Appointments at Mayo Clinic
Stem cells: what they are and what they do.
Stem cells offer promise for new medical treatments. Learn about stem cell types, current and possible uses, and the state of research and practice.
You've heard about stem cells in the news, and perhaps you've wondered if they might help you or a loved one with a serious disease. Here are some answers to frequently asked questions about stem cells.

What are stem cells?
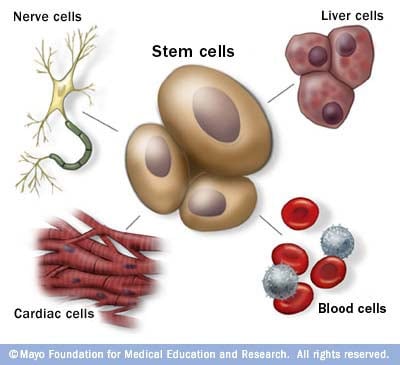
Stem cells: The body's master cells
Stem cells are the body's master cells. All other cells arise from stem cells, including blood cells, nerve cells and other cells.
Stem cells are a special type of cells that have two important properties. They are able to make more cells like themselves. That is, they self-renew. And they can become other cells that do different things in a process known as differentiation. Stem cells are found in almost all tissues of the body. And they are needed for the maintenance of tissue as well as for repair after injury.
Depending on where the stem cells are, they can develop into different tissues. For example, hematopoietic stem cells reside in the bone marrow and can produce all the cells that function in the blood. Stem cells also can become brain cells, heart muscle cells, bone cells or other cell types.
There are various types of stem cells. Embryonic stem cells are the most versatile since they can develop into all the cells of the developing fetus. The majority of stem cells in the body have fewer abilities to give rise to cells and may only help maintain and repair the tissues and organs in which they reside.
No other cell in the body has the natural ability to generate new cell types.
Why is there such an interest in stem cells?
Researchers are studying stem cells to see if they can help to:
- Increase understanding of how diseases occur. By watching stem cells mature into cells in bones, heart muscle, nerves, and other organs and tissue, researchers may better understand how diseases and conditions develop.
Generate healthy cells to replace cells affected by disease (regenerative medicine). Stem cells can be guided into becoming specific cells that can be used in people to regenerate and repair tissues that have been damaged or affected by disease.
People who might benefit from stem cell therapies include those with leukemia, Hodgkin disease, non-Hodgkin lymphoma and some solid tumor cancers. Stem cell therapies also might benefit people who have aplastic anemia, immunodeficiencies and inherited conditions of metabolism.
Stem cells are being studied to treat type 1 diabetes, Parkinson's disease, amyotrophic lateral sclerosis, heart failure, osteoarthritis and other conditions.
Stem cells may have the potential to be grown to become new tissue for use in transplant and regenerative medicine. Researchers continue to advance the knowledge on stem cells and their applications in transplant and regenerative medicine.
Test new drugs for safety and effectiveness. Before giving drugs in development to people, researchers can use some types of stem cells to test the drugs for safety and quality. This type of testing may help assess drugs in development for toxicity to the heart.
New areas of study include the effectiveness of using human stem cells that have been programmed into tissue-specific cells to test new drugs. For the testing of new drugs to be accurate, the cells must be programmed to acquire properties of the type of cells targeted by the drug. Techniques to program cells into specific cells are under study.
Where do stem cells come from?
There are several sources of stem cells:
Embryonic stem cells. These stem cells come from embryos that are 3 to 5 days old. At this stage, an embryo is called a blastocyst and has about 150 cells.
These are pluripotent (ploo-RIP-uh-tunt) stem cells, meaning they can divide into more stem cells or can become any type of cell in the body. This allows embryonic stem cells to be used to regenerate or repair diseased tissue and organs.
- Adult stem cells. These stem cells are found in small numbers in most adult tissues, such as bone marrow or fat. Compared with embryonic stem cells, adult stem cells have a more limited ability to give rise to various cells of the body.
Adult cells altered to have properties of embryonic stem cells. Scientists have transformed regular adult cells into stem cells using genetic reprogramming. By altering the genes in the adult cells, researchers can make the cells act similarly to embryonic stem cells. These cells are called induced pluripotent stem cells (iPSCs).
