The dual-task practice advantage: Empirical evidence and cognitive mechanisms
- Theoretical Review
- Published: 31 May 2019
- Volume 27 , pages 3–14, ( 2020 )

Cite this article
- Tilo Strobach 1
6402 Accesses
34 Citations
1 Altmetric
Explore all metrics
Practice of two simultaneous component tasks in dual-task situations leads to an improvement in dual-task performance. The present paper reviews empirical evidence for this practice-related improvement and discusses its underlying cognitive mechanisms. In particular, the robustness of the phenomenon of dual-task practice advantage (DTPA) is evaluated. This phenomenon is described as an advantage in dual-task performance at the end of dual-task practice (i.e., simultaneous task practice) in comparison to the dual-task performance after single-task practice (i.e., separate task practice). The review investigates the existence of empirical evidence of the DTPA phenomenon and specifications of its mechanisms in the context of the allocation and scheduling hypothesis and the integration hypothesis. Evidence for the existence of the DTPA phenomenon and the allocation and scheduling hypothesis was demonstrated in dual tasks with (1) combinations of two sensorimotor tasks, (2) combinations of one continuous task and one sensorimotor task, (3) combinations of two working-memory tasks, and (4) combinations of one motor task and one cognitive task. In contrast, the findings demonstrate that the DTPA phenomenon in dual tasks with (5) combinations of two long-term memory retrieval tasks can instead be explained by the integration hypothesis. The relevance of these findings is discussed in relation to real-world problems.
Similar content being viewed by others
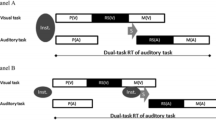
A mechanism underlying improved dual-task performance after practice: Reviewing evidence for the memory hypothesis
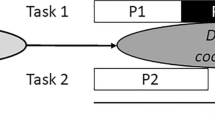
Cognitive control and meta-control in dual-task coordination
Training Based on Multitasking – With a Specific Focus on Motor-Cognitive Multitasking
Avoid common mistakes on your manuscript.
Introduction
When performing two tasks simultaneously, real-world experience and data in systematically conducted lab studies demonstrate performance impairments in comparison to separated task performance in single-task situations. These dual-task costs are demonstrated by an increase in reaction times (RTs) and/or error rates under dual tasks in contrast to single tasks. However, numerous studies have shown a strong (and in rare cases even complete) reduction of these costs after dual-task practice. For instance, a practice-related cost reduction was evident in dual-task situations with combinations of sensorimotor tasks (e.g., Göthe, Oberauer, & Kliegl, 2016 ; Hazeltine, Teague, & Ivry, 2002 ; Kamienkowski, Pashler, Dehaene, & Sigman, 2011 ; Ruthruff, Johnston, & Van Selst, 2001 ; Ruthruff, Johnston, Van Selst, Whitsell, & Remington, 2003 ; Ruthruff, Van Selst, Johnston, & Remington, 2006 ; Van Selst, Ruthruff, & Johnston, 1999 ), working-memory (WM) updating tasks (e.g., Oberauer & Bialkova, 2011 ; Oberauer & Kliegl, 2004 ), memory-retrieval tasks (e.g., Nino & Rickard, 2003 ), and motor tasks (e.g., Wollesen & Voelcker-Rehage, 2013 ).
Typically, high dual-task costs at the beginning of dual-task practice are explained by interference in one or both component tasks due to capacity limitations in the cognitive system (i.e., processing bottlenecks; Logan & Gordon, 2001 ; Meyer & Kieras, 1997 ; Pashler, 1994 ; Townsend & Wenger, 2004 ). Generally, it is assumed that this interference is reduced with practice. However, there is debate as to the details of how this interference is reduced and how cognitive mechanisms explain the reduction of dual-task costs with practice. Strobach and Schubert ( 2017 ) therefore provided a review of several mechanisms within the domain of dual tasks with simple, well-controlled, and discrete sensorimotor tasks. Most of these simple tasks are fairly straightforward; involve a rather small set of stimuli, responses, and thus stimulus-response mappings; and usually take less than a second to complete. The relative simplicity of these tasks allows for testing assumptions regarding precise practice-related mechanisms associated with a reduction of these costs and with improved dual-task performance. However, reviewing practice-related mechanisms within dual tasks only with sensorimotor tasks may limit the validity of the conclusions of Strobach and Schubert to this specific type of dual-task practice. It might be that this dual-task type and its component tasks require different mechanisms for the reduction of dual-task costs than alternative task types and combinations thereof.
A more general contribution of this review is to add to the literature on cognitive plasticity and practice. Theories about underlying cognitive mechanisms that explain practice-related changes are largely lacking and are under-represented in the literature (Strobach & Karbach, 2016 ; Taatgen, 2013 ), and this review aims to provide one step in overcoming this deficit. Such theories should help in gaining knowledge about cognitive practice mechanisms from a scientific point of view, but should also add to solutions of real-world problems (Strobach & Schubert, 2014 ). In many such problems, information technology has greatly increased in complexity recently, in domains such as (air) traffic control, unmanned aircraft systems, and laparoscopic surgery. In these domains, operators need to coordinate the performance of multiple tasks with limited attentional resources. Traffic control, for example, incorporates the surveillance of multiple cameras and sensing equipment by a single operator. Although automation tools are being developed and their net effect will likely be to reduce physical workloads, the cognitive workload increases. This increased workload requires optimal performance, which might result from well-designed practice procedures for operators. These practice procedures, however, require knowledge of the underlying cognitive mechanisms in dual-task practice, which is focused on in this review.
The dual-task practice advantage and its mechanisms
To pursue the aim of looking for mechanisms of improved dual-task performance with practice, the present paper evaluates the robustness of one specific phenomenon: the dual-task practice advantage (DTPA), an idea going back to the empirical findings of Hirst, Spelke, Reaves, Caharack, and Neisser ( 1980 ) as well as to the theoretical assumptions of Damos and Wickens ( 1980 ): “[single-task skills] fail, in short, to account for the development of timesharing skills that may be unique to the multiple-task situation, such as the parallel processing of information, rapid switching between tasks, or the use of efficient response strategies” (p. 16). The DTPA phenomenon can thus be described as an advantage in dual-task performance at the end of dual-task practice in comparison with the dual-task performance after single-task practice, i.e., when component tasks are practiced in separated conditions.
To generate an overarching theoretical contribution, the present review considers two general explanations of cognitive mechanisms underlying the DTPA phenomenon: the allocation and scheduling hypothesis and the integration hypothesis . According to the former, dual-task practice induces participants to adopt a strategy of coordinating the two tasks, which enables them to develop dual-task skills of cognitive resource allocation and scheduling. For instance, such resource allocation and scheduling is generally proposed by the executive-process interactive control theory. In the context of this theory, Meyer and Kieras ( 1997 ) developed an adaptive executive control model to account for dual-task costs. This model assumes that according to specific task demands, the processing stages of two component tasks can be performed either in parallel or sequentially when strategic bottlenecks are introduced. In contrast, alternative structural bottleneck models assume unavoidable bottlenecks at processing stages with mentally demanding, capacity-limited operations (e.g., stimulus-response mapping stages in sensorimotor tasks; Pashler, 1994 ; Pashler & Johnston, 1998 ). However, both strategic and structural models suggest that controlling bottlenecks requires allocation and scheduling processes (e.g., Jiang, Saxe, & Kanwisher, 2004 ; Lien, Schweickert, & Proctor, 2003 ; Szameitat, Lepsien, von Cramon, Sterr, & Schubert, 2006 ; Szameitat, Schubert, Müller, & Von Cramon, 2002 ); for instance, these extensions assume switching processes for inhibition, activation, and instantiation of task information between component tasks and their bottleneck stages (Band & van Nes, 2006 ; Lien et al., 2003 ).
Irrespective of the strategic or structural nature of bottlenecks, the first general prediction of the allocation and scheduling hypothesis is that dual-task practice enables development of the improved dual-task skills of cognitive resource allocation and scheduling (Kieras, Meyer, Ballas, & Lauber, 2000 ; Oberauer & Kliegl, 2004 ; Schumacher et al., 2001 ); the same prediction can be derived from a theory like that proposed by Gopher ( 1993 ), who argued that sharing attention between two tasks is a skill that can be achieved with dual-task practice. One characteristic of such dual-task skills in the context of the allocation and scheduling hypothesis is that this hypothesis does not exclude a generalization of these skills beyond practiced tasks. Hence, these skills could be at least partially independent from the tasks presented during practice. If so, the DTPA phenomenon is transferable to alternative unpracticed dual tasks or other task situations. This assumption of transferable dual-task skills acquired during practice is consistent with the general assumption of transferable cognitive control and executive processes (e.g., Karbach & Kray, 2016 ).
Alternatively, according to the integration hypothesis, dual-task practice might produce an efficient integration of two tasks and combine them, in an extreme case, into a single super task (e.g., Hazeltine, Aparicio, Weinstein, & Ivry, 2007 ; Hazeltine et al., 2002 ; Oberauer & Kliegl, 2004 ; Ruthruff et al., 2006 ). Whereas two separate tasks and their separate capacity-limited processes are performed at the beginning of practice, a single capacity-limited process of the combined task is processed after dual-task practice. The processing of two capacity-limited processes increases the likelihood of dual-task costs (e.g., due to sequential processing of task components), while the likelihood is reduced with only one such process. However, this mechanism proposed in the context of the integration hypothesis should exclusively shape the processing of the two tasks combined during practice since integration exclusively works for task information processed during practice. So, in contrast to the allocation and scheduling hypothesis, the integration hypothesis predicts that the DTPA phenomenon is specific to practiced tasks, is not independent from these tasks, and cannot be transferred to alternative task situations after practice.
Irrespective of the general mechanism explaining the DTPA phenomenon (i.e., the allocation and scheduling hypothesis versus the integration hypothesis), this phenomenon describes mechanisms beyond mechanisms of single-task practice with separate practice of component tasks. Such mechanisms of single-task practice are related to the concept of automatization (e.g., Fisk & Schneider, 1984 ; Logan & Gordon, 2001 ; Schneider & Shiffrin, 1977 ) and the shortening of processing stages in tasks (e.g., Pashler & Baylis, 1991 ) that are combined in dual-task situations (e.g., Dux et al., 2009 ; Kamienkowski et al., 2011 ; Maquestiaux, Laguë-Beauvais, Bherer, & Ruthruff, 2008 ; Ruthruff et al., 2006 ; Strobach, Liepelt, Pashler, Frensch, & Schubert, 2013 ; Van Selst et al., 1999 ). Evidence for these mechanisms (i.e., automatization and shortening of component tasks) comes from studies that demonstrated reduced dual-task costs after separate practice of two tasks in single-task situations. In contrast to dual-task practice, however, processes of allocation and scheduling are not required in single-task situations. So such processes are not practiced and no improved dual-task skills are acquired. Similarly, single-task practice should not lead to an integration of processes of two component tasks because these tasks are not processed simultaneously during this practice type.
The present review
As a consequence of introducing the potential effects of dual-task practice, the explicit aims of this paper are: (1) Is there empirical evidence for the DTPA phenomenon? If so, does this evidence exist across dual-task types that differ with regard to the combined component tasks? (2) If the DTPA phenomenon exists across different dual-task practice types, what are the specific cognitive changes that can explain this phenomenon (i.e., practice mechanisms )? Is there evidence demonstrating transfer effects beyond the practiced dual-task situation to inform about the allocation and scheduling hypothesis versus the integration hypothesis and the mechanisms associated with these hypotheses? These questions will guide the remainder of this paper, across different types of dual tasks and thus different combinations of component tasks: (1) combinations of two sensorimotor tasks, (2) combinations of one continuous task and one sensorimotor task, (3) combinations of two WM tasks, (4) combinations of one motor task and one cognitive task, and (5) combinations of two long-term memory tasks (a literature overview is illustrated in Table 1 ).
Dual-task combinations of two sensorimotor tasks
Empirical evidence.
Empirical findings demonstrating that the DTPA phenomenon is no trivial phenomenon come from a study by Ruthruff et al. ( 2006 ). In that study, the authors investigated the potential existence of this phenomenon in a dual-task situation of the psychological refractory period (PRP) type. In this dual-task type, a first Task 1 (e.g., an auditory sensorimotor task) and a second Task 2 (e.g., a visual sensorimotor task) are separated by a varying time interval (i.e., stimulus onset asynchrony [SOA]), and participants are instructed to respond as fast and as accurately as possible with priority on Task 1. The applied component tasks included a relatively high number of stimulus-response mappings: four plus eight stimulus-response mapping rules in Task 1 and Task 2, respectively, and these mappings were demanding due to a mix of compatible and incompatible mappings between stimulus and response information. Both the number and the compatibility relation of the stimulus-response mappings resulted in highly complex tasks in Ruthruff et al. ( 2006 ). Dual-task costs in PRP dual-task situations are generally indicated by increasing RTs in Task 2 with decreasing SOA. This RT increase is the prominent PRP effect (Pashler, 1994 ; Welford, 1952 ) and is explained by a structural (e.g., Pashler, 1994 ; Welford, 1952 , 1980 ) or a strategic limitation (e.g., Hazeltine, Ruthruff, & Remington, 2006 ; Meyer & Kieras, 1997 ; Miller, Ulrich, & Rolke, 2009 ) in processing two stimulus-response mappings at the same time (Fig. 1 ). Practice-related reductions of the PRP effect would indicate dual-task improvement.
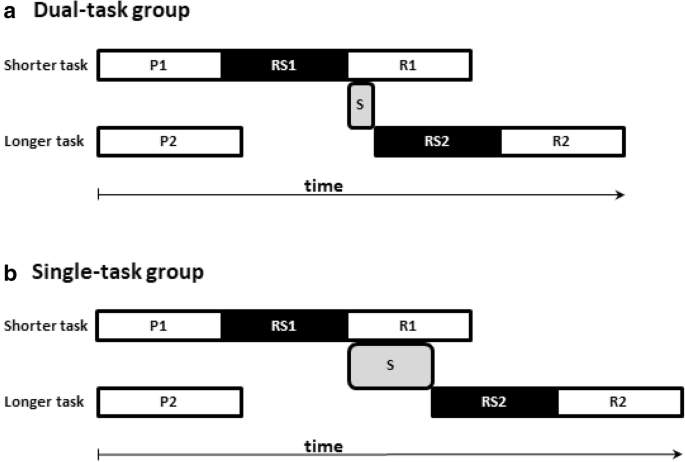
Dual-task processing architecture after dual-task practice (Panel A: Dual-task group) and single-task practice (Panel B: Single-task group) with two sensorimotor tasks. According to bottleneck models, central response-selection (RS) stages in sensorimotor tasks are processed sequentially even with practice (Maquestiaux, Hartley, & Bertsch, 2004 ; Ruthruff et al., 2003 ) while perception (P1, P2) and response (R1, R2) stages are processed in parallel. A potential switching stage (S) after the RS stage in the shorter task (RS1) and before this stage in the longer task (RS2) is shortened after dual-task practice in contrast to single-task practice (Schubert & Strobach, 2018 ). This phenomenon is a promising candidate to explain improved dual-task performance after experience with dual tasks in contrast to this performance after single-task experience (the dual-task practice advantage). Note that the latencies of the individual processing stages (i.e., P1, P2, RS1, RS2, S, R1, R2) in the figure are schematic illustrations and may not represent actual stage latencies of a combination of tasks with a first response (i.e., the visual task) and a second response (i.e., the auditory task) (Liepelt et al., 2011 ; Strobach et al., 2015 ; Strobach et al., 2012 ; Strobach et al., 2014a )
The existence of the DTPA in the PRP context was investigated by Ruthruff et al. ( 2006 ) in three practice groups. While Group 1 practiced Task 1 and Task 2 under PRP dual-task conditions for eight sessions, Group 2 practiced only Task 1, and Group 3 practiced only Task 2 for the same amount of time under single-task conditions. In the final test sessions, all three groups performed the same dual-task situation that Group 1 performed during practice. One of the main findings of the test sessions was the observation of a similar PRP effect in the dual-task Group 1 and the single-task Group 2 that practiced only Task 1 (Ruthruff et al., 2006 ; Group 3 showed still an increased PRP effect). This finding shows no existence of the DTPA phenomenon and is consistent with the assumption that the practice-related improvement of dual-task performance is not related to this phenomenon when combining highly complex sensorimotor tasks.
The first empirical evidence in favor of the DTPA phenomenon was provided by Liepelt, Strobach, Frensch, and Schubert ( 2011 ) as well as Strobach, Frensch, Soutschek, and Schubert ( 2012 ), with combinations of less complex sensorimotor tasks. In their studies, a visual-manual task (i.e., visual task) and an auditory-verbal task (i.e., auditory task) were presented in separate single-task trials and were presented simultaneously in dual-task trials (SOA = 0 ms; Hazeltine et al., 2002 ; Schumacher et al., 2001 ); both component tasks included sets of three stimulus-response pairs and compatible mappings between stimulus and response information. The dual-task costs were investigated as the performance impairment in dual tasks in comparison to single tasks. While dual-task practice included presentations of the visual and auditory tasks in single-task and in dual-task blocks, single-task practice included the presentation of both tasks in single-task blocks exclusively. In fact, after six practice sessions in the dual-task group, dual-task performance was improved, with reduced dual-task costs in the seventh test session when compared to the dual-task performance after six sessions of single-task practice; single-task performance did not differ. In detail, the dual-task cost reduction was exclusively demonstrated by reduced RT costs in the auditory task, while there was no such evidence in the visual task (Liepelt et al., 2011 ; Strobach et al., 2012 ). A comparison of the specific magnitudes of the costs in the auditory task RTs after dual-task practice and single-task practice shows that the dual-task groups produced only 25% and 28% of the costs of the single-task groups in Liepelt et al. ( 2011 ; Experiment 1) and Strobach et al. ( 2012 ; Experiment 1), respectively. Since the auditory task is typically executed after the visual task (i.e., the auditory task is performed after the visual task; see also Hartley, Maquestiaux, & Butts, 2011 ; Schumacher et al., 2001 ; Strobach, Frensch, & Schubert, 2008 ; Tombu & Jolicœur, 2004 ), the DTPA is associated with the secondly performed component task in this dual-task situation.
Evidence for the existence of the DTPA phenomenon in the studies of Liepelt et al. ( 2011 ) and Strobach et al. ( 2012 ) was demonstrated in young adults (aged between 19 and 29 years). However, Strobach, Frensch, Müller, and Schubert ( 2015 ) showed that this phenomenon in dual tasks with a combination of two sensorimotor tasks is age-independent to some degree. Dual-task practice in comparison to single-task practice in older adults (aged between 57 and 68 years) also resulted in reduced dual-task costs in the RTs of the longer auditory component task; a comparison of the specific magnitudes of the costs shows that the dual-task group produced only 43% of the costs in the single-task group.
Practice mechanisms
What are the practice-related mechanisms that might explain the DTPA phenomenon in dual tasks with two less complex sensorimotor tasks? To answer this question, it is important to look for evidence of transfer beyond the practiced tasks. If there is transfer, this finding would support the allocation and scheduling hypothesis, while no evidence of transfer is consistent with the assumptions of the integration hypothesis. Importantly, findings showed improved dual-task performance in dual-task groups in comparison to single-task groups when the identical components are presented during practice and during the dual-task test at the end of practice (as shown in Experiment 1 of Liepelt et al., 2011 ; Strobach et al., 2015 ; Strobach et al., 2012 ). However, this DTPA phenomenon was also evident when stimuli and stimulus-response mappings of the visual and/or the auditory task were changed in the final dual-task tests (Liepelt et al., 2011 , Experiments 2 & 3; Schubert, Liepelt, Kübler, & Strobach, 2017 ; Strobach et al., 2015 ); thus, the DTPA phenomenon was independent of the specific perceptual and stimulus-response mapping processes in these tasks. These findings are not consistent with the assumption that both tasks were integrated into one super-task representation, which leaves only one integrated bottleneck (e.g., at the response selection mechanism).
This transfer of the DTPA phenomenon to changed dual tasks, however, is predicted by the allocation and scheduling hypothesis and its assumption of acquired and improved dual-task skills (Hirst et al., 1980 ; Kramer, Larish, & Strayer, 1995 ). As illustrated in Fig. 1 , one specific realization of these skills that explains the DTPA phenomenon in the longer auditory task might be as follows: The dual-task processing architecture includes (1) a capacity limitation (i.e., a bottleneck process) in the shorter visual task (e.g., at a central response selection stage) followed by (2) a switching operation (related to the inhibition of mapping rules of irrelevant tasks as well as activation and instantiation of such rules in relevant tasks such as the longer auditory task in WM), and (3) the capacity limitation in the longer auditory task (Band & van Nes, 2006 ; Lien et al., 2003 ). After dual-task practice (Fig. 1A ) in contrast to single-task practice (Fig. 1B ), inhibition, activation, and instantiation processes are highly efficient, leading to a shortening of the switching operation and improved dual-task performance in the longer auditory task (Strobach, Salminen, Karbach, & Schubert, 2014a , for a more detailed discussion). A theoretically important aspect is that the DTPA phenomenon might be limited to less complex tasks, but cannot be generalized to highly complex tasks (Ruthruff et al., 2006 ; Schubert & Strobach, 2018 ). Less complex tasks put a rather low load on WM capacity, which enables participants to acquire and apply dual-task skills, while highly complex tasks with a high load on WM capacity enable no such skill acquisition and application (Schubert & Strobach, 2018 ).
Dual-task combinations of two working memory (WM) tasks
An early study investigating the DTPA phenomenon in the context of dual tasks with two WM tasks was published by Oberauer and Kliegl ( 2004 ). In this study, young participants practiced updating two items in WM through two sequences of operations (one numerical operation: a high tone requiring the addition of 2 and a low tone subtraction of 1 from the current value of a digit; one spatial operation: spatial operations were indicated by visually presented red arrows, displayed in the central cell of a grid and pointing in one of eight possible directions horizontally, vertically, or diagonally; these arrows required the mental shift of a dot from its current position in the indicated direction by one cell). In different blocks, stimuli for the two sequences of operations were presented either separately in alternating order or simultaneously. At the beginning of the dual-task practice, participants experienced substantial dual-task costs which might result from the capacity-limited nature of the WM component as well as the processes to coordinate different sets of information in this component. However, at the end of six to 12 practice sessions, operation latencies for simultaneous processing were similar to the latencies for the two operations in the alternating single-task condition, suggesting improved dual-task performance. Important for the present context, this dual-task improvement increased the improvement after separate single-task practice of the numerical and spatial operations (Oberauer & Kliegl, 2004 ), demonstrating the DTPA phenomenon with two WM tasks. A comparison of the specific magnitudes of the dual-task costs in these tasks after dual-task practice and single-task practice (Oberauer & Kliegl, 2004 ) shows that the dual-task group produced only about 14% of the costs of the single-task group.
Such a demonstration was also evident in a study combining two n -back WM tasks (Salminen, Kühn, Frensch, & Schubert, 2016 ); in such n -back tasks, sequences of stimuli are presented and participants have to decide for each sequence whether the current stimulus matches the one that was presented n stimuli back in the sequence. In detail, two groups of participants practiced with n -back tasks, including visual position and auditory letter stimuli, for 16 days (Jaeggi, Buschkuehl, Jonides, & Perrig, 2008 ; see Soveri, Antfolk, Karlsson, Salo, & Laine, 2017 , for a recent meta-analysis). While the dual-task group practiced both tasks simultaneously, there was isolated practice of the visual and the auditory n -back tasks in the single-task group. In both groups, the n -back situations were designed in an adaptive way so that, when participants responded correctly in most of the trials (e.g., 18 correct responses in 20 trials), the level of n increased by one (e.g., participants were advanced from 2-back to 3-back). In contrast, it was decreased by one (e.g., from 3-back to 2-back), if participants performed a lower number of correct responses (e.g., less than 15 correct responses in 20 trials), while the n -back level remained constant in all other cases. As a consequence, the achieved n -back level under the dual-task condition is a valid indicator of practiced dual-task performance in the n -back task.
To test transfer, a WM updating task with sequences of items was presented and the length of the sequences varied randomly. Participants were unaware of the length of the ongoing sequence and were asked to constantly update the contents of their WM. Under single-task conditions, sequences included either auditory numbers or visual bars at different locations, and participants had to report the last four numbers or bar locations, respectively. Under dual-task conditions, sequences of auditory and visual items were presented simultaneously and participants were asked to report either the auditory items or the visual items; item modality was unpredictable before the end of the sequences. The WM updating task can be considered a transfer task since the practiced n -back and the updating task differed with respect to stimuli (squares and letters in the n -back tasks versus digits and stimulus locations in the updating tasks) and task rules; the n -back task requires the recognition of previously presented items while the updating task requires recollection and reproduction of item information.
When comparing the achieved n -back level in the practice task at the end of practice in the dual-task and single-task group, there was improved dual n -back performance in the former group while single n -back performance did not differ (Salminen et al., 2016 ); a comparison of the specific magnitudes of the dual-task costs in the achieved n -back level after dual-task practice and single-task practice shows that the dual-task group actually produced a dual-task benefit of 96% of these costs in the single-task group (Table 1 ). Thus, this study validated the DTPA phenomenon in dual tasks with two WM tasks. This validation was not limited to the practiced n -back task, but was also evident in the WM updating transfer tasks. While the dual-task group showed improved performance under the dual-task updating condition at the end of practice (i.e., the number of correctly reported items), there was no such improvement in the single-task conditions in comparison to the single-task group.
Oberauer and Kliegl ( 2004 ) assumed that the DTPA phenomenon could be explained by learning to combine two WM tasks. However, their design was not able to validate this hypothesis in contrast to the allocation and scheduling hypothesis because the authors conducted no transfer conditions. Such conditions are required to test for the acquisition of dual-task skills in the context of this latter hypothesis.
Accordingly, Salminen et al. ( 2016 ) strongly focused on the potential transfer effects to non-practiced tasks after dual-task practice with two simultaneous WM tasks. The analysis of these transfer effects might show which non-practiced tasks benefit from practice and might offer a window onto the shared processing components between practiced and non-practiced tasks; such a benefit might also inform about the cognitive changes, explaining the DTPA phenomenon. In fact, the practice of Salminen et al. produced evidence for transfer: The DTPA transferred to the dual-task condition of the non-trained WM updating task, but not to single-task versions of this updating task and the n -back single tasks; the single-task group showed no such transfer to the non-practiced dual-WM updating task. Thus, dual n -back practice improved skills that are not specific to the practiced task situation but are transferable. These transferable skills explain the DTPA phenomenon in dual n -back tasks in the context of the allocation and scheduling hypothesis, while these skills are inconsistent with the integration hypothesis that does not predict any transfer beyond the practice tasks. These acquired skills optimize processes generalizable to all types of “WM updating of stimuli from two modalities” (Salminen et al., 2016 , p. 10198); such skills are not required when only one stimulus is processed during single n -back practice. In the context of multicomponent WM models, such generalizable skills could be conceptualized in an executive control module that coordinates updating of stimulus processing in different short-term memory components. For the exemplary case of Baddeley’s ( 2012 ) WM model, a central executive applies these skills on a phonological loop (to update the auditory letter information) and on a visuo-spatial sketch pad (to process the visual position information).
Dual-task combinations of one motor task and one cognitive task
Silsupadol et al. ( 2009 ) investigated both the impairment of and the effects of practice on the control of motor balance under dual-task conditions in older adults. Because impaired dual-task balance performance predicts adverse outcomes like falling as well as declines in both cognitive and physical functioning, interventions that improve dual-task balance performance are crucial and inform about real-world problems. During the dual-task practice of this study, different balance tasks, such as standing with eyes closed, walking backward, and catching a ball, were performed simultaneously with cognitive tasks (e.g., naming objects or remembering numbers), while the balance tasks were practiced separately during single-task practice. After practice, dual-task performance on balance was tested in combination with a set of other cognitive tasks, such as addition/subtraction questions. In this balance test after practice, participants had to walk 10 m and the time to complete the middle 6 m (i.e., gait speed in m/sec) in combination with the cognitive tasks was measured. Dual-task costs in the balance task at low practice levels might be explained by interference between this task and the cognitive tasks because of capacity-limited attentional requirements of locomotor and cognitive control (Woollacott & Shumway-Cook, 2002 ).
In essence, dual-task gait speed was increased after dual-task practice in comparison to single-task practice effects during the balance test (Silsupadol et al., 2009 ). Such an increase was not evident under single-task conditions, reflecting the DTPA phenomenon; a comparison of the specific magnitudes of the dual-task costs in gait speed after dual-task practice and single-task practice shows that the dual-task practice condition produced only 81% of the costs of single-task practice conditions. These findings replicate and extend the findings of a prior single case study (Silsupadol, Siu, Shumway-Cook, & Woollacott, 2006 ). However, a recent study was not able to replicate the DTPA phenomenon in patients with Parkinson’s disease (Perumal, Melam, Alhusaini, Buragadda, & Sharma, 2017 ). The results of this study showed that dual-task practice is not more effective than single-task practice in improving dual-task balance performance of these patients. Thus, similar to dual tasks with two sensorimotor tasks (Ruthruff et al., 2006 ), the DTPA phenomenon is no trivial finding and is not generalizable to all conditions. One might speculate that patients with Parkinson’s disease do not possess the potential capacity and plasticity as non-patients do to produce a DTPA.
What is the specific cognitive change that explains faster gait speed in dual tasks after dual-task practice (i.e., the DTPA phenomenon)? Silsupadol et al. ( 2009 ) assumed that their findings support the assumption of the development of dual-task skills for the optimized attentional control of balance and cognitive tasks during dual-task practice. This assumption is based on the finding that the tasks during balance practice and balance test differed (e.g., balance tasks: standing with eyes closed, catching a ball vs. walking/cognitive tasks: naming objects vs. addition questions). So the DTPA phenomenon was evident in an unpracticed transfer task at the final test. These findings show that potentially acquired dual-task skills are not tied to the specific practice tasks, but are transferable between dual-task situations with different balance tasks. These conclusions are consistent with the allocation and scheduling hypothesis when explaining the DTPA phenomenon in the context of combinations of a motor task and a cognitive task. In contrast, these conclusions are not consistent with the integration hypothesis, which does not predict such transfer between different situations.
Dual-task combinations of one continuous task and one sensorimotor task
Dual tasks combining one continuous task and one sensorimotor task were realized in situations with a video race game and a detection task, respectively (Anguera et al., 2013 ). The participants with the video race game were instructed to track a car on a simulated road with left/right turns as well as uphill/downhill sections, while the participants of the detection task were instructed to execute a response when a green sign, among red signs, was presented. Older adults in a dual-task group and a single-task group practiced both tasks either simultaneously or in separate single-task blocks, respectively, across 12 sessions and 4 weeks. Dual-task costs in the detection task (i.e., signal-detection performance under single tasks in comparison to dual tasks) were substantial in both the dual-task and the single-task group at the beginning of practice and vaguely explained by interference between tasks. These costs were, however, reduced in the dual-task group in contrast to the single-task group at the end of practice (Anguera et al., 2013 ); a comparison of the specific magnitudes of the dual-task costs after dual-task practice and single-task practice shows that the dual-task group produced only 37% of the costs in the single-task group. This study thus realized the DTPA phenomenon with one continuous task and one sensorimotor task, and the phenomenon was even evident in a follow-up after 6 months.
Bender, Filmer, Naughtin, and Dux ( 2017 ) extended the findings of Anguera et al. ( 2013 ) to a group of young and middle-aged participants and a new set of continuous and sensorimotor tasks. That is, across six sessions of practice, a dual-task and a single-task practice group performed a visuomotor-tracking task and a shape-discrimination task simultaneously and separately, respectively. In the visuomotor-tracking task, participants had to continuously pursue a visually presented moving black target with a computer mouse so that a black cursor remained as close as possible to the center of the moving target, while those in the discrimination task were instructed to detect a colored shape target among distractor stimuli. The data across these component tasks demonstrated similar dual-task costs in the single-task group and the dual-task group before practice. After practice, these costs were mainly reduced in the discrimination task in the dual-task group compared to the single-task group (the former group produced only about 55% of the costs in the latter group), illustrating the DTPA phenomenon.
To investigate the specific cognitive changes that might explain the DTPA phenomenon in dual tasks with a continuous and a sensorimotor task, the study by Anguera et al. ( 2013 ) provides the opportunity to analyze the effects beyond the practiced task situation. In such non-practiced transfer situations, the dual-task group, in comparison to the single-task group, showed significantly improved performance in the domains of WM and sustained attention; the former domain is investigated by a delayed-recognition task, while the latter is measured by more rapid responses in a vigilance test. However, these transfer improvements were limited to WM and sustained attention processes, and were not the result of generalized increases in speed of processing, as the dual-task practice group of Anguera et al. did not improve in two tests of processing speed. Further, there was no significant improvement in a letter-number dual-task test (multitasking with two sensorimotor tasks), in the useful field of view test (visual attention), as well as in a change-detection task (visual short-term memory). The lack of improvement is consistent with the transfer investigations of Bender et al. ( 2017 ) in a non-practiced PRP dual task that showed no better dual-task performance in the dual-task group in contrast to the single-task group. These authors were also not able to show transfer effects on an attentional blink test, Stroop task, Flanker task, or Go–No-go task (investigating inhibition and action control).
Importantly, however, the transfer effects to WM and sustained attention (Anguera et al., 2013 ) suggest that a common, underlying mechanism of cognitive coordination was challenged and enhanced by dual tasks with one continuous and one sensorimotor task; however, this common mechanism is not fully general and does not boost performance in alternative domains, for example visual attention. Focused on the aim to specify practice-related mechanisms explaining the DTPA phenomenon, these findings thus suggest that practice with simultaneous continuous and sensorimotor tasks improves dual-task skills that are not specific for the practice situation, but this practice improves skills that are transferable to some extent (at least to processes of WM and sustained attention). These conclusions on the transferability of these skills are consistent with the allocation and scheduling hypothesis and are inconsistent with assumptions of the integration hypothesis.
Dual-task combinations of two long-term memory tasks
The investigation of the DTPA phenomenon and its transfer effects in the context with two long-term memory tasks has a crucial difference from the previously reported dual-task combinations in that dual-task practice and single-task practice were not realized in different groups of participants. In this case, dual-task and single-task practice were realized in the same of group of participants, but in different sets of cues on which long-term memory practice was performed (Orscheschek, Strobach, Schubert, & Rickard, 2019 ; Strobach, Schubert, Pashler, & Rickard, 2014b ). Footnote 1 In detail, participants had to associate each of a set of 14 color-word cues with a unique spatial key-press or a unique vocal-digit response in the beginning of practice on the long-term memory tasks. After forming these associations, participants were given multiple triads of blocks of retrieval practice. Within each triad, there was one single-retrieval key-press block, one single-retrieval vocal-digit block, and one dual-retrieval block; dual-retrieval blocks required participants to execute both the key-press and the vocal-digit responses. Importantly, one set of seven cues (old cues) received practice under single- and dual-retrieval conditions, while the other set of seven cues was practiced under single-retrieval conditions exclusively (new cues).
At the beginning of practice, RTs of the first and second responses on old cues in dual-retrieval blocks (i.e., RT1 and RT2, respectively) were above the single-retrieval RTs in the vocal-digit and key-press blocks. Dual-retrieval RTs above single-retrieval RTs could be explained by a bottleneck at the memory retrieval stage of processing and only sequential retrieval of information on two responses from long-term memory. During practice, however, a subgroup of participants showed an RT pattern on old cues with short inter-response intervals (IRIs) between RT1 and RT2, and these participants gave very reduced RT2s at the end of three practice sessions, indicative of improved dual-retrieval performance. Since RT2s violated the lower bound prediction of a mathematical sequential retrieval model, some form of learned parallelism was acquired by this subgroup of participants. In a transfer phase following this learned retrieval parallelism at the end of the practice, old and new cues were presented intermixed in the same single- and dual-retrieval blocks. RT2 on old cues still demonstrated learned retrieval parallelism, while RT2 on new cues did not, which was consistent with a sequential retrieval assumption; single-task RTs of old and new cues did not show a difference during the transfer phase (Strobach, Schubert, et al., 2014 ). A comparison of the specific magnitudes of the dual-task costs after dual-retrieval practice shows that participants actually produced a dual-task benefit of about 224% on old cues in comparison to the costs on new cues. Thus, dual tasks with two long-term memory retrieval tasks showed the DTPA phenomenon on old cues with dual-retrieval practice in comparison to new cues without such practice.
