Ritsema, C.; Jetten, V.G. Integrated, Spatial Distributed Modelling of Surface Runoff and Soil Erosion during Winter and Spring. Catena 2018 , 166 , 147–157. [ Google Scholar ] [ CrossRef ]
- van den Bout, B.; van Westen, C.J.; Jetten, V.G. Non-Monotonic Relationships between Return Periods of Precipitation Surface Hazard Intensity. Water 2022 , 14 , 1348. [ Google Scholar ] [ CrossRef ]
- Serere, H.N. Developing a Worst-Case Tropical Cyclone Rainfall Scenario for Flood on Dominica ; Faculty of Geo-Information Science and Earth Observation of the University of Twente: Enschede, The Netherlands, 2020. [ Google Scholar ]
- Paul-Rolle, A. Commonwealth of Dominica Disaster Risk Reduction Country Profile ; Office for Disaster Management: Roseau, Dominica, 2014. [ Google Scholar ]
- Barclay, J.; Wilkinson, E.; White, C.S.; Shelton, C.; Forster, J.; Few, R.; Lorenzoni, I.; Woolhouse, G.; Jowitt, C.; Stone, H.; et al. Historical Trajectories of Disaster Risk in Dominica. Int. J. Disaster Risk Sci. 2019 , 10 , 149–165. [ Google Scholar ] [ CrossRef ]
- Matyas, C.J. Quantifying the Shapes of U.S. Landfalling Tropical Cyclone Rain Shields. Prof. Geogr. 2007 , 59 , 158–172. [ Google Scholar ] [ CrossRef ]
- Ayala, J.J.; Matyas, C.J. Tropical Cyclone Rainfall over Puerto Rico and Its Relations to Environmental and Storm-specific Factors. Int. J. Climatol. 2016 , 36 , 2223–2237. [ Google Scholar ] [ CrossRef ]
- van Westen, C.J. National Scale Landslide Susceptibility Assessment for Dominica ; University of Twente: Enschede, The Netherlands, 2016. [ Google Scholar ]
- Rouse, W.C.; Reading, A.J.; Walsh, R.P.D. Volcanic Soil Properties in Dominica, West Indies. Eng. Geol. 1986 , 23 , 1–28. [ Google Scholar ] [ CrossRef ]
- Llasat, M. An Objective Classification of Rainfall Events on the Basis of Their Convective Features: Application to Rainfall Intensity in the Northeast of Spain. Int. J. Climatol. 2001 , 21 , 1385–1400. [ Google Scholar ] [ CrossRef ]
- Dilmi, M.D.; Mallet, C.; Barthes, L.; Chazottes, A. Data-Driven Clustering of Rain Events: Microphysics Information Derived from Macro-Scale Observations. Atmos. Meas. Tech. 2017 , 10 , 1557–1574. [ Google Scholar ] [ CrossRef ]
- Zhang, Y.; Moges, S.; Block, P. Optimal Cluster Analysis for Objective Regionalization of Seasonal Precipitation in Regions of High Spatial–Temporal Variability: Application to Western Ethiopia. J. Clim. 2016 , 29 , 3697–3717. [ Google Scholar ] [ CrossRef ]
- Chen, L.; Chen, Y.; Zhang, Y.; Xu, S. Spatial Patterns of Typhoon Rainfall and Associated Flood Characteristics over a Mountainous Watershed of a Tropical Island. J. Hydrol. 2022 , 613 , 128421. [ Google Scholar ] [ CrossRef ]
- Zhou, Y.; Matyas, C.J. Spatial Characteristics of Rain Fields Associated with Tropical Cyclones Landfalling over the Western Gulf of Mexico and Caribbean Sea. J. Appl. Meteorol. Climatol. 2018 , 57 , 1711–1727. [ Google Scholar ] [ CrossRef ]
- Zagrodnik, J.P.; Jiang, H. Investigation of PR and TMI Version 6 and Version 7 Rainfall Algorithms in Landfalling Tropical Cyclones Relative to the NEXRAD Stage-IV Multisensor Precipitation Estimate Dataset. J. Appl. Meteorol. Climatol. 2013 , 52 , 2809–2827. [ Google Scholar ] [ CrossRef ]
- Matyas, C.J. Conditions Associated with Large Rain-Field Areas for Tropical Cyclones Landfalling over Florida. Phys. Geogr. 2014 , 35 , 93–106. [ Google Scholar ] [ CrossRef ]
= 5 | ||||||||||||
RPS | T (mm) | I (mm/h) | D (h) | T (mm) | I (mm/h) | D (h) | T (mm) | I (mm/h) | D (h) | T (mm) | I (mm/h) | D (h) |
Q | 518.9 | 73.1 | 17.5 | 307.1 | 50.2 | 15.5 | 107.6 | 20.2 | 14.5 | 159.7 | 24.6 | 18.0 |
Q | 729.9 | 94.9 | 25.0 | 440.0 | 63.8 | 17.5 | 209.9 | 38.9 | 20.5 | 427.3 | 61.6 | 22.5 |
Q | 1029.1 | 112.4 | 31.5 | 587.0 | 75.8 | 23.5 | 345.3 | 55.2 | 23.5 | 834.9 | 94.5 | 25.0 |
= 4 | ||||||||||||
T (mm) | I (mm/h) | D (h) | T (mm) | I (mm/h) | D (h) | T (mm) | I (mm/h) | D (h) | ||||
Q | 727.3 | 91.4 | 25.0 | 446.2 | 65.0 | 18.0 | 230.4 | 42.2 | 18.0 | |||
= 3 | ||||||||||||
T (mm) | I (mm/h) | D (h) | T (mm) | I (mm/h) | D (h) | |||||||
Q | 676.6 | 84.9 | 24.5 | 381.3 | 59.0 | 17.5 |
= 4 | ||||||
Flood extent (km ) | Flood depth (m) | Flood volume (million m ) | Runoff ratio | Infiltration (mm) | Flood duration (h) | |
T1 | 4.80 | 4.22 | 2.22 | 0.85 | 98.13 | 23.73 |
T3 | 3.70 | 3.65 | 1.42 | 0.77 | 88.95 | 19.16 |
Diff | 1.09 | 0.57 | 0.79 | 0.08 | 9.18 | 4.57 |
T1 | 4.80 | 4.22 | 2.22 | 0.85 | 98.13 | 23.73 |
T4 | 2.47 | 1.84 | 0.84 | 0.62 | 74.08 | 29.33 |
Diff | 2.32 | 2.39 | 1.38 | 0.23 | 24.06 | −5.61 |
T3 | 3.70 | 3.65 | 1.42 | 0.77 | 88.95 | 19.16 |
T4 | 2.47 | 1.84 | 0.84 | 0.62 | 74.08 | 29.33 |
Diff | 1.23 | 1.82 | 0.59 | 0.15 | 14.87 | −10.17 |
= 3 | ||||||
Flood extent (km ) | Flood depth (m) | Flood volume (million m ) | Runoff ratio | Infiltration (mm) | Flood duration (h) | |
T1 | 4.62 | 4.18 | 2.05 | 0.84 | 97.69 | 22.76 |
T3 | 3.48 | 3.35 | 1.28 | 0.74 | 84.40 | 20.83 |
Diff | 1.14 | 0.83 | 0.77 | 0.10 | 13.29 | 1.93 |
The statements, opinions and data contained in all publications are solely those of the individual author(s) and contributor(s) and not of MDPI and/or the editor(s). MDPI and/or the editor(s) disclaim responsibility for any injury to people or property resulting from any ideas, methods, instructions or products referred to in the content. |
Share and Cite
Nabukulu, C.; Jetten, V.G.; Ettema, J.; van den Bout, B.; Haarsma, R.J. Deriving Tropical Cyclone-Associated Flood Hazard Information Using Clustered GPM-IMERG Rainfall Signatures: Case Study in Dominica. Atmosphere 2024 , 15 , 1042. https://doi.org/10.3390/atmos15091042
Nabukulu C, Jetten VG, Ettema J, van den Bout B, Haarsma RJ. Deriving Tropical Cyclone-Associated Flood Hazard Information Using Clustered GPM-IMERG Rainfall Signatures: Case Study in Dominica. Atmosphere . 2024; 15(9):1042. https://doi.org/10.3390/atmos15091042
Nabukulu, Catherine, Victor G. Jetten, Janneke Ettema, Bastian van den Bout, and Reindert J. Haarsma. 2024. "Deriving Tropical Cyclone-Associated Flood Hazard Information Using Clustered GPM-IMERG Rainfall Signatures: Case Study in Dominica" Atmosphere 15, no. 9: 1042. https://doi.org/10.3390/atmos15091042
Article Metrics
Article access statistics, further information, mdpi initiatives, follow mdpi.