This new technique may allow use of reprogrammed cells instead of embryonic stem cells and prevent immune system rejection of the new stem cells. However, scientists don't yet know whether using altered adult cells will cause adverse effects in humans.
Researchers have been able to take regular connective tissue cells and reprogram them to become functional heart cells. In studies, animals with heart failure that were injected with new heart cells had better heart function and survival time.
Perinatal stem cells. Researchers have discovered stem cells in amniotic fluid as well as umbilical cord blood. These stem cells can change into specialized cells.
Amniotic fluid fills the sac that surrounds and protects a developing fetus in the uterus. Researchers have identified stem cells in samples of amniotic fluid drawn from pregnant women for testing or treatment — a procedure called amniocentesis.
Why is there controversy about using embryonic stem cells?
The National Institutes of Health created guidelines for human stem cell research in 2009. The guidelines define embryonic stem cells and how they may be used in research and include recommendations for the donation of embryonic stem cells. Also, the guidelines state that embryonic stem cells from embryos created by in vitro fertilization can be used only when the embryo is no longer needed.
Where do these embryos come from?
The embryos being used in embryonic stem cell research come from eggs that were fertilized at in vitro fertilization clinics but never implanted in women's uteruses. The stem cells are donated with informed consent from donors. The stem cells can live and grow in special solutions in test tubes or petri dishes in laboratories.
Why can't researchers use adult stem cells instead?
Progress in cell reprogramming and the formation of iPSCs has greatly enhanced research in this field. However, reprogramming is an inefficient process. When possible, iPSCs are used instead of embryonic stem cells since this avoids the ethical issues about use of embryonic stem cells that may be morally objectionable for some people.
Although research into adult stem cells is promising, adult stem cells may not be as versatile and durable as are embryonic stem cells. Adult stem cells may not be able to be manipulated to produce all cell types, which limits how adult stem cells can be used to treat diseases.
Adult stem cells are also more likely to contain irregularities due to environmental hazards, such as toxins, or from errors acquired by the cells during replication. However, researchers have found that adult stem cells are more adaptable than was first thought.
What are stem cell lines, and why do researchers want to use them?
A stem cell line is a group of cells that all descend from a single original stem cell and are grown in a lab. Cells in a stem cell line keep growing but don't become specialized cells. Ideally, they remain free of genetic defects and continue to create more stem cells. Clusters of cells can be taken from a stem cell line and frozen for storage or shared with other researchers.
What is stem cell therapy (regenerative medicine), and how does it work?
Stem cell therapy, also known as regenerative medicine, promotes the repair response of diseased, dysfunctional or injured tissue using stem cells or their derivatives. It is the next chapter in organ transplantation and uses cells instead of donor organs, which are limited in supply.
Researchers grow stem cells in a lab. These stem cells are manipulated to specialize into specific types of cells, such as heart muscle cells, blood cells or nerve cells.
The specialized cells can then be implanted into a person. For example, if the person has heart disease, the cells could be injected into the heart muscle. The healthy transplanted heart muscle cells could then contribute to repairing the injured heart muscle.
Researchers have already shown that adult bone marrow cells guided to become heart-like cells can repair heart tissue in people, and more research is ongoing.
Have stem cells already been used to treat diseases?
Yes. Doctors have performed stem cell transplants, also known as bone marrow transplants, for many decades. In hematopoietic stem cell transplants, stem cells replace cells damaged by chemotherapy or disease or serve as a way for the donor's immune system to fight some types of cancer and blood-related diseases. Leukemia, lymphoma, neuroblastoma and multiple myeloma often are treated this way. These transplants use adult stem cells or umbilical cord blood.
Researchers are testing adult stem cells to treat other conditions, including some degenerative diseases such as heart failure.
What are the potential problems with using embryonic stem cells in humans?
For embryonic stem cells to be useful, researchers must be certain that the stem cells will differentiate into the specific cell types desired.
Researchers have discovered ways to direct stem cells to become specific types of cells, such as directing embryonic stem cells to become heart cells. Research is ongoing in this area.
Embryonic stem cells also can grow irregularly or specialize in different cell types spontaneously. Researchers are studying how to control the growth and development of embryonic stem cells.