The findings of the dual-retrieval practice studies (Orscheschek et al., 2019 ; Strobach, Schubert, et al., 2014 ) are incompatible with assumptions of the allocation and scheduling hypothesis since there is no evidence for transfer of learned retrieval parallelism between old and new cues within the same blocks of dual-memory retrieval (i.e., old and new cues were presented under very similar conditions). Alternatively, the literature on dual tasks with two long-term memory tasks discusses one variation of the integration hypothesis. According to this discussion, participants with reduced IRIs learned to chunk the two responses independently for each cue, such that both responses could be retrieved in one pass through a potential retrieval bottleneck that appears to govern performance at the beginning of the practice (Nino & Rickard, 2003 ). That chunking process may only be possible when both responses are concurrently in WM, as should be the case for old cues and low IRIs on their responses. Because response chunking is an associative memory process that would occur during a dual-retrieval trial, this response chunking account suggests that learned retrieval parallelism occurs at the individual cue-response level (i.e., for old cues only) rather than at the task level (i.e., for old and new cues). Consistent with that possibility, Orscheschek et al. ( 2019 ) and Strobach, Schubert, et al. ( 2014 ) have shown that this parallelism did not transfer to cues for which only single-retrieval practice occurred during the practice phase, with dual-retrieval trials introduced only during a transfer phase. In this way, empirical evidence in dual tasks with two long-term memory retrieval tasks showed the existence of the DTPA phenomenon and provided evidence that is consistent with the integration hypothesis (i.e., the chunking mechanism; Nino & Rickard, 2003 ). Footnote 2
Conclusions
The aims of the present paper were to evaluate the robustness of the DTPA phenomenon, assuming an advantage in dual-task performance at the end of dual-task practice in comparison with the dual-task performance after single-task practice, as well as to specify mechanisms explaining this phenomenon. As a first approach to specify practice-related mechanisms in an overarching theoretical framework, findings consistent with the allocation and scheduling hypothesis versus the integration hypothesis were looked for. The DTPA phenomenon in dual tasks was investigated with (1) combinations of two sensorimotor tasks, (2) combinations of one continuous and one sensorimotor task, (3) combinations of two WM tasks, (4) combinations of one motor and one cognitive task, and (5) combinations of two long-term memory tasks.
First, all considered types of dual tasks and age groups provided empirical evidence consistent with the existence of the DTPA phenomenon. Thus, this phenomenon is robust across dual-task types and is age-independent to some degree, since the DTPA occurred in young and older adults. So empirical evidence for the DTPA phenomenon is generally consistent with the theoretical assumptions of Damos and Wickens ( 1980 ). The specific magnitudes of the DTPA phenomenon differ tremendously from dual-task benefits after dual-task practice (i.e., improved dual-task performance in comparison to single-task performance after dual-task practice, while single-task practice produced dual-task costs as in dual-task combinations of two WM tasks) to only small DTPAs (i.e., slightly reduced dual-task costs after dual-task practice in comparison to these costs after single-task practice as in dual-task combinations of one motor task and one cognitive task).
Second, the review of potential mechanisms explaining the DTPA phenomenon generally showed empirical evidence consistent with the acquisition of dual-task skills in the context of the allocation and scheduling hypothesis in dual tasks with (1) combinations of two sensorimotor tasks, (2) combinations of one continuous task and one sensorimotor task, (3) combinations of two WM tasks, and (4) combinations of one motor task and one cognitive task. However, the specifications of these skills across the different types of dual-task practice are as diverse as the number of these situations at present, that is, there is low generalizability of the specific mechanisms explaining dual-task skills in the context of the allocation and scheduling hypothesis (e.g., combinations of two sensorimotor tasks: skills for a faster switch between central response-selection stages; combinations of two WM tasks: improved updating of stimuli from two modalities in WM).
These conclusions differ partly from those of studies combining two long-term memory tasks. Such studies also produced evidence consistent with the assumption of the DTPA phenomenon. However, they offer no evidence that is consistent with the acquisition of dual-task skills (Strobach, Schubert, et al., 2014 ). Rather, findings in studies with two long-term memory tasks supported the assumption of chunking of two memory retrievals, thus the integration and restructuring of the architecture of the long-term memory tasks. So the integration hypothesis provides a promising practice-related mechanism in the context of dual tasks with two long-term memory tasks.
Differences between the consequences of the different dual-task practice types reviewed in this paper call for future studies that include similar empirical tests of their effects. Such studies could, for instance, investigate the consequences of the different practice types in the same set of transfer tasks. These transfer tasks should be designed in a way in which they allow for an elaborated analysis of the underlying cognitive mechanisms that explain the DTPA phenomenon across different practice types, thus allowing for a specification of the allocation and scheduling hypothesis.
Referring to real-world problems, the present review can provide some suggestions. First, although the real-world situations with multiple tasks might differ from the ones investigated in the reviewed studies, it might be that also real-world dual-task situations and practice in these situations could show the DTPA phenomenon. From the author’s perspective, studies on situations with one continuous task and one sensorimotor task (Anguera et al., 2013 ; Bender et al., 2017 ) represent a first step from lab research towards modeling real-world dual-task situations. Second, if the DTPA phenomenon also exists in real-world problems, this suggests practicing these problems’ multiple tasks in dual tasks, but not in isolation as in single-task practice. Third, investigating practice combinations with two long-term memory tasks produced no evidence for transferable dual-task skills (i.e., this practice type provided evidence for the integration hypothesis), while findings in studies with other combinations were consistent with the allocation and scheduling hypothesis. That is, practicing these task combinations leads to the acquisition of improved dual-task skills that are not specific for the practice situations, but are at least partially independent of these situations and are transferable. Although the findings on the characteristics of transfer are diverse across the different types of dual-task practice, it is plausible that a dual-task practice is transferable to and between real-world problems. From a broader perspective, the present review on the DTPA phenomenon adds to the general literature on cognitive plasticity and practice. That is, this review specifies theoretical assumptions about the underlying cognitive mechanisms in the context of the allocation and scheduling hypothesis as well as the integration hypothesis that explain changes after dual-task practice in contrast to single-task practice.
Nino and Rickard ( 2003 ) investigated the effects of single-retrieval practice and dual-retrieval practice. Although they produced findings consistent with the assumption of the DTPA phenomenon, they realized these types of practice in different experiments and different designs that do not warrant a systematic comparison of both practice types and their effects.
Note that the absence of an empirical generalization to other cues is consistent with the integration hypothesis. Although this absence is not consistent with the assumptions of the allocation and scheduling hypothesis, it does not conclusively allow a differentiation between this hypothesis and the integration hypothesis, because it might be that learning in the context of the allocation and scheduling hypothesis is (for some reason) specific to the practiced long-term memory tasks or that the selection of another set of tests would produce such a transfer. However, it is assumed that this latter argument is rather unlikely in the present context, since if this would be the case then the specificity would have been highly focused and skills would not generalize to cues within the same set of cues, presented in the same blocks, and with the same instructions.
Anguera, J. A., Boccanfuso, J., Rintoul, J. L., Al-Hashimi, O., Faraji, F., Janowich, J., . . . Johnston, E. (2013). Video game training enhances cognitive control in older adults. Nature, 501 (7465), 97-101.
Article PubMed PubMed Central Google Scholar
Baddeley, A. (2012). Working memory: theories, models, and controversies. Annual Review of Psychology, 63 , 1-29.
Article PubMed Google Scholar
Band, G. P., & van Nes, F. T. (2006). Reconfiguration and the bottleneck: Does task switching affect the refractory period effect? European Journal of Cognitive Psychology, 18 (4), 593-623.
Article Google Scholar
Bender, A. D., Filmer, H. L., Naughtin, C. K., & Dux, P. E. (2017). Dynamic, continuous multitasking training leads to task-specific improvements but does not transfer across action selection tasks. npj Science of Learning, 2: 14 .
Damos, D. L., & Wickens, C. D. (1980). The identification and transfer of timesharing skills. Acta Psychologica, 46 (1), 15-39.
Dux, P. E., Tombu, M. N., Harrison, S., Rogers, B. P., Tong, F., & Marois, R. (2009). Training improves multitasking performance by increasing the speed of information processing in human prefrontal cortex. Neuron, 63 (1), 127-138.
Fisk, A. D., & Schneider, W. (1984). Memory as a function of attention, level of processing, and automatization. Journal of Experimental Psychology: Learning, Memory, and Cognition, 10 (2), 181-197.
PubMed Google Scholar
Gopher, D. (1993). The skill of attention control: Acquisition and execution of attention strategies. In D. E. Meyer & S. Kornblum (Eds.), Attention and performance XIV: Synergies in experimental psychology, artificial intelligence, and cognitive neuroscience (pp. 299-322). Cambridge, MA: MIT Press.
Google Scholar
Göthe, K., Oberauer, K., & Kliegl, R. (2016). Eliminating dual-task costs by minimizing crosstalk between tasks: The role of modality and feature pairings. Cognition, 150 , 92-108.
Hartley, A. A., Maquestiaux, F., & Butts, N. S. (2011). A demonstration of dual-task performance without interference in some older adults. Psychology and Aging, 26 (1), 181-187.
Hazeltine, E., Aparicio, P., Weinstein, A., & Ivry, R. B. (2007). Configural response learning: The acquisition of a nonpredictive motor skill. Journal of Experimental Psychology: Human Perception and Performance, 33 (6), 1451-1467.
Hazeltine, E., Ruthruff, E., & Remington, R. W. (2006). The role of input and output modality pairings in dual-task performance: Evidence for content-dependent central interference. Cognitive Psychology, 52 (4), 291-345.
Hazeltine, E., Teague, D., & Ivry, R. B. (2002). Simultaneous dual-task performance reveals parallel response selection after practice. Journal of Experimental Psychology: Human Perception and Performance, 28 (3), 527.
Hirst, W., Spelke, E. S., Reaves, C. C., Caharack, G., & Neisser, U. (1980). Dividing attention without alternation or automaticity. Journal of Experimental Psychology: General, 109 (1), 98.
Jaeggi, S. M., Buschkuehl, M., Jonides, J., & Perrig, W. J. (2008). Improving fluid intelligence with training on working memory. Proceedings of the National Academy of Sciences, 105 (19), 6829-6833.
Jiang, Y., Saxe, R., & Kanwisher, N. (2004). Functional magnetic resonance imaging provides new constraints on theories of the psychological refractory period. Psychological Science, 15 (6), 390-396.
Kamienkowski, J. E., Pashler, H., Dehaene, S., & Sigman, M. (2011). Effects of practice on task architecture: Combined evidence from interference experiments and random-walk models of decision making. Cognition, 119 (1), 81-95.
Karbach, J., & Kray, J. (2016). Executive functions. In T. Strobach & J. Karbach (Eds.), Cognitive training – An overview of features and applications (pp. 93-103). New York, NY: Springer.
Kieras, D. E., Meyer, D. E., Ballas, J. A., & Lauber, E. J. (2000). Modern computational perspectives on executive mental processes and cognitive control: Where to from here. In S. Monsell & J. Driver (Eds.), Control of cognitive processes: Attention and performance XVIII (pp. 681-712). Cambridge, MA: MIT Press.
Kramer, A. F., Larish, J. F., & Strayer, D. L. (1995). Training for attentional control in dual task settings: a comparison of young and old adults. Journal of Experimental Psychology: Applied, 1 (1), 50-76.
Lien, M.-C., Schweickert, R., & Proctor, R. W. (2003). Task switching and response correspondence in the psychological refractory period paradigm. Journal of Experimental Psychology: Human Perception and Performance, 29 (3), 692-712.
Liepelt, R., Strobach, T., Frensch, P., & Schubert, T. (2011). Improved intertask coordination after extensive dual-task practice. The Quarterly Journal of Experimental Psychology, 64 (7), 1251-1272.
Logan, G. D., & Gordon, R. D. (2001). Executive control of visual attention in dual-task situations. Psychological Review, 108 (2), 393.
Maquestiaux, F., Hartley, A. A., & Bertsch, J. (2004). Can practice overcome age-related differences in the psychological refractory period effect? Psychology and Aging, 19 (4), 649-667.
Maquestiaux, F., Laguë-Beauvais, M., Bherer, L., & Ruthruff, E. (2008). Bypassing the central bottleneck after single-task practice in the psychological refractory period paradigm: Evidence for task automatization and greedy resource recruitment. Memory & Cognition, 36 (7), 1262-1282.
Meyer, D. E., & Kieras, D. E. (1997). A computational theory of executive cognitive processes and multiple-task performance: Part 2. Accounts of psychological refractory-period phenomena. Psychological Review, 104 (4), 749-791.
Miller, J., Ulrich, R., & Rolke, B. (2009). On the optimality of serial and parallel processing in the psychological refractory period paradigm: Effects of the distribution of stimulus onset asynchronies. Cognitive Psychology, 58 (3), 273-310.
Nino, R. S., & Rickard, T. C. (2003). Practice effects on two memory retrievals from a single cue. Journal of Experimental Psychology: Learning, Memory, and Cognition, 29 (3), 373.
Oberauer, K., & Bialkova, S. (2011). Serial and parallel processes in working memory after practice. Journal of Experimental Psychology: Human Perception and Performance, 37 (2), 606-614.
Oberauer, K., & Kliegl, R. (2004). Simultaneous cognitive operations in working memory after dual-task practice. Journal of Experimental Psychology: Human Perception and Performance, 30 (4), 689-707.
Orscheschek, F., Strobach, T., Schubert, T., & Rickard, T. (2019). Two retrievals from a single cue: A bottleneck persists across episodic and semantic memory. Quarterly Journal of Experimental Psychology, 72 (5), 1005-1028.
Pashler, H. (1994). Dual-task interference in simple tasks: Data and theory. Psychological Bulletin, 116 (2), 220-244.
Pashler, H., & Baylis, G. (1991). Procedural learning: I. Locus of practice effects in speeded choice tasks. Journal of Experimental Psychology: Learning, Memory and Cognition, 17 , 20-32.
Pashler, H., & Johnston, J. C. (1998). Attentional limitations in dual-task performance. In H. Pashler (Ed.), Attention (pp. 155-189). Hove, England: Psychology Press/Erlbaum (Uk) Taylor & Francis.
Perumal, V., Melam, G. R., Alhusaini, A. A., Buragadda, S., & Sharma, N. (2017). Instruction prioritization in task-based balance training for individuals with idiopathic Parkinson’s disease. Somatosensory & Motor Research, 34 (1), 27-33.
Ruthruff, E., Johnston, J. C., & Van Selst, M. (2001). Why practice reduces dual-task interference. Journal of Experimental Psychology: Human Perception and Performance, 27 (1), 3.
Ruthruff, E., Johnston, J. C., Van Selst, M., Whitsell, S., & Remington, R. (2003). Vanishing dual-task interference after practice: Has the bottleneck been eliminated or is it merely latent? Journal of Experimental Psychology: Human Perception and Performance, 29 (2), 280 - 289.
Ruthruff, E., Van Selst, M., Johnston, J. C., & Remington, R. (2006). How does practice reduce dual-task interference: Integration, automatization, or just stage-shortening? Psychological Research, 70 (2), 125-142.
Salminen, T., Kühn, S., Frensch, P. A., & Schubert, T. (2016). Transfer after Dual n-Back Training Depends on Striatal Activation Change. Journal of Neuroscience, 36 (39), 10198-10213.
Schneider, W., & Shiffrin, R. M. (1977). Controlled and automatic human information processing: I. Detection, search, and attention. Psychological Review, 84 (1), 1-57.
Schubert, T., Liepelt, R., Kübler, S., & Strobach, T. (2017). Transferability of dual-task coordination skills after practice with changing component tasks. Frontiers in Psychology, 8:956 .
Schubert, T., & Strobach, T. (2018). Practice-related optimization of dual-task performance: Efficient task instantiation during overlapping task processing. Journal of Experimental Psychology: Human Perception and Performance, 44 (12), 1884-1904.
Schumacher, E. H., Seymour, T. L., Glass, J. M., Fencsik, D. E., Lauber, E. J., Kieras, D. E., & Meyer, D. E. (2001). Virtually perfect time sharing in dual-task performance: Uncorking the central cognitive bottleneck. Psychological Science, 12 (2), 101-108.
Silsupadol, P., Shumway-Cook, A., Lugade, V., van Donkelaar, P., Chou, L.-S., Mayr, U., & Woollacott, M. H. (2009). Effects of single-task versus dual-task training on balance performance in older adults: a double-blind, randomized controlled trial. Archives of Physical Medicine and Rehabilitation, 90 (3), 381-387.
Silsupadol, P., Siu, K.-C., Shumway-Cook, A., & Woollacott, M. H. (2006). Training of balance under single-and dual-task conditions in older adults with balance impairment. Physical Therapy, 86 (2), 269-281.
Soveri, A., Antfolk, J., Karlsson, L., Salo, B., & Laine, M. (2017). Working memory training revisited: A multi-level meta-analysis of n-back training studies. Psychonomic Bulletin & Review, 24 (4), 1077-1096.
Strobach, T., Frensch, P., Müller, H., & Schubert, T. (2015). Evidence for the acquisition of dual-task coordination skills in older adults. Acta Psychologica, 160 , 104-116.
Strobach, T., Frensch, P., & Schubert, T. (2008). The temporal stability of skilled dual-task performance. Paper presented at the Cognitive Science 2007. Proceedings of the 8th Annual Conference of the Cognitive Science Society of Germany. Saarbrücken.
Strobach, T., Frensch, P., Soutschek, A., & Schubert, T. (2012). Investigation on the improvement and transfer of dual-task coordination skills. Psychological Research, 76 (6), 794-811.
Strobach, T., & Karbach, J. (2016). Cognitive training . New York, NY: Springer.
Book Google Scholar
Strobach, T., Liepelt, R., Pashler, H., Frensch, P., & Schubert, T. (2013). Effects of extensive dual-task practice on processing stages in simultaneous choice tasks. Attention, Perception, & Psychophysics, 75 (5), 900-920.
Strobach, T., Salminen, T., Karbach, J., & Schubert, T. (2014a). Practice-related optimization and transfer of executive functions: a general review and a specific realization of their mechanisms in dual tasks. Psychological Research, 78 (6), 836-851.
Strobach, T., & Schubert, T. (2014). Positive consequences of action-video game experience on human cognition: Potential benefits on a societal level. In K. K. Mak (Ed.), Epidemiology of online game addiction. : OMICS Group.
Strobach, T., & Schubert, T. (2017). Mechanisms of practice-related reductions of dual-task interference with simple tasks: data and theory. Advances in Cognitive Psychology, 13 (1), 28-41.
Strobach, T., Schubert, T., Pashler, H., & Rickard, T. (2014b). The specificity of learned parallelism in dual-memory retrieval. Memory & Cognition, 42 (4), 552-569.
Szameitat, A. J., Lepsien, J., von Cramon, D. Y., Sterr, A., & Schubert, T. (2006). Task-order coordination in dual-task performance and the lateral prefrontal cortex: an event-related fMRI study. Psychological Research, 70 (6), 541-552.
Szameitat, A. J., Schubert, T., Müller, K. U., & Von Cramon, D. Y. (2002). Localization of executive functions in dual-task performance with fMRI. Journal of Cognitive Neuroscience, 14 (8), 1184-1199.
Taatgen, N. A. (2013). The nature and transfer of cognitive skills. Psychological Review, 120 (3), 439-471.
Tombu, M., & Jolicœur, P. (2004). Virtually no evidence for virtually perfect time-sharing. Journal of Experimental Psychology: Human Perception and Performance, 30 (5), 795-810.
Townsend, J. T., & Wenger, M. J. (2004). A theory of interactive parallel processing: new capacity measures and predictions for a response time inequality series. Psychological Review, 111 (4), 1003-1035.
Van Selst, M., Ruthruff, E., & Johnston, J. C. (1999). Can practice eliminate the Psychological Refractory Period effect? Journal of Experimental Psychology: Human Perception and Performance, 25 (5), 1268-1283.
Welford, A. T. (1952). The ‘psychological refractory period’and the timing of high-speed performance—a review and a theory. British Journal of Psychology. General Section, 43 (1), 2-19.
Welford, A. T. (1980). The single-channel hypothesis. In A. T. Welford (Ed.), Reaction times (pp. 215-252). London: Academic Press.
Wollesen, B., & Voelcker-Rehage, C. (2013). Training effects on motor–cognitive dual-task performance in older adults. European Review of Aging and Physical Activity, 11:122 .
Woollacott, M., & Shumway-Cook, A. (2002). Attention and the control of posture and gait: a review of an emerging area of research. Gait & Posture, 16 (1), 1-14.
Download references
Acknowledgements
The present paper was supported by a Research Grant of the German Research Foundation (Deutsche Forschungsgemeinschaft, STR 1223/1-1). Correspondence concerning this article should be addressed to Tilo Strobach, Medical School Hamburg, Department of Psychology, Am Kaiserkai 1, 20457 Hamburg, Germany. Emails may be sent to [email protected].
Author information
Authors and affiliations.
Department of Psychology, Medical School Hamburg, Am Kaiserkai 1, 20457, Hamburg, Germany
Tilo Strobach
You can also search for this author in PubMed Google Scholar
Corresponding author
Correspondence to Tilo Strobach .
Additional information
Publisher’s note.
Springer Nature remains neutral with regard to jurisdictional claims in published maps and institutional affiliations.
Rights and permissions
Reprints and permissions
About this article
Strobach, T. The dual-task practice advantage: Empirical evidence and cognitive mechanisms. Psychon Bull Rev 27 , 3–14 (2020). https://doi.org/10.3758/s13423-019-01619-4
Download citation
Published : 31 May 2019
Issue Date : February 2020
DOI : https://doi.org/10.3758/s13423-019-01619-4
Share this article
Anyone you share the following link with will be able to read this content:
Sorry, a shareable link is not currently available for this article.
Provided by the Springer Nature SharedIt content-sharing initiative
- Cognitive mechanisms
- Dual-task skills
- Find a journal
- Publish with us
- Track your research
- Original article
- Open access
- Published: 04 January 2021
The impact of predictability on dual-task performance and implications for resource-sharing accounts
- Laura Broeker ORCID: orcid.org/0000-0001-7552-1060 1 ,
- Harald Ewolds 2 ,
- Rita F. de Oliveira 3 ,
- Stefan Künzell 2 &
- Markus Raab 1 , 3
Cognitive Research: Principles and Implications volume 6 , Article number: 1 ( 2021 ) Cite this article
3807 Accesses
13 Citations
1 Altmetric
Metrics details
The aim of this study was to examine the impact of predictability on dual-task performance by systematically manipulating predictability in either one of two tasks, as well as between tasks. According to capacity-sharing accounts of multitasking, assuming a general pool of resources two tasks can draw upon, predictability should reduce the need for resources and allow more resources to be used by the other task. However, it is currently not well understood what drives resource-allocation policy in dual tasks and which resource allocation policies participants pursue. We used a continuous tracking task together with an audiomotor task and manipulated advance visual information about the tracking path in the first experiment and a sound sequence in the second experiments (2a/b). Results show that performance predominantly improved in the predictable task but not in the unpredictable task, suggesting that participants did not invest more resources into the unpredictable task. One possible explanation was that the re-investment of resources into another task requires some relationship between the tasks. Therefore, in the third experiment, we covaried the two tasks by having sounds 250 ms before turning points in the tracking curve. This enabled participants to improve performance in both tasks, suggesting that resources were shared better between tasks.
According to capacity-sharing accounts, people can flexibly allocate generic processing resources to different competing tasks and stages of processing, which allows concurrent dual tasking (cf. Koch et al. 2018 , regarding a flexibility perspective in multitasking; Meyer and Kieras 1997 ). Limitations in dual-task processing may however occur because the total amount of utilizable resources is limited and may be depleted once different stimulus and response modalities draw on this pool of central attentional resources [Kahneman 1973 ; Posner and Petersen 1990 ; Tombu and Jolicœur 2003 ; but see Wickens ( 2002 , 2008 ), for a modality-specific resources account].
With a limited pool of processing resources, critical aspects to successful dual tasking would thus be the reduction of required resources and an effective resource allocation policy. In this study, we have examined the impact of predictability on dual-task performance and its potential implications for a reduction in resource needs and resource allocation policy. Considering that prediction is a permanently ongoing process of the human perceptual, cognitive and motor system (Bubic et al. 2010 ; de Oliveira et al. 2014 ) and single-task (ST) studies have shown that predictable tasks are processed more efficiently and require fewer attentional resources [as indicated by decreased cortical activity, see Eagleman et al. ( 2009 ], we expected predictability to also have an impact on resource utilization in dual tasks. This view has also been supported by Wahn and König ( 2017 ). They claimed that the degree to which a stimulus in the environment can be predicted influences the allocation of attentional resources, and that one future direction for research is to “investigate the extent to which attentional resource limitations can be circumvented by varying the predictability of the presented stimuli” (p. 91). In continuous tasks, predictability may take the form of visual information about the route ahead enabling participants to plan action required in a few milliseconds (de Oliveira et al. 2014 ). Predictability thus leads to an optimal configuration of the sensory system prior to stimulus onset, which facilitates processing of environmental input (Fougnie et al. 2018 ; Król and Król 2017 ). For this reason, predictability may be of particular importance in dual-task (DT) situations; if predictable tasks require fewer resources, then there should be residual resources available for the other task. For settings where the primary task is predictable, this process has also been termed the trickle-down effect of predictability (Król and Król 2017 ). None of the capacity-sharing accounts do however provide hypotheses about the utilization of residual resources. This might be due to the fact that testing the utilization of resources and residual resources is difficult as the metaphorical construct “resource” is not directly measurable or quantifiable [for a critical evaluation of dual-task theories see Hommel ( 2020 )]. In the literature however, any reduction in costs (either comparing single against dual tasks, or comparing two different dual tasks) and improvements on the dependent variable have been accepted as a proxy for reduced resources (e.g., Fougnie et al. 2018 ; Gopher et al. 1982 ; Wahn and König 2015 ). In addition, it seems advisable to not only look at performance improvements in the primary tasks or dual-task costs (difference between single- and dual-task performance), but to also report performance, and potentially changes, in the secondary task. A closer look at secondary task performance might give an indication of how resources are allocated.
We hypothesize that the human system draws on one general pool of resources [Kahneman 1973 ; Tombu and Jolicœur 2003 ; for an opposing view see Wickens ( 2008 ), as well as the discussion below], and that a predictable primary task frees resources that can be used for a secondary task. This allocation policy should result in improved performance in both tasks. On the contrary, if predictability improves performance in only one (the predictable) task, this would be in line with the previously suggested economic processing mode where humans aim to reduce, not reinvest resources (see also Navon and Gopher 1979 ; Plessow et al. 2012 ). In the literature, there is evidence for both secondary tasks benefiting from a predictable primary task (Cutanda et al. 2015 ; Töllner et al. 2012 ) and for improved performance in the predictable task but not in the secondary task (Corr 2003 ; Ewolds et al. 2017 ). For instance, Cutanda et al. ( 2015 ) showed that when participants concurrently performed an irregular vs. rhythmic auditory response task with an N-back memory task, they responded faster after regular rhythms compared to irregular rhythms, and this was regardless of memory load. By contrast, Ewolds et al. ( 2017 ) used a tracking task which became predictable through learning the track over several days. They showed that performance in a tracking task improved, yet reaction times to the auditory secondary task did not differ between the reactions needed during the learnt versus random tracking segments. Taken together, there is both limited and conflicting empirical evidence regarding the benefits of predictability in the primary task on the secondary task. On the other hand, there is empirical evidence that resource allocation policy can be influenced, and consequently that resources can be unevenly distributed among tasks. For instance, instructing participants to put more emphasis on one vs. the other task (Lehle and Hubner 2009 ; Tsang 2006 ), different perceptions of potential outcome value and the saliency of tasks (Schmidt and Dolis 2009 ; Wickens et al. 2003 , 2015 ; Wickens and Colcombe 2007 ), or distractions during dual-task execution (Strayer and Drews 2007 ) can impact resource allocation policy. However, these studies do not report what implications such an allocation policy might have for the other task which is why further attention should be given to potential drivers of resource reduction and allocation in order to optimize dual-task behavior (Salvucci and Taatgen 2008 ; Tombu and Jolicœur 2003 ).
In this study, we have taken a systematic approach, manipulating predictability in the first task, in the second task, and in both tasks to examine the impact of predictability on dual-task performance and the implications for resource reduction and resource allocation policies.
We used a continuous visuomotor tracking paradigm together with a discrete auditory reaction time task, because it has been shown that this combination of tasks reliably leads to dual-task costs (Ewolds et al. 2017 ; Fougnie et al. 2018 ; Lang et al. 2013 ). More importantly, tracking tasks allow the measure of temporal-spatial variables (i.e., velocity) which give insight into performance changes as soon as another task intervenes. If velocity increases or decreases once participants respond to the auditory task, this indicates that resources are taken away from tracking and we can make inferences about the resource allocation policy. Predictability was manipulated by displaying parts of the tracking path (Experiment 1) and sequencing sounds in the auditory task (Experiment 2a/b). In Experiment 3, we covaried both tasks by playing target sounds 250 ms before the inflection points of the tracking curve and as such the auditory task could be used to predict changes in the tracking task. The covariation created a meaningful relation between tasks, serving as an incentive for participants to reinvest resources into this task or even integrate the tasks into one (Ewolds et al. 2020 ; Schmidtke and Heuer 1997 ).
Experiment 1
Considering that predictability is provided by information in the environment or prior knowledge of a person (Gentsch et al. 2016 ; Körding and Wolpert 2006 ; Wolpert et al. 2003 ), the first experiment manipulated predictability in the tracking environment by providing participants with advance visual information about the tracking path. The continuous task provides a suitable paradigm to examine the hypothesized processes of resource allocation because it allows for flexible scheduling, in contrast to using two discrete tasks. This gives insights into allocation policies at the moment an interfering secondary stimulus occurs. In addition, the information about the tracking path allows feedforward control which can correct positional errors, delays between target and controller, or jerkier trajectories (Engel and Soechting 2000 ; Hill and Raab 2005 ; Lange 2013 ; Scott 2012 ; Weir et al. 1989 ; Wolpert et al. 2011 ). With fewer resources needed in one predictable task there should be residual resources available that can be used for another task. A DT tracking study by Eberts ( 1987 ) already showed that participants receiving visual information on both sides of a moving target improve DT tracking performance, but as no reaction times (RTs) for verbal secondary-task responses were reported, a look into the performance on the secondary task is required in order to make inferences about potential resource allocation.
Participants
In total, 38 participants were recruited on a university campus, via a mailing list or through a participant data bank. Three participants were identified as outliers and were excluded from the analysis, yielding a final sample of 35 participants (22 males and 13 females; aged between 19 and 30 years, M = 21.80 years, SD = 2.56). An a priori G*Power (version 3.1.9.2) analysis revealed a required sample size of 32 participants for a test power of 0.80 (effect size f = 0.25 for 2 groups (ST vs. DT) and 5 conditions (predictability), α = 0.005 corrected for alpha-error accumulation, 1 − β = 0.80, r = 0.5).
Participants in this and the following experiments had self-reported normal or corrected-to-normal vision, normal hearing ability, and no musculoskeletal or neurological disorders. Participants gave written informed consent prior to the experiment and received a small remuneration for taking part. The experiments were approved by the local ethics committee and conformed to the principles of the Declaration of Helsinki 2013.
Participants were seated in a dimly lit room at a viewing distance of 60 cm from a 24-in computer screen (144 Hz, 1920 × 1080 pixel resolution). The tracking software ran on a Windows 10, 64-bit system with a GTX750 graphics card. A spring-loaded joystick was fixed to the table 30 cm from the screen (SpeedLink Dark Tornado, max. sampling rate 60 Hz), and the pedal was fixed to the floor under each participant’s self-reported dominant foot (f-pro USB foot switch, 9 × 5 cm; Fig. 1 ). Participants wore headphones (Sennheiser HD 65TV). The experimenter sat out of view, behind an opaque divider to monitor compliance with the task.
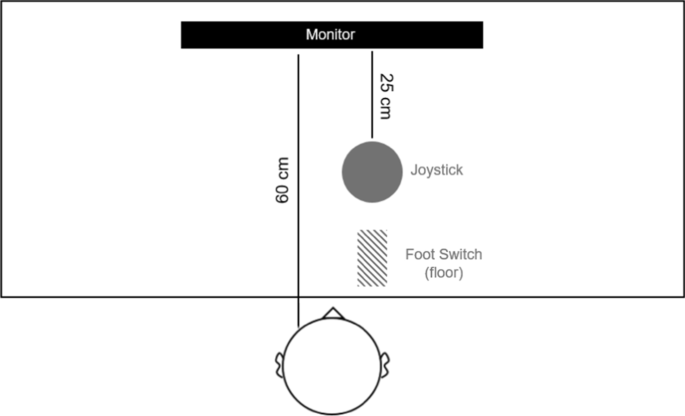
Illustration of the experimental setup
Tasks and display
Visuomotor tracking task.
Participants performed a two-dimensional pursuit-tracking task with a joystick (adapted from Wulf and Schmidt 1997 ) while concurrently reacting to tones by pedal press. Participants operated the joystick with their self-reported dominant hand and controlled a white cursor cross to track a red target square. Unbeknownst to the participants, the cursor cross’s range of motion was limited to the vertical y axis, because its motion on the x -axis was coupled to target speed. This was implemented to prevent participants from moving the cursor straight to the right edge of the screen to cut trials short. Every tracking path was composed of three different segments (adapted from Pew 1974 ), each obeying the formula
with a i and b i being randomly generated numbers ranging from − 5 to 5 and x being a real number in the range [0; 2π]. As different amplitudes have been shown to lead to differences in performance (Magill 1998 ), all randomly generated segments were balanced with regard to mean amplitude beforehand (Wickens 1980 ). This yielded a final set of 41 segments from which the three segments were selected for each trial. Each trial therefore displayed a different path to prevent participants from learning target trajectories (Ewolds et al. 2017 ; Van Roon et al. 2008 ). To avoid the anticipation of peaks (Zhou et al. 2009 ), the red target followed a constant path velocity of 10.5 cm/s, and as a result, trial length varied from 25.6 to 27.9 s depending on the curve’s trajectory (cf., 27 s used in Raab et al. 2013 , and 25 s and 35 s used in de Oliveira et al. 2017 ).
Audiomotor task
The second task was an auditory discrimination task with high-pitched and low-pitched tones occurring randomly along the tracking path (1,086 Hz and 217 Hz, 75-ms duration). Participants reacted to the occurrence of high-pitched tones as fast and as accurately as possible while continuously ignoring the low-pitched tones. Both tones were scaled to the same sound intensity with equal loudness contours (Fletcher and Munson 1933 ). To avoid learning effects, the number of target and distractor sounds per trial varied between 9 and 14 (every 1.9–3.0 s, following Raab et al. 2013 ), but all participants received the same total number of sounds across the whole experiment. The first tone appeared no earlier than 500 ms after the trial had started, and to guarantee sufficient response time, the last tone was presented at least 500 ms before the trial ended. Because average RTs for auditory discrimination in earlier DT studies were 500–950 ms (e.g., Bherer et al. 2005 ), we used a minimum gap between two sounds of 1001 ms, and responses were considered valid only when they were given within 800 ms after the target sound was played.
Manipulation of predictability
The visuomotor tracking task was made predictable by rendering a portion of the tracking path ahead of the target visible (see Fig. 2 ). The visible path was a white line extending 200 ms (to account for visuomotor delay; e.g., Van Rullen and Thorpe 2001 ), 400 ms, 600 ms, or 800 ms ahead of the target square (cf., de Oliveira et al. 2014 ). None of the objects displayed left a trail on the screen. The 0-ms condition represented the unpredictable condition. All five predictability conditions were completed in blocks randomized across participants to avoid training effects (McNeil et al. 2006 ). High-pitched and low-pitched sounds occurred randomly along the tracking path.
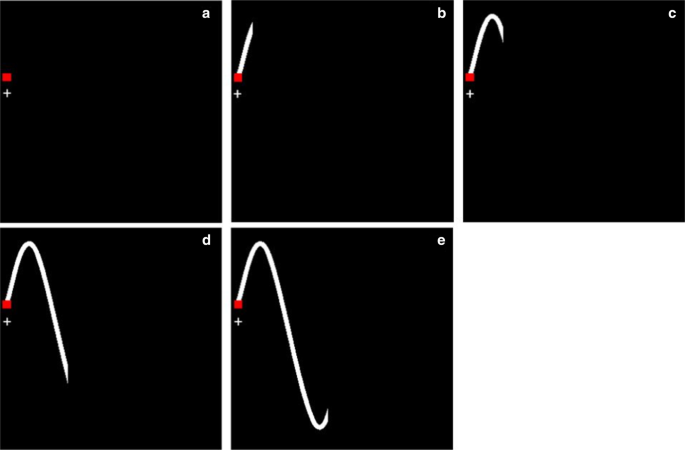
In Experiment 1 participants did not receive any information ( a ; 0 ms) or saw b 200 ms, c 400 ms, d 600 ms, and e 800 ms of the tracking path ahead of the red target square. Participants had to follow the red square and its path as accurately as possible by controlling the white cross
In the familiarization phase, participants completed two ST tracking trials to become familiar with the joystick, then two ST auditory trials to familiarize themselves with the high- and low-pitched sounds, and finally two DT trials to become familiar with the DT setting. They were told that during the experiment these conditions would appear in random blocks. Participants were instructed to follow the target square as closely as possible, to react to target tones as fast and as accurately as possible, and to put equal emphasis on both tasks. To stimulate motivation, a feedback window informing participants about their tracking performance and RTs popped up after every five trials (McDowd 1986 ).
In the experimental phase which took approximately 60 min, participants performed 110 trials in total: 50 ST tracking trials (10 × 5 predictability conditions), 10 ST auditory trials, and 50 DT trials (10 × 5 predictability conditions). After completing the experiment, participants answered a questionnaire about their possible use of a specific DT coping strategy. We also asked which predictability condition they felt was the most helpful to improve DT performance by showing five screen shots of the predictability conditions.
Data analysis
To measure tracking performance, we calculated the root-mean-square error (RMSE), as a measure of mean deviation from the target tracking path (Wulf and Schmidt 1997 ; 1 RMSE ≅ 0.56 cm on screen). Performance on the audiomotor task was evaluated by RTs and errors for target sounds. We also measured participants’ absolute velocities. As outlined above, the target moved at a constant path velocity meaning the tracking cross had the same x coordinates as the target. Participants could control upward and downward movement of the tracking cross on the y -axis only. Thus, the tracking cross’s velocity was composed of participants’ y values and the path’s x values and mirrored participants’ speed changes on the y axis. Velocities make it possible to investigate changes in tracking behavior at different intervals around the discrete auditory event. We computed four velocity intervals, Footnote 1 one prior to and three after target sound onset: 200 ms before sound onset until the moment of sound onset; 200 ms after , which was 75–200 ms after sound onset (given audiomotor delay of 75 ms; Vu and Proctor 2002 ); 400 ms after , which was 200–400 ms after sound onset; and 600 ms after , which was from 400 to 600 ms after sound onset.
Prior to the analyses we checked for outliers in the data. Participants were removed from the datasets when RMSE or RT scores exceeded two standard deviations. The first trial of every condition was treated as a familiarization trial and excluded from the analysis. Pairwise comparisons were made using Bonferroni correction ( α = 0.001), and Greenhouse–Geisser correction was used when sphericity was violated.
We use subscripts to denote the specific conditions of the STs and DTs. For example, we use DT 200 to denote a DT with 200-ms predictability or ST 400 to denote an ST with 400-ms predictability. DT costs (DT cost ) were calculated with the formula [(RMSE ST − RMSE DT )/RMSE ST ] × 100 (Bock 2008 ).
RMSE and RTs were submitted to 2 × 5 repeated-measures analyses of variance (ANOVAs) with the factors Task Type (ST vs. DT) and Predictability (0 ms vs. 200 ms vs. 400 ms vs. 600 ms vs. 800 ms). Velocities were analyzed with a 5 × 4 repeated-measures ANOVA with the factors predictability (0 ms vs. 200 ms vs. 400 ms vs. 600 ms vs. 800 ms) and interval (200 ms before vs. 200 ms after vs. 400 ms after vs. 600 ms after sound onset).
Questionnaire
Of the 35 participants, two thirds stated that they did not pursue any specific DT strategy; the other third prioritized tracking over tone response. When asked about their preferred predictability condition, 28.6% chose 800 ms, 40.0% chose 600 ms, 25.7% chose 400 ms, and 2.85% each chose 200 ms and 0 ms. Some participants verbally reported that they felt distracted by too much visual information (cf., de Oliveira et al. 2014 ). Participants reported that the 600 ms predictability was most helpful although their best performance was at 400 ms.
There was a significant main effect of task type, F (1, 34) = 11.63, p = 0.002, η 2 = 0.255, because participants were better in single-task tracking, and there was a significant effect of predictability, F (4, 136) = 165.62, p < 0.001, η 2 = 0.830, with RMSE being lowest in the 400-ms predictability condition. There was no significant interaction, F (4, 136) = 0.69, p = 0.597, η 2 = 0.020. There were significant differences between 0 ms and all conditions containing visual information, as well as between 200 ms and the remaining visual conditions, in both single- and dual-task trials. There were no significant differences between 600 and 800 ms (Fig. 3 a). Looking further into those conditions that contained visual information (200–800 ms), we found that the relationship between predictability and RMSE was best described by a quadratic function, F (1, 34) = 26.80, p < 0.001.