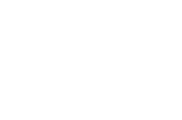
Subscribe to receive issue release notifications and newsletters from MDPI journals
- Systematic Review
- Open access
- Published: 07 September 2024
Assessing the effectiveness of greater occipital nerve block in chronic migraine: a systematic review and meta-analysis
- Muhamad Saqlain Mustafa 1 , 7 ,
- Shafin bin Amin 2 , 8 ,
- Aashish Kumar 2 , 8 ,
- Muhammad Ashir Shafique 1 , 7 ,
- Syeda Mahrukh Fatima Zaidi 3 , 9 ,
- Syed Ali Arsal 2 , 8 ,
- Burhanudin Sohail Rangwala 1 , 7 ,
- Muhammad Faheem Iqbal 3 , 9 ,
- Adarsh Raja 2 , 8 ,
- Abdul Haseeb 1 , 7 ,
- Inshal Jawed 3 , 9 ,
- Khabab Abbasher Hussien Mohamed Ahmed ORCID: orcid.org/0000-0003-4608-5321 5 ,
- Syed Muhammad Sinaan Ali 6 , 10 &
- Giustino Varrassi 4
BMC Neurology volume 24 , Article number: 330 ( 2024 ) Cite this article
Metrics details
Background & aims
Chronic migraine poses a global health burden, particularly affecting young women, and has substantial societal implications. This study aimed to assess the efficacy of Greater Occipital Nerve Block (GONB) in individuals with chronic migraine, focusing on the impact of local anesthetics compared with placebo.
A meta-analysis and systematic review were conducted following the PRISMA principles and Cochrane Collaboration methods. Eligible studies included case-control, cohort, and randomized control trials in adults with chronic migraine, adhering to the International Classification of Headache Disorders, third edition (ICHD3). Primary efficacy outcomes included headache frequency, duration, and intensity along with safety assessments.
Literature searches across multiple databases yielded eight studies for qualitative analysis, with five included in the final quantitative analysis. A remarkable reduction in headache intensity and frequency during the first and second months of treatment with GONB using local anesthetics compared to placebo has been reported. The incidence of adverse events did not differ significantly between the intervention and placebo groups.
The analysis emphasized the safety and efficacy of GONB, albeit with a cautious interpretation due to the limited number of studies and relatively small sample size. This study advocates for further research exploring various drugs, frequencies, and treatment plans to enhance the robustness and applicability of GONB for chronic migraine management.
Peer Review reports
Introduction
Among headache disorders, migraine is particularly ranked second worldwide in terms of disability and is the leading cause of disability among young women, according to the Global Burden of Disease 2019 data [ 1 ]. Recent findings indicate that the global prevalence of migraine is approximately 15%, which translates to 4.9% of all ill health measured in years lived with disability (YLDs) [ 2 ]. Women are more likely to experience migraine than men, particularly those aged 15–49 years [ 3 ]. Migraine has a substantial societal and financial impact owing to both direct and indirect costs resulting from decreased productivity and missed work [ 4 ].
Migraine is a complex neurovascular disorder that affects sensory processing and is characterized by a range of symptoms, with headache being the most common symptom [ 5 ]. Chronic migraine (CM) is defined as the frequent occurrence of headache episodes, with at least 15 or more episodes (which, on at least 8 days/month, have the features of migraine headache) occurring per month for more than three months [ 6 ]. Several medications are available for the preventive treatment of migraine, including anticonvulsants, antidepressants, beta-blockers, calcium channel blockers, botulinum toxin A, and more recently, drugs that block the calcitonin gene-related peptide (CGRP) pathway (i.e., monoclonal antibodies and antagonists) [ 7 ]. Despite the potential of anti-CGRP monoclonal antibodies (mAbs) in managing chronic migraine, a remarkable proportion of patients do not respond to this treatment [ 8 ]. Approximately 25% of patients are unresponsive to anti-CGRP monoclonal antibodies [ 9 ].
An important component of the brainstem, the Trigeminocervical Complex (TCC) acts as a central processing unit for pain and sensory data from the head and neck. This is the point of convergence of the upper cervical spinal nerves and the trigeminal nerve, which supplies feeling to the face, head, and some regions of the neck [ 10 , 11 ].
One of the TCC’s primary functions is the confluence of the occipital and trigeminal nerves there. The trigeminal nerve transmits sensory data from the face, scalp, and meninges through its three main branches (ophthalmic, maxillary, and mandibular). In the meanwhile, feelings from the back of the head are transmitted by the occipital nerves, which originate from the upper cervical spinal roots [ 10 , 11 ]. Wide-ranging integration of sensory inputs from the head and neck is made possible by the network formed when these neurons converge at the TCC. The brainstem area known as the trigeminocervical complex is crucial to migraine pain processing since it is responsible for processing pain signals originating from the head and neck. [ 10 , 11 ]. The face, head, and neck region’s sensory data—especially pain—are integrated by the TCC. Because of this integration, the TCC is an important piece of the migraine jigsaw when it comes to interpreting the location and degree of pain. The trigeminal, occipital, and TCC nerves are intricately intertwined with one another. A series of neurological events are set off during a migraine episode, beginning with the stimulation of the trigeminal nerve. This activation increases pain signals by causing the production of inflammatory chemicals around the TCC and blood arteries in the brain [ 10 , 11 ]. Accompanying this, the occipital nerves may also be affected, particularly if the headache radiates to the rear of the head. Because of its connection, the TCC is further stimulated by pain signals from the occipital area, worsening the migraine sensation (it produces a feedback loop) [ 10 , 11 ].
The main sensory nerve that serves the occipital region is the Greater Occipital Nerve (GON), which predominantly originates from the C2 dorsal root. The GON block is used in acute and preventive headache treatments as it targets the anatomical and functional connections between the trigeminal and cervical fibers within the trigemino-cervical complex (TCC). The rationale for using GON blocks is based on the integration of sensory neurons from C2 in the upper cervical spinal cord with neurons in the trigeminal nucleus caudalis (TNC). However, the precise mechanisms by which GON blocks may affect the TCC and potentially reduce its activity are still being researched [ 12 ]. However, there is currently no standard protocol for GONB. Local anesthetics function by preventing the activation of voltage-gated sodium channels, which reduce the transmission of sensory signals originating from areas innervated by the greater occipital nerve, such as the medial region of the posterior scalp [ 13 , 14 ], thereby preventing the activation of convergent neurons in the trigeminal-cervical complex. Combination therapy with corticosteroids may reduce inflammation, thereby attenuating pain, however, this role of corticosteroids also seems to be under debate.