Embryonic stem cells also might trigger an immune response in which the recipient's body attacks the stem cells as foreign invaders, or the stem cells might simply fail to function as expected, with unknown consequences. Researchers continue to study how to avoid these possible complications.
What is therapeutic cloning, and what benefits might it offer?
Therapeutic cloning, also called somatic cell nuclear transfer, is a way to create versatile stem cells independent of fertilized eggs. In this technique, the nucleus is removed from an unfertilized egg. This nucleus contains the genetic material. The nucleus also is removed from the cell of a donor.
This donor nucleus is then injected into the egg, replacing the nucleus that was removed, in a process called nuclear transfer. The egg is allowed to divide and soon forms a blastocyst. This process creates a line of stem cells that is genetically identical to the donor's cells — in essence, a clone.
Some researchers believe that stem cells derived from therapeutic cloning may offer benefits over those from fertilized eggs because cloned cells are less likely to be rejected once transplanted back into the donor. And it may allow researchers to see exactly how a disease develops.
Has therapeutic cloning in people been successful?
No. Researchers haven't been able to successfully perform therapeutic cloning with humans despite success in a number of other species.
Researchers continue to study the potential of therapeutic cloning in people.
There is a problem with information submitted for this request. Review/update the information highlighted below and resubmit the form.
From Mayo Clinic to your inbox
Sign up for free and stay up to date on research advancements, health tips, current health topics, and expertise on managing health. Click here for an email preview.
Error Email field is required
Error Include a valid email address
To provide you with the most relevant and helpful information, and understand which information is beneficial, we may combine your email and website usage information with other information we have about you. If you are a Mayo Clinic patient, this could include protected health information. If we combine this information with your protected health information, we will treat all of that information as protected health information and will only use or disclose that information as set forth in our notice of privacy practices. You may opt-out of email communications at any time by clicking on the unsubscribe link in the e-mail.
Thank you for subscribing!
You'll soon start receiving the latest Mayo Clinic health information you requested in your inbox.
Sorry something went wrong with your subscription
Please, try again in a couple of minutes
- Stem cell basics. National Institutes of Health. https://stemcells.nih.gov/info/basics/stc-basics/#stc-I. Accessed March 21, 2024.
- Lovell-Badge R, et al. ISSCR guidelines for stem cell research and clinical translation: The 2021 update. Stem Cell Reports. 2021; doi:10.1016/j.stemcr.2021.05.012.
- AskMayoExpert. Hematopoietic stem cell transplant. Mayo Clinic; 2024.
- Stem cell transplants in cancer treatment. National Cancer Institute. https://www.cancer.gov/about-cancer/treatment/types/stem-cell-transplant/. Accessed March 21, 2024.
- Townsend CM Jr, et al. Regenerative medicine. In: Sabiston Textbook of Surgery: The Biological Basis of Modern Surgical Practice. 21st ed. Elsevier; 2022. https://www.clinicalkey.com. Accessed March 21, 2024.
- Kumar D, et al. Stem cell based preclinical drug development and toxicity prediction. Current Pharmaceutical Design. 2021; doi:10.2174/1381612826666201019104712.
- NIH guidelines for human stem cell research. National Institutes of Health. https://stemcells.nih.gov/research-policy/guidelines-for-human-stem-cell-research. Accessed March 21, 2024.
- De la Torre P, et al. Current status and future prospects of perinatal stem cells. Genes. 2020; doi:10.3390/genes12010006.
- Yen Ling Wang A. Human induced pluripotent stem cell-derived exosomes as a new therapeutic strategy for various diseases. International Journal of Molecular Sciences. 2021; doi:10.3390/ijms22041769.
- Alessandrini M, et al. Stem cell therapy for neurological disorders. South African Medical Journal. 2019; doi:10.7196/SAMJ.2019.v109i8b.14009.
- Goldenberg D, et al. Regenerative engineering: Current applications and future perspectives. Frontiers in Surgery. 2021; doi:10.3389/fsurg.2021.731031.
- Brown MA, et al. Update on stem cell technologies in congenital heart disease. Journal of Cardiac Surgery. 2020; doi:10.1111/jocs.14312.