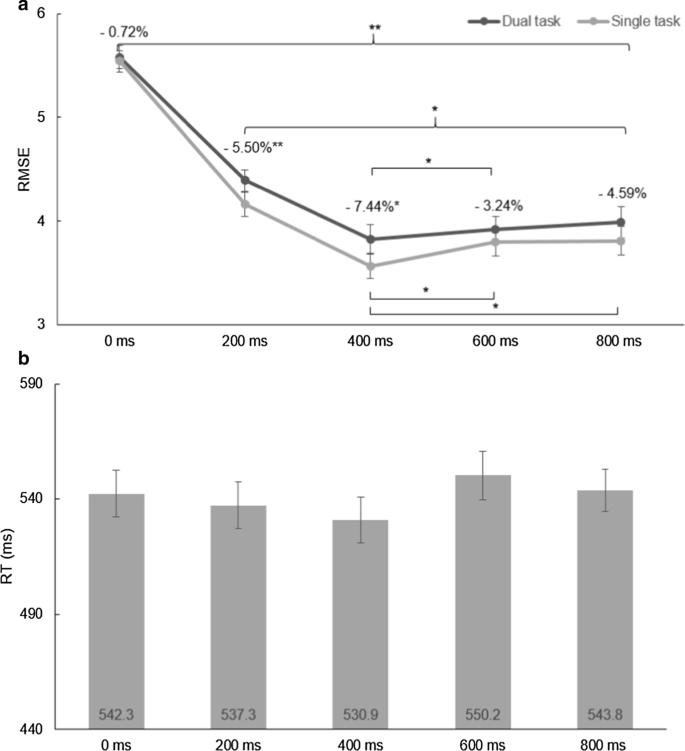
Performance on the 6 predictability conditions. a Tracking performance in Experiment 1 as indicated by root-mean-square error (RMSE). The light gray line represents mean RMSE for dual-task conditions, the dark gray line single-task conditions. Asterisks denote significant differences between single- and dual-task conditions. Dual-task costs, which are added to the graph as percentages, were significantly reduced in the 200-ms and 400-ms conditions. b Reaction times in dual-task conditions. Asterisks denote significant differences between predictability conditions. Conditions varied in predictability (i.e., length of the visible path) from 0 to 800 ms. In both panels, error bars show the standard error
The repeated-measures ANOVA revealed a significant main effect of predictability, F (4, 124) = 7.81, p = 0.036, η 2 = 0.079, because there was a tendency toward faster tracking with less visual information (0 and 200 ms) and slower tracking with more visual information (600 ms and 800 ms). There was a significant main effect of interval, F (23, 93) = 16.71, p < 0.001, η 2 = 0.350, because in all visual predictability conditions participants were fastest in the interval of 400 ms after sound onset (Fig. 4 ). There was also a significant Predictability × Interval interaction, F (12, 372) = 3.19, p < 0.001, η 2 = 0.093, because velocity in the unpredictable condition DT 0 was furthest from target velocity and velocity in the DT 400 condition was closest to target velocity.
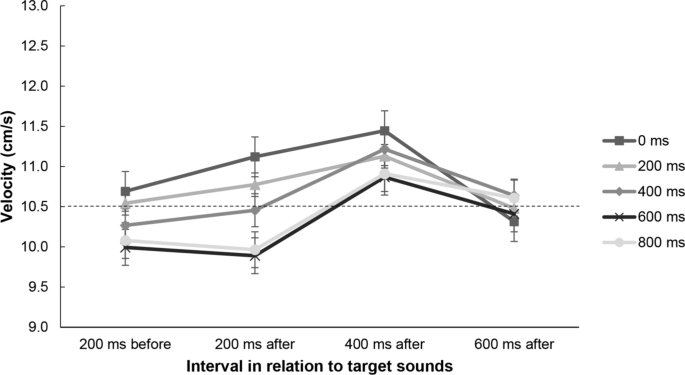
Results of velocity analyses in Experiment 1. The dashed horizontal line represents the constant target velocity (10.5 cm/s). Baseline tracking velocity (i.e., 200 ms before the sound onset) was compared against 200 ms, 400 ms, and 600 ms after the sound onset. Error bars show the standard error. Different symbols represent the different predictability conditions
Audiomotor task RTs
There was no significant effect of predictability on RTs, F (4, 136) = 2.11, p = 0.083, η 2 = 0.058. ST performance for the auditory task was M = 464 ms (SD = 52).
Errors in the audiomotor task
There were two types of response errors in the auditory task: late responses (Err late ) were given after the valid period which was between 800 ms after the target sound and the onset of the next sound, or missing responses (Err miss ) where there was no response between target onset and the following target onset (Fig. 5 ). There was no significant effect of predictability on late responses, F (4, 124) = 1.84, p = 0.126, η 2 = 0.056, or on missing responses, F (4, 124) = 1.90, p = 0.115, η 2 = 0.058. Paired t tests showed significant differences between single- and dual-task error rates (all t (31) > 5.56, all p < 0.001, all d > 0.652).
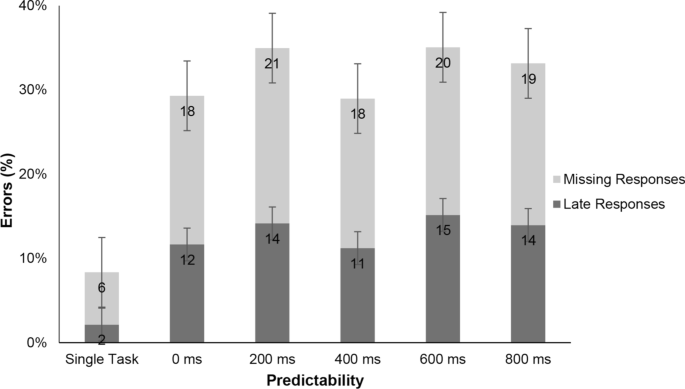
Errors in Experiment 1 were either late responses given later than 800 ms after sound onset (in dark grey) or missing responses that were not given at all (in light grey). Error bars show the standard errors
First, predictability significantly improved visuomotor performance, because dual-task performance improved with visual information. Therefore we conclude that predictability reduces the need for resources. The beneficial effect was most evident for 400 ms, as this was the condition with the lowest RMSE, a more accurate velocity and also lower RT and fewer errors. However, there were no beneficial effects of predictability on secondary task performance, neither on RT nor errors, Footnote 2 which is why we infer that resources were most likely not reinvested. We will discuss the details of these results below.
Regarding the general impact of predictability we conclude that, in line with the basic premise that visual information fosters feedforward control (Weir et al. 1989 ), predictability enabled more accurate movements in the predictable task. As there was no significant improvement of tracking accuracy beyond 400 ms advance information, this amount of information seems sufficient for performance and optimal for feedforward control as already demonstrated by de Oliveira et al. ( 2014 ). It is also in line with research on oculomotor prediction, showing that 500 ms of visual information prior to stimulus occlusion is enough to scale ocular responses (Bennett et al. 2010 ). It is also in line with research on aiming movements, which demonstrated that people who practiced aiming and were provided with 600-ms vision performed equally well when later provided with only 400 ms (Elliott et al. 1995 ). This makes (visual) predictability also different from task difficulty. One could have intuitively suggested that with increasing predictability, the task gets simply easier. However, it has been suggested that the relationship between task difficulty and dual-task performance can be described by a linear relationship (e.g., Isreal et al. 1980 ; McDowd and Craik 1988 ), but our results suggest that visual information may not be unlimitedly beneficial.
Velocity profiles demonstrated that participants across all conditions showed more speed changes approximately 400 ms after onset of the auditory stimulus. This can be interpreted as DT interference, possibly around response selection, considering that RTs were 540 ms on average. This contrasts with interference found in prior tracking studies, where it typically propagates to Task 2 and results in longer RTs. Interference in Task 1 can be the result of limbs’ coupling and thus a neuromuscular effect (neural cross-over effect; Wages et al. 2016 ), an attentional spillover effect (Beilock and Gray 2012 ), or the result of a strategic timing gain to compensate for the reaction to the sound.
Therefore, regarding our aim to make inferences about resource allocation policy, our results are in line with the notion that visual and auditory tracking tasks draw on the same general pool of resources (Fougnie et al. 2018 ), because the tracking task seems to have claimed most of the resources, but the peak in velocity demonstrates that a share of resources was temporarily allocated to the audio task to prepare pedal responses. This share of resources satisfied the minimum requirement of giving a response, yet it seems that not enough residual resources were invested to actually reduce reaction times and improve secondary task performance. According to modality-specific resource accounts (Wickens 2008 ), resources utilized for a visual task should not interfere with demands from an auditory task and thus could not explain the increased tracking velocity. The velocity change was most pronounced in the 0-ms condition, which was the condition without any predictive component and therefore fundamentally different from the other conditions. It seems that the constantly changing environment forced participants to overtake and drop back behind the target more often, which resulted in more overall velocity changes, reflecting the highest need for resources (as also mirrored by no differences between ST and DT performance in RMSE). In contrast, the effect was least pronounced in the 600- and 800-ms conditions, suggesting forward control in response to the upcoming path (Hill and Raab 2005 ) which enabled participants to stay closely behind the target, without the need for constant alignment around the target, and possibly less need for resources.
Another possible explanation for the results is that the increased share of resources to the visually predictable task might be the result of task prioritization. It is plausible that more resources were allocated to the task that was most achievable, which would be in line with increasing error rates for conditions where visual information was present.
Experiment 2a
Experiment 1 showed that participants’ performance improved in the predictable task but not in the secondary, unpredictable task. It seems that most of the resources, drawn from one general pool of resources, were allocated to the predictable task but that residual resources freed by predictability were not reinvested into the secondary task. In Experiment 2, we turned the manipulations around by making the secondary task predictable and leaving the continuous task unpredictable, and examined resource allocation policies for a predictable secondary task.
Prior knowledge , as the second source of predictability (Wolpert and Kawato 1998 ), can be induced via sequences in discrete tasks. Sequences and regularities increase the likelihood of stimulus occurrence and reduce uncertainty about stimulus onset, which enables participants to respond in a timely fashion (Capizzi et al. 2012 ; Nobre et al. 2007 ; Requin et al. 1991 ; Rolke and Hofmann 2007 ). In line with the argument presented above, this should result in enhanced accuracy, considerably reduced RTs, and fewer attentional resources required (de la Rosa et al. 2012 ). Töllner et al. ( 2012 ) explained that knowledge about a stimulus or task leads to a pre-activation of that sensory modality, freeing up general resources and consequently enhancing encoding and leading to faster response selection. If this holds, sequence learning could lead to faster visual processing and shorter motor response execution times in visuomotor tasks (De Jong 1995 ; Sigman and Dehaene 2006 ). In fact, two DT studies (Cutanda et al. 2015 ; de la Rosa et al. 2012 ) demonstrated that regular auditory sequences led to faster reaction times, equally effective in ST and DT conditions and irrespective of high or low load in the working memory task of the DT condition. However, RTs for ST and DT performance of the secondary working memory task were not explicitly contrasted and allocation policies could not be inferred.
For Experiment 2a, we recruited 24 participants. Two participants were excluded from the analyses because testing was terminated due to a technical malfunction, yielding a final sample of 22 participants (10 males and 12 females; aged between 18 and 30 years, M = 22.82 years, SD = 3.20). As Experiment 1 showed stable performance on the tracking task after very few trials, and thus high correlations among trials [DT 0 : Cronbach’s α = 0.927 (mean correlation among trials: r = 0.674), DT 200 : α = 0.935 (r = 0.648), DT 400 : α = 0.959 (r = 0.769), or DT 600 : α = 0.943 (r = 0.669)], the a priori sample-size estimations for Experiment 2 were adapted: α = 0.05, 1 − β = 0.80, r = 0.7 (G*Power 3.1.9.2). This revealed a test power of 0.81 and a required sample size of 22 participants.
The setup was the same as in Experiment 1. We used a 16-bit joystick (Thrustmaster T16000M FCS, max sampling rate 120 Hz).
The task and display were the same as in Experiment 1, but only the unpredictable 0-ms condition was applied.
The secondary task was an auditory discrimination task. In the predictable/sequenced condition, tones were arranged in a sequence with every fourth sound being the high-pitched target sound (see Fig. 6 ) with varying inter-stimulus intervals ranging between 750 and 1,050 ms. In the unpredictable/random condition, high- and low-pitched tones occurred randomly with the same varying inter-stimulus intervals. The number of target sounds per trial varied between 9 and 12 in unpredictable conditions where sounds occurred randomly (every 1.9 to 3.0 s, following Raab et al. 2013 ).
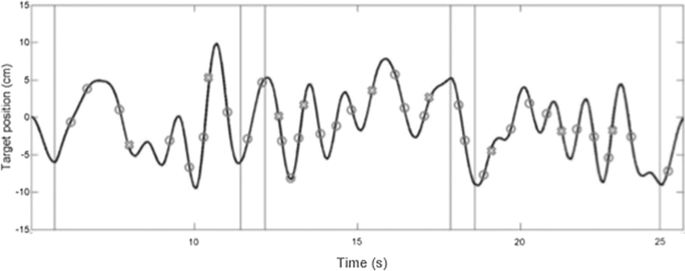
An example of a sequenced dual-task trial in Experiment 2a. A tracking target followed the sinusoidal path, which was invisible to the participants. Circles along the tracking path represent the occurrence of distractor sounds; crosses along the tracking path represent target sounds. All sounds had varying inter-stimulus intervals. The only regularity in the predictable condition was the occurrence of a target sound every fourth sounds
After the familiarization phase, participants took about 30 min to perform 50 trials. Participants began with 10 ST tracking trials, after which they completed four more blocks that were randomized across participants: 10 ST auditory trials with random sounds, 10 ST auditory trials with sequenced sounds, 10 DT trials with random sounds, and 10 DT trials with sequenced sounds.
As in Experiment 1, we calculated the average RMSE and tracking velocities as a measure of tracking performance and RTs plus errors as a measure of performance in the audiomotor task. We used rand to denote trials with randomly occurring sounds and seq to denote trials with sequenced sounds (e.g., DT rand , ST seq ).
The RMSE were compared between ST and DT trials with two paired- t tests (ST vs. DT rand ; ST vs. DT seq ). Further, for DT trials, RMSE was submitted to a one-way repeated-measures ANOVA with factor Sound Order (random vs. sequenced). Velocities were analyzed with a 2 × 4 repeated-measures ANOVA with factors Sound Order (random vs. sequenced) and Interval (200 ms before sound onset vs. 200 ms after onset vs. 400 ms after onset vs. 600 ms after onset). RTs were submitted to a 2 × 2 repeated-measures ANOVA with the factors Sound Order (random vs. sequenced) and Task Type (ST vs. DT).
There was no effect of sound order on RMSE, F (1, 21) = 0.03, p = 0.873, η 2 = 0.001. Pairwise comparisons between ST and DT conditions revealed significant differences both when sounds were random, t (21) = 3.51, p = 0.002, d = 0.749, DT cost = − 5.76%, and when sounds were sequenced, t (21) = 2.84, p = 0.010, d = 0.605, DT cost = − 5.44%.
The repeated-measures ANOVA revealed a main effect of interval, F (3, 63) = 8.34, p < 0.001, η 2 = 0.284, because there was an increase in velocity in the 400-ms after interval. There was also a significant Sound Order × Interval interaction, F (3, 63) = 2.87 , p = 0.043, η 2 = 0.120, because this increase after target sound onset was less pronounced in sequenced compared to random trials (see Fig. 7 , top). There was no main effect of sound order on velocity, F (1, 21) = 0.44, p = 0.516, η 2 = 0.020. In general, participants had higher velocities compared to the target square across all intervals and conditions, which means that the control cursor was ahead of the target square.
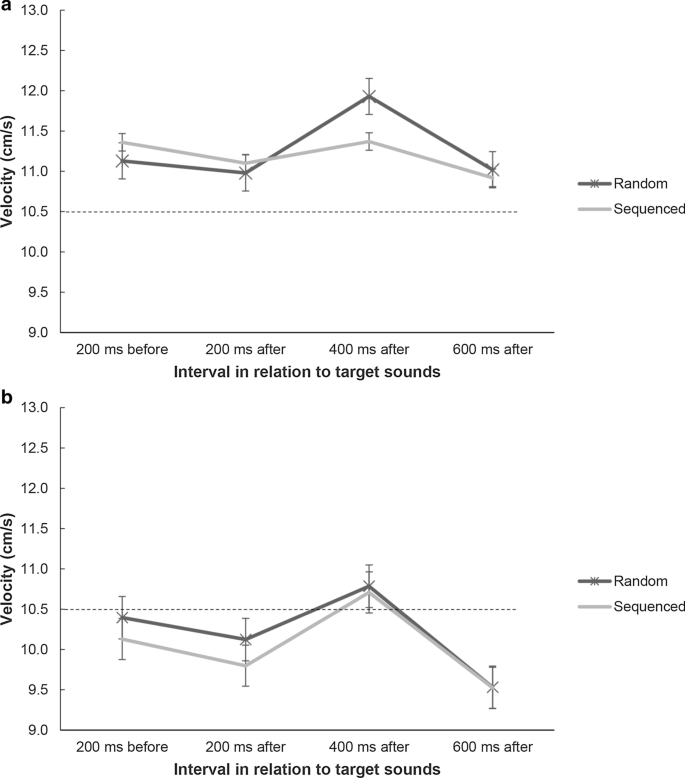
Tracking velocity analyses in Experiments 2a ( a ) and 2b ( b ). Baseline tracking velocity (200 ms before the occurrence of a target sound) was compared against 200 ms, 400 ms, and 600 ms after the sound onset. The dashed horizontal line represents the constant target velocity (10.5 cm/s). Error bars show standard errors
The repeated-measures ANOVA revealed a significant main effect of sound order, F (1, 21) = 136.29, p < 0.001, η 2 = 0.866, because participants were faster in sequenced compared to random trials. There was also a significant effect of task type, F (1, 21) = 28.01, p < 0.001, η 2 = 0.571, because participants were faster in ST conditions compared to DT conditions. There was no significant Sound Order × Task Type interaction, F (1, 21) = 3.81, p = 0.065, η 2 = 0.153 (see Fig. 8 ). Mean RTs are shown in Table 1 .
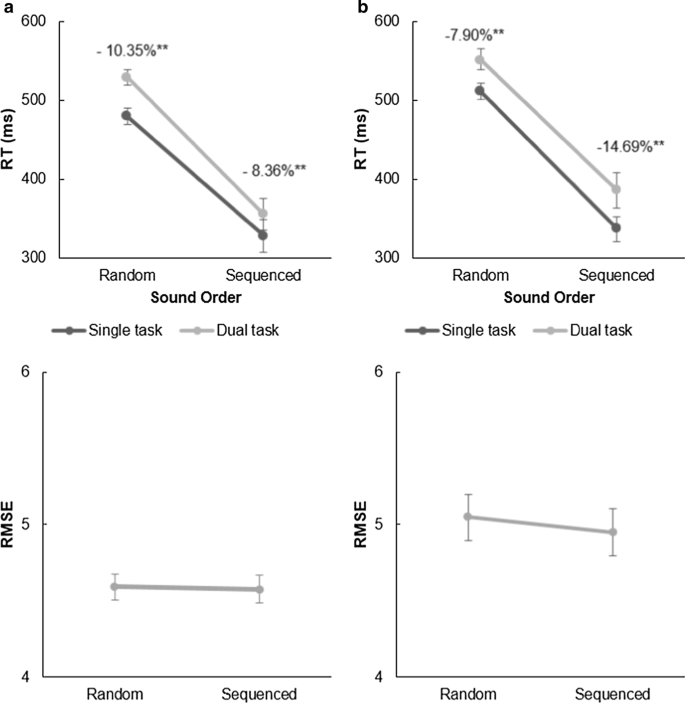
Reaction time (RT) and root-mean-square error (RMSE) analyses in Experiment 2a ( a ) and 2b ( b ). Light gray lines depict dual-task conditions, dark gray lines depict single-task conditions. DT costs are the differences between single- and dual-task conditions, presented as percentages; asterisks denote significant DT costs, ** p < .001, * p < .005. Error bars show standard errors
There were two types of response errors in the auditory task: false responses when participants pressed the pedal in reaction to distractor sounds, and missing responses (Err miss ) which did not occur between two consecutive target onsets (Fig. 9 ). Importantly, responses which were given before sound onset (“premature”) were also counted as missing responses.
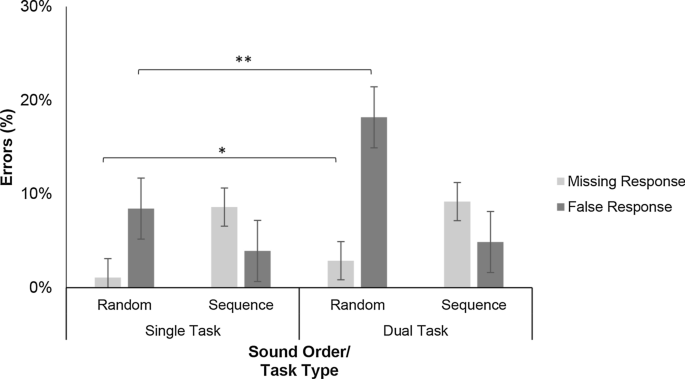
Errors in Experiment 2a were either false responses to distractor sounds or missing responses. There were only significant differences between single- and dual-task conditions when sounds were random, with Err _miss , t (21) = 2.96, p = .007, d = .711 and Err _false , t (21) = 3.83 , p < .001, d = 967, respectively
There was no significant effect of sound order on false responses, F (1, 21) = 2.13, p = 0.160, η 2 = 0.092. There was a main effect of task type, F (1, 21) = 7.13, p = 0.014, η 2 = 0.253, because participants performed better in single-task trials. There was no significant Sound Order × Task Type interaction, F (1, 21) = 2.83, p = 0.105, η 2 = 0.120.
There was a significant effect of sound order on missing responses, F (1, 21) = 6.00, p = 0.023, η 2 = 0.222, because participants missed fewer responses in the random conditions. There was no effect of task type on missing responses, F (1, 21) = 3.24, p = 0.086, η 2 = 0.134, and no significant Sound Order × Task Type interaction, F (1, 21) = 3.98, p = 0.059, η 2 = 0.159.
Contrary to our expectations, participants failed to react to target sounds and erroneously reacted to distractor sounds more often in sequenced conditions than random conditions (see Fig. 9 ). As only responses after sound onset were taken into consideration, the high number of missing responses might be explained by premature responses given before sound onset. Therefore, this result is somewhat inconclusive.
When comparing the difference between single- and dual-task conditions, as expected, participants more frequently failed to react to target sounds and falsely reacted to distractor sounds in dual-task conditions compared to single-task conditions.
First, like in Experiment 1, predictability significantly improved dual-task performance, suggesting that predictability reduced the need for resources. Inferring whether performance improvements only emerge in the predictable task and thus a conclusion about whether or not residual resources were reinvested is contingent on the dependent variables as we outline below.
In general, when comparing ST and DT performance in the two conditions, we found typical performance impairment for DT conditions, which was less pronounced for sequenced trials. Sequenced trials lowered RTs to target sounds, lowered DT cost , and possibly also reduced the need for resources. This effect occurred even though sequences had varying inter-stimulus intervals making the exact timing of sound onset unpredictable (in contrast to the rhythms used by Capizzi et al. 2012 ; Cutanda et al. 2015 ; Halvorson et al. 2013 ). However, the benefit of sequences was not apparent in the tracking’s RMSE. So while RMSE would not support the hypothesis that residual resources from one general pool were reinvested, velocities show a different pattern. Like in Experiment 1, there were more changes in velocity 400 ms after sound onset—interpretable as interference—but this was not significant for sequenced trials (no significant difference between the intervals 200 ms after and 400 ms after). The velocity analysis would thus suggest that predictability in the auditory task freed enough resources to maintain motor control and accuracy in tracking while preparing pedal responses, defeating, or diminishing interference. Considering that tracking is a continuous task, velocities allow a more fine-grained analysis of performance and interference compared to the exclusively spatial measure RMSE.
Experiment 2b
Experiment 2a showed that predictability reduced the need for resources, which have been possibly redistributed to tracking in order to maintain motor control during response preparation. Experiment 2b was designed to challenge this finding by increasing cognitive and motor load in the auditory task. To do so, we transformed the go/no-go task into a choice RT task. Participants were no longer required to ignore the low-pitched tones but had to react to both tones with a double pedal, using both feet.
For Study 2b, we recruited 24 participants. Four participants dropped out during the experiment, leaving a final sample of 20 participants (15 males and 5 females; aged between 18 and 36 years, M = 24.80 years, SD = 4.32).
The setup was the same as in Experiment 2a, except for the foot pedal, which was now a double-foot switch (Scythe USB 2FS-2), fixed centrally under the table.
Task and display
The tracking task and display were identical to those in Experiment 2a.
The audio task was a choice task, and participants reacted to both tones via the double pedal. They responded to low-pitched (distractor) sounds by pressing the left pedal with the left foot and to high-pitched (target) sounds by pressing the right pedal with the right foot. The sound-order conditions (random and sequenced sounds) remained the same as in Experiment 2a.
After the familiarization phase, participants took approximately 30 min to complete 50 trials: 10 ST tracking trials, 20 ST auditory trials (10 × random sounds, 10 × sequenced sounds), and 20 DT trials (10 × random sounds, 10 × sequenced sounds).
RMSE and velocities were calculated as a measure of visuomotor performance, and RTs were calculated as a measure of audiomotor performance. Differences in RMSE between ST and DT trials were analyzed with two paired- t tests (ST vs. DT rand ; ST vs. DT seq ). Further, for DT trials, RMSE was submitted to a one-way repeated-measures ANOVA with factor Sound Order (random vs. sequenced). Velocities were analyzed with a 2 × 4 repeated-measures ANOVA with factors Sound Order (random vs. sequenced) and Interval (200 ms before sound onset vs. 200 ms after onset vs. 400 ms after onset vs. 600 ms after onset). RTs were submitted to a 2 × 2 ANOVA with factors Sound Order (random vs. sequenced) and Task Type (ST vs. DT). Errors were subject to a 2 × 2 × 2 ANOVA with factors Sound Order (random vs. sequenced), Task Type (ST vs. DT), and Sound Type (target vs. distractor sound).
There was no effect of sound order on RMSE, F (1, 19) = 2.12, p = 0.162, η 2 = 0.100. Pairwise comparisons between ST and DT trials revealed deteriorated tracking performance in DTs, both when sounds were random, t (19) = 5.91, p < 0.001, d = 1.322 (ST: M = 4.43, SD = 0.35; DT rand : M = 5.05, SD = 0.68), and when sounds were sequenced, t (19) = 4.61, p < 0.001, d = 0.1031 (ST: M = 4.43, SD = 0.35; DT seq : M = 4.95, SD = 0.68).
The repeated-measures ANOVA revealed a main effect of interval, F (3, 51) = 9.57, p < 0.001, η 2 = 0.360 (see Fig. 7 , bottom), but there was no main effect of sound order on velocity, F (1, 17) = 0.92, p = 0.350, η 2 = 0.051, and no significant interaction, F (3, 51) = 0.50 , p = 0.686, η 2 = 0.028.
The repeated-measures ANOVA revealed a significant main effect of sound order, F (1, 19) = 86.33, p < 0.001, η 2 = 0.820, because participants were faster in sequenced compared to random trials. There was a significant main effect of task type, F (1, 19) = 15.84, p < 0.001, η 2 = 0.455, because participants were generally faster in ST conditions than DT conditions, as in Experiment 2a. However, there was no significant Sound Order × Task Type interaction, F (1, 19) = 0.16, p = 0.690, η 2 = 0.009 (Fig. 7 ). Mean RTs in the double-pedal experiment are presented in Table 2 .
There were two types of response errors in the auditory task: false responses when participants used the wrong pedal, i.e., left instead of right pedal for target sounds and right instead of left pedal for distractor sounds; and missing responses (Err miss ) for target and distractor sounds. As in Experiment 2a, responses which were given before sound onset (“premature”) were also counted as missing responses. There was large percentage of false responses to target sounds, most likely due to a large amount of premature responses (as they were counted in the interval after distractor sounds). We therefore decided not to consider errors further, but details can be seen in appendix.
In Experiment 2b we showed that predictability had a positive impact on audiomotor performance, even though this effect was less pronounced than in Experiment 2a. Whereas in Experiment 2a the impact of predictability on tracking performance seemed to be dependent on the variable examined, results of Experiment 2b were more clear-cut. There was neither a positive impact on RMSE nor a less pronounced velocity increase for sequenced conditions. We conclude that auditory predictability was strong enough to buffer load induced by simple reactions (Experiment 2a), but that more complex choice reactions require additional resources that could not be reinvested in tracking (possibly because they include the excitation of different hemispheres and the initiation of motor action in different limbs). Note that Experiment 2b included fewer participants than planned and this may put into question the nonsignificant results obtained; this is a limitation of this study. However, given the effect sizes and significant results obtained in the experiment we believe the sample size was adequate for the statistical analysis done.
In sum, Experiments 1 and 2 showed that predictability reduced the need for resources; visual predictability reduced the need for resources in tracking and auditory predictability reduced the need for resources in audiomotor reactions. As there were no improvements in the unpredictable task, it seems unlikely that residuals were reinvested; however, velocity profiles speak for one general rather than modality-specific pools of resources.
It is possible that participants did not reinvest residuals because the two tasks were unrelated. Naturally, participants invested more resources in the tracking task because of its continuous nature. The auditory task was therefore always disruptive, irrespective of whether it was predictable, and required fewer resources. Hence, there may have been little incentive to invest in a disruptive task. If, however, the distractive task was transformed into a helping task, then this could be an incentive for reinvestment. This could be achieved by having one task predict changes in the other task. Therefore, in Experiment 3 we examined the role of task structure and between-task predictability in resource allocation policies.
Experiment 3
So far, Experiments 1 and 2 showed that predictability positively influences dual-task performance, predominantly through improvements in the predictable task. While this result per se could have questioned a general pool of resources, velocity analyses have shown that the auditory task takes away some of the resources from tracking and thus support the generic resource assumption. Yet our data did not support reinvestment of resources into a secondary task.
Wahn and König ( 2015 , 2017 ) argued that resource allocation can be task-dependent and that while object-based vs. spatial tasks (visual and auditory) partially share resources, two spatial tasks (visual and auditory) fully share resources. If this is true, then adding a spatial component to the auditory discrimination task in our study, should enable resource reinvestment. We therefore placed target sounds 250 ms before inflection points of the curve and hypothesized that this would decrease the need for resources and enable participants to reinvest resources. Similar approaches have been taken by task integration studies that covaried two tasks (e.g., de Oliveira et al. 2017 ). Schmidtke and Heuer ( 1997 ) showed for instance that sequences could be more easily implemented when they were temporally correlated with another discrete task. Likewise, de Oliveira et al. ( 2017 ) also positioned target tones 250 ms before inflection points of a tracking path, so that participants could relate the occurrence of a tone to a motor action and found that participants in the covariation group showed significantly better performance in DT than in ST. This effect was pronounced not only in repeating segments of the curve but also in random outer segments, suggesting that covariation can facilitate performance even in otherwise unpredictable environments.
We recruited 22 participants. After we removed one person as an outlier, the final sample consisted of 21 participants (11 males and 10 females; aged between 19 and 35 years, M = 23.90 years, SD = 3.49). Sample size estimations were based on Experiment 2 (i.e., α = 0.05, 1 − β = 0.80, r = 0.7, test power of 0.81 and a required sample size of 22 participants).
The setup of Experiment 3 was the same as in Experiment 1.
The tracking task and display were identical to those in the other experiments, but the tracking path was calculated using a different formula. To guarantee enough distance between sounds and curves, the new paths were stretched out. They were composed of three segments, each obeying the formula:
with a i and b i being randomly generated numbers ranging from − 10 to 10 and x being a real number in the range [0; 2π].
Participants responded to high-pitched sounds by pressing on a pedal. High-pitched sounds always occurred 250 ms before a turning point in the tracking curve (integrated conditions); low-pitched sounds occurred randomly between these events and did not require a response by the participant.
After the familiarization phase (DT familiarization with random sounds), participants took about 35 min to perform 60 trials: 20 ST tracking trials, 20 ST auditory trials, and 20 DT trials (10 × random, 10 × integrated). After completing the experiment, participants answered a questionnaire that contained five questions designed to gradually reveal participants’ knowledge of the manipulation. The primary purpose of this questionnaire was to label participants with “knowledge” vs. “no knowledge,” so that knowledge could be entered as a between-subjects factor (see Data Analysis). We first asked whether they had noticed anything special during the experiment, then whether they felt supported or distracted in some of the DT conditions, and then whether they had detected any regularities. After this, participants were told that high-pitched tones served to indicate changes in tracking and were asked whether they had noticed this. If participants answered yes, the fifth question asked them how the tone indicated changes.
For Experiment 3 we use DT cov for DT trials where tracking and auditory task covaried (i.e., stimuli could be integrated) and DT rand for random sounds. We compared the RMSE between ST and DT trials with two paired t tests (ST vs. DT rand ; ST vs. DT cov ). Further, for DT trials, RMSE was submitted to a one-way repeated-measures ANOVA with the factor Sound Location (random vs. covariation). Velocities were analyzed with a two-way repeated-measures ANOVA with the factors Sound Location (random vs. covariation) and Interval (200 ms before onset vs. 200 ms after onset vs. 400 ms after onset vs. 600 ms after onset). RTs were submitted to a 2 × 2 ANOVA with the factors Sound Location (random vs. covariation) and Task Type (ST vs. DT). Knowledge about the task integration was entered into the analysis as a between-subjects factor.
Participants were classified as having knowledge about the manipulation when they were able to correctly describe the task integration manipulation in the fifth question. In total, 10 participants (47.62%) were able to verbalize the positioning of sounds in the questionnaire after finishing the experiment.
Visuomotor tracking task.
There was a significant main effect of sound location on RMSE, F (1, 20) = 5.46, p = 0.030, η 2 = 0.214, because participants showed better tracking performance when sounds were indicative of turns in the tracking task (DT cov : M = 3.93, SD = 0.54; DT rand : M = 4.10, SD = 0.47; ST rand : M = 3.95, SD = 0.49; Fig. 10 ). Participants who acquired knowledge about the manipulation did not show better tracking performance, Sound Location × Knowledge, F (1, 19) = 2.28, p = 0.148, η 2 = 0.085.
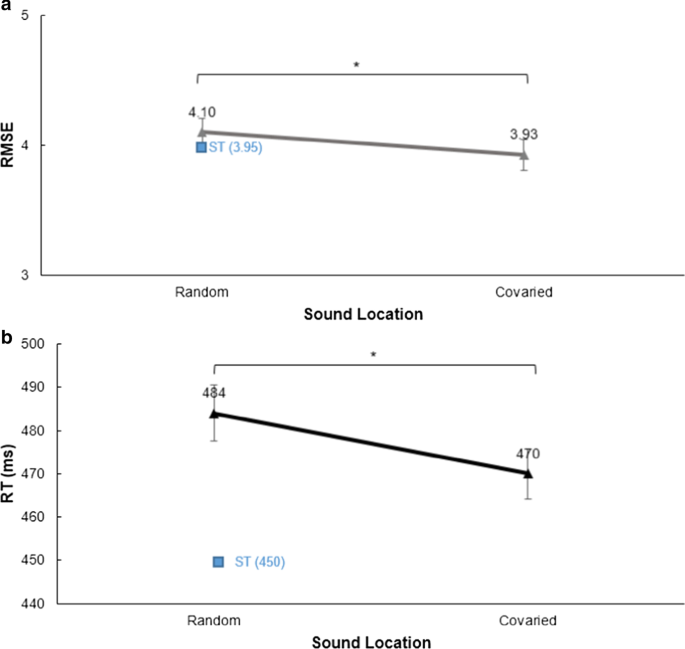
Performance in Experiment 3 by covaried or random sound location. a Results of tracking performance for dual-task conditions in Experiment 3 as indicated by root-mean-square error (RMSE). b Reaction times (RTs) in milliseconds in dual-task conditions. In both panels, single-task (ST) performance is depicted by a single data point represented by a square
For tracking velocities, there were main effects of sound location, F (1, 20) = 46.14, p < 0.001, η 2 = 0.698, and interval , F (3, 60) = 23.74, p < 0.001, η 2 = 0.543, as well as a significant interaction, F (3, 60) = 21.83, p < 0.001, η 2 = 0.522. For random conditions the velocity pattern was similar to that in Experiments 1 and 2, but for integrated conditions there was a very different pattern as participants slowed down after target sound onset (see Fig. 11 ).
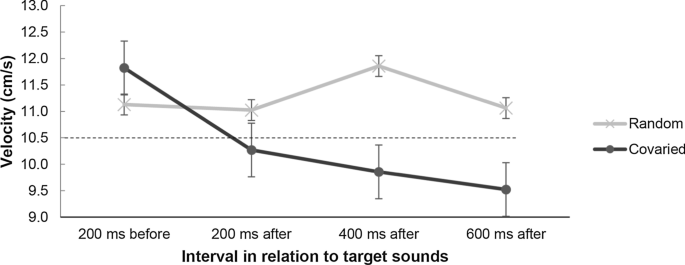
Velocity analyses in Experiment 3. Baseline tracking velocity (200 ms before the occurrence of a target sound) was compared against 200 ms, 400 ms, and 600 ms after the sound onset. The dashed horizontal line represents the constant target velocity (10.5 cm/s). Covaried refers to dual-task trials in which sounds were coupled to the tracking path. Error bars show standard errors
There was a main effect of sound location on RTs, F (1, 20) = 6.59, p = 0.018, η 2 = 0.248 (Fig. 10 ), showing that participants reacted significantly faster when sounds covaried with the tracking path (DT rand : M = 483 ms, SD = 30; DT cov : M = 470 ms, SD = 26; ST rand : M = 449 ms, SD = 34). Knowledge about the location of sounds did not affect RTs, Sound Location × Knowledge, F (1, 19) = 0.32, p = 0.581, η 2 = 0.012.
There were two types of response errors in the auditory task: late responses (Err late ) which were given after the valid period (from 800 ms after the target sound until onset of the next sound), and missing responses (Err miss ) where there was no response between two consecutive target onsets (Fig. 12 ). There was a significant main effect of predictability on late responses, F (1, 20) = 5.19, p = 0.034, η 2 = 0.034, and on missing responses, F (1, 20) = 26.96, p < 0.001, η 2 = 0.100, showing that errors were larger when the tasks covaried. Paired t tests showed significant differences between single- and dual-task error rates (all t (20) > 7.21, all p < 0.001, all d > 1.47), as well as between the random and covaried dual-task conditions (late responses: t (20) = 2.28, p = 0.034, d = 0.497; missing responses: t (20) = 5.19, p < 0.001, d = 1.132).
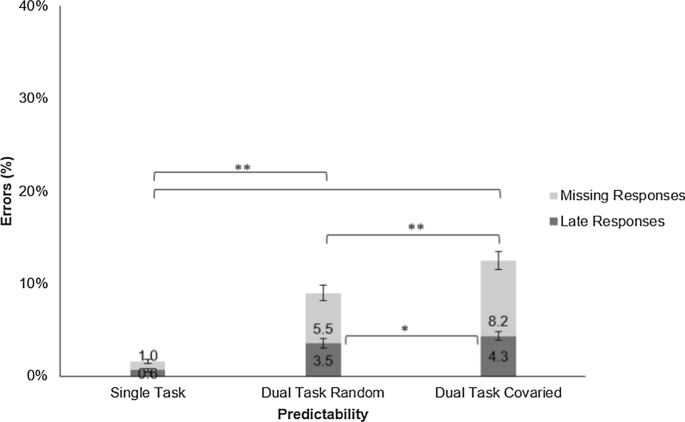
Errors in Experiment 3 were either late responses given later than 800 ms after sound onset (in dark grey) or missing responses that were not given at all (in light grey). Error bars show standard errors
In Experiment 3 we found a beneficial effect of predictability on performance in both tasks, which is in contrast with Experiments 1 and 2. Participants had faster reaction times and also fewer tracking errors. Further, they decreased velocity after sound onset, showing that they probably learned to drop back behind the target and prepare motor responses as soon as the sound had announced upcoming changes in the tracking curve. It is conceivable that the target sounds and their clear spatial location thus served as a warning signal, which in turn eased resource allocation. As the sound was no longer intrusive but helpful, resources could be more easily allocated and shared between tasks, which is why we conclude that covariation between tasks improves DT performance by fostering resource reinvestment. Future research could examine possible mechanisms underlying this effect. As suggested by Künzell et al. ( 2018 ) and Koch et al. ( 2018 ), covariation and a shared higher-level goal can prompt participants to treat two tasks as one integrated task (see also Schmidtke and Heuer 1997 ). One such (implicit) goal or action might have been “turn after pressing” rather than the separate goals of “pedal press” and “track the cursor,” but further measures are needed to test such conceptualization mechanisms. Whether the conceptualization is implicit or explicit does not seem to matter, given that the one half of our subjects which was able to verbalize the position of the sound, performed as well as those without explicit knowledge.
General discussion
The purpose of our experiments was to examine the impact of predictability on dual-task performance and gain insight into resource allocation policies. Experiments 1 and 2, which manipulated predictability in either the first or the second task, showed that performance improved in the predictable task, but that residual resources were not reinvested in the other task. This is in line with economical processing accounts (Navon and Gopher 1979 ). In contrast, Experiment 3 covaried two tasks by including an auditory element in the tracking task (and conversely, a spatial element in the auditory task). The results show clear performance improvements in both tasks and thus possibly better resource sharing and reinvestment across tasks. We therefore conclude that predictability helps to circumvent attentional resource limitations (cf., Wahn and König 2017 , p. 91) and that the extent to which resources can be shared among tasks depends on the tasks and their characteristics [see also the claim by Tombu and Jolicœur ( 2003 , p. 4), that “determining exactly which task characteristics affect capacity allocation is an empirical issue that will need to be resolved”].
Overall, our results contribute to the ongoing debate about whether limited resources are specific to modalities. Our findings lend support to the theory of general resources rather than modality-specific resources. It is possible that predictability freed up modality-specific resources that could not be reinvested into the other-modality task. However both the velocity profiles in all Experiments and the results of Experiment 3 (an auditory cue aiding visuomotor performance) demonstrate that the visual tracking task and the auditory RT seem to draw on common central attentional resources. Because velocity data demonstrate that participants are able to continue tracking while responding to sounds [i.e., called hesitations in Klapp et al. ( 1987 ) and Tsang and Chan ( 2015 )], this strengthens the basic premise that parallel processing and execution is possible. However, dual-task costs showed a small impact of the secondary task, so it is possible that the tracking task demands constant resources and a certain share is always taken by this task. If we consider the concurrent use of hand and foot as same-modality response, the results further strengthen the hypothesis that interference occurs when tasks draw on the same resources (Meyer and Kieras 1997 ; Wickens 2002 ). Consistent velocity increases around pedal responses suggest interference at response-activation or execution stages, because motor-related resources would have to be taken away from manual tracking. It has been suggested that such cross-talk can be overcome with practice by integrating two tasks (Bratzke et al. 2009 ; Heuer and Schmidtke 1996 ; Swinnen and Wenderoth 2004 ), which would also be substantiated by the findings of Experiment 3.
An alternative explanation for our findings concerns task prioritization. Wickens et al. ( 2015 ) suggested in their strategic task overload management model that some task characteristics such as salience can foster the prioritization of a task. It is possible that participants did not reinvest resources into the other task in Experiments 1 and 2 because predictability prompted a shift in priority toward the predictable task. In a dual-task learning experiment (Broeker et al. 2020a ), participants performed the tracking task with a constant middle segment (random outer segments) for two days. One group was informed about the repeating segment, the other group was supposed to acquire implicit motor knowledge. On day three, visual information (400 ms) was added to the tracking task. Results showed an additive effect of knowledge and visual information, meaning that both sources of predictability independently improved tracking performance, but importantly, reaction times did not improve. Capacity-sharing accounts support the notion that cognitive capacity can be voluntarily allocated and that allocation may be dependent on task priority (Tombu and Jolicœur 2003 ; Wickens 2002 ). This would mean participants strategically allocated resources to predictable tasks because they were most likely to be accomplished. This interpretation is valid for Experiment 3 because predictability referred to both tasks together and could not be disentangled.
Regarding limitations of our study, theorizing should be addressed first. The interpretations of our results are based on a hypothetical basic premise, namely that resources exist and that resource allocation policy can explain dual-task limitations. As Hommel ( 2020 ) recently emphasized, this assumption can neither be falsified nor be replaced by a mechanistic model so far. With this study, however, we did not aim at establishing a mechanism, yet we are aware of the theoretical discourse of the research field. Second, some technical limitations should be mentioned. For example, we interpreted the impact of sequenced tone structures as overall faster RTs, because participants were instructed to press the pedal after hearing the target sound and therefore only responses given after onset were taken into consideration. Even though there was no rhythm and we varied the inter-stimulus intervals, it is possible that participants learned the sequence so well that they gave “anticipatory responses.” Because the tracking software did not capture early responses, any pedal presses ahead of sound onset counted as very late responses to distractor sounds. Hence late responses to distractor sounds in sequenced trials might actually be very early responses to target sounds and thus neither errors nor anticipatory responses could be interpreted with certainty. Future uses of the paradigm should carefully consider three aspects in order to allow more reliable error analyses: varying trial lengths, using different amounts of distractor and target sounds in every trial, and varying inter-stimulus intervals in order to allow for instance d-prime or similar error analyses.
The unique contributions of this study are that it strengthens empirical evidence for the beneficial impact of predictability on performance in general and for the perceptual, cognitive, and motor system’s ability to use covariations in the environment. The implementation of a continuous task and thereby the temporal variable velocity were an important methodological extension to classic tracking/DT studies. Velocities allowed us to examine resource allocation at the moment of interference because they demonstrate changes in tracking behavior during secondary-task processing. This is not possible only with RMSE, which is the standard measure in DT research. Another methodological extension was contrasting ST and DT performance for both tasks instead of only reporting DT costs. This was important to understand resource allocation. Experiment 1 also contributes an innovative redesign of Wulf and Schmidt’s paradigm ( 1997 ). Past research has mainly manipulated the middle segment to examine motor learning and its impact on dual tasking, but the implementation of visual information allowed us to examine tracking behavior with online information in a fully unpredictable task environment.