The current management of chronic migraines is inadequate, as it lacks clear guidelines despite the various treatment options available. The evidence supporting the efficacy of GONB in preventing chronic migraines is limited and not recent [ 15 , 16 , 17 ]. However, the emergence of new clinical trials offers a promising opportunity for this study to provide valuable insights to healthcare providers. This study aims to fill the knowledge gaps by conducting a comprehensive systematic review and meta-analysis, providing healthcare professionals with a more complete understanding of the collective results of this approach for the treatment of chronic migraines.
A meta-analysis and a comprehensive systematic review were conducted to assess the efficacy of GONB in patients with CM, adhering to Preferred Reporting Items for Systematic Reviews and Meta-Analyses (PRISMA) guidelines [ 18 ]. The PICO framework, a cornerstone of evidence-based medicine, organizes clinical questions and study designs into Population, Intervention, Comparison, and Outcome. In our research on chronic migraine treatment, we examine the efficacy of greater occipital nerve block (Intervention) with local anesthetics alone versus a placebo (Comparison) among adults with chronic migraine (Population), focusing on changes in migraine intensity measured by VAS, frequency, and adverse effects (Outcome).
Eligibility criteria
Inclusion criteria for studies considered in this meta-analysis encompassed randomized controlled trials (RCTs) evaluating the efficacy of greater occipital nerve block (GONB) with local anesthetics alone compared to a placebo in adult individuals diagnosed with chronic migraine. Studies were required to report outcomes including changes in migraine intensity measured by Visual Analog Scale (VAS), frequency of migraine episodes, and documentation of adverse effects. Exclusion criteria comprised studies that incorporated corticosteroids in conjunction with local anesthetics for GONB, non-randomized or non-controlled trials, studies with insufficient data for outcome assessment, and those involving populations other than adults with chronic migraine.
The primary efficacy endpoints were the change in headache intensity as measured by any scale, the frequency of headache (days per month) in the intervention group compared to the placebo group at a specific point in time, and the intensity of headache in the intervention group compared to the placebo group. To assess safety, the analysis focused on the number of participants who experienced at least one adverse event (AE) and the total number of participants who experienced AEs.
Literature search and study selection
A systematic search of PubMed, Medline, Scopus, Embase, Cochrane, Web of Science, and PsycINFO was performed as of June 2023 by two authors AR and AH. All languages and publication dates were considered and the search strategy involved both free and restricted terms pertaining to migraine and GONB, using key word ‘Chronic migraine’ or Migraine’ or ‘Greater Occipital Nerve Block’. Duplicates were eliminated and the titles and abstracts of the remaining articles were assessed to identify relevant studies. Subsequently, a full-text assessment was performed by two independent investigators (AK and BSR) and any discrepancies were resolved by a third investigator (MSM). The PRISMA flowchart (Fig. 1 ) illustrates the selection process.
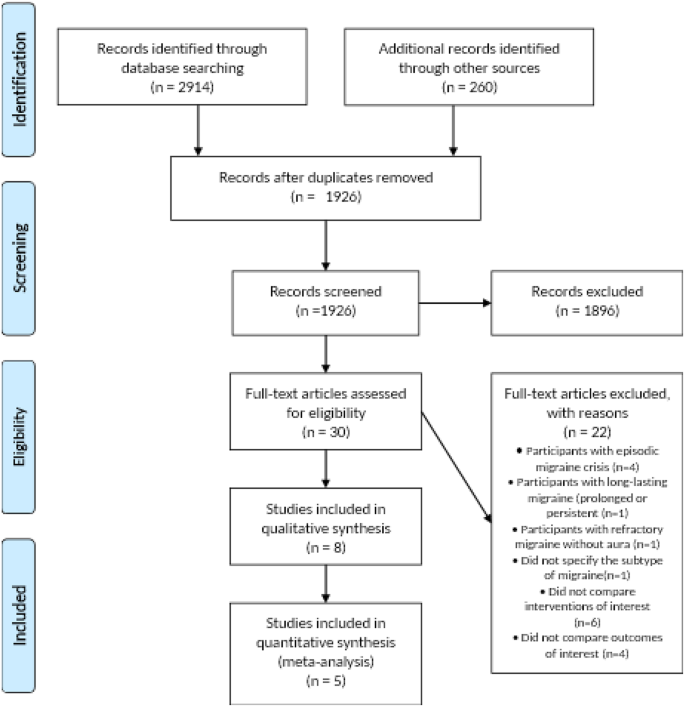
Prisma flow chart
Data extraction
We utilized a standard Microsoft Excel 2021 spreadsheet to gather data from each study included in a predetermined format. Two unbiased investigators (MAS and SMFZ) collected the following information from each study: author, year of publication, population, intervention and comparison drugs, techniques, primary and secondary outcomes, funding and potential conflicts of interest. If a disagreement arose, a third investigator made the final decision (GV).
Statistical analysis
Statistical analysis was conducted using Review Manager 5.3.22 and Comprehensive Meta-analysis. In order to account for anticipated between-study heterogeneity, we employed random-effects models in our meta-analysis of continuous outcomes. We reported the effect sizes as weighted mean differences (MD) with 95% confidence intervals (CI) for trials with similar results. The I 2 statistics were used to assess the statistical heterogeneity of the pooled estimates. While recognizing that statistical heterogeneity may not be significant when I 2 is < 40%, we performed this test. Regrettably, due to the limited number of included papers, we were unable to carry out a subgroup analysis or funnel plot assessment of publication bias.
Studies selection
The initial literature search yielded 3174 studies. After a detailed review of the selected studies and removal of duplicate entries, 1964 articles remained. These articles were then evaluated based on their titles and abstracts to determine whether they met the inclusion criteria for our study and those that did not were excluded. A comprehensive screening of the full text was performed in the remaining 30 studies. Studies which did not meet the inclusion criteria were excluded. The final quantitative analysis included five studies and 3 studies were included in the qualitative assessment as these studies used other drugs like corticosteroids thus with different interventions. A visual representation of the PRISMA flowchart effectively illustrated the study selection process (Fig. 1 ).
Quality assessment
In assessing the quality of RCTs, we extensively utilized the Cochrane Risk of Bias tool which categorizes studies into three risk levels: high, uncertain, and low, across seven specific domains encompassing aspects of selection, comparability, and outcome. Following rigorous evaluation, all studies included in our analysis were consistently classified as having low risk across these domains. A detailed presentation of the Risk of Bias assessment is shown in Fig. 2 .