- Li M, et al. Brachyury engineers cardiac repair competent stem cells. Stem Cells Translational Medicine. 2021; doi:10.1002/sctm.20-0193.
- Augustine R, et al. Stem cell-based approaches in cardiac tissue engineering: Controlling the microenvironment for autologous cells. Biomedical Pharmacotherapy. 2021; doi:10.1016/j.biopha.2021.111425.
- Cloning fact sheet. National Human Genome Research Institute. https://www.genome.gov/about-genomics/fact-sheets/Cloning-Fact-Sheet. Accessed March 21, 2024.
- Dingli D (expert opinion). Mayo Clinic. Nov. 17, 2023.
Products and Services
- Sign up for Email: Get Your Free Resource – Coping with Cancer
- A Book: Living Medicine
- Give today to find cancer cures for tomorrow
- Acute lymphocytic leukemia
- Acute myelogenous leukemia
- Adjuvant therapy for cancer
- Amyloidosis
- Aplastic anemia
- Atypical cells: Are they cancer?
- Biopsy procedures
- Blood Cancers and Disorders
- Bone marrow transplant
- Cancer blood tests
- Myths about cancer causes
- Infographic: Cancer Clinical Trials Offer Many Benefits
- Cancer diagnosis: 11 tips for coping
- Cancer-related fatigue
- Cancer pain: Relief is possible
- Cancer risk: What the numbers mean
- Cancer surgery
- Cancer survival rate
- Cancer survivors: Care for your body after treatment
- Cancer survivors: Late effects of cancer treatment
- Cancer survivors: Managing your emotions after cancer treatment
- Cancer treatment myths
- Chemotherapy side effects: A cause of heart disease?
- Chronic lymphocytic leukemia
- Chronic myelogenous leukemia
- Curcumin: Can it slow cancer growth?
- What is type 1 diabetes? A Mayo Clinic expert explains
- Type 1 diabetes FAQs
- Cancer-related diarrhea
- DiGeorge syndrome (22q11.2 deletion syndrome)
- Eating during cancer treatment: Tips to make food tastier
- Epidermolysis bullosa
- Gaucher disease
- Heart cancer: Is there such a thing?
- High-dose vitamin C: Can it kill cancer cells?
- Hodgkin's lymphoma (Hodgkin's disease)
- Hodgkin's vs. non-Hodgkin's lymphoma: What's the difference?
- Low blood counts
- Measles Virus as a Cancer Fighter
- Monoclonal antibody drugs
- Mort Crim and Cancer
- Mouth sores caused by cancer treatment: How to cope
- Multiple myeloma
- Infographic: Multiple Myeloma
- Myelofibrosis
- Neuroblastoma
- No appetite? How to get nutrition during cancer treatment
- Non-Hodgkin's lymphoma
- Scleroderma
- Self-Image During Cancer
- Sickle cell anemia
- Sisters' Bone Marrow Transplant
- Small cell, large cell cancer: What this means
- Stem Cells 101
- Thalassemia
- Tumor vs. cyst: What's the difference?
- Type 1 diabetes
- Stem cell transplant
- How cancer spreads
- PICC line placement
- When cancer returns: How to cope with cancer recurrence
Mayo Clinic does not endorse companies or products. Advertising revenue supports our not-for-profit mission.
- Opportunities
Mayo Clinic Press
Check out these best-sellers and special offers on books and newsletters from Mayo Clinic Press .
- Mayo Clinic on Incontinence - Mayo Clinic Press Mayo Clinic on Incontinence
- The Essential Diabetes Book - Mayo Clinic Press The Essential Diabetes Book
- Mayo Clinic on Hearing and Balance - Mayo Clinic Press Mayo Clinic on Hearing and Balance
- FREE Mayo Clinic Diet Assessment - Mayo Clinic Press FREE Mayo Clinic Diet Assessment
- Mayo Clinic Health Letter - FREE book - Mayo Clinic Press Mayo Clinic Health Letter - FREE book
- Stem cells What they are and what they do
Your gift holds great power – donate today!
Make your tax-deductible gift and be a part of the cutting-edge research and care that's changing medicine.