The study also offers practical implications and may guide practitioners who design work spaces or training interventions. First, the workload humans face at work often involves continuous processing and parallel handling of multiple tasks. Our results suggest that, where possible in working spaces, either one task should be made predictable or the environment should allow for tasks’ covariation in space or time. For instance it is possible that an air traffic controller can more efficiently attend to radar control and flight progress strips together, because those two tasks are related in time. Ideally, warning signals help to prepare responses in the more complex task to coordinate tasks more effectively. Second, results from the continuous tracking task may generalize to more complex tasks like driving. Future applied studies should investigate task integration in driving to test the role of predictability. For example, manipulating the temporal positioning of braking signs to effectively maintain steering control might ultimately improve safety in driving. Discussions on using smart phones, voice control, navigation systems, and new technology in (semi-)autonomous driving make such investigations societally relevant. In a related study (Broeker et al. 2020b ), participants’ tracking accuracy was compared with performance in a driving simulator and showed that visual predictability has an impact on dual-task driving performance. This is a first step toward generalizing the present results to more applied settings. Third, the finding that task integration improves continuous dual tasks could be relevant for clinical settings and training programs. For instance, if practitioners used co-varying dual tasks such as counting while walking rather than independent dual tasks, performance might improve due to reduced demand for resources and additional risks like falling could be avoided. This would be a promising avenue for further applied research.
Availability of data and materials
The datasets used and/or analyzed during the current study are available from the corresponding author upon reasonable request.
In a pilot analysis, we compared 200 ms, 400 ms, and 600 ms before onset. We hypothesized that if participants had a constant baseline velocity, these intervals would not differ from each other, and this was indeed the case. Therefore, we used only 200 ms before as the baseline velocity before stimulus onset.
This effect is robust given that there was also no effect of predictability on RTs in a replication study with another 28 participants, F (2, 54) = 2.20, p = .120, η 2 = .075. In this replication study, only dual-task trials were tested and no dual-task costs were examined, which is why the study is not presented in full here.
Beilock, S. L., & Gray, R. (2012). From attentional control to attentional spillover: A skill-level investigation of attention, movement, and performance outcomes. Human Movement Science, 31 (6), 1473–1499. https://doi.org/10.1016/j.humov.2012.02.014 .
Article PubMed Google Scholar
Bennett, S. J., De Xivry, J. J. O., Lefèvre, P., & Barnes, G. R. (2010). Oculomotor prediction of accelerative target motion during occlusion: Long-term and short-term effects. Experimental Brain Research, 204 (4), 493–504. https://doi.org/10.1007/s00221-010-2313-4 .
Bherer, L., Kramer, A. F., Peterson, M. S., Colcombe, S., Erickson, K., & Becic, E. (2005). Training effects on dual-task performance: Are there age-related differences in plasticity of attentional control? Psychology and Aging, 20 (4), 695–709. https://doi.org/10.1037/0882-7974.20.4.695 .
Bock, O. (2008). Dual-task costs while walking increase in old age for some, but not for other tasks: An experimental study of healthy young and elderly persons. Journal of Neuroengineering and Rehabilitation, 5, 27. https://doi.org/10.1186/1743-0003-5-27 .
Article PubMed PubMed Central Google Scholar
Bratzke, D., Rolke, B., & Ulrich, R. (2009). The source of execution-related dual-task interference: Motor bottleneck or response monitoring? Journal of Experimental Psychology: Human Perception and Performance, 35 (5), 1413–1426. https://doi.org/10.1037/a0015874 .
Broeker, L., Ewolds, H. E., de Oliveira, R. F., Künzell, S., & Raab, M. (2020). Additive effects of prior knowledge and predictive visual information in improving continuous tracking performance. Journal of Cognition . https://doi.org/10.5334/joc.130 .
Broeker, L., Haeger, M., Bock, O., Kretschmann, B., Ewolds, H., Künzell, S., & Raab, M. (2020). How visual information influences dual-task driving and tracking. Experimental Brain Research, 238 (3), 675–687. https://doi.org/10.1007/s00221-020-05744-8 .
Bubic, A., Von Cramon, D. Y., & Schubotz, R. I. (2010). Prediction, cognition and the brain. Frontiers in Human Neuroscience . https://doi.org/10.3389/fnhum.2010.00025 .
Capizzi, M., Sanabria, D., & Correa, Á. (2012). Dissociating controlled from automatic processing in temporal preparation. Cognition, 123 (2), 293–302. https://doi.org/10.1016/j.cognition.2012.02.005 .
Corr, P. J. (2003). Personality and dual-task processing: Disruption of procedural learning by declarative processing. Personality and Individual Differences, 34 (7), 1245–1269. https://doi.org/10.1016/S0191-8869(02)00112-5 .
Article Google Scholar
Cutanda, D., Correa, Á., & Sanabria, D. (2015). Auditory temporal preparation induced by rhythmic cues during concurrent auditory working memory tasks. Journal of Experimental Psychology: Human Perception and Performance, 41 (3), 790–797. https://doi.org/10.1037/a0039167 .
De Jong, R. (1995). The role of preparation in overlapping-task performance. The Quarterly Journal of Experimental Psychology. A, Human Experimental Psychology, 48 (1), 2–25. https://doi.org/10.1080/14640749508401372 .
de la Rosa, M. D., Sanabria, D., Capizzi, M., & Correa, A. (2012). Temporal preparation driven by rhythms is resistant to working memory interference. Frontiers in Psychology, 3 (AUG), 1–9. https://doi.org/10.3389/fpsyg.2012.00308 .
de Oliveira, R. F., Billington, J., & Wann, J. P. (2014). Optimal use of visual information in adolescents and young adults with developmental coordination disorder. Experimental Brain Research, 232 (9), 2989–2995. https://doi.org/10.1007/s00221-014-3983-0 .
de Oliveira, R. F., Raab, M., Hegele, M., & Schorer, J. (2017). Task integration facilitates multitasking. Frontiers in Psychology, 8, 398. https://doi.org/10.3389/fpsyg.2017.00398 .
Eagleman, D., Pariyadath, V., & Churchill, S. J. (2009). Predictability engenders more efficient neural responses. Nature Precedings . https://doi.org/10.1038/npre.2009.2847.1 .
Eberts, R. E. (1987). Internal models, tracking strategies, and dual-task performance. Human Factors, 29 (4), 407–419.
Elliott, D., Chua, R., Pollock, B. J., & Lyons, J. (1995). Optimizing the use of vision in manual aiming: The role of practice. The Quarterly Journal of Experimental Psychology Section A, 48 (1), 72–83. https://doi.org/10.1080/14640749508401376 .
Engel, K. C., & Soechting, J. F. (2000). Manual tracking in two dimensions. Journal of Neurophysiology, 83 (6), 3483–3496. https://doi.org/10.1152/jn.2000.83.6.3483 .
Ewolds, H., Broeker, L., de Oliveira, R. F., Raab, M., & Künzell, S. (2020). No impact of instructions and feedback on task integration in motor learning. Memory & Cognition . https://doi.org/10.3758/s13421-020-01094-6 .
Ewolds, H. E., Bröker, L., de Oliveira, R. F., Raab, M., & Künzell, S. (2017). Implicit and explicit knowledge both improve dual task performance in a continuous pursuit tracking task. Frontiers in Psychology . https://doi.org/10.3389/fpsyg.2017.02241 .
Fletcher, H., & Munson, W. A. (1933). Loudness, its definition, measurement and calculation. The Journal of the Acoustical Society of America, 5 (2), 82–108. https://doi.org/10.1121/1.1915637 .
Fougnie, D., Cockhren, J., & Marois, R. (2018). A common source of attention for auditory and visual tracking. Attention, Perception, & Psychophysics . https://doi.org/10.3758/s13414-018-1524-9 .
Gentsch, A., Weber, A., Synofzik, M., Vosgerau, G., & Schütz-Bosbach, S. (2016). Towards a common framework of grounded action cognition: Relating motor control, perception and cognition. Cognition, 146, 81–89. https://doi.org/10.1016/j.cognition.2015.09.010 .
Gopher, D., Brickner, M., & Navon, D. (1982). Different difficulty manipulations interact differently with task emphasis: Evidence for multiple resources. Journal of Experimental Psychology: Human Perception and Performance, 8 (1), 146–157. https://doi.org/10.1037/0096-1523.8.1.146 .
Halvorson, K. M., Wagschal, T. T., & Hazeltine, E. (2013). Conceptualization of task boundaries preserves implicit sequence learning under dual-task conditions. Psychonomic Bulletin & Review, 20 (5), 1005–1010. https://doi.org/10.3758/s13423-013-0409-0 .
Heuer, H., & Schmidtke, V. (1996). Secondary-task effects on sequence learning. Psychological Research , 59 , 119–133. https://doi.org/10.1007/BF01792433 .
Hill, H., & Raab, M. (2005). Analyzing a complex visuomotor tracking task with brain-electrical event related potentials. Human Movement Science, 24 (1), 1–30. https://doi.org/10.1016/j.humov.2004.11.002 .
Hommel, B. (2020). Dual-Task Performance: Theoretical analysis and an event-coding account. Journal of Cognition, 3 (1), 1–13. https://doi.org/10.5334/joc.114 .
Isreal, J. B., Chesney, G. L., Wickens, C. D., & Donchin, E. (1980). P300 and tracking difficulty: Evidence for multiple resources in dual-task performance. Psychophysiology, 17 (3), 259–273. https://doi.org/10.1111/j.1469-8986.1980.tb00146.x .
Kahneman, D. (1973). Attention and effort . Upper Saddle River: Prentice-Hall.
Google Scholar
Klapp, S. T., Kelly, P. A., & Netick, A. (1987). Hesitations in continuous tracking induced by a concurrent discrete task. Human Factors, 29 (3), 327–337. https://doi.org/10.1177/001872088702900306 .
Koch, I., Poljac, E., Müller, H., & Kiesel, A. (2018). Cognitive structure, flexibility, and plasticity in human multitasking—an integrative review of dual-task and task-switching research. Psychological Bulletin, 144 (6), 557–583. https://doi.org/10.1037/bul0000144 .
Körding, K. P., & Wolpert, D. M. (2006). Bayesian decision theory in sensorimotor control. Trends in Cognitive Sciences, 10 (7), 319–326. https://doi.org/10.1016/j.tics.2006.05.003 .
Król, E. M., & Król, M. (2017). The trickle-down effect of predictability: Secondary task performance benefits from predictability in the primary task. PLoS ONE, 12 (7), 1–20. https://doi.org/10.1371/journal.pone.0180573 .
Künzell, S., Broeker, L., Dignath, D., Ewolds, H., Raab, M., & Thomaschke, R. (2018). What is a task? An ideomotor perspective. Psychological Research Psychologische Forschung, 82 (1), 4–11. https://doi.org/10.1007/s00426-017-0942-y .
Lang, A., Gapenne, O., Aubert, D., & Ferrel-Chapus, C. (2013). Implicit sequence learning in a continuous pursuit-tracking task. Psychological Research Psychologische Forschung, 77 (5), 517–527. https://doi.org/10.1007/s00426-012-0460-x .
Lange, K. (2013). The ups and downs of temporal orienting: a review of auditory temporal orienting studies and a model associating the heterogeneous findings on the auditory N1 with opposite effects of attention and prediction. Frontiers in Human Neuroscience, 7 (June), 263. https://doi.org/10.3389/fnhum.2013.00263 .
Lehle, C., & Hubner, R. (2009). Strategic capacity sharing between two tasks: Evidence from tasks with the same and with different task sets. Psychological Research Psychologische Forschung, 73 (5), 707–726. https://doi.org/10.1007/s00426-008-0162-6 .
Magill, R. A. (1998). Knowledge is more than we can talk about: Implicit learning in motor skill acquisition. Research Quarterly for Exercise and Sport, 69 (2), 104–110. https://doi.org/10.1080/02701367.1998.10607676 .
McDowd, J. M. (1986). The effects of age and extended practice on divided attention performance. Journal of Gerontology, 41 (6), 764–769. https://doi.org/10.1093/geronj/41.6.764 .
McDowd, J. M., & Craik, F. I. M. (1988). Effects of aging and task difficulty on divided attention performance. Journal of Experimental Psychology: Human Perception and Performance, 14 (2), 267–280. https://doi.org/10.1037/0096-1523.14.2.267 .
McNeil, M. R., Matthews, C. T., Hula, W. D., Doyle, P. J., & Fossett, T. R. D. (2006). Effects of visual-manual tracking under dual-task conditions on auditory language comprehension and story retelling in persons with aphasia. Aphasiology, 20 (2–4), 167–174. https://doi.org/10.1080/02687030500472660 .
Meyer, D. E., & Kieras, D. E. (1997). A computational theory of executive cognitive processes and multiple-task performance: Part I. Basic mechanisms. Psychological Review, 104 (1), 3–65. https://doi.org/10.1037/0033-295X.104.1.3 .
Navon, D., & Gopher, D. (1979). On the economy of the human-processing system. Psychological Review, 86 (3), 214–255.
Nobre, A., Correa, A., & Coull, J. (2007). The hazards of time. Current Opinion in Neurobiology, 17 (4), 465–470. https://doi.org/10.1016/j.conb.2007.07.006 .
Pew, R. W. (1974). Levels of analysis in motor control. Brain Research, 71 (2–3), 393–400. https://doi.org/10.1016/0006-8993(74)90983-4 .
Plessow, F., Schade, S., Kirschbaum, C., & Fischer, R. (2012). Better not to deal with two tasks at the same time when stressed? Acute psychosocial stress reduces task shielding in dual-task performance. Cognitive, Affective, & Behavioral Neuroscience, 12 (3), 557–570. https://doi.org/10.3758/s13415-012-0098-6 .
Posner, M. I., & Petersen, S. E. (1990). The attention system of the human brain. Annual Review of Neuroscience, 13 (1), 25–42. https://doi.org/10.1146/annurev.ne.13.030190.000325 .
Raab, M., de Oliveira, R. F., Schorer, J., & Hegele, M. (2013). Adaptation of motor control strategies to environmental cues in a pursuit-tracking task. Experimental Brain Research, 228 (2), 155–160. https://doi.org/10.1007/s00221-013-3546-9 .
Requin, J., Brener, J., & Ring, C. (1991). Preparation for action. In J. R. Jennings & M. G. H. Coles (Eds.), Handbook of cognitive psychophysiology: Central and autonomic nervous system approaches (pp. 357–448). New York: Wiley.
Rolke, B., & Hofmann, P. (2007). Temporal uncertainty degrades perceptual processing. Psychonomic Bulletin and Review, 14 (3), 522–526. https://doi.org/10.3758/BF03194101 .
Salvucci, D. D., & Taatgen, N. A. (2008). Threaded cognition: An integrated theory of concurrent multitasking. Psychological Review, 115 (1), 101–130. https://doi.org/10.1037/0033-295X.115.1.101 .
Schmidt, A. M., & Dolis, C. M. (2009). Something’s got to give: The effects of dual-goal difficulty, goal progress, and expectancies on resource allocation. Journal of Applied Psychology, 94 (3), 678–691. https://doi.org/10.1037/a0014945 .
Schmidtke, V., & Heuer, H. (1997). Task integration as a factor in secondary-task effects on sequence learning. Psychological Research Psychologische Forschung, 60 (1–2), 53–71. https://doi.org/10.1007/BF00419680 .
Scott, S. H. (2012). The computational and neural basis of voluntary motor control and planning. Trends in Cognitive Sciences, 16 (11), 541–549. https://doi.org/10.1016/j.tics.2012.09.008 .
Sigman, M., & Dehaene, S. (2006). Dynamics of the central bottleneck: Dual-task and task uncertainty. PLoS Biology, 4 (7), 1227–1238. https://doi.org/10.1371/journal.pbio.0040220 .
Strayer, D. L., & Drews, F. A. (2007). Cell-phone-induced driver distraction. Current Directions in Psychological Science, 16 (3), 128–131. https://doi.org/10.1111/j.1467-8721.2007.00489.x .
Swinnen, S. P., & Wenderoth, N. (2004). Two hands, one brain: Cognitive neuroscience of bimanual skill. Trends in Cognitive Sciences, 8 (1), 18–25. https://doi.org/10.1016/j.tics.2003.10.017 .
Töllner, T., Strobach, T., Schubert, T., & Müller, H. J. (2012). The effect of task order predictability in audio-visual dual task performance: Just a central capacity limitation? Frontiers in Integrative Neuroscience, 6 (September), 75. https://doi.org/10.3389/fnint.2012.00075 .
Tombu, M., & Jolicœur, P. (2003). A central capacity sharing model of dual-task performance. Journal of Experimental Psychology: Human Perception and Performance, 29 (1), 3–18. https://doi.org/10.1037/0096-1523.29.1.3 .
Tsang, P. S. (2006). Regarding time-sharing with convergent operations. Acta Psychologica, 121 (2), 137–175. https://doi.org/10.1016/j.actpsy.2005.07.002 .
Tsang, S. N. H., & Chan, A. H. S. (2015). Tracking and discrete dual task performance with different spatial stimulus-response mappings. Ergonomics, 58 (3), 368–382. https://doi.org/10.1080/00140139.2014.978901 .
Van Roon, D., Caeyenberghs, K., Swinnen, S. P., & Smits-Engelsman, B. C. M. (2008). Development of feedforward control in a dynamic manual tracking task. Child Development, 79 (4), 852–865. https://doi.org/10.1111/j.1467-8624.2008.01163.x .
Van Rullen, R., & Thorpe, S. J. (2001). The time course of visual processing: From early perception to decision-making. Journal of Cognitive Neuroscience, 13 (4), 454–461. https://doi.org/10.1162/08989290152001880 .
Vu, K.-P.L., & Proctor, R. W. (2002). The prevalence effect in two-dimensional stimulus-response compatibility is a function of the relative salience of the dimensions. Perception & Psychophysics, 64 (5), 815–828. https://doi.org/10.3758/BF03194748 .
Wages, N. P., Beck, T. W., Ye, X., & Carr, J. C. (2016). Examination of a neural cross-over effect using resting mechanomyographic mean frequency from the vastus lateralis muscle in different resting positions following aerobic exercise. European Journal of Applied Physiology, 116 (5), 919–929. https://doi.org/10.1007/s00421-016-3351-9 .
Wahn, B., & König, P. (2015). Audition and vision share spatial attentional resources, yet attentional load does not disrupt audiovisual integration. Frontiers in Psychology, 6, 1084. https://doi.org/10.3389/fpsyg.2015.01084 .
Wahn, B., & König, P. (2017). Is attentional resource allocation across sensory modalities task-dependent? Advances in Cognitive Psychology, 13 (1), 83–96. https://doi.org/10.5709/acp-0209-2 .
Weir, D. J., Stein, J. F., & Miall, R. C. (1989). Cues and control strategies in visually guided tracking. Journal of Motor Behavior, 21 (3), 185–204. https://doi.org/10.1080/00222895.1989.10735477 .
Wickens, C. D. (1980). The structure of attentional resources. In R. Nickerson (Ed.), Attention and performance VII (pp. 239–257). New Jersey: Lawrence Erlbaum.
Wickens, C. D. (2002). Multiple resources and performance prediction. Theoretical Issues in Ergonomics Science, 3 (2), 159–177. https://doi.org/10.1080/14639220210123806 .
Wickens, C. D. (2008). Multiple resources and mental workload. Human Factors, 50 (3), 449–455. https://doi.org/10.1518/001872008X288394 .
Wickens, C. D., Goh, J., Helleberg, J., Horrey, W. J., & Talleur, D. A. (2003). Attentional models of multitask pilot performance using advanced display technology. Human Factors, 45 (3), 360–380. https://doi.org/10.1518/hfes.45.3.360.27250 .
Wickens, C. D., Gutzwiller, R. S., & Santamaria, A. (2015). Discrete task switching in overload: A meta-analyses and a model. International Journal of Human Computer Studies, 79, 79–84. https://doi.org/10.1016/j.ijhcs.2015.01.002 .
Wickens, & Colcombe, A. . (2007). Dual-task performance consequences of imperfect alerting associated with a cockpit display of traffic information. Human Factors, 49 (5), 839–850. https://doi.org/10.1518/001872007X230217 .
Wolpert, D. M., Diedrichsen, J., & Flanagan, J. R. (2011). Principles of sensorimotor learning. Nature Reviews Neuroscience, 12 (12), 739–751. https://doi.org/10.1038/nrn3112 .
Wolpert, D. M., Doya, K., & Kawato, M. (2003). A unifying computational framework for motor control and social interaction. Philosophical Transactions of the Royal Society B: Biological Sciences, 358 (1431), 593–602. https://doi.org/10.1098/rstb.2002.1238 .
Wolpert, D. M., & Kawato, M. (1998). Multiple paired forward and inverse models for motor control. Neural Networks, 11 (7–8), 1317–1329. https://doi.org/10.1016/S0893-6080(98)00066-5 .
Wulf, G., & Schmidt, R. A. (1997). Variability of practice and implicit motor learning. Journal of Experimental Psychology: Learning, Memory, and Cognition, 23 (4), 987–1006. https://doi.org/10.1037/0278-7393.23.4.987 .
Zhou, X., Cao, X., & Ren, X. (2009). Speed-accuracy tradeoff in trajectory-based tasks with temporal constraint. In Lecture notes in computer science (including subseries lecture notes in artificial intelligence and lecture notes in bioinformatics) , 5726 LNCS(PART 1) (pp. 906–919). https://doi.org/10.1007/978-3-642-03655-2_99
Download references
Acknowledgements
We thank Dietmar Sach for programming the tracking software, Bettina Kretschmann and Ismael Pedraza for help with collecting data and Anita Todd for providing language help in earlier versions.
Open Access funding enabled and organized by Projekt DEAL. This research was supported by a grant within the Priority Program SPP 1772 from the German Research Foundation (Deutsche Forschungsgemeinschaft, DFG), Grant No. RA 940/17-1 and KU 1557/3-1.
Author information
Authors and affiliations.
Institute of Psychology, German Sport University Cologne, Am Sportpark Müngersdorf 6, 50933, Cologne, Germany
Laura Broeker & Markus Raab
Institute of Sports Science, Augsburg University, Universitätsstraße 3, 86135, Augsburg, Germany
Harald Ewolds & Stefan Künzell
School of Applied Sciences, London South Bank University, 103 Borough Road, London, SE1 0AA, UK
Rita F. de Oliveira & Markus Raab
You can also search for this author in PubMed Google Scholar
Contributions
LB made substantial contributions to the conception, design, acquisition, analysis, interpretation, and writing the work. RO made substantial contributions to the conception, design, and draft. HE made substantial contributions to the analysis and creation of the software. SK made substantial contributions to the conception and draft; MR made substantial contributions to the conception, interpretation, and revision. All authors read and approved the final manuscript.
Corresponding author
Correspondence to Laura Broeker .
Ethics declarations
Ethical approval and consent to participate.
All experiments with human participants performed by the authors were approved by the University’s local ethics committee and were performed according to the Declaration of Helsinki 2008.
Consent for publication
Not applicable.
Competing interests
The authors declare that they have no conflict of interest.
Additional information
Publisher's note.
Springer Nature remains neutral with regard to jurisdictional claims in published maps and institutional affiliations.
Rights and permissions
Open Access This article is licensed under a Creative Commons Attribution 4.0 International License, which permits use, sharing, adaptation, distribution and reproduction in any medium or format, as long as you give appropriate credit to the original author(s) and the source, provide a link to the Creative Commons licence, and indicate if changes were made. The images or other third party material in this article are included in the article's Creative Commons licence, unless indicated otherwise in a credit line to the material. If material is not included in the article's Creative Commons licence and your intended use is not permitted by statutory regulation or exceeds the permitted use, you will need to obtain permission directly from the copyright holder. To view a copy of this licence, visit http://creativecommons.org/licenses/by/4.0/ .
Reprints and permissions
About this article
Cite this article.
Broeker, L., Ewolds, H., de Oliveira, R.F. et al. The impact of predictability on dual-task performance and implications for resource-sharing accounts. Cogn. Research 6 , 1 (2021). https://doi.org/10.1186/s41235-020-00267-w
Download citation
Received : 19 September 2019
Accepted : 10 December 2020
Published : 04 January 2021
DOI : https://doi.org/10.1186/s41235-020-00267-w
Share this article
Anyone you share the following link with will be able to read this content:
Sorry, a shareable link is not currently available for this article.
Provided by the Springer Nature SharedIt content-sharing initiative
- Multitasking
- Predictability
- Task integration
Thank you for visiting nature.com. You are using a browser version with limited support for CSS. To obtain the best experience, we recommend you use a more up to date browser (or turn off compatibility mode in Internet Explorer). In the meantime, to ensure continued support, we are displaying the site without styles and JavaScript.
- View all journals
- Explore content
- About the journal
- Publish with us
- Sign up for alerts
- Published: 02 March 2014
Neural mechanisms of dual-task interference and cognitive capacity limitation in the prefrontal cortex
- Kei Watanabe 1 , 2 nAff3 &
- Shintaro Funahashi 2
Nature Neuroscience volume 17 , pages 601–611 ( 2014 ) Cite this article
11k Accesses
133 Citations
18 Altmetric
Metrics details
- Cognitive control
- Working memory
Simultaneous performance of two tasks often leads to performance deficits in the component tasks. This effect, known as dual-task interference, is thought to be a proof of capacity limitation in cognition, and the lateral prefrontal cortex (LPFC) has been highlighted as its putative neural substrate. Here we recorded single-neuron activities in LPFC while monkeys performed dual tasks that required the simultaneous performance of a varying-load spatial attention task and a spatial memory task. We found that the performance of the monkeys exhibited dual-task interference, and prefrontal neuron activities showed a decreased ability to represent task-relevant information to a degree proportional to the increased demand of the concurrent counterpart task. The locus of the interference was shown to originate in the simultaneous, overloaded recruitment of the same LPFC neural population by the two tasks. These results provide direct neurophysiological evidence for, and constraints to, psychological models of dual-task interference and capacity limitation.
This is a preview of subscription content, access via your institution
Access options
Subscribe to this journal
Receive 12 print issues and online access
195,33 € per year
only 16,28 € per issue
Buy this article
- Purchase on Springer Link
- Instant access to full article PDF
Prices may be subject to local taxes which are calculated during checkout
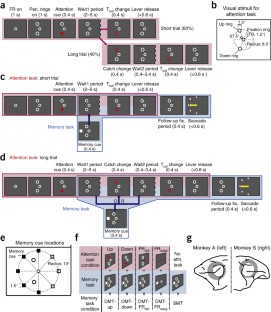
Similar content being viewed by others
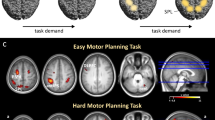
Cross-hemispheric recruitment during action planning with increasing task demand
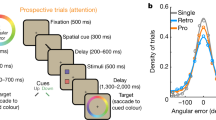
Shared mechanisms underlie the control of working memory and attention
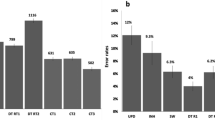
Characterising the unity and diversity of executive functions in a within-subject fMRI study
Duncan, J. The demonstration of capacity limitation. Cognit. Psychol. 12 , 75–96 (1980).
Google Scholar
Redelmeier, D.A. & Tibshirani, R.J. Association between cellular-telephone calls and motor vehicle collisions. N. Engl. J. Med. 336 , 453–458 (1997).
Strayer, D.L. & Johnston, W.A. Driven to distraction: dual-task studies of simulated driving and conversing on a cellular telephone. Psychol. Sci. 12 , 462–466 (2001).
Pashler, H. Dual-task interference in simple tasks: data and theory. Psychol. Bull. 116 , 220–244 (1994).
Marois, R. & Ivanoff, J. Capacity limits of information processing in the brain. Trends Cogn. Sci. 9 , 296–305 (2005).
Baddeley, A. & Hitch, G.J. Working memory. in The Psychology of Learning and Motivation Vol. 8 (ed. Bower, G.H.) 47–89 (Academic Press, New York, 1974).
Telford, C.W. The refractory phase of voluntary and associative responses. J. Exp. Psychol. 14 , 1–36 (1931).
Welford, A. The 'psychological refractory period' and the timing of high speed performance–a review and theory. Br. J. Psychol. Gen. Sect. 43 , 2–19 (1952).
Kahneman, D. Attention and Effort (Prentice Hall, Englewood Cliffs, New Jersey, 1973).
Wickens, C.D. The structure of attentional resources. in Attention and Performance VIII (ed. Nickerson, R.S.) 239–257 (Lawrence Erlbaum, Hillsdale, New Jersey, 1980).
Wickens, C.D. Multiple resources and performance prediction. Theor. Issues Ergon. 3 , 159–177 (2002).
Klingberg, T. Concurrent performance of two working memory tasks: potential mechanisms of interference. Cereb. Cortex 8 , 593–601 (1998).
Adcock, R.A., Constable, R.T., Gore, J.C. & Goldman-Rakic, P.S. Functional neuroanatomy of executive processes involved in dual-task performance. Proc. Natl. Acad. Sci. USA 97 , 3567–3572 (2000).
Bunge, S.A., Klingberg, T., Jacobsen, R.B. & Gabrieli, J.D.E. A resource model of the neural basis of executive working memory. Proc. Natl. Acad. Sci. USA 97 , 3573–3578 (2000).
Passingham, R.E. Attention to action. Phil. Trans. R. Soc. Lond. B 351 , 1473–1479 (1996).
Klingberg, T. & Roland, P.E. Interference between two concurrent tasks is associated with activation of overlapping fields in the cortex. Brain Res. Cogn. Brain Res. 6 , 1–8 (1997).
Baddeley, A., Della Sala, S., Papagno, C. & Spinnler, H. Dual-task performance in dysexecutive and nondysexecutive patients with a frontal lesion. Neuropsychology 11 , 187–194 (1997).
D'Esposito, M. et al. The neural basis of the central executive system of working memory. Nature 378 , 279–281 (1995).
Jiang, Y., Saxe, R. & Kanwisher, N. Functional magnetic resonance imaging provides new constraints on the theories of the psychological refractory period. Psychol. Sci. 15 , 390–396 (2004).
Dux, P.E., Ivanoff, J., Asplund, C.L. & Marois, R. Isolation of a central bottleneck of information processing with time-resolved fMRI. Neuron 52 , 1109–1120 (2006).
Funahashi, S., Bruce, C.J. & Goldman-Rakic, P.S. Dorsolateral prefrontal lesions and oculomotor delayed response performance: evidence for mnemonic “scotomas.”. J. Neurosci. 13 , 1479–1497 (1993).
Rossi, A.F., Bichot, N.P., Desimone, R. & Ungerleider, L.G. Top down attentional deficits in macaques with lesions of lateral prefrontal cortex. J. Neurosci. 27 , 11306–11314 (2007).
Zanto, T.P., Rubens, M.T., Thangavel, A. & Gazzaley, A. Causal role of the prefrontal cortex in top-down modulation of visual processing and working memory. Nat. Neurosci. 14 , 656–661 (2011).
Funahashi, S., Bruce, C.J. & Goldman-Rakic, P.S. Mnemonic coding of visual space in the monkey's dorsolateral prefrontal cortex. J. Neurophysiol. 61 , 331–349 (1989).
Constantinidis, C., Franowicz, M.N. & Goldman-Rakic, P.S. The sensory nature of mnemonic representation in the primate prefrontal cortex. Nat. Neurosci. 4 , 311–316 (2001).
Everling, S., Tinsley, C.J., Gaffan, D. & Duncan, J. Filtering of neural signals by focused attention in the monkey prefrontal cortex. Nat. Neurosci. 5 , 671–676 (2002).
Lebedev, M.A., Messinger, A., Kralik, J.D. & Wise, S.P. Representation of attended versus remembered locations in prefrontal cortex. PLoS Biol. 2 , e365 (2004).
Desimone, R. & Duncan, J. Neural mechanisms of selective visual attention. Annu. Rev. Neurosci. 18 , 193–222 (1995).
Knudsen, E.I. Fundamental components of attention. Annu. Rev. Neurosci. 30 , 57–78 (2007).
Fougnie, D. & Marois, R. Distinct capacity limits for attention and working memory—evidence from attentive tracking and visual working memory. Psychol. Sci. 17 , 526–534 (2006).
Franconeri, S.L., Alvarez, G.A. & Cavanagh, P. Flexible cognitive resources: competitive content maps for attention and memory. Trends Cogn. Sci. 17 , 134–141 (2013).
Rao, S.G., Williams, G.V. & Goldman-Rakic, P.S. Destruction and creation of spatial tuning by disinhibition: GABAA blockade of prefrontal cortical neurons engaged by working memory. J. Neurosci. 20 , 485–494 (2000).
Wallis, J.D., Anderson, K.C. & Miller, E.K. Single neurons in prefrontal cortex encode abstract rules. Nature 411 , 953–956 (2001).
Fuster, J.M. & Alexander, G.E. Neuron activity related to short-term memory. Science 173 , 652–654 (1971).
Kubota, K. & Niki, H. Prefrontal cortical unit activity and delayed alternation performance in monkeys. J. Neurophysiol. 34 , 337–347 (1971).
Goldman-Rakic, P.S. Cellular basis of working memory. Neuron 14 , 477–485 (1995).
Curtis, C.E. & D'Esposito, M. Persistent activity in the prefrontal cortex during working memory. Trends Cogn. Sci. 7 , 415–423 (2003).
Wang, X.J. Synaptic reverberation underlying mnemonic persistent activity. Trends Neurosci. 24 , 455–463 (2001).
Meyers, E.M., Freedman, D.J., Kreiman, G., Miller, E.K. & Poggio, T. Dynamic population coding of category information in inferior temporal and prefrontal cortex. J. Neurophysiol. 100 , 1407–1419 (2008).
Stokes, M.G. et al. Dynamic coding for cognitive control in prefrontal cortex. Neuron 78 , 364–375 (2013).
Zucker, R.S. & Regehr, W.G. Short-term synaptic plasticity. Annu. Rev. Physiol. 64 , 355–405 (2002).
Mongillo, G., Barak, O. & Tsodyks, M. Synaptic theory of working memory. Science 319 , 1543–1546 (2008).
Katsuki, F. & Constantinidis, C. Unique and shared roles of the posterior parietal and dorsolateral prefrontal cortex in cognitive functions. Front. Integr. Neurosci. 6 , 17 (2012).
Suzuki, M. & Gottlieb, J. Distinct neural mechanisms of distractor suppression in the frontal and parietal lobe. Nat. Neurosci. 16 , 98–104 (2013).
Rissman, J., Gazzaley, A. & D'Esposito, M. The effect of non-visual working memory load on top-down modulation of visual processing. Neuropsychologia 47 , 1637–1646 (2009).
Bourke, P.A., Duncan, J. & Nimmo-Smith, I. A general factor involved in dual-task performance decrement. Q. J. Exp. Psychol. A 49A , 525–545 (1996).
Watanabe, K., Igaki, S. & Funahashi, S. Contribution of prefrontal cue-, delay-, and response-period activity to the decision process of saccade direction in a free-choice ODR task. Neural Netw. 19 , 1203–1222 (2006).
Robinson, D.A. A method of measuring eye movement using a scleral search coil in a magnetic field. IEEE Trans. Biomed. Eng. 10 , 137–145 (1963).
Bruce, C.J., Goldberg, M.E., Bushnell, M.C. & Stanton, G.B. Primate frontal eye fields. II. Physiological anatomical correlates of electrically evoked eye movements. J. Neurophysiol. 54 , 714–734 (1985).
Page, E.B. Ordered hypotheses for multiple treatments: a significance test for linear ranks. J. Am. Stat. Assoc. 58 , 216–230 (1963).
Kutner, M.H., Nachtsheim, C.J., Neter, J. & Li, W. Applied Linear Statistical Models (McGraw-Hill, New York, 2005).
Buschman, T.J., Siegel, M., Roy, J.E. & Miller, E.K. Neural substrates of cognitive capacity limitations. Proc. Natl. Acad. Sci. USA 108 , 11252–11255 (2011).
Kline, R.B. Beyond Significance Testing: Reforming Data Analysis Methods in Behavioral Research (American Psychological Association, Washington DC, 2004).
Download references
Acknowledgements
We thank M. Buckley, J. Duncan, M. Kusunoki and M. Stokes for their comments on the manuscript and R. Akaishi, K. Mochizuki and A. Tanaka for their helpful discussions. This work was supported by Grant-in-Aids for Scientific Research (21240024 and 25240021) from the Ministry of Education, Culture, Sports, Science and Technology (MEXT), Japan to S.F. and by Research Fellowships for Young Scientists from the Japan Society for the Promotion of Science to K.W. (20-8015). Two monkeys used for this experiment were supplied from the National Bioresource Project (Japanese Monkeys) supported by MEXT.
Author information
- Kei Watanabe
Present address: Present address: Department of Experimental Psychology, University of Oxford, Oxford, UK.,
Authors and Affiliations
Japan Society for the Promotion of Science (JSPS), Tokyo, Japan
Kokoro Research Center, Kyoto University, Kyoto, Japan
Kei Watanabe & Shintaro Funahashi
You can also search for this author in PubMed Google Scholar
Contributions
K.W. designed the experiment, collected and analyzed the data and wrote the manuscript. S.F. designed the experiment, supervised all aspects of the project and wrote the manuscript.
Corresponding author
Correspondence to Shintaro Funahashi .
Ethics declarations
Competing interests.
The authors declare no competing financial interests.
Integrated supplementary information
Supplementary figure 1 event sequence of example trials..
( a ) Example trials in the standard dual-task. The upper row depicts an example dual-task trial in which the attention task is performed as a short trial. The bottom row shows a trial in which the attention task is performed as a long trial. Note that, in the long trial, there were two possible patterns in the temporal order of catch change and memory cue presentation. ( b ) Same as in panel ( a ), but for the easy dual-task. Note that, in the long trial (bottom row), catch change was scheduled and executed as an ‘empty event’. ( c ) Example trials in the single memory task (SMT). The time course of the task was matched with that in the standard and easy dual-tasks. However, while all attention task events were scheduled, they were executed as ‘empty events’. Trials were automatically initiated by the appearance of FR after an intertrial interval (4.0–7.0 s).
Supplementary Figure 2 Additional evidence supporting the presence of dual-task interference effect in the DMT conditions.
( a ) Mean percent correct rates in the SMT and four DMT conditions plotted separately for the trials with short (< 2.0 s), medium (2.0–4.0 s) and long (> 4.0 s) memory delay periods. In monkey S (left), a two-way mixed-design ANOVA showed significant main effects of both Task condition and Delay length ( P < 10 –4 ), and a nonsignificant interaction effect ( P = 0.27). In monkey A (right), there were significant main effects of both Task condition and Delay length ( P < 10 –4 ), and an interaction effect ( P = 0.02). Asterisks indicate the result of the simple effect ANOVA for the factor Delay length. ( b ) Time course of FB error rates relative to memory cue onset averaged across all sessions for monkeys S (left) and A (right). Inset bar graphs indicate the mean FB error rate during the 1-s period following memory cue onset. Error bars indicate s.e.m. During this period, monkey S showed a significant increase in the FB error rate in DMT-Up and DMT-Down compared with SMT ( post-hoc Steel-Dwass test, P < 10 –4 ; omnibus Kruskal-Wallis test, P < 10 –4 ), indicating that the oculomotor aspect of memory task performance was substantially interfered with by the concurrent attention task. ( c , d ) Trajectories and end points of FB eye movements that occurred during the 1-s period following memory cue onset in monkeys S ( c ) and A ( d ). Three unfilled black circles indicate the ring stimuli for the attention task. Colors are assigned to each memory cue location (square) and each FB eye movement trajectory so that the color of a given FB eye movement trajectory indicates the location of the memory cue presentation that preceded this FB error. End points of FB eye movements are shown as black dots. Numbers shown on each colored square indicate the cumulative number of FB errors across sessions in each memory cue location. The total number of trials (N) in which the memory cue was presented is shown at the bottom of each panel. In the DMT-Up and DMT-Down conditions in monkey S, regardless of memory cue location, FB eye movements after memory cue onset were predominantly directed toward the attention target ring, rather than the memory cue location as observed in SMT. However, importantly, at the time of memory cue onset, the attention cue had been removed from the monitor for 1.6–5.1 s, and the monkeys were simply viewing the still images of three rings. This indicates that around the time of memory cue presentation, information regarding the position of the target ring for the attention task was occupying monkey S’s processing capacity, suggesting that the level of readiness for memory cue encoding was severely disrupted. Monkey A’s FB errors were characterized by short eye movements clustered on the vertical axis, regardless of the memory task conditions. The similarity in FB eye movement trajectories between the SMT and DMT conditions indicates that in all of the four DMT conditions, the preparatory state for memory cue encoding was not disrupted by the concurrent attention task. Thus, we concluded that, for monkey A, dual-task interference on the oculomotor aspect memory task performance was minimal.
Supplementary Figure 3 Behavioral performance in the modified standard dual-task.
( a ) Schematic diagram of the event sequence for non-cued trials of the attention task that were randomly inserted among the Up, Down, FR std conditions (cued trials). A catch change was scheduled but executed as an ‘empty event’ without actual changes in the display items. ( b ) Distribution of the session-by-session percent correct rates in the attention task in the modified standard dual-task (consecutive 25 sessions in each monkey) in monkeys S (left) and A (right). Only data from cued trials are shown. The results in FR easy ( Fig. 2a ) are also shown in the rightmost box plot. The statistical testing procedure and conventions were the same as in Fig. 2a . The results in FR easy ( Fig. 2b ) are also shown in the rightmost box plot. ( c ) Distribution of the session-by-session median RTs in the attention task for monkeys S (left) and A (right). ( d ) Distribution of the session-by-session percent correct rates in the three DMT conditions for monkeys S (left) and A (right). The results in SMT ( Fig. 2c ) are also shown in the rightmost box plot. The dotted line indicates the mean percent correct rates after “corrected-for-guessing” transformation. P -values were adjusted for three multiple comparisons between the SMT and three DMT conditions. ( e ) Comparison of the session-by-session percent correct rates between cued (C) and non-cued (NC) trials in the attention task. In monkey S, the percent correct rates in cued trials were significantly higher than those in non-cued trials (two-way repeated-measures ANOVA: main effect of Cueing, P = 2 × 10 –4 ; Attention condition, P < 10 –4 ; interaction, P < 10 –4 ) (simple main effect of Cueing: Up, P < 10 –4 ; FR std , P = 0.006; both C > NC). ( f ) Comparison of the session-by-session median lever-release RTs between cued (C) and non-cued (NC) trials in the attention task. In both monkeys, the RTs in cued trials were significantly shorter than those in non-cued trials (monkey S: main effect of Cueing, P = 5 × 10 –4 ; Attention condition, P < 10 –4 ; interaction, P = 0.06; monkey A: main effect of Cueing, P < 10 –4 ; Attention condition, P = 0.17; interaction, P = 0.09) (simple main effect of Cueing: P < 0.009 for the Up and Down conditions in monkey S; P < 2×10 –4 for all three conditions in monkey A; all NC > C).
Supplementary Figure 4 Cue-period activity of example neurons.
( a ) Activity of a single neuron (monkey S, right hemi.) recorded in the SMT pre , DMT-Up, DMT-Down, DMT-FR std (standard dual-task), and SMT post conditions. Conventions as in Fig. 4 . ( b ) Same as in panel ( a ), but for a neuron recorded from monkey A (left hemi.) This neuron exhibited significant spatial selectivity in all three DMT conditions in the standard dual-task. However, the strength of cue-period activity in the maximum response location (270°) is significantly attenuated in DMT-Up and DMT-Down compared to that in SMT pre . ( c ) Activity of a single neuron (monkey A, left hemi.) recorded in the SMT pre , DMT-FR easy (easy dual-task), and SMT post conditions. In DMT-FR easy , attenuation of both the magnitude and selectivity of cue-period activity was absent.
Supplementary Figure 5 Delay-period activity of example neurons.
( a ) Activity of a single neuron (monkey S, right hemi.) recorded in the SMT pre , DMT-Up, DMT-Down, DMT-FR std , DMT-FR easy , and SMT post conditions. Conventions as in Fig. 4 . From left to right, seven memory task conditions, including two SMT post blocks (SMT post -1 and SMT post -2), are shown in the order of recording, except for DMT-Up, DMT-Down and DMT-FR std . For this and three other neurons (monkey S) with spatially-selective delay-period activity in SMT pre , activities were obtained in both the standard and easy dual-tasks. ( a ) Activity of a single neuron (monkey A, left hemi.) recorded in SMT pre , DMT-Up, DMT-Down, DMT-FR std , and SMT post . Note that in DMT-Down, the activity level was elevated in all memory cue locations because the attention cue had been presented near the neuron’s maximum response location (315°). Nevertheless, spatial selectivity of delay-period activity was lost in this condition ( P = 0.75). ( b ) Activity of a single neuron (monkey A, left hemi.) recorded in SMT pre , DMT-FR easy , and SMT post . This neuron exhibited delay-period activity similar to that in panel ( b ). However, attenuation of both the magnitude and selectivity of delay-period activity was absent in DMT-FR easy .