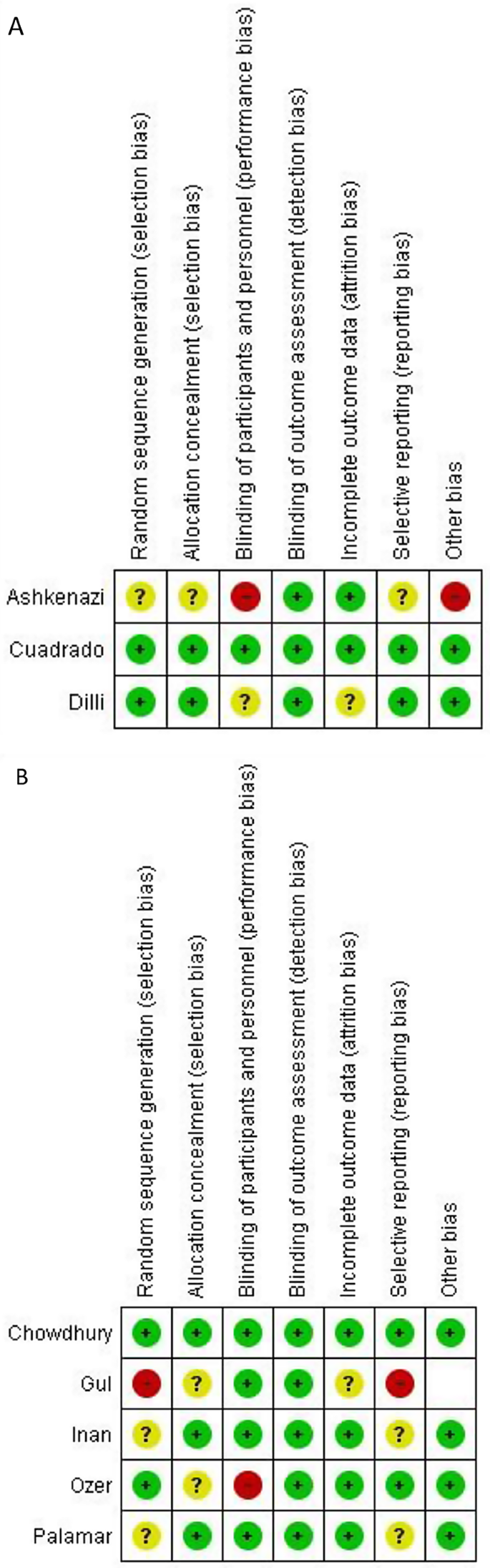
Risk of bias Assessment ( A ) Qualitative ( B ) Quantitative
Study and patient characteristics
All the included studies assessed outcomes in patients aged 18–75 years. The intervention group in three studies [ 19 , 20 , 21 ] used bupivacaine 0.5% 1.5 ml with or without 1 ml of saline (0.9%); one study [ 22 ] used lidocaine 2% 1 ml with 1 ml of saline solution (0.9%); and lastly, one study [ 23 ] used lidocaine 2% 2 ml as the interventional group. In the control groups, a saline solution of 0.9% (1.5, 2, or 2.5 ml) was used as a placebo. A total of 268 patients were included in all studies, ranging in age from 18 to 75 years. The studies differed in their follow-up procedures. Two studies were followed up at 4 weeks, one study was followed up for up to 2 months, and two studies were checked every month for up to 3 months A summary of patients’ baseline characteristics is provided in Table 1 .
Effect of GONB on headache intensity
In the initial month following GONB treatment, the meta-analysis of three studies showed a significant reduction in headache intensity as measured by the Visual Analog Scale (VAS). The standardized mean difference (SMD) was − 0.653, with a 95% confidence interval (CI) of -0.996 to -0.311 and a p-value of 0.0001. This indicates that the local anesthetic group experienced a greater reduction in headache intensity compared to the placebo group. Importantly, the I² value of 0% suggests that there was no observed heterogeneity among the studies, indicating consistent results across the studies analyzed. (Fig. 3 )

Forest plot illustrating the effect of GONB on headache intensity, evaluated using VAS within the initial month
In the second month, an analysis of five studies continued to show a significant reduction in headache intensity with an SMD of -0.628 (95% CI -1.148 to -0.107; p = 0.018). However, the I² value increased to 74%, indicating substantial heterogeneity among the studies. This heterogeneity was primarily due to one study (Inan et al.), which had an outlier SMD of 0.136. (Fig. 4 ) A leave-one-out analysis was conducted to address this issue and is shown in Fig. 5 .

Forest plot illustrating the impact of GONB on headache intensity, evaluated using VAS during the second month
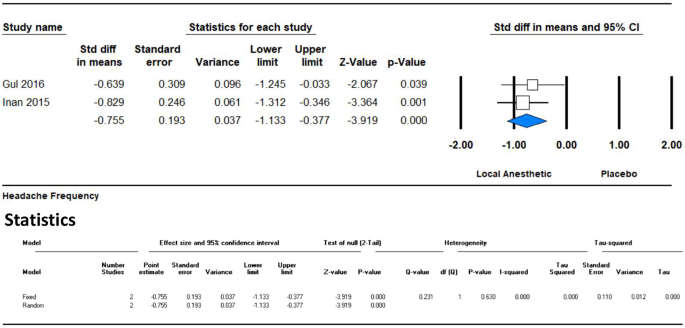
Forest plot illustrating the effect of GONB on headache frequency within the initial month
Headache frequency
Within the initial month, the analysis of two studies showed a significant reduction in headache frequency, with an SMD of -0.755 (95% CI -1.133 to -0.377; p = 0.0001). The results indicate a notable decrease in headache frequency in the local anesthetic group compared to the placebo group. The I² value of 0% indicates no heterogeneity between the studies, suggesting that the results were consistent. (Fig. 6 )

Forest plot illustrating the impact of GONB on headache frequency during the second month
At the two-month mark, the analysis of four studies also showed a significant reduction in headache frequency with an SMD of -0.577 (95% CI -0.887 to -0.266; p = 0.0001). The low I² value of 8.9% indicates minimal heterogeneity among the studies, reinforcing the consistency of the observed effect (Fig. 7 ).
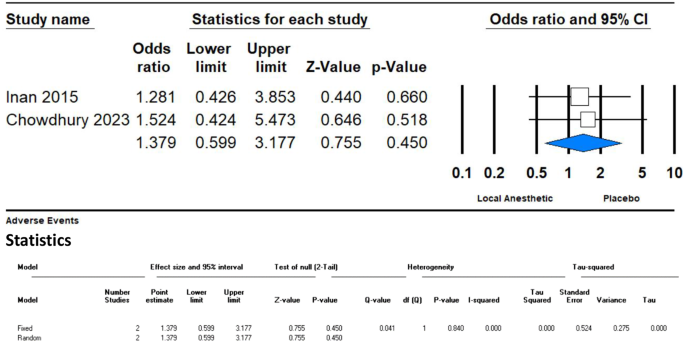
Forest plot displaying adverse events associated with the use of GONB
Adverse events
The meta-analysis of two studies on adverse events revealed no significant difference between the GONB treatment and placebo groups. The odds ratio (OR) was 1.379 with a 95% CI of 0.599 to 3.177 and a p-value of 0.450. The confidence interval crosses one, indicating that there is no clear increased risk of adverse events associated with GONB treatment. Additionally, the I² value of 0% suggests no heterogeneity between the studies, indicating consistent findings regarding the safety profile of GONB (Fig. 7 ).
We conducted an updated meta-analysis of GONB in patients with CM, incorporating findings from five RCTs. All RCTs used local anesthetics for GONB, while 0.9% saline served as the placebo. Our study focused on evaluating the impact of GONB on headache frequency, intensity, and associated adverse effects. The results demonstrated the beneficial effects of local anesthetics in reducing both the frequency and intensity of headaches during the first and second months of treatment. However, the outcomes related to adverse effects did not reach statistical significance. This meta-analysis included studies employing two distinct local anesthetics: 0.5% bupivacaine and 2% lidocaine. This suggests that the use of any local anesthetic could yield positive outcomes when compared with the effects of a placebo. Despite the positive results observed, we approached the evidence with caution because of the assessment of low certainty. Therefore, additional studies are warranted to further substantiate our findings and to enhance the reliability of the conclusions drawn from our meta-analysis.