Masks Strongly Recommended but Not Required in Maryland, Starting Immediately
Due to the downward trend in respiratory viruses in Maryland, masking is no longer required but remains strongly recommended in Johns Hopkins Medicine clinical locations in Maryland. Read more .
- Vaccines
- Masking Guidelines
- Visitor Guidelines
Institute for Basic Biomedical Sciences
Stem cell research at johns hopkins institute of basic biomedical sciences.
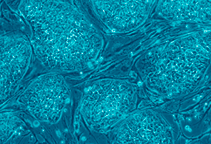
Researchers at the IBBS are studying stem cells to figure out how they can give rise to a complex body, how cells of the body can revert back to stem cells and how this knowledge can be used to develop therapies for diseases and injuries.
Stem cells are cells that don’t have an identity but have the potential to develop into many types of cells for many purposes, liking building a complete organism, healing a wound or replacing old, worn-out cells in a tissue.
Embryonic stem cells can become any of the cells in the body and can form entire animals.
Not all stem cells come from embryos, adult stem cells are found throughout the body too. These cells don’t have the ability to become any cell in the body, but can transform into many different cell types. For instance, there are stem cells in our bone marrow that can become fat cells, cartilage cells or bone cells, but they can’t become eye cells or skin cells. Researchers have also figured out how to make adult cells, like a skin cell, turn back into cells with the properties of embryonic stem cells, called induced pluripotent stem cells or iPS cells for short.
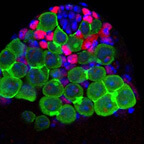
Erika Matunis , in the Department of Cell Biology , studies in fruit flies how testis stem cells decide to stay stem cells and not become other cell types, like sperm. She has also discovered how cells that are turning into other cell types can revert back to stem cells if the permanent reservoir of stem cells is depleted and she is exploring the mechanism of how this happens. Her research, learning more about the most fundamental aspects of stem cell biology, helps all stem cell researchers better understand the cells they work with.
Jennifer Elisseeff , of the Department of Biomedical Engineering , studies the differences between embryonic stem cells and adult stem cells. She has found that embryonic stem cells are better at forming new tissues, whereas adult stem cells are better at secreting therapeutic molecules that promote healing of damaged tissue. Elisseeff is particularly interested in the factors released by stem cells that can help a tissue heal. She uses this information in the development of biosynthetic (part-natural and part-man-made) materials used for therapies. One of the materials her lab has developed is a bio-adhesive—essentially a glue that can be used in the body that is made of part synthetic and part natural components. The glue is used in conjunction with stitches to help prevent leakage of blood or fluids, but it’s flexible enough to allow cells to move in and heal the incision. Also, Elisseeff is collaborating with the military to develop a treatment for soft tissue facial reconstruction for people who have suffered severe trauma. They are developing tissue blueprints that can be transplanted in the face—or any other place in the body for that matter— that would allow a person’s own cells to move into a region to heal and restructure the tissue.
Warren Grayson , of the Department of Biomedical Engineering , takes stem cells from fat and bone marrow as well as stem cells that have the potential to become many different cell types, known as pluripotent stem cells, and coaxes them to regenerate bone or skeletal muscle in the lab. He does this by incubating stem cells in biosynthetic structures to give the cells a structured three-dimensional volume to grow in, and then places these either in bioreactors that provide heat, nutrients, movement, mechanical stress or control of any other condition like oxygen concentration to guide the stem cell to become a specific cell type or within a defect in animals to study the regenerative process. He hopes to one day be able to take a person’s own stem cells and grow tissues, like bone or muscle, to be implanted into their body to replace damaged tissue. Using a person’s own cells and tissues will reduce the likelihood that the transplanted tissue will be rejected by the immune system.
Related Links : Stem Cell Research at Johns Hopkins
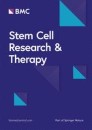
Volumes and issues
Volume 15 december 2024.