Supplementary Figure 6 Population cue-period activities of individual monkeys.
( a , b ) Data are for monkeys S ( a ) and A ( b ). Upper row: population cue-period activity in the maximum (blue line) and minimum (red line) response locations across the six memory task conditions. In both monkeys, significant interaction effects [Task condition × Cue location] were observed among cue-period activity (monkey S: main effect of Task condition, F 5,187 = 10.85, P < 10 –4 ; Cue location, F 1,187 = 114.88, P < 10 –4 ; interaction, F 5,187 = 10.52, P < 10 –4 ; monkey A : main effect of Task condition, F 5,185 = 0.54, P = 0.76; Cue location, F 1,185 = 154.90, P < 10 –4 ; interaction, F 5,185 = 3.57, P = 0.004; two-way mixed design ANOVA). Bottom row: population spatial tuning during the cue-period. Conventions as in Fig. 5 . Although, in both monkeys, cue-period activity exhibited a significant attenuation of spatial selectivity under DMT, the degree of attenuation was smaller in monkey A, whose cue-period activity exhibited a robust increase following memory cue onset. This suggests that, in monkey A, information processing of the memory task was rather unaffected in the initial phase (time period immediately following memory cue presentation), whereas in monkey S, processing of the memory task was considerably disrupted from this phase. In agreement with this notion, comparison of the behavioral performance between the two monkeys showed that monkey A exhibited a more moderate dual-task interference on memory task performance than monkey S ( Fig. 2c ). This was particularly evident in the trials that had short memory delay period (< 2.0 s) ( Supplementary Fig. 2a ). In addition, monkey A’s oculomotor behavior following memory cue presentation did not show signs of dual-task interference, whereas monkey S’s oculomotor behavior in the same epoch clearly exhibited interference by the attention task, as indicated by a significant increase in FB error rates in DMT-Up and DMT-Down relative to SMT ( Supplementary Fig. 2b–d ).
Supplementary Figure 7 Population delay-period activities of individual monkeys.
( a, b ) Data are for monkeys S ( a ) and A ( a ). Upper row: population delay-period activity in the maximum (blue line) and minimum (red line) response locations across the six memory task conditions. In both monkeys, significant interaction effects [Task condition × Cue location] were observed among delay-period activity (monkey S: main effect of Task condition, F 5,126 = 4.10, P = 0.002; Cue location, F 1,126 = 66.05, P < 10 –4 ; interaction, F 5,126 = 5.13, P = 3 × 10 –4 ; monkey A : main effect of Task condition, F 5,165 = 0.64, P = 0.67; Cue location, F 1,165 = 65.64, P < 10 –4 ; interaction, F 5,165 = 3.77, P = 0.003). Bottom row: population spatial tuning during the delay-period. Conventions as in Fig. 5 . In contrast to the cue-period activity, the degree of selectivity attenuation among delay-period activity was comparable between the two monkeys in the DMT conditions, suggesting that, in both monkeys, memory task processing was substantially disrupted in the later stage by the presence of the concurrent attention task. In accordance with this notion, behavioral results showed that, in both monkeys, prominent dual-task interference was observed in the trials that had long memory delay-period (> 4.0 s) ( Supplementary Fig. 2a ). The close correspondence between the individual variability among behavioral performance and that among response patterns of cue- and delay-period activities further supports the notion that the attenuation of neuronal selectivity for the memory cue location under DMT is a direct neural correlate of the behavioral cost of dual-task performance.
Supplementary Figure 8 Comparison of cue- and delay-period activities in SMT between neurons assigned to the recording in the standard dual-task and the easy dual-task.
(a,b) Upper row: population cue-period activities in the SMT pre ( a ) and SMT post ( b ) conditions for neurons assigned to the recording in the standard dual-task (left) and the easy dual-task (right). Conventions are the same as in Fig. 5 . In both conditions, the activity patterns were highly similar between the groups of neurons assigned to the standard dual-task (left) and the easy dual-task (right) (SMT pre : main effect of Task assignment, F 1,96 = 0.83, P = 0.36; Cue location, F 1,96 = 170.30, P < 10 –4 ; interaction, F 1,96 = 0.06, P = 0.81; SMT post : main effect of Task assignment, F 1,77 = 0.40, P = 0.53; Cue location, F 1,77 = 67.03, P < 10 –4 ; interaction, F 1,77 = 0.07, P = 0.79; two-way mixed-design ANOVA).Bottom row: population spatial tuning during the cue-period in SMT pre ( a ) and SMT post ( b ). In both SMT pre and SMT post , the tuning slopes and intercepts did not differ between the assigned task (SMT pre : slope, P = 0.92; intercept, P = 0.44; SMT post : slope, P = 0.56; intercept, P = 0.60). ( c , d ) Same as in ( a ) and ( b ), but for delay-period activity (SMT pre : main effect of Task assignment, F 1,73 = 0.16, P = 0.69; Cue location, F 1,73 = 92.18, P < 10 –4 ; interaction, F 1,73 = 0.28, P = 0.60; SMT post : main effect of Task assignment, F 1,56 = 0.78, P = 0.38; Cue location, F 1,56 = 43.21, P < 10 –4 ; interaction, F 1,56 = 1.54, P = 0.22). The tuning slopes and intercepts did not differ between the assigned task (SMT pre : slope, P = 0.65; intercept, P = 0.26; SMT post : slope, P = 0.49; intercept, P = 0.10).
Supplementary Figure 9 Comparison of single-neuron PEV values between the SMT and DMT conditions.
( a ) Upper row: scatter diagrams comparing PEV values of cue-period activity in SMT pre to those in the four DMT and SMT post conditions. Integration time window for PEV calculation was 0.4 s (0.1–0.5 s from memory cue onset). Blue dashed lines indicate the mean PEV values in SMT pre . Red dashed lines indicate the same neurons’ mean PEV values in the corresponding conditions for comparison. Fractions show the number of neurons that showed a decrease in PEV relative to SMT pre , divided by the number of neurons that exhibited spatially-selective cue-period activity in SMT pre . Bottom row: histograms comparing the distribution of PEV values between SMT pre (blue bars) vs. each of the four DMT and SMT post conditions (inverted red bars). ( b ) Same as in ( a ), but for delay-period activity. Integration time window was 1.0 s (0–1.0 s from memory cue offset). ( c ) Summary of the five paired-comparisons shown in ( a ). Note that n = 91 for SMT pre . The center of a notched bar indicates the median value, edges are CI 68% , and the error bar is the CI 95% of the median (bootstrap method). Open black circles indicate mean values. PEV values for cue-period activity were significantly different across memory task conditions (Kruskal-Wallis test, P = 4 × 10 –4 ), and SMT pre showed a significantly greater PEV value than DMT-Up, DMT-Down, and DMT-FR std ( post-hoc Steel-Dwass test, P < 0.02). All six memory task conditions gave median PEV values significantly larger than zero (one-sample Wilcoxon signed-rank test). ( d ) Summary of the five paired-comparisons in ( b ). Note that n = 71 for SMT pre . PEV values in delay-period activity were significantly different across memory task conditions (Kruskal-Wallis test, P = 0.001). SMT pre showed a significantly greater PEV value than DMT-Up, DMT-Down ( post-hoc Steel-Dwass test, P < 0.03) and a substantially greater PEV value than DMT-FR std ( P = 0.06). All six memory task conditions gave median PEV values significantly larger than zero.
Supplementary Figure 10 Comparison of memory task-related activity between the three-ring and one-ring layout types in the modified single memory task.
( a ) Spatially-selective cue-period activity of a representative neuron that exhibited almost identical activities in the two layout types. Conventions as in Fig. 3 . ( b ) Population activity in the 3-ring (top left) and 1-ring layout types (top right) for 13 spatially-selective cue neurons. A scatter diagram (bottom) shows a comparison of the strength of cue-period activity in the maximum (blue) and minimum (red) response locations that were selected from the five cue locations that were also used in DMT. Dotted lines indicate the mean cue-period activity across the population. The strength of cue-period activity was comparable between the two ring layout types at both the maximum ( P = 0.31) and minimum ( P = 0.19) response locations (Wilcoxon signed-rank test). ( c ) Same as in panel ( b ), but for 10 spatially-selective delay neurons. There was no significant difference in the strength of delay-period activity between the two layout types at both the maximum ( P = 0.92) and minimum ( P = 0.43) response locations.
Supplementary Figure 11 Temporal dynamics of neuronal signals representing attention and memory task information in the standard and easy dual-tasks.
( a ) Time course of neuronal signals of an example neuron (the neuron shown in Supplementary Fig. 5b ) representing the location of the attention cue (PEV attention , magenta), the memory cue (PEV memory , blue), and their interaction (PEV interaction , green) in the standard dual-task. Dashed cyan line indicates the same neuron’s PEV memory in SMT pre . Conventions are the same as in Fig. 8a . ( b ) Time course of neuronal signals of an example neuron (the neuron shown in Supplementary Fig. 5c ) representing the location of the memory cue (PEV memory , blue) in the easy dual-task. Dashed cyan line indicates the same neuron’s PEV memory obtained in SMT pre . ( c ) Population-averaged time course of PEV memory in the easy dual-task (solid blue line, n = 24). Shaded areas indicate s.e.m. The same neurons’ population-averaged PEV memory time series in SMT pre are plotted as a solid cyan line. Dashed blue line and dashed cyan line indicate population-averaged PEV memory time series in the standard dual-task and SMT pre , respectively for 51 neurons analyzed in Fig. 8 (the curves are the same as those shown in Fig. 8a ). ( d ) Time course of the proportion of neurons that exhibited significant information ( P < 0.05) about the memory cue location (solid blue line). The same neurons’ results in SMT pre are plotted as a solid cyan line. Dashed blue line and dashed cyan line indicate the results of the same analysis in the standard dual-task and SMT pre , respectively (both n = 51, the curves are the same as in Fig. 8f ). Horizontal dashed lines indicate the proportion expected by chance (5%).
Supplementary Figure 12 Comparison of spatial selectivity between the SMT and DMT conditions.
( a ) Comparison of behavioral performance between the SMT pre sessions with low percent correct rates and the DMT (standard dual-task) sessions with high percent correct rates. To perform this analysis, session-by-session percent correct rates in each memory task condition were rank-ordered and split at the median. The bottom half of SMT pre sessions and the top half of DMT sessions were selected. This analysis included 51 sessions where spatially-selective delay-period activity was recorded in SMT pre . Data from the three DMT conditions in the standard dual-task (DMT-Up, DMT-Down and DMT-FR std ) were collapsed. The sessions from the individual monkey were separately rank-ordered to avoid a biased subsampling from one monkey. The subsampled sessions gave highly similar percent correct rates between SMT pre and DMT (SMT pre : 95.4%, n = 26; DMT: 96.2%, n = 26; Wilcoxon rank-sum test, P = 0.99). Conventions as in Fig. 2c . ( b ) Time course of PEV attention (magenta), PEV memory (blue), and PEV interaction (green) in the standard dual-task for the 26 subsampled sessions. The magnitude of PEV memory during the delay-period ( D ) was significantly attenuated relative to that in SMT pre ( n = 26, dashed cyan line) (Wilcoxon rank-sum test, P = 0.03). Conventions as in Fig. 8 . ( c ) Comparison of PEV memory between the pre-T col change period and the follow-up fixation period in the standard dual-task. Following the conclusion of the attention task events, PEV memory in the standard dual-task exhibited significant reawakening. ( d ) Comparison of PEV memory and PEV attention during the follow-up fixation period in the standard dual-task. The reawakening of PEV memory during the follow-up fixation period coincided with the reprioritization of task processing between the attention and memory tasks. ( e ) Normalized population-averaged delay-period activity (grey shaded area) in the maximum (blue line) and minimum (red line) response locations in SMT pre and the three DMT conditions in the standard dual-task for the subsampled sessions. For comparing delay-period activity across the four conditions, behavioral performance in DMT-Up, DMT-Down and DMT-FR std were rank-ordered separately. The subsampled sessions gave similar percent correct rates across the four conditions ( P = 0.14, n = 26 for each of the four conditions,). For each neuron, firing rate in each 50-ms bin was divided by the peak delay-period firing rate at the maximum response location in the SMT pre condition. Compared with SMT pre , the difference in activity between the maximum and minimum response locations was remarkably attenuated in DMT-Up, DMT-Down and DMT-FR std (main effect of Task condition, F 3,100 = 0.15, P = 0.93; Cue location, F 1,100 = 52.95, P < 10 –4 ; interaction, F 3,100 = 6.25, P = 6 × 10 –4 ; two-way mixed design ANOVA). Conventions as in Fig. 5c . ( f ) Comparison of PEV values of the delay-period activity between the SMT and three DMT conditions. The three DMT conditions in the standard dual-task exhibited attenuation in spatial selectivity relative to SMT pre (Kruskal-Wallis test, P = 0.03). Conventions as in Supplementary Fig. 9d . Similar result was obtained when the rank-order of sessions was done over monkey-collapsed data (dotted line, P = 0.03).
Supplementary information
Supplementary text and figures.
Supplementary Figures 1–12 (PDF 11028 kb)
Source data
Source data to fig. 1, source data to fig. 2, source data to fig. 3, source data to fig. 4, source data to fig. 5, rights and permissions.
Reprints and permissions
About this article
Cite this article.
Watanabe, K., Funahashi, S. Neural mechanisms of dual-task interference and cognitive capacity limitation in the prefrontal cortex. Nat Neurosci 17 , 601–611 (2014). https://doi.org/10.1038/nn.3667
Download citation
Received : 12 September 2013
Accepted : 03 February 2014
Published : 02 March 2014
Issue Date : April 2014
DOI : https://doi.org/10.1038/nn.3667
Share this article
Anyone you share the following link with will be able to read this content:
Sorry, a shareable link is not currently available for this article.
Provided by the Springer Nature SharedIt content-sharing initiative
This article is cited by
Dataset of human-single neuron activity during a sternberg working memory task.
- Michael Kyzar
- Jan Kamiński
- Ueli Rutishauser
Scientific Data (2024)
Change in activity patterns in the prefrontal cortex in different phases during the dual-task walking in older adults
- Chang Yoon Baek
- Hyeong Dong Kim
- Jang Woo Lee
Journal of NeuroEngineering and Rehabilitation (2023)
Knowledge generalization and the costs of multitasking
- Kelly G. Garner
- Paul E. Dux
Nature Reviews Neuroscience (2023)
Cycles of goal silencing and reactivation underlie complex problem-solving in primate frontal and parietal cortex
- Mikiko Kadohisa
- John Duncan
Nature Communications (2023)
Modulation of inhibitory communication coordinates looking and reaching
- Maureen A. Hagan
- Bijan Pesaran
Nature (2022)
Quick links
- Explore articles by subject
- Guide to authors
- Editorial policies
Sign up for the Nature Briefing newsletter — what matters in science, free to your inbox daily.