Our meta-analysis demonstrated that GONB treatment significantly reduces both headache intensity and frequency in the initial and subsequent months post-treatment compared to placebo. During the first month, the studies consistently showed a marked reduction in headache intensity with no observed heterogeneity, indicating uniform results across the studies analyzed. In the second month, while the reduction in headache intensity remained remarkable, some heterogeneity was noted due to an outlier study. Similarly, the analysis revealed a notable decrease in headache frequency within the first month, again with consistent findings and no heterogeneity between the studies. By the second month, the reduction in headache frequency continued to be noteworthy, with minimal heterogeneity observed, reinforcing the consistency of the treatment effect. Furthermore, the analysis of adverse events indicated no significant difference between the GONB treatment and placebo groups, suggesting that GONB does not increase the risk of adverse events. The studies consistently supported the safety profile of GONB, with no observed heterogeneity. In terms of both safety and efficacy, our findings suggest that the use of local anesthetics in GONB is generally safe, as we did not identify any notable adverse effects in our intervention group. However, the certainty of our evidence is moderate, primarily because our results did not reach statistical significance, potentially influenced by the limited number of studies and relatively short follow-up phase. In our updated meta-analysis, building upon the original study by Velezquez et al. [ 24 ], we included an additional randomized RCT, contributing to a more comprehensive quantitative analysis. Although most of our study findings align with Velezquez’s findings [ 24 ], demonstrating the safety and effectiveness of GONB in treating chronic migraine, it is important to acknowledge some variations. Velezquez highlighted occasional negative effects associated with local anesthetics but found no remarkable side effects. In contrast, our study did not yield statistically significant outcomes in defining these results. A noteworthy distinction lies in the consideration of adjuvants: while our study did not account for steroids or other adjuvants, Velezquez considered steroids for every study outcome. This discrepancy underscores the need for further exploration and standardization of variables in future research to establish a more definitive understanding of the safety and efficacy of GONB in the management of chronic migraine.
Our findings strongly suggest that GONB is a safe and effective method for treating migraine. This assertion is consistent with existing research that characterizes GONB as a highly effective and safe therapy with minimal adverse effects, recommending its consideration when alternative treatments are unsuccessful [ 21 ]. This viewpoint is further supported by another study that affirms our findings, emphasizing a preference for GONB in cases of resistant migraine [ 22 ]. Moreover, evidence suggests the potential applicability of GONB in the treatment of various types of headaches [ 23 , 25 ]. A retrospective cohort study also indicated that GONB may be beneficial in addressing acute migraine episodes, albeit with a cautionary note regarding the potential negative effects occurring during the procedure rather than during the follow-up period [ 26 ]. Additional observational studies [ 25 , 27 ] reinforce our findings. However, a study comparing the effectiveness of GONB with placebo in preventing migraine revealed that while there was no marked change in headache frequency, GONB still played a remarkable role in lowering intensity [ 28 ]. Notably, these studies underscored the benefits of GONB, often involving the adjunct use of steroids. In a randomized controlled trial that focused on patients treated with bilateral GONB, the results indicated that the administration of a local anesthetic was associated with lower frequency, reduced intensity, and increased pressure thresholds. However, it is important to note that this study predominantly involved female participants [ 29 ]. However, it is essential to acknowledge that trials exclusively assessing the independent use of local anesthetics in GONB are currently lacking, as steroids are commonly employed as adjuvants in the majority of studies. This finding suggests the need for further investigation to delineate the unique contributions of local anesthetics to GONB outcomes.
Prior research has emphasized the necessity of comparing various treatment plans for GONB, incorporating diverse anesthetics and adjuncts to comprehensively evaluate its effectiveness, the need for additional intervention, and safety considerations, it is crucial to note that we did not incorporate any adjuncts, preventing us from commenting on their potential impact on the treatment outcomes. The absence of adjunct utilization in our study underscores the need for further exploration of how these additions may influence the overall efficacy and safety of GONB. Most trials in our analysis used weekly injections, resulting in a lack of comprehensive data for comparing various frequencies. Nevertheless, some studies have suggested the potential advantages associated with monthly use [ 26 ]. The American Headache Society also suggests and has shown interest in the efficacy of nerve blocks for headache treatment. Their endorsement highlights the growing recognition of nerve blocks as a valuable therapeutic option for managing headaches [ 30 , 31 ].
Included studies present diverse methodologies in terms of dosage, injection sites, duration and timing of the intervention, and primary endpoints for the evaluation of GONB efficacy in migraine treatment. The administration and makeup of the GONB differed substantially across the studies. For example, Gul et al. [ 20 ] used 0.5% bupivacaine diluted in 1 ml, while Inan et al. [ 19 ] used a slightly larger volume of the same concentration. Ozer et al. [ 22 ] combined 2% lidocaine with saline, and Ashkenazi et al. [ 32 ] mixed lidocaine and bupivacaine. These variations could lead to differences in efficacy and side effects. The addition of corticosteroids, as observed in Dilli et al. [ 33 ], introduces another variable that may enhance the anti-inflammatory effects but could also influence the outcome independently of the nerve block’s anesthetic action. Although the studies targeted the GON, the exact injection sites varied slightly. Most studies, such as those by Gul et al. [ 20 ], Inan et al. [ 19 ], and Cuadrado et al. [ 34 ], selected a site approximately 2 cm lateral and 2 cm inferior to the external occipital protuberance. Palamar et al. [ 21 ] used ultrasound guidance, which might improve accuracy and potential efficacy. Ashkenazi et al. [ 32 ] included additional trigger point injections (TPIs), which could complicate the specific effects of the GONB.
The administration of GONB varied in frequency and duration among different studies. While some research, such as that conducted by Gul et al. [ 20 ] and Inan et al. [ 19 ], administered the blocks weekly for four weeks, others like Chowdury et al. [ 23 ] extended the injections over a period of 12 weeks. On the other hand, Cuadrado et al. [ 34 ] and Dilli et al. [ 33 ] examined single-time administrations. These discrepancies in timing may affect both short-term and long-term outcomes, with more frequent administrations potentially leading to more sustained relief, but also increasing the risks of cumulative side effects. The primary endpoints of the studies varied but generally included measures of headache frequency and intensity. For instance, Gul et al. [ 20 ] and Palamar et al. [ 21 ] focused on the number of headache days per month, while Inan et al. assessed both frequency and intensity. Ozer et al. [ 22 ] and Cuadrado et al. [ 34 ] emphasized the reduction in headache frequency, while Dilli et al. [ 33 ] sought a 50% reduction in migraine frequency as a measure of success. The variation in endpoints underscores the multifaceted nature of migraine impact and the significance of selecting appropriate, consistent measures for evaluating the efficacy of treatments.
Despite the differences in methodology, the studies collectively indicate that GONB can effectively decrease the frequency and severity of migraines. The consistent reporting of substantial improvements across a range of dosages, injection techniques, and primary outcomes reinforces the potential usefulness of GONB in clinical practice. However, the variation in methodologies highlights the need for standardized protocols to improve the comparability and generalizability of the findings. While the reviewed studies indicate promising outcomes for GONB in migraine treatment, the variability in dosage, injection sites, administration timing, and primary endpoints necessitates caution.
Examining these frequencies is particularly vital because of the invasive nature of the procedure, which offers valuable insights into its safety profile. An essential aspect of chronic migraine management is patient adherence, which markedly contributes to treatment success. It is imperative to assess the level of adherence to GONB. Unfortunately, we could not find relevant research on participants discontinuing their medication owing to side effects, hindering our ability to determine the tolerability of the treatment. Another unresolved concern revolves around the choice between unilateral and bilateral GONB and their relative efficacy. A retrospective cohort study comparing patients who underwent bilateral versus unilateral GONB demonstrated equal effectiveness [ 35 ]. However, a definitive conclusion remains elusive as additional evidence from diverse studies is lacking. Addressing these gaps in research would contribute substantially to refining our understanding of GONB’s optimal parameters for improved outcomes in chronic migraine management. Longitudinal studies and studies on the frequency of nerve block use are needed to assess long-term efficacy.