- December 2024, issue 1
Volume 14 December 2023
- December 2023, issue 1
Volume 13 December 2022
- December 2022, issue 1
Volume 12 December 2021
- December 2021, issue 1
Volume 11 December 2020
- December 2020, issue 1
Volume 10 December 2019
- December 2019, issue 1
Volume 9 December 2018
- December 2018, issue 1
Volume 8 December 2017
- December 2017, issue 1
Volume 7 December 2016
- December 2016, issue 1
Volume 6 December 2015
- December 2015, issue 1
Volume 5 March - December 2014
- December 2014, issue 6
- December 2014, issue 5
- December 2014, issue 4
- September 2014, issue 3
- June 2014, issue 2
- March 2014, issue 1
Volume 4 March - December 2013
- December 2013, issue 6
- December 2013, issue 5
- December 2013, issue 4
Stem cells on bioengineered microphysiological platforms for disease modeling and drug testing
- September 2013, issue 3
- June 2013, issue 2
- March 2013, issue 1
Volume 3 March - December 2012
- December 2012, issue 6
- December 2012, issue 5
- December 2012, issue 4
- September 2012, issue 3
- June 2012, issue 2
- March 2012, issue 1
Volume 2 February - December 2011
- December 2011, issue 6
- December 2011, issue 5
- August 2011, issue 4
- June 2011, issue 3
- April 2011, issue 2
- February 2011, issue 1
Volume 1 March - December 2010
- December 2010, issue 5
- December 2010, issue 4
- September 2010, issue 3
- June 2010, issue 2
- March 2010, issue 1
For authors
- Find a journal
- Publish with us
- Track your research
Stem cells: past, present, and future
Affiliations.
- 1 Department of Experimental Surgery and Biomaterials Research, Wroclaw Medical University, Bujwida 44, Wrocław, 50-345, Poland. [email protected].
- 2 Department of Conservative Dentistry and Pedodontics, Krakowska 26, Wrocław, 50-425, Poland.
- 3 Department of Experimental Surgery and Biomaterials Research, Wroclaw Medical University, Bujwida 44, Wrocław, 50-345, Poland.
- PMID: 30808416
- PMCID: PMC6390367
- DOI: 10.1186/s13287-019-1165-5
In recent years, stem cell therapy has become a very promising and advanced scientific research topic. The development of treatment methods has evoked great expectations. This paper is a review focused on the discovery of different stem cells and the potential therapies based on these cells. The genesis of stem cells is followed by laboratory steps of controlled stem cell culturing and derivation. Quality control and teratoma formation assays are important procedures in assessing the properties of the stem cells tested. Derivation methods and the utilization of culturing media are crucial to set proper environmental conditions for controlled differentiation. Among many types of stem tissue applications, the use of graphene scaffolds and the potential of extracellular vesicle-based therapies require attention due to their versatility. The review is summarized by challenges that stem cell therapy must overcome to be accepted worldwide. A wide variety of possibilities makes this cutting edge therapy a turning point in modern medicine, providing hope for untreatable diseases.
Keywords: Differentiation; Growth media; Induced pluripotent stem cell (iPSC); Pluripotency; Stem cell derivation; Stem cells; Teratoma formation assay; Tissue banks; Tissue transplantation.
Publication types
- Research Support, Non-U.S. Gov't
- Cell Differentiation / genetics*
- Cell- and Tissue-Based Therapy / trends*
- Graphite / chemistry
- Graphite / therapeutic use
- Induced Pluripotent Stem Cells / transplantation*
- Stem Cell Transplantation / classification
- Stem Cells / classification
- Stem Cells / cytology*
- Tissue Scaffolds / chemistry

Stem cells provide new insight into genetic pathway of childhood cancer
Scientists have discovered a new insight into the genetic pathway of childhood cancer, offering new hope for tailored treatments.
Researchers from the University of Sheffield have created a stem cell model designed to investigate the origins of neuroblastoma, a cancer primarily affecting babies and young children.
Neuroblastoma is the most common childhood tumour occurring outside the brain, affecting the lives of approximately 600 children in the European Union and the United Kingdom each year.
Until now, studying genetic changes and their role in neuroblastoma initiation has been challenging due to the lack of suitable laboratory methods. A new model developed by researchers at the University of Sheffield, in collaboration with the St Anna Children's Cancer Research Institute in Vienna, replicates the emergence of early neuroblastoma cancer-like cells, giving an insight into the genetic pathway of the disease.