EDITORIAL article
Editorial: multitasking: executive functioning in dual-task and task switching situations.
- 1 Department of Psychology, Medical School Hamburg, Hamburg, Germany
- 2 Department of Psychology, Eberhard Karls University Tübingen, Tübingen, Germany
Editorial on the Research Topic Multitasking: Executive Functioning in Dual-Task and Task Switching Situations
Persons are often engaged in activities that combine multiple tasks (so called multitasking ), even though this combination is typically accompanied by performance costs in the individual tasks in comparison to their performance as single tasks. Such performance costs suggest that performing multiple tasks brings the cognitive processing system to its limits. However, the observed limitations can inform theories of how cognitive processing is generally organized. In other words, investigations on the limitations of multitasking performance can reveal fundamental aspects of the cognitive processing architecture and mechanisms of human information processing.
These aspects have been investigated with a variety of experimental paradigms typically comprising two different component tasks that vary in the degree of temporal overlap. While it is difficult to define with precision what constitutes a “task” ( Rogers and Monsell, 1995 ; Monsell, 2003 ; Kiesel et al., 2010 ), one can define “task” broadly, so that (i) simple stimulus-response (S-R) translations [e.g., press a response key when hearing a low tone in so-called choice reaction time (RT) tasks], (ii) continuous tasks like motor tracking, (iii) complex movements (e.g., type writing), or (iv) tasks without necessarily yielding overt behavior (e.g., counting) can constitute a task if a person aims to achieve a discriminable goal state. Irrespective of the specific type of task, multitasking research includes research on dual-task performance and task switching performance ( Pashler, 2000 ). While dual-task performance requires concurrent and simultaneous task processing and motor responses, task switching focuses on multitasking with sequentially processed component tasks.
Dual-task Paradigms, Theories, and Executive Functioning
Generally speaking, there are two paradigms to investigate dual-tasking. In the simplest version, dual-task performance is compared with single-task performance, with only one stimulus/task being presented in the latter condition. Notably, in this paradigm, there is either a single stimulus or two simultaneous stimuli at the same time, and task load is manipulated in a one-vs.-two manner. In other words, participants either perform one task or two tasks per block. Dual-task performance costs are reflected in worse performance in dual- compared with the single-task conditions (e.g., Fagot and Pashler, 1992 ; Huestegge and Koch, 2009 ) 1 .
A second dual-task paradigm employs (most often) two choice RT tasks and varies the amount of temporal overlap of the two tasks. This overlapping task paradigm is nowadays often referred to as the Psychological Refractory Period (PRP) paradigm ( Welford, 1952 ; Pashler and Johnston, 1989 ; Pashler, 1994 ). More specifically, stimuli of the two tasks are presented in a predictable order separated by a variable stimulus onset asynchrony (SOA). With short SOA (e.g., 50 ms), task overlap is high while with a long SOA (e.g., 1,000 ms) task overlap is low. Typically, RTs of Task 2 increase, the shorter the SOA between both tasks are (i.e., the PRP effect; see Janczyk et al., 2014 , for exceptions to the PRP effect), while the SOA has no or only a small influence on RTs of Task 1 (see Strobach et al., 2015 , for more information on Task 1 data and results).
In particular to explain the PRP effect, the prominent central bottleneck theory ( Welford, 1952 ) holds that the selection of a response cannot be made for two tasks in parallel, while the initial perception stage (during which stimulus information is processed) and the final motor response stage (during which the motor response is executed) can run in parallel. Thus, response selection is conceived as a structural and unavoidable central processing bottleneck, leading to a long interruption of Task 2 processing at short vs. long SOAs and, hence, the PRP effect ( Pashler, 1994 ). According to other bottleneck theories, a bottleneck exists in the motor response stage, preventing two responses from being initiated simultaneously or in close succession, as an alternative to the response selection bottleneck or in addition to it (e.g., De Jong, 1993 ; Sigman and Dehaene, 2006 ; Bratzke et al., 2009 ).
Resource theories , in contrast, assume that the critical capacity-limited stages can run in parallel, but as they share a common and limited attentional resource, this processing is less efficient compared with a single-task condition (e.g., Navon and Miller, 2002 ; Tombu and Jolicœur, 2003 ; Wickens, 2008 ). As was shown by Navon and Miller (2002) and Tombu and Jolicœur (2003) , such capacity-sharing models can in fact explain many of the phenomena usually taken as evidence for bottleneck models. Further, if all capacity is first devoted to Task 1 and then to Task 2, the models mimic essentially a bottleneck model, which can thus be seen as a special case of capacity-sharing models.
Several studies also used variations of the PRP paradigm for analyses of executive control functions ( Jiang et al., 2004 ; Strobach et al., 2012 , 2014 ), for example, PRP experiments in which the order of the two tasks was not predictable ( Sigman and Dehaene, 2006 ; Kamienkowski et al., 2011 ; Riuz Fernández et al., 2011 ; Hendrich et al., 2012 ; Töllner et al., 2012 ). The executive functions thought to be involved in performing such tasks are conceived as general-purpose control mechanisms that regulate the dynamics of human cognition and action ( Miyake et al., 2000 ; Miyake and Friedman, 2012 ). In the context of dual-tasks, such control mechanisms coordinate the processing of two simultaneous task streams and the access to capacity-limited processing stages (e.g., De Jong, 1995 ; Luria and Meiran, 2003 ; Sigman and Dehaene, 2006 ; Szameitat et al., 2006 ). Exemplary empirical evidence for the flexible access to capacity-limited stages comes from the observation of a general increase of RTs for Task 1 in PRP dual-task RTs compared to single-task RTs, which points to the implication of time-consuming coordination processes at the beginning of dual-task trials (e.g., Jiang et al., 2004 ). From a perspective of executive processes, dual-task performance data may thus point to a set of well-identifiable task coordination processes. Recent studies investigated, for example, the impact of practice (e.g., Strochbach and Schubert, 2017 ), age (e.g., Maquestiaux, 2016 ), compatibility of stimulus and response information (e.g., Hazeltine et al., 2006 ), or recently experienced conflict (e.g., Janczyk, 2016 ) on dual-task performance and executive functioning in dual-tasks. In the following section, we provide a brief overview on papers of the present research topic aiming to contribute to the further specification of executive functions implicated in dual-tasking.
Dual-task Studies in the Present Research Topic
Hommel et al. investigated the impact of binaural beats on cognitive flexibility to control two simultaneous tasks with overlapping task information in the PRP paradigm. Their findings showed that binaural beats can modulate the flexibility of executive control functions in dual-tasks. Thus, this method has the potential to bias the executive control style in dual-tasks. Schubert et al. investigated the contribution of dual-task coordination skills to a reduction of dual-task costs as a result of practice. The authors showed that these skills are fully independent from practice situations and are transferable to new dual-tasks. Pieczykolan and Huestegge investigated whether flexible control of dual responses varies depending on task complexity, manipulated as the number of task-relevant response combinations and the to-be-retrieved S-R translation rules. Their findings showed that the increase of both, response combination and the S-R translation rules as well as their preparation yielded an increase of dual-task costs. In sum, the findings stress the importance of memory retrieval processes in dual-response control.
From an aging perspective, it is known that older adults are particularly impaired in dual-tasks compared with single-tasks and young adults (e.g., Verhaeghen et al., 2003 ; Verhaeghen, 2011 ). Therefore, it is relevant to investigate why older adults are impaired in dual-task situations and whether they are particularly impaired in these situations' executive control functions. In a real-world task setting, Stelzel et al. investigated the characteristics of this impairment with a specific focus on the compatibility of input and output modality pairings in the two tasks. They demonstrated that dual-task postural control is impaired in older adults in contrast to young adults particularly with incompatible input-output modality pairings. A real-world task was also applied by Steinborn and Huestegge who combined mental arithmetic and phone conversation in a continuous dual-task paradigm. In the context of their attentional-failure account, they showed that mental arithmetic affected different aspects of phone conversation: information processing in participants' conversation was particularly slowed down for controlled processing components in comparison to automatic components. de Tomasso et al. analyzed electroencephalic and electromyographic responses in a passive auditory oddball paradigm for both patients with Huntington's Disease and healthy controls. A similar increase in the amplitude of the P3 component was observed for both groups when auditory stimulation was presented in dual-task situations with walking. Finally, Xing and Sun applied a dual-task situation to characterize rule-based category learning. These authors showed that the effectiveness of this type of learning is mainly affected by the load of visuospatial information on working memory in a dual-task context. Thus, this dual-task study potentially informs about the structure of the working memory component that coordinates dual-tasking.
Task Switching Paradigms, Theories, and Executive Functioning
Task switching refers to a multitasking situation where two or more tasks are presented sequentially without temporal overlap (e.g., Monsell, 2003 ; Kiesel et al., 2010 ). Contrasting with most of the studies on dual-tasking, the stimuli presented in task switching situations afford not only the currently relevant task but also the other task(s). For instance, participants may be presented with colored shapes as stimuli and frequently alternate between judging the color (Task A) or the shape (Task B). Another frequently used experimental protocol requires switching between purely semantic tasks, such as when participants judge the magnitude (Task A) vs. the parity (Task B) of stimulus digits. In single-task blocks, either Task A or Task B is presented exclusively. In mixed blocks, participants are confronted with both tasks either in a pre-specified task sequence such as AABBAABB (i.e., alternating runs paradigm; Rogers and Monsell, 1995 ) or with a random task sequence and a task cue that precedes or accompanies stimulus presentation (i.e., task cueing paradigm; Meiran, 1996 ). In these mixed blocks, the tasks can either repeat from one trial to the next (i.e., task repetitions) or switch (i.e., task switches), and two types of performance costs can be assessed. First, mixing costs are defined as the difference between the mean performance in trials with task repetitions in mixed blocks and the mean performance in single-task blocks ( Koch et al., 2005 ; Rubin and Meiran, 2005 ). Second, switch costs are defined as the difference between the performance in task switch trials and the performance in task repetition trials within the mixed blocks ( Rogers and Monsell, 1995 ).
The most prominent theoretical issue in task switching research has been the question of the origin of switch costs, particularly of so-called residual switch costs that are consistently found even after long preparation intervals during which participants have foreknowledge about the identity of the upcoming task. Although some accounts attribute residual switch costs to the duration of an executive process of task-set reconfiguration, occurring after encoding of the task stimulus ( Rogers and Monsell, 1995 ; Rubinstein et al., 2001 ), there seems to be broad consensus that at least part of residual switch costs reflect priming from previous execution of the other task (e.g., Allport et al., 1994 ). In this regard, particular interest has been devoted to the role of task-set inhibition. Although the precise role of task-set inhibition concerning the residual switch costs is still unclear, convincing evidence for task-set inhibition is seen in the N-2 task repetition effect (a.k.a. the backward inhibition effect), found in task switching protocols that involve three different tasks (i.e., Tasks A, B, and C). The N-2 task repetition effect refers to the finding that the final trial of an ABA task sequence tends to be associated with slower responses than the final trial of a CBA sequence ( Mayr and Keele, 2000 ), as would be expected if performance suffered from inhibition of the task-set for Task A in the former but not (or less so) in the latter case. Another major point in the task switching literature refers to attempts of specifying the processes involved in task preparation. Effective task preparation has been inferred from findings of improved performance when the preparation interval that precedes the presentation of the imperative stimulus is increased ( Rogers and Monsell, 1995 ; Meiran, 1996 ). The precise processes involved in task preparation have proved difficult to determine, however (overviews in Karayanidis et al., 2010 ; Kiesel et al., 2010 ). More recent developments in task switching research refer to effects of task switching practice (e.g., Minear and Shah, 2008 ), individual differences (e.g., von Bastian and Druey, 2017 ), or the comparison of voluntary task selection with instructional task cuing (e.g., Arrington and Logan, 2004 ).
Task Switching Studies in the Present Research Topic
Articles included in the present research topic contribute to our understanding concerning the classical questions of task-set inhibition and task preparation as well as concerning practice, age-related differences, and voluntary task selection. As regards task-set inhibition, Schuch , using a diffusion model analysis, specified that older adults are not generally impaired in task inhibition in comparison to younger adults. Alternatively, there are age differences in dealing with task inhibition as reflected by differences in the speed-accuracy trade-off between these age groups. Jost et al. investigated whether task dominance determines backward inhibition. The results of their study showed that inhibition was stronger for more dominant tasks, suggesting that the amount of inhibition is adjusted in a context-sensitive manner. Concerning task preparation, Kleinsorge and Scheil presented redundant pre-cues that constrain the number of possible tasks from four to two before the task was cued unambiguously (replicating previous findings of an advantage of such pre-cuing; Kleinsorge and Scheil, 2015 ) and analyzed spontaneous eye blink rates. Changes in the eye blink rate during the initial part of the experimental session were correlated with pre-cuing benefit. Distinguishing between the preparation of perceptual and non-perceptual task processes, Wendt et al. focused on situations in which tasks differed regarding their perceptual demands of stimulus selection. Intermixing trials of a probe task they found evidence for preparatory adoption of task-specific attentional sets, that is, for focusing or defocusing of visual attention depending on the stimulus selection demands of a likely upcoming task. Wendt et al. , by contrast, investigated preparation in the absence of a difference in perceptual demands between tasks. Analyzing task switching performance across six consecutive sessions, they extended previous evidence suggesting that task switching practice results in a speed-up of the preparation to non-perceptual preparatory processes. This study also introduced a probe task method—similar to the one applied by Wendt et al. —to research on task switching practice.
Buttelmann and Karbach's as well as Kray and Fehér's focus of interest is on age-related effects on task switching practice. Buttelmann and Karbach review the findings of training interventions and transfer in early and middle childhood, revealing substantial plasticity for different aspects of cognitive flexibility. Kray and Fehér assess the transferability of improved task switching performance after practice in young and older adults. Their findings suggest that the requirement to resolve interference between tasks is critical for the occurrence of transfer particularly in the elderly. A comparison of voluntary and instructed task selection—concerning the impact of task-specific action effects—was made by Sommer and Lukas . Finally, Moon et al. investigated interruptions of a visuo-tactile task by a second task that also involved visual and haptic stimuli, providing evidence for a helpful role of redundant haptic information in reducing the cost of interruption.
In sum, the present research topic combines recent research in dual-tasks and task switching, focusing on the impact of executive functioning in these types of multitasking situations. In addition to the specific research issues addressed by the individual contributions, this collection of studies nicely shows the diversity of theoretical questions and methodological approaches in contemporary cognitive-neuroscientific research in this area.
Author Contributions
All authors listed have made a substantial, direct and intellectual contribution to the work, and approved it for publication.
Conflict of Interest Statement
The authors declare that the research was conducted in the absence of any commercial or financial relationships that could be construed as a potential conflict of interest.
1. ^ To avoid confounds with, for example, how many S-R translations need to be maintained in working memory, a third condition is sometimes employed where in each trial only one stimulus is presented, but potentially stimuli from both tasks can occur within one block (so called mixed blocks or heterogeneous single-task blocks; e.g., Schumacher et al., 2001 ; Strobach et al., 2014 ; Janczyk et al., 2015 ).
Allport, A., Styles, E. A., and Hsieh, S. (1994). “Shifting intentional set: exploring the dynamic control of tasks,” in Conscious and Nonconscious Information Processing: Attention and Performance XV , eds C. Umilta and M. Moscovitch (Cambridge, MA: MIT Press), 421–452.
Arrington, C. M., and Logan, G. D. (2004). The cost of a voluntary task switch. Psychol. Sci. 15, 610–615. doi: 10.1111/j.0956-7976.2004.00728.x
PubMed Abstract | CrossRef Full Text | Google Scholar
Bratzke, D., Rolke, B., and Ulrich, R. (2009). The source of execution-related dual-task interference: motor bottleneck or response monitoring? J. Exp. Psychol. Hum. Percept. Perform. 35, 1413–1426. doi: 10.1037/a0015874
CrossRef Full Text | Google Scholar
De Jong, R. (1993). Multiple bottlenecks in overlapping task performance. J. Exp. Psychol. Hum. Percept. Perform. 19, 965–980. doi: 10.1037/0096-1523.19.5.965
De Jong, R. (1995). The role of preparation in overlapping-task performance. Q. J. Exp. Psychol. 48, 2–25. doi: 10.1080/14640749508401372
Fagot, C., and Pashler, H. (1992). Making two responses to a single object: implications for the central attentional bottleneck. J. Exp. Psychol. Hum. Percept. Perform. 18, 1058–1079.
PubMed Abstract | Google Scholar
Hazeltine, E., Ruthruff, E., and Remington, R. W. (2006). The role of input and output modality pairings in dual-task performance: evidence for content-dependent central interference. Cogn. Psychol. 52, 291–345. doi: 10.1016/j.cogpsych.2005.11.001
Hendrich, E., Strobach, T., Buss, M., Müller, H. J., and Schubert, T. (2012). Temporal-order judgment of visual and auditory stimuli: modulations in situations with and without stimulus discrimination. Front. Integr. Neurosci. 6:63. doi: 10.3389/fnint.2012.00063
Huestegge, L., and Koch, I. (2009). Dual-task crosstalk between saccades and manual responses. J. Exp. Psychol. Hum. Percept. Perform. 35, 352–362. doi: 10.1037/a0013897
Janczyk, M. (2016). Sequential modulation of backward crosstalk and task-shielding in dual-tasking. J. Exp. Psychol. Hum. Percept. Perform. 42, 631–647. doi: 10.1037/xhp0000170
Janczyk, M., Nolden, S., and Jolicoeur, P. (2015). No differences in dual-task costs between forced-and free-choice tasks. Psychol. Res. 79, 463–477. doi: 10.1007/s00426-014-0580-6
Janczyk, M., Pfister, R., Wallmeier, G., and Kunde, W. (2014). Exceptions to the PRP effect? A comparison of prepared and unconditioned reflexes. J. Exp. Psychol. Learn. Mem. Cognit. 40, 776–786. doi: 10.1037/a0035548
Jiang, Y., Saxe, R., and Kanwisher, N. (2004). Functional magnetic resonance imaging provides new constraints on theories of the psychological refractory period. Psychol. Sci. 15, 390–396. doi: 10.1111/j.0956-7976.2004.00690.x
Kamienkowski, J. E., Pashler, H., Dehaene, S., and Sigman, M. (2011). Effects of practice on task architecture: combined evidence from interference experiments and random-walk models of decision making. Cognition 119, 81–95. doi: 10.1016/j.cognition.2010.12.010
Karayanidis, F., Jamadar, S., Ruge, H., Phillips, N., Heathcote, A., and Forstmann, B. U. (2010). Advance preparation in task-switching: converging evidence from behavioral, brain activation, and model-based approaches. Front. Psychol. 1:25. doi: 10.3389/fpsyg.2010.00025
Kiesel, A., Steinhauser, M., Wendt, M., Falkenstein, M., Jost, K., Philipp, A. M., et al. (2010). Control and interference in task switching—a review. Psychol. Bull. 136, 849–874. doi: 10.1037/a0019842
Kleinsorge, T., and Scheil, J. (2015). Task switching among two or four tasks: effects of a short-term variation of the number of candidate tasks. Psychol. Res. 79, 163–173. doi: 10.1007/s00426-013-0532-6
Koch, I., Prinz, W., and Allport, A. (2005). Involuntary retrieval in alphabet-arithmetic tasks: Task-mixing and task-switching costs. Psychol. Res. 69, 252–261. doi: 10.1007/s00426-004-0180-y
Luria, R., and Meiran, N. (2003). Online order control in the psychological refractory period paradigm. J. Exp. Psychol. Hum. Percept. Perform. 29, 556–574. doi: 10.1037/0096-1523.29.3.556
Maquestiaux, F. (2016). Qualitative attentional changes with age in doing two tasks at once. Psychon. Bull. Rev. 23, 54–61. doi: 10.3758/s13423-015-0881-9
Mayr, U., and Keele, S. W. (2000). Changing internal constraints on action: the role of backward inhibition. J. Exp. Psychol. Gen. 129, 4–26. doi: 10.1037/0096-3445.129.1.4
Meiran, N. (1996). Reconfiguration of processing mode prior to task performance. J. Exp. Psychol. Learn. Mem. Cognit. 22, 1423–1442. doi: 10.1037/0278-7393.22.6.1423
Minear, M., and Shah, P. (2008). Training and transfer effects in task switching. Mem. Cognit. 36, 1470–1483. doi: 10.3758/MC.336.8.1470
Miyake, A., and Friedman, N. P. (2012). The nature and organization of individual differences in executive functions: four general conclusions. Curr. Dir. Psychol. Sci. 21, 8–14. doi: 10.1177/0963721411429458
Miyake, A., Friedman, N. P., Emerson, M. J., Witzki, A. H., Howerter, A., and Wager, T. D. (2000). The unity and diversity of executive functions and their contributions to complex “frontal lobe” tasks: a latent variable analysis. Cogn. Psychol. 41, 49–100. doi: 10.1006/cogp.1999.0734
Monsell, S. (2003). Task switching. Trends Cogn. Sci. (Regul. Ed). 7, 134–140. doi: 10.1016/S1364-6613(03)00028-7
Navon, D., and Miller, J. (2002). Queuing or sharing? A critical evaluation of the single-bottleneck notion. Cognit. Psychol. 44, 193–251. doi: 10.1006/cogp.2001.0767
Pashler, H. (1994). Dual-task interference in simple tasks: data and theory. Psychol. Bull. 116, 220–244. doi: 10.1037/0033-2909.116.2.220
Pashler, H. (2000). “Task switching and multitask performance,” in Attention and Performance, XVIII: Control of Mental Processes , eds S. Monsell and J. Driver (Cambridge, MA: MIT Press), 277–307.
Google Scholar
Pashler, H., and Johnston, J. C. (1989). Chronometric evidence for central postponement in temporally overlapping tasks. Q. J. Exp. Psychol. A 41, 19–45. doi: 10.1080/14640748908402351
Riuz Fernández, S., Leonhard, T., Rolke, B., and Ulrich, R. (2011). Processing two tasks with varying task order: central stage duration influences central processing order. Acta Psychol. (Amst). 137, 10–17. doi: 10.1016/j.actpsy.2011.01.016
Rogers, R. D., and Monsell, S. (1995). Costs of a predictible switch between simple cognitive tasks. J. Exp. Psychol. Gen. 124, 207–231. doi: 10.1037/0096-3445.124.2.207
Rubin, O., and Meiran, N. (2005). On the origins of the task mixing cost in the cuing task-switching paradigm. J. Exp. Psychol. Learn. Mem. Cognit. 31, 1477–1491. doi: 10.1037/0278-7393.31.6.1477
Rubinstein, J. S., Meyer, D. E., and Evans, J. E. (2001). Executive control of cognitive processes in task switching. J. Exp. Psychol. Hum. Percept. Perform. 27, 763–797. doi: 10.1037/0096-1523.27.4.763
Schumacher, E. H., Seymour, T. L., Glass, J. M., Fencsik, D. E., Lauber, E. J., Kieras, D. E., et al. (2001). Virtually perfect time sharing in dual-task performance: uncorking the central cognitive bottleneck. Psychol. Sci. 12, 101–108. doi: 10.1111/1467-9280.00318
Sigman, M., and Dehaene, S. (2006). Dynamics of the central bottleneck: dual-task and task uncertainty. PLoS Biol. 4:e220. doi: 10.1371/journal.pbio.0040220
Strobach, T., Liepelt, R., Schubert, T., and Kiesel, A. (2012). Task switching: effects of practice on switch and mixing costs. Psychol. Res. 76, 74–83. doi: 10.1007/s00426-011-0323-x
Strobach, T., Salminen, T., Karbach, J., and Schubert, T. (2014). Practice-related optimization and transfer of executive functions: a general review and a specific realization of their mechanisms in dual tasks. Psychol. Res. 78, 836–851. doi: 10.1007/s00426-014-0563-7
Strobach, T., and Schubert, T. (2017). Mechanisms of practice-related reductions of dual-task interference with simple tasks: data and theory. Adv. Cognit. Psychol. 13, 28–41. doi: 10.5709/acp-0204-7
Strobach, T., Schütz, A., and Schubert, T. (2015). On the importance of Task 1 and error performance measures in PRP dual-task studies. Front. Psychol. 6:406. doi: 10.3389/fpsyg.2015.00403
Szameitat, A. J., Lepsien, J., von Cramon, D. Y., Sterr, A., and Schubert, T. (2006). Task-order coordination in dual-task performance and the lateral prefrontal cortex: an event-related fMRI study. Psychol. Res. 70, 541–552. doi: 10.1007/s00426-005-0015-5
Töllner, T., Strobach, T., Schubert, T., and Müller, H. J. (2012). The effect of task order predictability in audio-visual dual task performance: just a central capacity limitation? Front. Integr. Neurosci. 6:75. doi: 10.3389/fnint.2012.00075
Tombu, M., and Jolicœur, P. (2003). A central capacity sharing model of dual-task performance. J. Exp. Psychol. Hum. Percept. Perform. 29, 3–18. doi: 10.1037/0096-1523.29.1.3
Verhaeghen, P. (2011). Aging and executive control: reports of a demise greatly exaggerated. Curr. Dir. Psychol. Sci. 20, 174–180. doi: 10.1177/0963721411408772
Verhaeghen, P., Steitz, D. W., Sliwinski, M. J., and Cerella, J. (2003). Aging and dual-task performance: a meta-analysis. Psychol. Aging 18, 443–460. doi: 10.1037/0882-7974.18.3.443
von Bastian, C. C., and Druey, M. D. (2017). Shifting between mental sets: an individual differences approach to commonalities and differences of task switching components. J. Exp. Psychol. Gen. 146, 1266–1285. doi: 10.1037/xge0000333
Welford, A. T. (1952). The ‘psychological refractory period’ and the timing of high-speed performance—a review and a theory. Br. J. Psychol. Gen. Sect. 43, 2–19. doi: 10.1111/j.2044-8295.1952.tb00322.x
Wickens, C. D. (2008). Multiple resources and mental workload. Hum. Factors 50, 449–455. doi: 10.1518/001872008X288394
Keywords: dual tasking, task switching, executive functions, task coordination, task preparation
Citation: Strobach T, Wendt M and Janczyk M (2018) Editorial: Multitasking: Executive Functioning in Dual-Task and Task Switching Situations. Front. Psychol . 9:108. doi: 10.3389/fpsyg.2018.00108
Received: 21 December 2017; Accepted: 23 January 2018; Published: 15 February 2018.
Edited and reviewed by: Bernhard Hommel , Leiden University, Netherlands
Copyright © 2018 Strobach, Wendt and Janczyk. This is an open-access article distributed under the terms of the Creative Commons Attribution License (CC BY) . The use, distribution or reproduction in other forums is permitted, provided the original author(s) and the copyright owner are credited and that the original publication in this journal is cited, in accordance with accepted academic practice. No use, distribution or reproduction is permitted which does not comply with these terms.
*Correspondence: Tilo Strobach, [email protected]
Disclaimer: All claims expressed in this article are solely those of the authors and do not necessarily represent those of their affiliated organizations, or those of the publisher, the editors and the reviewers. Any product that may be evaluated in this article or claim that may be made by its manufacturer is not guaranteed or endorsed by the publisher.
- Search Menu
- Advance articles
- Editor's Choice
- Continuing Education
- Author Guidelines
- Submission Site
- Open Access
- Why publish with this journal?
- About Archives of Clinical Neuropsychology
- About the National Academy of Neuropsychology
- Journals Career Network
- Editorial Board
- Advertising and Corporate Services
- Self-Archiving Policy
- Dispatch Dates
- Journals on Oxford Academic
- Books on Oxford Academic
Article Contents
Introduction, materials and methods, conflict of interest, acknowledgement.
- < Previous
Assessing Dual-Task Performance Using a Paper-and-Pencil Test: Normative Data
- Article contents
- Figures & tables
- Supplementary Data
Sergio Della Sala, Jennifer A. Foley, Nicoletta Beschin, Mike Allerhand, Robert H. Logie, Assessing Dual-Task Performance Using a Paper-and-Pencil Test: Normative Data, Archives of Clinical Neuropsychology , Volume 25, Issue 5, August 2010, Pages 410–419, https://doi.org/10.1093/arclin/acq039
- Permissions Icon Permissions
Although several studies have described dual-tasking ability in normal aging, Mild Cognitive Impairment and Alzheimer's disease, no normative data for dual-task performance exist. Dual-tasking ability of 436 healthy individuals, aged 16–88 years, was assessed using a new paper-and-pencil dual-task paradigm. In this study, no age effect was detected, providing strong evidence that age does not affect dual-tasking abilities. Psychometric data for this new assessment are presented, which may enable clinicians and researchers to use this paradigm as a means of examining attentional control in dual-tasking.
Dual-tasking is the ability to perform two tasks concurrently. Dual-task paradigms have been instrumental in forging cognitive models of attentional control ( Fernandes & Moscovitch, 2000 ; Naveh-Benjamin, Craik, Guez & Kreuger, 2005 ), and in investigations of deficits in attentional control following focal and diffuse brain damage, including neurodegenerative disease ( Baddeley, Baddeley, Bucks & Wilcock, 2001 ; Balota & Faust, 2001 ; Logie, Cocchini, Della Sala & Baddeley, 2004 ). However, its use in clinical practice has been limited by the absence of a portable and easy-to-use version of the task, and by the lack of normative data.
Experimental paradigms assess dual-tasking ability by examining the ability to perform two tasks separately and then together. The difference between the performance on each of the single tasks and in the dual-task condition provides an index of dual-tasking ability. Therefore, it is important to minimize any difference in performance on each of the single tasks across individuals ( Salthouse, Rogan & Prill, 1984 ). Thus, the demand of individual tasks must be calibrated according to the ability of each individual to equate single task performance across participants.
The nature of the two tasks is a further important factor in the assessment of dual-tasking ability ( MacPherson, Della Sala, Logie & Wilcock, 2007 ). To enable examination of the ability to perform two tasks simultaneously, dual-task assessments should assess concurrent performance on two tasks that do not compete for the same processing mechanism ( Allport & Wylie, 2000 ; Monsell, 2003 ), such as aural presentation of digits for spoken recall coupled with visual and motor tracking ( Logie et al., 2004 ).
In addition, unless performance on both of these tasks is considered, this trade-off between tasks may go undetected ( Della Sala, Baddeley, Papagno & Spinnler, 1995 ). Thus, an accurate assessment of dual-tasking ability should include an overall measure of the impact of dual task on the performance of both tasks.
Several studies reported dual tasking to be poorer in older adults than in younger adults, with this age difference being exacerbated by increasing task demands ( Anderson, Craik & Naveh-Benjamin, 1998 ; Craik, Govoni, Naveh-Benjamin & Anderson, 1996 ; Craik & McDowd, 1987 ; Fernandes and Moscovitch, 2000 ; Hartley & Little, 1999 ; Lindenberger, Marsiske & Baltes, 2000 ; McDowd & Craik, 1988 ; Naveh-Benjamin et al., 2005 ). However, in these studies, the demands of each of the two individual tasks were not calibrated to the ability of each participant to equate single-task performance across age groups. Therefore, the age effect could have arisen because of baseline differences between age groups in their single-task abilities ( Salthouse, Fristoe, Lineweaver & Coon, 1995 ). Dual tasking is affected by aging when one of the two tasks involves reaction times ( Logie, Della Sala, MacPherson & Cooper, 2007 ; Macht & Buschke, 1983 ). Once care is taken to calibrate the performance on individual tasks so as to equate single-task performance across groups, and to use tasks that are not time based, this age effect disappears ( Baddeley, Logie, Bressi, Della Sala & Spinnler, 1986 ; Belleville, Rouleau & Caza, 1998 ; Logie et al., 2004 ; Salthouse et al., 1995 ). It has been suggested, therefore, that dual-tasking ability is not affected by normal aging when single-task differences with age are taken into account ( Logie et al., 2004 ).
In contrast, several studies that have calibrated the single-task performance between groups have found a striking impairment in dual-tasking ability in people with AD when compared with healthy age-matched controls ( Baddeley, Bressi, Della Sala, Logie & Spinnler, 1991 ; Baddeley et al., 1986 ; Della Sala et al., 1995 ; Holtzer, Burright & Donovick, 2004 ; Logie et al., 2004 ; MacPherson, Della Sala & Logie, 2004 ; MacPherson et al., 2007 ; Morris, 1986 ; Morris & Baddeley, 1988 ; Sebastian, Menor & Elosua, 2006 ). This dual-task deficit becomes more pronounced with the development of the disease and increasing severity ( Baddeley et al., 1991 , 2001 ; Della Sala et al., 1995 ). The specificity of the dual-task decrement in AD is further underlined by the lack of dual-task deficits in older people with chronic depression, even when they have been equated with AD patients for episodic memory performance ( Kaschel, Logie, Kazén & Della Sala, 2009 ). So, episodic memory appears to be sensitive to AD, but is not specific to the disease, whereas dual-task impairments appear to be specific to AD compared with chronic depression and with healthy aging. The cognitive function thought to be required for performing two concurrent tasks has been conceived as one of the executive functions within the working memory model ( Baddeley, 1996 ; Baddeley et al., 2001 ; Logie et al., 2004 ), with the AD impairments in dual tasks reflecting a specific deficit of this function ( Baddeley et al., 1986 , 1991 ; Della Sala et al., 1995 ; Della Sala & Logie, 2001 ; MacPherson et al., 2004 , 2007 ; Sebastian et al., 2006 ).
This dual-task impairment appears to be robust over practice ( Baddeley, Cocchini, Della Sala, Logie & Spinnler, 1999 ) and remains with many different task combinations, including memory and motor tasks ( Baddeley et al., 1986 ), two memory tasks ( Cocchini, Logie, Della Sala, MacPherson & Baddeley, 2002 ; MacPherson et al., 2007 ), and two everyday tasks, like walking and talking ( Cocchini et al., 2004 ).
Although the results were clear and have been replicated in a wide range of studies, the literature summarized above made use of computerized methods of assessing dual-tasking ability. These methods have a limited clinical utility, because they require bulky and expensive equipment, which necessitate training before usage.
Earlier attempts to develop simpler versions for clinical use ( Della Sala et al., 1995 ; Baddeley, Della Sala, Gray, Papagno & Spinnler, 1997 ) discussed the assessment of dual-tasking ability using digit sequence memory (with the experimenter reading out the sequences for oral recall) with either a paper-and-pencil maze test ( Della Sala et al., 1995 ; Baddeley et al., 1997 ) or a paradigm based on Fitts’ law ( Baddeley et al., 1997 ). Neither of these were successful as many participants found the Fitts’ law task too difficult, even in the single-task condition, and the mazes test was insufficiently reliable, rendering these tasks inadequate for the confident and accurate assessment of dual-tasking ability in clinical practice.
This paper describes a new version of dual task that uses paper and pencil for the tracking element. The aims of this study were to collect normative data on healthy individuals and identify the psychometric properties of a simplified version of the dual-task procedure.
Participants
A total of 486 people took part in this study. The older participants were recruited from the Somma Lombardo Hospital orthopedic department and from different social groups for older people. The younger participants were recruited among the relatives of the older people. The inclusion criteria were: No known neurological or psychiatric disease, no uncorrected visual or auditory impairment, and Mini-Mental Status Examination score above the age-specific cut-off. Participants were either tested in the Somma Lombardo Hospital or in their social groups, in Lombardy, Italy. Participants were assessed individually by an experienced Clinical Psychologist in a quiet room, free from distraction, either in the morning or in the afternoon.
Each participant completed the dual-task assessment. This consisted of performing digit recall and tracking tasks separately and then simultaneously. This dual-task assessment, relevant testing material and the full instructions for its administration and scoring, can be found at www.psy.ed.ac.uk/people/sdsala/tests/sdsdualtask/ .
Before commencing the digit recall task, digit span of each individual was established. Participants heard a list of digits at a rate of one per second. They were then asked to repeat these digits back in the same order as they heard them. The initial sequence length was two digits long and participants were presented with six sequences at each sequence length. If five out of the six sequences were recalled correctly, the digit sequence was lengthened by one digit. Once participants could no longer recall five out of the six digit sequences, digit span was taken as the maximum length at which the participant was able to recall five out of six digit sequences correctly. Next, participants heard a series of sequences, at an individual span length, for immediate oral serial-ordered recall over a period of 90 s. The number of lists that each participant heard and recalled during the 90-s period varied depending on their digit span, and thus the performance measure was the proportion of digits recalled correctly in the correct serial position.
The tracking task consisted of using a pencil to draw a line through circles arranged in a path around a sheet of A3 paper. Participants were given a shortened version for a practice trial, with only 17 circles, to ensure that they understood the task demands. After this, the participant was presented with the full version comprising 319 circles and was asked to start at one end of the path and draw a line through each successive circle as quickly as they could over a 90-s period. The performance measure was the number of circles crossed within the allotted time.
Reliability
To examine the test–retest reliability of the new dual-task assessment, a smaller sample of participants, representative of the larger group, completed the assessment a second time. All participants who agreed to be retested within the proposed interval were included in this reliability analysis. The time between the first and second assessments ranged from 1 hr to 1 day, based on the availability of the participant. This consisted of 176 healthy participants, aged 18–88 years (mean = 51.86, SD = 21.25) and an education range of 2–21 years (mean = 10.48, SD = 4.88).
Normative Data for the New Dual-Task Assessment
The data were not normally distributed, as they were significantly positively skewed ( z skewness = 8.07) and leptokurtic ( z kurtosis = 21.07). The data skew appeared to be caused by a few particularly high scores (as seen in Fig. 1 ). Therefore, the data were trimmed to exclude outliers more than two standard deviations ( SD s) above or below the mean decrement in digit recall, tracking, or combined performance.