Limitations
Although this meta-analysis offers valuable insights, it is crucial to acknowledge its limitations. First, the small sample size resulting from the limited availability of new studies may compromise the reliability and accuracy of our findings. Although incorporating more studies could alleviate this concern, the scarcity of available data remains an issue. Second, the absence of sufficient data from recent trials prevented consideration of baseline characteristics, hindering our ability to perform meta-regression. This limitation underscores the importance of comprehensive data collection in future studies to increase the depth of our analyses. Third, oversight of not accounting for pretreatment medications taken by patients during the procedure might introduce a confounding factor. Although the existing data may be insufficient to draw definitive conclusions, recognizing and addressing this aspect in future research is essential for a more nuanced understanding. Moreover, this meta-analysis did not explicitly address patient comorbidities. These factors could potentially influence the safety of the procedure in patients with various comorbidities. Future studies should delve into these aspects to provide a more comprehensive assessment of the safety profile of the procedure in diverse patient populations. In conclusion, although this meta-analysis provides valuable insights, researchers must remain cognizant of these limitations. Addressing these concerns in future studies will enhance the robustness and applicability of these findings in clinical settings.
Based on our investigation, we ascertained that the administration of Greater Occipital Nerve Blocks (GONB) with local anesthetic leads to a notable reduction in both the intensity and frequency of headaches when compared to placebo. Additionally, our research underscores the effectiveness of GONBs and affirms their satisfactory safety profile. However, it is important to acknowledge that our confidence in these findings is somewhat tempered by the limited number of studies and relatively modest sample size that underpins our conclusions. Therefore, we advocate that future studies should broaden their scope by incorporating larger and more diverse sample sizes. These studies should also explore a range of drugs, frequencies, and treatment plans to augment the robustness and applicability of the results, thereby providing a more comprehensive understanding of the potential benefits of GONBs for headache management.
Data availability
The data are available within the article and supplementary files. The authors confirm that data supporting the findings of this study are available in the article and supplementary files.
Steiner TJ, Stovner LJ, Jensen R, Uluduz D, Katsarava Z. Migraine remains second among the world’s causes of disability, and first among young women: findings from GBD2019. J Headache Pain. 2020;21(1):137.
Article PubMed PubMed Central Google Scholar
Steiner TJ, Stovner LJ. Global epidemiology of migraine and its implications for public health and health policy. Nat Rev Neurol. 2023;19(2):109–17.
Article PubMed Google Scholar
Stovner LJ, Nichols E, Steiner TJ, Abd-Allah F, Abdelalim A, Al-Raddadi RM, et al. Global, regional, and national burden of migraine and tension-type headache, 1990–2016: a systematic analysis for the global burden of Disease Study 2016. Lancet Neurol. 2018;17(11):954–76.
Article Google Scholar
Ferrari MD. The economic burden of migraine to society. PharmacoEconomics. 1998;13(6):667–76.
Goadsby PJ, Holland PR. An update: pathophysiology of Migraine. Neurol Clin. 2019;37(4):651–71.
The International Classification of Headache Disorders, 3rd edition (beta version). Cephalalgia. 2013;33(9):629–808.
Urits I, Yilmaz M, Bahrun E, Merley C, Scoon L, Lassiter G, et al. Utilization of B12 for the treatment of chronic migraine. Best Pract Res Clin Anaesthesiol. 2020;34(3):479–91.
Hong JB, Lange KS, Overeem LH, Triller P, Raffaelli B, Reuter U. A scoping review and Meta-analysis of Anti-CGRP monoclonal antibodies: Predicting Response. Pharmaceuticals (Basel). 2023;16(7).
Han L, Liu Y, Xiong H, Hong P. CGRP monoclonal antibody for preventive treatment of chronic migraine: an update of meta-analysis. Brain Behav. 2019;9(2):e01215.
Al-Khazali HM, Krøll LS, Ashina H, Melo-Carrillo A, Burstein R, Amin FM et al. Neck pain and headache: Pathophysiology, treatments and future directions. Musculoskeletal Science and Practice [Internet]. 2023;66:102804. https://doi.org/10.1016/j.msksp.2023.102804
Bartsch T, Goadsby PJ. The trigeminocervical complex and migraine: current concepts and synthesis. Curr Sci Inc. 2003;7:371–6. https://doi.org/10.1007/s11916-003-0036-y .
Chowdhury D, Datta D, Mundra A. Role of Greater Occipital nerve Block in Headache disorders: a narrative review. Neurol India. 2021;69(Supplement):S228–56.
Anthony M. Headache and the greater occipital nerve. Clin Neurol Neurosurg. 1992;94(4):297–301.
Selekler MH. [Greater occipital nerve blockade: trigeminicervical system and clinical applications in primary headaches]. Agri. 2008;20(3):6–13.
PubMed Google Scholar
Shauly O, Gould DJ, Sahai-Srivastava S, Patel KM. Greater Occipital nerve block for the treatment of chronic migraine headaches: a systematic review and Meta-analysis. Plast Reconstr Surg. 2019;144(4):943–52.
Tang Y, Kang J, Zhang Y, Zhang X. Influence of greater occipital nerve block on pain severity in migraine patients: a systematic review and meta-analysis. Am J Emerg Med. 2017;35(11):1750–4.
Zhang H, Yang X, Lin Y, Chen L, Ye H. The efficacy of greater occipital nerve block for the treatment of migraine: a systematic review and meta-analysis. Clin Neurol Neurosurg. 2018;165:129–33.
Page MJ, McKenzie JE, Bossuyt PM, Boutron I, Hoffmann TC, Mulrow CD, et al. The PRISMA 2020 statement: an updated guideline for reporting systematic reviews. BMJ. 2021;372:n71.
Inan LE, Inan N, Karadaş Ö, Gül HL, Erdemoğlu AK, Türkel Y, et al. Greater occipital nerve blockade for the treatment of chronic migraine: a randomized, multicenter, double-blind, and placebo-controlled study. Acta Neurol Scand. 2015;132(4):270–7.
Gul HL, Ozon AO, Karadas O, Koc G, Inan LE. The efficacy of greater occipital nerve blockade in chronic migraine: a placebo-controlled study. Acta Neurol Scand. 2017;136(2):138–44.
Palamar D, Uluduz D, Saip S, Erden G, Unalan H, Akarirmak U. Ultrasound-guided greater occipital nerve block: an efficient technique in chronic refractory migraine without aura? Pain Physician. 2015;18(2):153–62.
Özer D, Bölük C, Türk Börü Ü, Altun D, Taşdemir M, Köseoğlu Toksoy C. Greater occipital and supraorbital nerve blockade for the preventive treatment of migraine: a single-blind, randomized, placebo-controlled study. Curr Med Res Opin. 2019;35(5):909–15.
Chowdhury D, Tomar A, Deorari V, Duggal A, Krishnan A, Koul A. Greater occipital nerve blockade for the preventive treatment of chronic migraine: a randomized double-blind placebo-controlled study. Cephalalgia. 2023;43(2):3331024221143541.
Velásquez-Rimachi V, Chachaima-Mar J, Cárdenas-Baltazar EC, Loayza-Vidalon A, Morán-Mariños C, Pacheco-Barrios K, et al. Greater occipital nerve block for chronic migraine patients: a meta-analysis. Acta Neurol Scand. 2022;146(2):101–14.
Bovim G, Sand T. Cervicogenic headache, migraine without aura and tension-type headache. Diagnostic blockade of greater occipital and supra-orbital nerves. Pain. 1992;51(1):43–8.