Histogram of the distribution of the index of dual task performance ( μ ) before data trimming.
Fifty outliers were removed in total: 25 more than 2 SD s above and 25 more than two SD s below the mean decrement in digit recall, tracking, or combined dual-task performance. These 50 outliers (30 females, 57.7%) ranged in age between 23 and 87 years (mean = 62.12, SD = 20.24) and in education from 2 to 20 years (mean = 9.52, SD = 5.04). The remaining 436 individuals (254 females, 58.3%) ranged in age between 16 and 88 years (mean = 48.46, SD = 18.98) and in education from 2 to 22 years (mean = 11.62, SD = 4.45). Further participant demographic information, as a function of participant age, is found in Figs. 2 and 3 . The outliers were significantly older than the remaining group ( U = 6,879.50, p < .001), but there was no significant difference in the combined dual-tasking ability ( U = 11,274.00, p = .95).

Gender of participants, as a function of age.

Education of participants, as a function of age.
The outliers were relatively equally distributed about the mean, as seen in Fig. 4 , indicating that their removal should not introduce bias in the remaining data.

Distribution of outliers’ dual-task scores.
Group means and SD s for the digit span, digit recall, and tracking tasks are presented in Table 1 . Group means and SD s for dual-task performance of digit recall, tracking, and combined are presented in Table 2 .
Performance on digit span, digit recall (in the single- and dual-task conditions), and tracking (in the single- and dual-task conditions)
Dual-task performance of digit recall, tracking, and combined
Relationship between Dual-Task Performance and Gender, Age, and Education
Point-biserial correlation analyses showed that digit recall, tracking, and combined dual-task performance were unaffected by gender.
The dual-task performance of the tracking task was significantly correlated with years of education ( ρ = .21, p < .001) and age of participants ( ρ = −.25, p < .001). Similarly, the combined dual-task performance was significantly correlated with years of education ( ρ = .21, p <.001) and age of participants ( ρ = −.52, p < .001). However, the dual-task performance of the digit recall task was not affected by education ( ρ = −.01, p = .79) or age ( ρ = .04, p = .20). When the effect of education was partialled out, only tracking remained significantly correlated with age ( r = −.16, p < .01), but, crucially, not the combined dual-task performance ( r = −.06, p = .11).
There was a significant test–retest correlation for dual-task performance in digit recall ( r = .59, p < .001), tracking ( r = .73, p < .001), and combined ( r = .69, p < .001)]. There was a significant increase in digit recall [ t (175) = −2.27, p < .05], tracking [ t (175) = −3.84, p < .001], and combined dual-task performance [ t (175) = −4.34, p < .001), but these increases were small in magnitude (mean increase = 1.65%, 4.89%, 3.27%, respectively).
Dual-Task Performance: Normative Data
Fig. 5 shows the distribution of combined dual-task performance ( μ ), with a normal curve drawn in for comparison purposes. The distribution appears to be relatively normal, and passes the Shapiro–Wilk test of normality.

Histogram of the distribution of the index of dual-task performance ( μ ).
The uncertainty inherent in normality judgments based on hard cut-off scores is quantified using confidence levels. If cut-off scores are derived from sample estimates using parametric methods, the confidence levels are subject to assumptions about the distributions. However, the data obtained from task performance are typically skewed. The current data are skewed toward the higher scores ( z skewness = 0.51). It is argued elsewhere ( Capitani, 1997 ) that, for such data, thresholds for normality judgments are better established using nonparametric methods. The nonparametric tolerance interval ( Wilks, 1941 ) is distribution-free in the sense that the resulting tolerance limits depend solely upon the sample size and the coverage and confidence level parameters; they are entirely independent of the unknown distribution of data in the population. Nevertheless, the cut-off scores based on these limits can be regarded as inferential and population-based, since Wilks provided the exact sampling distribution of the tolerance interval that covers a given proportion of population values with a given confidence level.
Following Capitani (1997) , the normality judgments are based on the two cut-off scores, both derived from one-sided tolerance intervals: The outer and inner tolerance limits. The outer limit is calculated such that with 95% confidence the unbounded interval above the limit will cover at least 95% of normal population values. The corresponding inner limit is calculated such that with 95% confidence the interval above the limit will cover at most 95% of normal population values (see Supplementary Material for the method of calculation). The outer tolerance limit, which is the more conservative of the two, is used as the cut-off to classify a score as “abnormal”. The inner tolerance limit is used as the cut-off to judge a score “normal”. Scores between the outer and inner limits are considered “borderline”. Fig. 6 shows the scores in ranked order, and shows the cut-off scores corresponding to the outer and inner tolerance limits. Scores <81.0% are classified as “abnormal” on the basis that, with 95% confidence, the interval above this threshold covers at least 95% of the normal population, so the risk of classifying a score as abnormal when it is in fact normal is at most 5%. Similarly, scores >84.4% are judged to be “normal” as the interval below that threshold covers at most 95% of the abnormal population, so the risk of judging a score normal when it is in fact abnormal is at most 5%.

Cut-off scores using outer and inner one-sided nonparametric tolerance limits, N = 436, coverage β = 0.95, confidence level γ = 0.95
The nonparametric one-tailed lower 5% tolerance limits (with 95% confidence) for combined dual-task performance is 81.0%. This cut-off is then adjusted for education (see Table 3 ), to provide the corrected combined dual-task cut-off scores for the various levels of education.
Corrections to be added to the raw scores according to the level of schooling and the adjusted combined dual-task performance cut-off scores
Our aims were to establish a set of norms for a simplified version of a dual-task procedure that could be used for research and clinical purposes. The data gathered with the simpler dual-task procedure were not normally distributed. There were a few outliers considerably above the rest of the distribution, which created a positive skew and therefore the data were trimmed by two SD s. This technique removes outlier bias, but it is possible that this technique in itself gives a false impression of a ‘normal’ dual-task performance. However, the outlier analysis revealed that the excluded data points were relatively well balanced either side of the mean, suggesting that the exclusion of these would not, in itself, introduce bias.
The remaining data are normally distributed. There is an indication that the scores might be skewed toward the higher end, but this trend was not significant. Conservative non-parametric methods ( Capitani, 1997 ; Wilks, 1941 ) were used to identify cut-offs for ‘normal’ performance, which allow for some skewness in the data. Such conservative methods will limit type I errors when interpreting dual-task performance, and may prove useful in clinical practice. This paper-and-pencil assessment is simpler, easier to use, and cheaper than the existing computerized methodologies, and the test–retest reliability is sufficiently high, unlike previous assessments, making it ideally suitable for clinical use. Moreover, as the assessment is individually calibrated to each individual participant, thereby eliminating any single-task differences and only focusing on the intra-individual differences between single- and dual-task performance, the assessment also eliminates the possible measurement error by avoiding involvement of other cognitive processes, which are not the focus of interest here. Indeed, the bespoke nature of the dual-task assessment also ensures that it minimizes the effect of any cultural or educational variation. Therefore, the normative data should be widely generalizable. Although it is recognized that as it is not possible to calibrate the tracking task to individual level of ability, the dual-task assessment may be vulnerable to some single-task differences, although this was not found in this study.
The participants showed minimal reductions in performance under dual-task conditions, in either the digit recall, tracking, or the combined measure of dual-task performance. This suggests that, in general, healthy people demonstrate no difficulties in dual tasking under these testing conditions. No effect of age was detected, supporting the suggestion that the cognitive function involved in successfully coordinating the concurrent performance of two distinct tasks is unaffected by healthy aging ( Baddeley et al., 1986 ; Belleville et al., 1998 ; Salthouse et al., 1995 ).
Previous work using laboratory versions of the dual-task procedure had indicated that dual-task impairments could be signatures of AD that are not shown in other patient groups, such as chronic depression ( Kaschel et al., 2009 ) or MCI ( Foley, Kaschel, Logie, & Della Sala, submitted ), or in healthy older people ( Baddeley et al., 1986 , 1991 ; Della Sala et al., 1995 ; Holtzer et al., 2004 ; Logie et al., 2004 ; MacPherson et al., 2004 , 2007 ; Morris, 1986 ; Morris & Baddeley, 1988 ; Sebastian et al., 2006 ). This accompanies the well-established episodic memory deficit that is characteristic of AD but is not necessarily specific to the disease. This dual-task paradigm could therefore ideally complement measures of episodic memory in assessing cognitive competence in people with AD. Episodic memory tests are currently considered to be the most useful assessments to detect AD, but they are not specific to AD even if they are highly sensitive to the disease. Episodic memory impairment can be associated with a wide range of disorders and, to a certain extent, normal aging, which can lead to diagnostic uncertainty. Therefore, elements more specific to AD need to be added to improve the diagnostic utility of these tests. Moreover, as individuals with AD quickly reach ‘floor’ on episodic memory assessments, these tests are unsuitable for following up patients over time. As the dual-task assessment is adjusted to individual level of ability, this assessment can be used with patients for longer. This study has shown that the dual-task assessment is unaffected by normal aging and offers a set of norms as well as a simplified test protocol that could be used in a clinical setting. Thus, combining the sensitivity of episodic memory tests with the specificity of this dual-task assessment may prove to be particularly useful for singling out patients with AD and following up their performance over time.
This work was supported by the grant AS-90-2007 awarded by the Alzheimer's Society to SDS and RHL. JAF is the appointed Research Fellow on this grant.
None declared.
We are grateful to Dr Anna Cantagallo who assisted us in testing some of the older adult control participants.
Google Scholar
Google Preview
- alzheimer's disease
- psychometrics
- mental recall
- minimal cognitive impairment
Supplementary data
Email alerts, citing articles via.
- Recommend to your Library
Affiliations
- Online ISSN 1873-5843
- Copyright © 2024 Oxford University Press
- About Oxford Academic
- Publish journals with us
- University press partners
- What we publish
- New features
- Open access
- Institutional account management
- Rights and permissions
- Get help with access
- Accessibility
- Advertising
- Media enquiries
- Oxford University Press
- Oxford Languages
- University of Oxford
Oxford University Press is a department of the University of Oxford. It furthers the University's objective of excellence in research, scholarship, and education by publishing worldwide
- Copyright © 2024 Oxford University Press
- Cookie settings
- Cookie policy
- Privacy policy
- Legal notice
This Feature Is Available To Subscribers Only
Sign In or Create an Account
This PDF is available to Subscribers Only
For full access to this pdf, sign in to an existing account, or purchase an annual subscription.

An official website of the United States government
The .gov means it’s official. Federal government websites often end in .gov or .mil. Before sharing sensitive information, make sure you’re on a federal government site.
The site is secure. The https:// ensures that you are connecting to the official website and that any information you provide is encrypted and transmitted securely.
- Publications
- Account settings
Preview improvements coming to the PMC website in October 2024. Learn More or Try it out now .
- Advanced Search
- Journal List

The Effects of Different Types of Dual Tasking on Balance in Healthy Older Adults
Graça monteiro de barros.
1 Fisio-Lógica Centro de Fisioterapia, Lda, 1350-275 Lisboa, Portugal; moc.liamg@autnamacarg
2 Escola Superior de Saúde Atlântica, 2730-036 Barcarena, Portugal
Filipe Melo
3 Laboratório de Comportamento Motor, Faculdade de Motricidade Humana, Universidade de Lisboa, 1495-688 Cruz Quebrada, Portugal; tp.aobsilu.hmf@olemf
Josefa Domingos
4 Grupo de Patologia Médica, Nutrição e Exercício Clínico (PaMNEC) do Centro de Investigação Interdisciplinar Egas Moniz (CiiEM), Escola Superior de Saúde Egas Moniz, Caparica, 2829-511 Almada, Portugal; moc.liamg@afesojsognimod (J.D.); moc.liamg@10oleboiluj (J.B.F.)
Raul Oliveira
5 Neuromuscular Research Lab, CIPER, Faculdade de Motricidade Humana, Universidade de Lisboa, 1495-688 Cruz Quebrada, Portugal; tp.aobsilu.hmf@arievilor
Luís Silva
6 Physics Department, LIBPhys-UNL, Nova School of Science and Technology, Universidade Nova de Lisboa, Caparica, 2829-516 Almada, Portugal; [email protected]
Júlio Belo Fernandes
Catarina godinho, associated data.
The data presented in this study are available on request from the first author.
Numerous of our daily activities are performed within multitask or dual task conditions. These conditions involve the interaction of perceptual and motor processes involved in postural control. Age-related changes may negatively impact cognition and balance control. Studies identifying changes related to dual-task actions in older people are need. This study aimed to determine the effects of different types of dual-tasking on the balance control of healthy older adults. The sample included 36 community-living older adults, performing two tests—a sway test and a timed up-and-go test—in three conditions: (a) single motor task; (b) dual motor task; and (c) dual motor task with cognitive demands. Cognitive processes (dual-task and cognition) affected static balance, increasing amplitude ( p < 0.001) and frequency ( p < 0.001) of the center of mass displacements. Dynamic balance revealed significant differences between the single motor condition and the other two conditions during gait phases ( p < 0.001). The effect of dual-tasking in older adults suggests that cognitive processes are a main cause of increased variability in balance and gait when under an automatic control. During sit-to-stand, turning, and turn-to-sit movements under dual-tasking, the perceptive information becomes the most important focus of attention, while any cognitive task becomes secondary.
1. Introduction
Daily life is occupied by dual-tasking behaviors, such as walking while talking with someone or while taking a picture on the phone. Effective daily functioning requires people to share their attention resources between the cognitive and the postural requirements necessary to complete the tasks [ 1 ]. This ability to perform concurrent performances is known as dual-tasking. A decreased capacity to perform dual-tasking may reduce the person’s ability to participate in their life roles [ 2 ]. Due to the aging process and prevalence of chronic diseases, older adults show some levels of decline when performing postural tasks while dual-tasking [ 3 ]. Upholding and improving older adults’ ability to perform under dual-task situations is an imperative goal for extending their functionality.
The literature reports several factors that are considered to influence the person’s ability to divide their attention resources between the two tasks, namely external factors, such as the tasks’ complexity, and intrinsic factors, such as physical status, executive function, and task prioritization [ 4 , 5 ].
Activities such as driving or walking are considered automatic motor sequences, largely operating independently from more cognitively intensive processes such as communication. When combined into a dual-task activity, the interaction of the perceptual-motor and cognitive neurophysiologic processes may have an influence upon the postural control that has a primary support function [ 6 , 7 ]. The turning characteristics during ambulating are a major contributor to motor disability, falls, and reduced quality of life in older people, largely because the necessary (intrinsic) dynamic balance control decreases with age [ 8 ].
Previous research indicates that postural control and gait in older adults lose some level of automaticity, defined as the capacity to be independently executed with minimum attentional costs, and indicated by alterations of motor patterns during dual-task activities [ 9 , 10 ]. The degree to which postural control and gait change during the simultaneous performance of other tasks is considered related to the level of difficulty of the concurrent task [ 3 ].
Questions remain regarding the overall amount of influence of different dual-task combinations (motor–motor versus motor–cognition) on postural control and gait. Although different aspects of the influence that dual-task activities have on postural control and gait have been observed in healthy older adults [ 11 , 12 ], the factors that contribute to postural control and gait changes, in response to “dual-tasking” among this population have not been fully clarified. It is hypothesized that in a homogeneous sample of healthy older adults with no perturbation in mobility and cognitive function, postural control and gait will remain under greater automatic control and thus, dual-task decrement, although present, will remain at a reduced level.
It is of great relevance to develop a normative evaluation of the postural control during static and dynamic equilibrium conditions [ 13 ]. This evaluation should include the performance of single and dual-tasks in order to determine which parameters may be altered with aging.
Postural and gait analysis obtained during the execution of different motor tasks will allow for the understanding of the interaction between motor and cognitive capacity, as well as the effects upon healthy aging subjects [ 14 ].
The objective of this study was to evaluate the effects of dual-task activities on balance control in healthy older individuals, namely, by exploring its influence on the temporal and space parameters of static activities such as postural sway in addition to dynamic tasks (standing and sitting on a chair and walking in conjunction to rotational movements) while performing a balance control task such as carrying a tray with a full glass of water.
2. Materials and Methods
2.1. study design.
This investigation was based on an observational cross-sectional study with a single moment evaluation.
2.2. Sampling and Recruitment
Healthy older adults were recruited from the community by advertisements on social media and at seniors’ centers by phone or in person. The sampling method selection was non-probabilistic by convenience.
Subjects were included if they were seniors—aged 65 years and over, independent in their daily activities, and presenting independent walking.
Subjects were excluded if they presented vestibular disorders, neurological diseases, lack of cognitive skills according to the results of Mini Mental Score (MMS) < 20, musculoskeletal impairments that could affect gait, and inability to stand and walk unassisted.
2.3. Ethics and Procedures
This study follows the principles of the Declaration of Helsinki. All participants provided written informed consent and the study was approved by the Ethics Council of the Faculty of Human Kinetics (ID: CEFMH N°4/2016 in 15 February 2016).
2.4. Data Collection
Subjects were asked to perform two different tests randomly—the instrumented sway test (ISWAY) and the instrumented timed up-and-go test (ITUG)—during three task conditions that were applied randomly: (a) single motor task; (b) dual motor task (single motor task carrying a tray with a full glass of water); and (c) dual motor task with cognitive demands (the same as the dual motor task including counting back from 100, three by three). During the ISWAY test, subjects had to maintain a stable standing position.
Balance and gait were measured using four Opal inertial sensors and automated algorithms from Mobility Lab, by APDM (APDM Inc., Portland, OR, USA). Sensors were placed on both ankles, the chest, and the posterior trunk at the level of L5 (Center of Mass—CM), with elastic Velcro bands sufficiently stable to avoid any undesirable dress movement ( Figure 1 ).

Placement of the Opal sensors and tray with a glass of water.
Inertial sensor data was collected and wirelessly streamed to a laptop for automatic generation of gait and balance metrics provided in specific reports by the Mobility Lab software. In the ISWAY test, subjects were asked to stand quietly for 30 s. In the ITUG test, subjects had to stand up from a chair without using their arms, walk 7 m, turn around to walk back to the chair, and sit down. Subjects repeated the ITUG test three times, once for each test condition (single motor task, dual motor task and, dual motor task with cognitive demands). Before the tests, each subject was submitted to a familiarization trial.
2.5. Data Analysis
The APDM automated analysis algorithm identified the standing position of the ISWAY test as well as the sit-to-stand, gait, turning and turn-to-sit components/phases of the ITUG test, providing a total of 52 spatial-temporal metrics [ 15 ]. From this automatic analysis, we monitored the following measures: Postural Sway (ISWAY test)—Forward/Backward and Right/Left amplitude (cm), Frequency (Hz), and Ellipse Sway area (m 2 /s 4 ); ITUG: Sit-to-Stand—Time duration (s), Peak velocity ( 0 /s), and Trunk range of movement (RoM) ( 0 ); Gait—Duration (s), Stride length (% of height), Cadence (steps/min), Stride velocity (% of height/s), Double support time (% of total gait cycle duration), Swing phase (% of total gait cycle duration), and Stance phase (% of total gait cycle duration); Turning—Duration (s), Number of steps (n°), Turn Peak velocity ( 0 /s), and Step duration during turning (s); Turn-to-Sit—Duration (s), Turn Peak velocity ( 0 /s), and Trunk range of movement (RoM) ( 0 ).
Data results were subjected to analysis to identify the presence of any outliers. The assumption of normality was verified by Kolmogorov–Smirnov test. Kurtosis and skewness of the distributions were also analyzed. A repeated measures ANOVA was used for comparisons among the three conditions—(1) single motor task, (2) dual motor task, and (3) dual motor task with cognitive demands.
Sphericity was verified by Mauchly’s test, and when this assumption could not be accepted, this parameter was corrected through the Greenhouse–Geisser Epsilon. Significant differences in ANOVA led to multiple comparisons with Bonferroni adjustment. Friedman’s test was applied when the normality assumption was not verified, and in case of significant differences, Dunn’s multiple comparisons were applied. The level of significance was set at 0.05. Data analysis included kinematic parameters during the two different tests (ISWAY and ITUG) during the three different conditions (single motor task, dual motor task, and dual motor task with cognitive demands).
3.1. ISWAY Test
Thirty-six healthy older adults (9 men and 27 women, 73 ± 5.7 years) were recruited from the community. All participants consistently altered their static balance and gait pattern in response to additional dual-task load, although specific kinematic changes varied according to task conditions. Postural control in standing position, measured via the ISWAY, was only affected during the dual motor task with cognitive demands, due to the increased cognitive complexity when compared with the other two conditions (single task and dual motor task). There were no differences in kinematic data between the other two motor tasks (single and dual motor tasks). Specifically, instrumental assessments related to the ISWAY test showed an increase in the postural sway parameters of the center of mass (CM) movement in terms of forward–backward ( χ 2 (2) = 19.385; p < 0.001; N = 26), and right–left (𝐹 (2,50) = 18.956; p < 0.001) displacement amplitude, forward–backward ( χ 2 (2) = 20.846; p < 0.001; N = 26) and right–left ( χ 2 (2) = 22.020; p < 0.001; N = 26) displacement frequency, and ellipse sway area (𝐹 (1.488, 37.194) = 27.361; p < 0.001). Figure 2 shows significant differences in pairwise comparisons for ISWAY test parameters and the respective boxplots.

Balance parameters for the three conditions: single task, dual task, and dual and cognitive task during the ISWAY test. * Significant differences for pairwise comparisons.
3.2. ITUG Test
The dynamic balance analyzed during the ITUG test revealed highly significant differences ( p < 0.001) between the single motor condition and the other two dual task conditions (dual motor task and dual motor task with cognitive demands) in the kinematic data of the ITUG test different phases.
3.2.1. Sit-to-Stand Phase
With respect to time duration, there were no significant differences among the different test conditions, but there were highly significant ( p < 0.001) differences in peak velocity ( χ 2 (2) = 46.545; N = 33) and trunk range of movement (RoM) ( F (2,64) = 41,861; p < 0.001) between the single motor task and the other two tasks (dual motor task and dual motor task with cognitive demands) although there were no statistical significant differences between the two dual-task activities.
Figure 3 shows significant differences in pairwise comparisons for sit-to-stand parameters and the respective boxplots.

Sit-to-stand parameters for the three conditions: single task, dual task, and dual and cognitive task during the ITUG test. * Significant differences for pairwise comparisons.
3.2.2. Gait Phase
Significant differences in time duration were observed among all three conditions ( F (1.681, 58.831) = 61,377; p < 0.001). Since there were significant differences between all conditions, showing that the gait duration increases as the level of complexity of the task also increases.
Stride length revealed significant differences among all conditions ( F (2, 70) = 39.375; p < 0.001), again with the reduction in stride length relative to the level of complexity of the task.
The cadence parameter demonstrated significant differences between the dual motor task with cognitive demands condition and the other two conditions (single motor task and dual motor task) ( F (1.408, 49.281) = 41.767; <0.001). There were no significant differences between the other two conditions.
Stride velocity showed significant differences among all task conditions ( F (1.590, 55.665) = 54.459; p < 0.001), with a reduction of the results related to the level of complexity of the task.
Double support time revealed significant differences between the single motor task and the dual motor task with cognitive demands condition ( χ 2 (2) = 44.169; p < 0.001; N = 36). No significant differences were observed between the other two conditions.
Similarly, assessment of swing phase only demonstrated significant differences between the single motor task and the dual motor task with cognitive demands condition ( F (1.672, 58.529) = 48.018; p < 0.001), showing a marked reduction in swing time. No significant differences were found between the other two conditions.
Stance phase showed significant differences between the single motor task and the dual motor task compared to the dual motor task with cognitive demands condition ( χ 2 (2) = 43.504; p < 0.001; N = 36). No significant differences were observed between the other two conditions.
Figure 4 shows significant differences in pairwise comparisons for gait parameters and the respective boxplots.

Gait parameters for the three conditions: single task, dual task, and dual and cognitive task during the ITUG test. * Significant differences for pairwise comparisons.
3.2.3. Turning Phase
Turning duration showed significant differences between all the conditions ( F (2,64) = 87.413; p < 0.001), with an increase in time corresponding to the level of complexity of the task. For all other turning-related assessments, there was a consistent pattern of statistically significant changes when comparing the single motor task to the dual motor task activity but no statistically significant changes when comparing the dual motor task with the dual motor task with cognitive demands conditions.
The number of steps revealed significant differences between the different conditions ( χ 2 (2) = 42.365; p < 0.001; N = 32), with the single motor task condition presenting smaller values in relation to the other two dual motor task conditions. No statistically significant differences in the number of steps were identified during the two dual motor task conditions themselves ( Figure 5 ).

Turning parameters for the three conditions: single task, dual task, and dual and cognitive task during the ITUG test. * Significant differences for pairwise comparisons.
Similarly, highly significant differences in peak velocity were identified comparing the single motor task to both other dual motor task conditions ( F (1.349, 41834) = 72.817; p < 0.001). There were no statistically significant differences observed between the two dual motor task conditions.
Figure 5 shows significant differences in pairwise comparisons for turning parameters and the respective boxplots.
3.2.4. Turn-to-Sit Phase
Turn-to-sit duration revealed also significant differences only between the single motor task and the two dual motor tasks conditions ( χ 2 (2) = 33.515; p < 0.001; N = 33), with no statistically significant differences between the two dual motor task conditions ( Figure 6 ).

Turn-to-sit parameters for the three conditions: single task, dual task, and dual and cognitive task during the ITUG test. * Significant differences for pairwise comparisons.
Turn peak velocity revealed significant differences between the single motor task condition and both other dual motor task conditions, ( F (2,64) = 64.977, p < 0.001 and F (1.696, 54.267) = 49.739, p < 0.001, respectively). There were no significant differences between either of the dual motor task conditions.
Trunk range of movement revealed significant differences between the different conditions (𝐹 (1.696, 54.267) = 49.739; p < 0.001) with the single motor task condition being responsible for these differences, showing a smaller trunk amplitude related to the other two conditions which did not show significant differences.
Figure 6 shows significant differences in pairwise comparisons for turn-to-sit parameters and the respective boxplots.
4. Discussion
The process of ageing is associated with functional decline that affects the speed of the executive function processes, which are the main neurocognitive processes for the proper development of daily life activities [ 16 ]. Simple actions including walking while talking require people to divide attention between the two tasks. When cognitive functions deteriorate and processing two actions becomes difficult, older people are placed at risk, and independence is compromised [ 17 ]. Healthy adults have the ability to develop dual-task activities; however, as a result of the ageing process and/or stages of neurological disorders, the ability to perform multiple tasks can be affected [ 1 , 7 ]. Consequently, preserving and improving a person’s walking ability during other daily life activities is a crucial aim for extending functionality.
This study revealed that a change in walking performance was observed with the increasing path complexity and under dual-task conditions. Recent studies also showed that performing two tasks or dividing one’s attention between tasks can result in the decrease of walking performance not only in healthy adults [ 9 ] but also in people with neurological pathologies [ 7 , 18 ].
Consistently, dual motor tasks with cognitive demands resulted in the most instances of statistically significant differences between the two types of dual-task activity identified while monitoring static balance and gait. The demands of a single motor task highlighted statistically significant differences when monitoring more complex movements like sit-to-stand, turning, and turn-to-sit, as well as during tasks involved in changes in movement direction or height control. These types of actions are associated with girdle dissociative control, which, in our sample of healthy older adults, is constrained by a counter-control strategy based on a pelvic and scapular girdle blockage.
Regarding the different conditions and tasks involved, we may consider that standing and walking are motor actions that can be performed under an automatic control, mediated by subcortical structures, releasing our attention to concurrent tasks needing greater engagement like in cognitive operations.
Previous studies have also shown how older adults under dual task testing contexts show a reduced gait velocity, cadence, and stride time variability [ 11 , 12 ]. Our study is in line with previous research showing that gait performance change deteriorates, and instability increases when a cognitive load is augmented. These results have clinical impact, since low performance in dual-task activities is associated with an increased risk for dementia [ 19 , 20 ], falls, and functional decline [ 21 , 22 ]. Furthermore, in older adults low gait speed is a significant clinical parameter associated with functional decline, falls, morbidity, and survival [ 23 , 24 ]. Significant improvements in the standard walking speed are related with increased subsistence in community dwelling [ 24 ].
Turning requires dynamic balance control, which often deteriorates with age. Our results suggest that cognitive processes are highly involved in the increased variability identified during tasks where movement is under an automatic control (like in standing or walking) in our sample composed of healthy older adults. However, during more complex activities such as sit-to-stand, turning and turn-to-sit, where the efficiency and control of the secondary motor task (carrying a tray with a full glass of water) implies a need for additional attention, the primary focus appears to be upon the perceptive information and any cognitive task becomes secondary. Nevertheless, motor actions that require the manipulation and control of sliding objects, or changes in body movement direction or heights, may involve a greater need for attention in order to control and accomplish the intended task. When evaluating the performances of the balance tests, also measuring the accuracy of the given additional task may produce more objective data.
Overall, the study results revealed that there was an adaptation in the motor control of the different tasks, and this should be contemplated in active aging programs of physical activity in order to maintain the effectiveness of the different perceptual-motor processes. The promoting training incorporating neuroplasticity principles, must be considered so that the performance capacity to perform dual tasks can be improved [ 25 ]. Using frequent and varied repetitions of different specific exercises is necessary for improving or maintaining performance [ 26 , 27 ].
5. Conclusions
The results from this study show that dual-tasking influences balance behavior in healthy older adults. By increasing the task complexity with an additional cognitive task, we change balance control. Healthy older adults are prone to focus on the challenging dual motor task or dual motor task with cognitive demands and sacrifice balance performance to some extent.
Further investigations should test the effects of dual task training protocols in older adults’ balance and walking speed. The application of dual task training appears to be promising to improve the use of these parameters. Furthermore, longitudinal studies that assess the detraining effects are also needed. Therefore, we recommend that this assessment should be carried out in future studies and highlight the need to compare older populations with healthy young and adult subjects.
Acknowledgments
This work is financed by national funds through the FCT—Foundation for Science and Technology, I.P., under the project UIDB/04585/2020. The researchers would like to thank the Centro de Investigação Interdisciplinar Egas Moniz (CiiEM) for the support provided for the publication of this article.
Author Contributions
Conceptualization; Formal analysis; Investigation; Methodology; Writing and Reviewing, G.M.d.B.; Conceptualization; Formal analysis; Investigation; Methodology; Writing and Reviewing; writing—original draft preparation; Project administration, F.M.; Conceptualization; Data curation; Formal analysis; Investigation; Methodology; Writing—Reviewing and Editing, J.D.; Formal analysis; Investigation; Methodology; Writing and Reviewing, R.O.; Methodology; Formal analysis; Writing and Reviewing, L.S.; Methodology; Writing—Reviewing and Editing, J.B.F.; Conceptualization; Formal analysis; Project administration; Writing—Reviewing and Editing, C.G. All authors have read and agreed to the published version of the manuscript.
This research did not receive any specific grant from funding agencies in the public, commercial, or not-for-profit sectors.
Institutional Review Board Statement
The study was conducted according to the guidelines of the Declaration of Helsinki, and approved by the Institutional Review Board and Ethics Committee of Faculty of Human Kinetics (ID: CEFMH N°4/2016 in 15 February 2016).
Informed Consent Statement
Informed consent was obtained from all subjects involved in the study.
Data Availability Statement
Conflicts of interest.
The authors declare that they have no conflict of interests.
Publisher’s Note: MDPI stays neutral with regard to jurisdictional claims in published maps and institutional affiliations.
- UB Directory
- Initiative for Plastics Recycling Research and Innovation >
- Research >
- Ongoing Research Tasks >
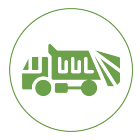
Improve the efficiency of businesses involved in collecting and processing plastics in New York State
Goals/objectives.
- Assess and improve plastic recovery efficiency (i.e., efficiency in sorting plastic from everything else in the material coming in), and sorting efficiency (i.e., how efficiently (or cleanly) recovered plastic is separated into different grades of plastic) in New York State.
- Assess the productivity of material recovery facilities (MRFs) in NYS, an integral part of the reverse supply chain, and empirically analyze how firm characteristics like economies of scale and economies of scope, and socioeconomic factors like residential Supplemental Nutrition Assistance Program (SNAP) use and education levels influence their recycling performance.
- Conduct a systematic evaluation of recycling efficiency of Material recycling facilities (MRFs) by measuring recycling efficiency of MRFs under framework of data envelopment analysis (DEA), which is an issue of significant interest in a circular economy.
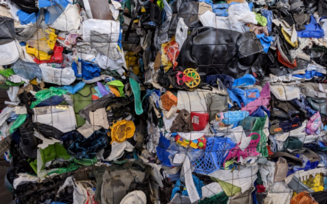
- Material Recovery Facilities (MRFS): divert between 25% to 75% waste from landfills (Kessler, 2009) and produces nine times lower greenhouse gas (GHG) emissions than alternatives (Möck et al., (2022).
- Challenges: high contamination rates and processing costs, low participation rates, and volatile commodity prices (Townsend et al., 2022). Lack of accessible and reliable data on recycling processes and outputs.
- Objective: Investigate key internal and external drivers that influence the volume of materials recovered by MRFS for recycling and improve their productivity.
Methodology used to perform the task:
- An econometric analysis utilizing a panel dataset comprising 91 MRFs operating in New York State (NYS) during the years 2016–2020, resulting in 361 records was used.
- A fixed effects panel regression analysis in which the intercept of the regression model is permitted to differ between individuals or groups was conducted to evaluate the model.
- Tests including the Hausman Test justified the use of a fixed effects regression model as compared to a pooled OLS or random effects regression model. The model was also assessed for the presence of cross-sectional dependence, serial correlation, and heteroskedasticity.
- Survey methods including questionnaires via email was also sent to MRFs in NYS requesting information about the sorting equipment used at their facilities.
- Analytical research to provide a novel mathematical model under Data Envelopment analysis (DEA) to measure recycling efficiency of MRFs.
Task Team Members
- Aditya Vedantam , Associate of Operations Management, School of Management, University at Buffalo
- Nallan Suresh , Associate Dean for Faculty, Research and Centers, UB Distinguished Professor, School of Management, University at Buffalo
- Nima Alizadeh Basban , PhD student, School of Management, University at Buffalo
Results/Preliminary Results
- MRF’s with greater economies of scale and economies of scope recover significantly higher volumes of recyclable materials in NYS.
- The onset of China’s National Sword policy was shown to significantly reduce the volume of materials recovered by MRFs in NYS.
- Interestingly, the positive impact of economies of scope/material variety on recycling output was higher after the implementation of China’s National Sword policy signaling diversification benefits.
- Residents with a high education level contribute positively towards volumes recovered by MRFs, whereas residents with a low education level contribute negatively towards volumes recovered by MRFs.
- There is a positive and significant effect of SNAP benefits use and the amount recovered by MRFs for recycling.
- The formulation of a new mathematical model to measure the recycling efficiency of MRFs in New York State using Slack Based Models (SBM), an advanced DEA approach.
- Calibrated the contributed SBM model with data from MRFs in New York State
Publications and Presentations
Publications.
Two working papers are targeted for submission for peer review:
- A novel approach to measure the recycling efficiency of material recycling facilities (MRFs) in New York State
- Uncovering the Drivers Influencing the Recycling Performance of Material Recovery Facilities
Conference Presentations
- Ajmal et al., (2024). Exploring the Factors Impacting Recycling Performance of Material Recovery Facilities. 34th Annual POMS Conference. April 2024 (Forthcoming).
- Uncovering the Drivers Influencing the Recycling Performance of Material Recovery Facilities, Federation of New York's Solid Waste/Recycling Conference & Trade Show at The Sagamore, Bolton Landing, NY, May 21 - 24, 2023
- Recycling efficiency of material recycling facilities in New York State using data envelopment analysis (DEA) approach. 2024 MIDWEST Decision Science Institute Annual Conference. (Will be presented at the Williamson College of Business Administration at Youngstown State University, OH, USA, 12th-13th April 2024)
Ongoing/Future Work
- As part of future work, we will study hub and spoke based network design models will be investigated for efficient recovery of plastic waste, particularly for rural areas in New York State.
- We will investigate secondary sortation-based business models for plastic recovery in New York State in collaboration with industry partners (e.g., Titus MRF Services).
- Stakeholder interviews will be conducted to explore why PRFs have failed in the past and what needs to be done differently for them to be successful in the future).
Task Alumni
- Dr. Khadija Ajmal , Assistant Professor of Transportation & Logistics, University of Wisconsin Superior

IMAGES
VIDEO
COMMENTS
To pursue the aim of looking for mechanisms of improved dual-task performance with practice, the present paper evaluates the robustness of one specific phenomenon: the dual-task practice advantage (DTPA), an idea going back to the empirical findings of Hirst, Spelke, Reaves, Caharack, and Neisser as well as to the theoretical assumptions of Damos and Wickens (): "[single-task skills] fail ...
Task switching paradigms, theories, and executive functioning. Task switching refers to a multitasking situation where two or more tasks are presented sequentially without temporal overlap (e.g., Monsell, 2003; Kiesel et al., 2010).Contrasting with most of the studies on dual-tasking, the stimuli presented in task switching situations afford not only the currently relevant task but also the ...
While the previous dual-task training literature has regularly shown that training on traditional multitasking paradigms leads to task-specific reductions in dual-task costs, 3,7,8,9,10,11 it is ...
Dual-task performance ostensibly requires the central executive because of the need for coordination (although this must be demonstrated empirically for any given pair of tasks, and not all studies have done this). ... such as using a device to delay the presentation of words in a word recall task and use of a handrail during a walking task ...
The aim of this study was to examine the impact of predictability on dual-task performance by systematically manipulating predictability in either one of two tasks, as well as between tasks. According to capacity-sharing accounts of multitasking, assuming a general pool of resources two tasks can draw upon, predictability should reduce the need for resources and allow more resources to be used ...
In addition, monkey A's oculomotor behavior following memory cue presentation did not show signs of dual-task interference, whereas monkey S's oculomotor behavior in the same epoch clearly ...
Many studies have applied the dual-task paradigm to examine if walking is automatic or if it demands attention (Woollacott and Shumway-Cook, 2002; Yogev-Seligmann et al., 2008).Among older adults and patients with neurologic or other disease, many aspects of gait typically deteriorate when subjects are asked to walk and perform another task at the same time (Springer et al., 2006; Yogev ...
For example, many dual-task neuroimaging studies associate prefrontal and parietal regions for dual-task processing (for a review, see Marois and Ivanoff, 2005), however others do not (e.g., Jiang et al., 2004) and Nijboer et al. (2014) suggest there is no specific region associated with dual-task processing, rather dual-task interference is ...
Use dual task assessment to measure key aspects of the motor and/or cognitive task performance in single and dual-task conditions. a. Be sure that single tasks have clear objective measures b. Use more than one combination of tasks 4. Determine the relationship between outcomes and
We suggest that the dual-task combination of multisensory cognitive stimulation and multimodal moderate physical exercise training, twice a week, may be adopted as an effective program to reduce progression of age-related cognitive decline and improve physical fitness and quality of life on healthy older adults.
The participants performed two tasks: a single task (ST) and a dual task (DT) consisting of a simple dual task (S-DT) or complex dual task (C-DT) (Fig. 1). The ST and DT (S-DT and C-DT) were defined as low-, medium-, and high-load divided attention tasks, respectively. The paradigm used here is similar to that employed in previous studies 9, 10 ...
The dual-presentation timing (2PT) task and its variants. The only common feature in all variants of the 2PT task is the defining characteristic that each trial presents two stimulus pairs, each one analogous to those used in SPT tasks but differing in the SOA with which the members of each pair are presented. Presentations occur in consecutive ...
This article discusses the concept of dual-tasking and multitasking. Dual-tasking is the ability to perform two tasks simultaneously. Dual-tasking measures a component of executive function as participants are required to coordinate their attention to both tasks while they are being performed. Dual tasking is differentiated from serial multitasking paradigms where individuals focus on and ...
In other words, participants either perform one task or two tasks per block. Dual-task performance costs are reflected in worse performance in dual- compared with the single-task conditions (e ... Rogers and Monsell, 1995) or with a random task sequence and a task cue that precedes or accompanies stimulus presentation (i.e., task cueing ...
Identify target areas for your patient and select tasks that a relevant. Recognize the goals and constraints of movement. Measure motor and/or cognitive task performance both for single and dual task conditions. Determine the relationship between clinical deficits and outcomes for interventions.
Introduction: With aging, dual task (DT) ability declines and is more cognitively demanding than single tasks. Rapidly declining DT performance is regarded as a predictor of neurodegenerative disease. Task training and non-invasive transcranial electrical stimulation (tES) are methods applied to optimize the DT ability of the elderly. Methods: A systematic search was carried out in the PUBMED ...
Introduction. Dual-tasking is the ability to perform two tasks concurrently. Dual-task paradigms have been instrumental in forging cognitive models of attentional control (Fernandes & Moscovitch, 2000; Naveh-Benjamin, Craik, Guez & Kreuger, 2005), and in investigations of deficits in attentional control following focal and diffuse brain damage, including neurodegenerative disease (Baddeley ...
Now, the SRTT followed the 8-element sequence, each block starting at a randomly drawn sequence position. A dual-task trial began with the presentation of the visual SRTT target (the "X") at one of the four different target locations and, simultaneously, one of the two different auditory stimuli of the tone-discrimination task.
Study authors conclude, "Children with ADHD do not demonstrate deficits in dual-task performance of a texting and walking task indoors or outdoors.". They suggest that a more personalize ...
Plastic pollution is one of the biggest problems to tackle in the 21 st century since plastic production has increased from 2.3 million tons in 1950 to 448 million tons in 2015 and is expected to double that by 2050. It is estimated that one ton of recycled plastic saves 5.7MWh of electricity, 685 gallons of oil, and 30 cubic yards of landfill ...
A dual task was designed to involve a tracking mission with various tracking speeds and a spatial compatibility task with various signal-key mappings and presentation modalities. This dual task was used to investigate the effects of workload and resource competition in distinct parts of the dual-task process. The results demonstrated that ...
Collect multiple rounds of survey data to identify key areas of misperception about recycling and reuse. Analyze the quantitative and qualitative responses to the PSA video designed to identify pathways for further improvement. Produce a report for the general public that includes procedural information (i.e., how to recycle properly) (Year 1).
Abstract. Considerable attention has recently focused on the role of dual-task exercises (DT) in the older adult. The aim was to conduct a review to describe the dual-task exercises that have been shown to be effective in improving balance and other physical characteristics such as decreased falling and walking speed in older adults.
Air pollution is one of the main risk factors for noncommunicable diseases. In the WHO European Region, tackling air pollution is a regional priority to improve health. Reducing the emissions of certain pollutants has also double benefits for health, since it helps to mitigate climate change.At its annual meeting, the Joint Convention/WHO Task Force on the Health Aspects of Long-range ...
Atkinson, J.D. "Microplastics Associated with Cigarette Waste" Public Health Law Center, October 2023, Invited Keynote Presentation, Zoom (160 attendees). Atkinson, J.D. and Smist, B. "Microplastics in the Natural Environment" NYS Department of Environmental Conservation - Microplastics Working Group, Invited Speaker, October 2022.
Dual task training aims to improve the ability to do two or more things simultaneously and thus reduce the risk of falling. One can differentiate between 1. dual task training with a cognitive dual task, and 2. dual task training with a manual dual task. Dual task training consists of a primary task and an additional secondary task.
Task presentation and data recording were run on an Intel Core i5 processor with a Windows 7 enterprise platform using custom code created in the Processing language ... except in the tracking word presentation dual-task without a warning signal in which a single participant missed reading aloud 3 of the words. Any differences in word recall ...
This study aimed to determine the effects of different types of dual-tasking on the balance control of healthy older adults. The sample included 36 community-living older adults, performing two tests—a sway test and a timed up-and-go test—in three conditions: (a) single motor task; (b) dual motor task; and (c) dual motor task with cognitive ...
There is a positive and significant effect of SNAP benefits use and the amount recovered by MRFs for recycling. The formulation of a new mathematical model to measure the recycling efficiency of MRFs in New York State using Slack Based Models (SBM), an advanced DEA approach. Calibrated the contributed SBM model with data from MRFs in New York ...