Allen SM, Mookadam F, Cha SS, Freeman JA, Starling AJ, Mookadam M. Greater Occipital nerve block for Acute Treatment of Migraine Headache: a large Retrospective Cohort Study. J Am Board Fam Med. 2018;31(2):211–8.
Austin M, Hinson MR. Occipital nerve Block. StatPearls. Treasure Island (FL) ineligible companies. Disclosure: Melissa Hinson declares no relevant financial relationships with ineligible companies.: StatPearls Publishing Copyright © 2024. StatPearls Publishing LLC.; 2024.
Afridi SK, Shields KG, Bhola R, Goadsby PJ. Greater occipital nerve injection in primary headache syndromes–prolonged effects from a single injection. Pain. 2006;122(1–2):126–9.
Santos Lasaosa S, Cuadrado Pérez ML, Guerrero Peral AL, Huerta Villanueva M, Porta-Etessam J, Pozo-Rosich P, et al. Consensus recommendations for anaesthetic peripheral nerve block. Neurologia. 2017;32(5):316–30.
Rothrock JF. Occfipital nerve blocks. Headache the Journal of Head and Face Pain [Internet]. 2010;50(5):917–8. https://doi.org/10.1111/j.1526-4610.2010.01668.x
Blumenfeld A, Ashkenazi A, Napchan U, Bender SD, Klein BC, Berliner R et al. Expert Consensus Recommendations for the performance of Peripheral nerve blocks for headaches – A Narrative review. Headache the Journal of Head and Face Pain [Internet]. 2013;53(3):437–46. https://doi.org/10.1111/head.12053
Ashkenazi A, Matro R, Shaw JW, Abbas MA, Silberstein SD. Greater occipital nerve block using local anaesthetics alone or with triamcinolone for transformed migraine: a randomised comparative study. J Neurol Neurosurg Psychiatry. 2008;79(4):415–7.
Dilli E, Halker R, Vargas B, Hentz J, Radam T, Rogers R, et al. Occipital nerve block for the short-term preventive treatment of migraine: a randomized, double-blinded, placebo-controlled study. Cephalalgia. 2015;35(11):959–68.
Cuadrado ML, Aledo-Serrano Á, Navarro P, López-Ruiz P, Fernández-de-Las-Peñas C, González-Suárez I, et al. Short-term effects of greater occipital nerve blocks in chronic migraine: a double-blind, randomised, placebo-controlled clinical trial. Cephalalgia. 2017;37(9):864–72.
Karaoğlan M, Durmuş İE, Küçükçay B, Takmaz SA, İnan LE. Comparison of the clinical efficacy of bilateral and unilateral GON blockade at the C2 level in chronic migraine. Neurol Sci. 2022;43(5):3297–303.
Download references
Acknowledgements
We would like to thank the Paolo Procacci Foundation for their support.
The study was funded by the Paolo Procacci Foundation.
Author information
Authors and affiliations.
Department of Medicine, Jinnah Sindh Medical University, Karachi, 75510, Pakistan
Muhamad Saqlain Mustafa, Muhammad Ashir Shafique, Burhanudin Sohail Rangwala & Abdul Haseeb
Department of Medicine, Shaheed Mohtarma Benazir Bhutto Medical College, Karachi, 75400, Pakistan
Shafin bin Amin, Aashish Kumar, Syed Ali Arsal & Adarsh Raja
Department of Medicine, Dow University of Health Science, Karachi, 74200, Pakistan
Syeda Mahrukh Fatima Zaidi, Muhammad Faheem Iqbal & Inshal Jawed
Fondazione Paolo Procacci, Roma, 00193, Italy
Giustino Varrassi
Faculty of Medicine, University of Khartoum, Khartoum, 11111, Sudan
Khabab Abbasher Hussien Mohamed Ahmed
Department of Medicine, Liaquat National Hospital and Medical College, Karachi, Pakistan
Syed Muhammad Sinaan Ali
Jinnah Sindh Medical University, Rafiqi H J Shaheed Road, Karachi, 75510, Pakistan
Shaheed Mohtarma Benazir Bhutto Medical College, Lyari Hospital Rd, Rangiwara Karachi, Karachi, 75400, Pakistan
Dow University of Health Sciences, Mission Rd, New Labour Colony Nanakwara, Karachi, 74200, Pakistan
Liaquat National Hospital & Medical College, Stadium Road, Karachi, 74800, Pakistan
You can also search for this author in PubMed Google Scholar
Contributions
M.S.M., S.B.A., A.K., M.A.S., S.M.F.Z., S.A.A. and B.S.R. wrote the main manuscript, visualized, validated and analyzed data. M.F.I., A.R., A.H., I.J., K.A.H.M.A., S.M.S.A. and G.V. wrote the main manuscript, conceived, visualized, validated, reviewed and edited.All authors made a significant contribution to the work reported, whether that is in the conception, study design, execution, acquisition of data, analysis and interpretation, or in all these areas; took part in drafting, revising or critically reviewing the article; gave final approval of the version to be published; have agreed on the journal to which the article has been submitted; and agree to be accountable for all aspects of the work.
Corresponding author
Correspondence to Khabab Abbasher Hussien Mohamed Ahmed .
Ethics declarations
Ethics approval and consent to participate.
Not applicable.
Consent for publication
Competing interests.
The authors declare no competing interests.
Additional information
Publisher’s note.
Springer Nature remains neutral with regard to jurisdictional claims in published maps and institutional affiliations.
Rights and permissions
Open Access This article is licensed under a Creative Commons Attribution 4.0 International License, which permits use, sharing, adaptation, distribution and reproduction in any medium or format, as long as you give appropriate credit to the original author(s) and the source, provide a link to the Creative Commons licence, and indicate if changes were made. The images or other third party material in this article are included in the article’s Creative Commons licence, unless indicated otherwise in a credit line to the material. If material is not included in the article’s Creative Commons licence and your intended use is not permitted by statutory regulation or exceeds the permitted use, you will need to obtain permission directly from the copyright holder. To view a copy of this licence, visit http://creativecommons.org/licenses/by/4.0/ .
Reprints and permissions
About this article
Cite this article.
Mustafa, M.S., bin Amin, S., Kumar, A. et al. Assessing the effectiveness of greater occipital nerve block in chronic migraine: a systematic review and meta-analysis. BMC Neurol 24 , 330 (2024). https://doi.org/10.1186/s12883-024-03834-6
Download citation
Received : 19 April 2024
Accepted : 28 August 2024
Published : 07 September 2024
DOI : https://doi.org/10.1186/s12883-024-03834-6
Share this article
Anyone you share the following link with will be able to read this content:
Sorry, a shareable link is not currently available for this article.
Provided by the Springer Nature SharedIt content-sharing initiative
- Chronic migraine
- Greater occipital nerve block (GONB)
- Local anesthetics
BMC Neurology
ISSN: 1471-2377
- General enquiries: [email protected]

COMMENTS
Design flood estimation is an essential step in many water engineering design tasks such as the planning and design of infrastructure to reduce flood damage. Flood frequency analysis (FFA) is widely used in estimating design floods when the at-site flood data length is adequate. One of the problems in FFA with an annual maxima (AM) modeling approach is deciding how to handle smaller discharge ...
A case study is performed for 10 alpine catchments in Tyrol, Austria, where the 100-year floods are estimated by (a) flood frequency statistics and (b) an event based runoff model.
Flood Frequency Analysis (FFA) is a systematic tool used for an efficient hydraulic structures design, operation and maintenance. An effort is made to study and compare the Linear Log Regression Graphical Method (LLRGM) and Gumbel's Analytical Method (GAM) to assess the future flood magnitude for any given Return Period (RP). ... A Case study ...
The below mentioned flowchart shows the outline of the methodology for flood frequency analysis. 3.1 Methods of Flood Frequency Analysis (FFA) The maximum discharge value from the Nehru Bridge, between 1980 and 2020 (41 years) was considered for the FFA by applying the Gumbel's method, Log Pearson Type-III, and Generalized Extreme Value (GEV).
The analysis also reveals that the observed and anticipated peak discharges of the Log Pearson type III (LP3) and Gumble (EV1) models have a positive correlation (R2 = 0.71) and (R2 = 0.52). As a result, the best-fit models for modeling and flood frequency analysis of yearly peak flows on the Pohru River are the LP3 and G(EV1).
Copulas were first used in hydrological studies by De Michele and Salvadori in rainfall frequency analysis. Naz et al. used copula functions to investigate the flood at Tarbela Dam, Pakistan, and developed a method for forecasting floods along the Indus River. Other studies have also been used in flood analysis [17, 25 - 27].
Regional flood frequency analysis (RFFA) is widely used to estimate design floods in ungauged catchments. Both linear and non-linear methods are adopted in RFFA. The development of the non-linear RFFA method Adaptive Neuro-fuzzy Inference System (ANFIS) using data from 181 gauged catchments in south-eastern Australia is presented in this study. Three different types of ANFIS models, Fuzzy C ...
Since their inception a number of approaches to regional flood frequency analysis (RFFA) have emerged, varying from index-flood approaches first described by Dalrymple ... regional flood frequency studies to date have largely been limited to specific regions or climatologies [Meigh et al., ... In the case of the GRDC stations, the shortest ...
A case study for Norway Master thesis in Climate Change Management Author: Simen Norheim Author sign. Thesis submitted: Spring 2018 Open/confidential thesis Main Supervisor: Florian Kobierska Baffie, HVL Co-supervisors: Kolbjørn Engeland, NVE Keywords: Flood frequency analysis, Annual maximum series, Peak over threshold,
A process-based flood frequency analysis within a trivariate statistical framework. Application to a semi-arid Mediterranean case study ... For the extraordinary flood recorded in the case study (October 2000, shown in Fig. 5), hydrological modelling has shown that the peak flow was produced by mixed runoff generation mechanisms, ...
PDF | On Dec 9, 2020, Bibek Acharya and others published Flood frequency analysis for an ungauged Himalayan river basin using different methods: a case study of Modi Khola, Parbat, Nepal | Find ...
The objective of the study is to carry out a flood frequency analysis of the lower Tapi River Basin, Surat, and to assess which method is more suitable for finding the return period of particular ...
The primary objective of this study is to see the efficacy of censoring, by comparing Regional Flood Frequency Analysis (RFFA) using Partial Linear Moments (PLM) for censored samples with RFFA using Linear Moments (LM) for uncensored samples of annual peak flows observed at ten stations of Indus Basin in Pakistan.
frequency analysis and at-site rainfall frequency analysis in different countries of the world. But in the case of flood frequency analysis, data availability is difficult for estimating floods for desired return periods. Therefore, this study uses regional frequency analysis as an alternative to at-site frequency analysis based on LQ-moments.
Flood Frequency Analysis (FFA) is a systematic tool used for an efficient hydraulic structures design, operation and maintenance. An effort is made to study and compare the Linear Log Regression Graphical Method (LLRGM) and Gumbel's Analytical Method (GAM) to assess the future flood magnitude for any given Return Period (RP). Twenty-four years of annual daily peak flood flow value recorded at ...
According to the current Norwegian guidelines for design flood estimation, at least 30 years of data should be available for a local flood frequency analysis, and if less than 50 years of data are available, a two-parameter distribution is recommended (Midttømme et al. 2011; Castellarin et al. 2012). The results in this paper indicate that ...
DOI: 10.35877/jetech489 Corpus ID: 246820840; Bivariate Flood Frequency Analysis: A case study of Rib River, Upper Blue Nile Basin, Ethiopia @article{Haile2022BivariateFF, title={Bivariate Flood Frequency Analysis: A case study of Rib River, Upper Blue Nile Basin, Ethiopia}, author={Mesfin Mamo Haile}, journal={ARRUS Journal of Engineering and Technology}, year={2022}, url={https://api ...
3.2 Studies Reviewed Some of the studies reviewed are as follows: Haktanir et al. (1993) analyzed a statistical model comprising nine different probability distributions used especially for flood frequency analysis was applied to annual flood peak series with at least 30 observations for 11 unregulated streams in the Rhine Basin in Germany and ...
Estimation of Peak Flood Discharge for a desired return period is a pre-requisite for planning, design and management of hydraulic structures like barrages, dams, spillways, bridges etc. This paper presents results of a study carried out at analyzing the frequency of Lower Mahi River floods using the Gumbel's distribution method which is one of the probability distribution methods used to ...
A visual analysis of the series showed the need to decrease variance. Thus, a logarithmic transformation was applied. The R software forecast package was used for model analysis. To capture the seasonality of the data, a frequency of 168 observations (the number of hours in one week) was defined for the time series.
The development of a regional flood frequency analysis (RFFA) method for the semi-arid and arid regions appears to be a difficult task due to paucity of recorded streamflow data and extreme hydrologic variability known to exist in these regions. In the hydrology literature, there is a limited presence of RFFA studies for the world arid regions.
Flood Frequency Analysis Using Gumbel's Distribution Method: A Case Study of Lower Mahi Basin, India. Journal of Water Resources and Ocean Science. Vol. 6, No. 4, 2017, pp. 51-54. doi: 10.11648/j ...
Floods are highly destructive natural disasters. Climate change and urbanization greatly impact their severity and frequency. Understanding flood causes in urban areas is essential due to significant economic and social impacts. Hydrological data and satellite imagery are critical for assessing and managing flood effects. This study uses satellite images, climate anomalies, reservoir data, and ...
To further validate the frequency-response stability analysis method proposed in this study, a comparative analysis is carried out based on a geological field survey and reliability theory.
Many studies in Australia and internationally focused on flood frequency analysis for both at-site and regional cases, and the majority of the studies were based on the AMF modelling approach (Ahammed et al., 2014, Burn et al., 2007, Haddad and Rahman, 2011, Haddad and Rahman, 2012, Haddad et al., 2011, Ishak and Rahman, 2015, Ishak et al ...
Study area. The Budhigandaki Hydropower Project (BGHPP) is a 1200 MW storage type proposed project of Nepal located approximately 2 km upstream of the confluence of Budhigandaki River with Trishuli River as shown in Fig. 1.The Budhigandaki Dam is a 263 m high double curvature concrete arch dam with a reservoir volume of 4.5 billion cubic meters (BCM), out of which the active storage is 2.2 BCM.
The analysis of extreme rainfall parameters, particularly rainfall intensities, plays a serious role in the protection, productivity, and resilience of hydrological systems against storms and floods. This is especially important in arid and semi-arid regions like Pakistan, where inclusive long-term rainfall data with short aggregation periods is limited. Addressing this need, the current study ...
Various stakeholders seek effective methods to communicate the potential impacts of tropical cyclone (TC) rainfall and subsequent flood hazards. While current methods, such as Intensity-Duration-Frequency curves, offer insights, they do not fully capture TC rainfall complexity and variability. This research introduces an innovative workflow utilizing GPM-IMERG satellite precipitation ...
Background & aims Chronic migraine poses a global health burden, particularly affecting young women, and has substantial societal implications. This study aimed to assess the efficacy of Greater Occipital Nerve Block (GONB) in individuals with chronic migraine, focusing on the impact of local anesthetics compared with placebo. Methods A meta-analysis and systematic review were conducted ...