The research, published in Nature Communications , sheds light on the intricate genetic pathways which initiate neuroblastoma. The international research team found that specific mutations in chromosomes 17 and 1, combined with overactivation of the MYCN gene, play a pivotal role in the development of aggressive neuroblastoma tumours.
Childhood cancer is often diagnosed and detected late, leaving researchers with very little idea of the conditions that led to tumour initiation, which occurs very early during fetal development. In order to understand tumour initiation, models which recreate the conditions that lead to the appearance of a tumour are vital.
The formation of neuroblastoma usually starts in the womb when a group of normal embryonic cells called 'trunk neural crest (NC)' become mutated and cancerous.
In an interdisciplinary effort spearheaded by stem cell expert Dr Ingrid Saldana from the University of Sheffield's School of Biosciences and computational biologist Dr Luis Montano from the St Anna Children's Cancer Research Institute in Vienna, the new study found a way in which to use human stem cells to grow trunk NC cells in a petri dish.
These cells carried genetic changes often seen in aggressive neuroblastoma tumours. Using genomics analysis and advanced imaging techniques, the researchers found that the altered cells started behaving like cancer cells and looked very similar to the neuroblastoma cells found in sick children.
The findings offer new hope for the creation of tailored treatments that specifically target the cancer while minimising the adverse effects experienced by patients from existing therapies.
Dr Anestis Tsakiridis, from the University of Sheffield's School of Biosciences and lead author of the study, said: "Our stem cell-based model mimics the early stages of aggressive neuroblastoma formation, providing invaluable insights into the genetic drivers of this devastating childhood cancer. By recreating the conditions that lead to tumour initiation, we will be able to understand better the mechanisms underpinning this process and thus design improved treatment strategies in the longer term.
"This is very important as survival rates for children with aggressive neuroblastoma are poor and most survivors suffer from side effects linked to the harsh treatments currently used, which include potential hearing, fertility and lung problems."
Dr. Florian Halbritter, from St. Anna Children's Cancer Research Institute and second lead author of the study, said: "This was an impressive team effort, breaching geographic and disciplinary boundaries to enable new discoveries in childhood cancer research."
This research supports the University of Sheffield's cancer research strategy. Through the strategy, the University aims to prevent cancer-related deaths by undertaking high quality research, leading to more effective treatments, as well as methods to better prevent and detect cancer and improve quality of life.
- Lung Cancer
- Breast Cancer
- Skin Cancer
- Brain Tumor
- Prostate Cancer
- Colon Cancer
- Stem cell treatments
- Cervical cancer
- Colorectal cancer
- Breast cancer
- Prostate cancer
Story Source:
Materials provided by University of Sheffield . Note: Content may be edited for style and length.
Journal Reference :
- Ingrid M. Saldana-Guerrero, Luis F. Montano-Gutierrez, Katy Boswell, Christoph Hafemeister, Evon Poon, Lisa E. Shaw, Dylan Stavish, Rebecca A. Lea, Sara Wernig-Zorc, Eva Bozsaky, Irfete S. Fetahu, Peter Zoescher, Ulrike Pötschger, Marie Bernkopf, Andrea Wenninger-Weinzierl, Caterina Sturtzel, Celine Souilhol, Sophia Tarelli, Mohamed R. Shoeb, Polyxeni Bozatzi, Magdalena Rados, Maria Guarini, Michelle C. Buri, Wolfgang Weninger, Eva M. Putz, Miller Huang, Ruth Ladenstein, Peter W. Andrews, Ivana Barbaric, George D. Cresswell, Helen E. Bryant, Martin Distel, Louis Chesler, Sabine Taschner-Mandl, Matthias Farlik, Anestis Tsakiridis, Florian Halbritter. A human neural crest model reveals the developmental impact of neuroblastoma-associated chromosomal aberrations . Nature Communications , 2024; 15 (1) DOI: 10.1038/s41467-024-47945-7
Cite This Page :
Explore More
- High-Efficiency Photonic Integrated Circuit
- Life Expectancy May Increase by 5 Years by 2050
- Toward a Successful Vaccine for HIV
- Highly Efficient Thermoelectric Materials
- Toward Human Brain Gene Therapy
- Whale Families Learn Each Other's Vocal Style
- AI Can Answer Complex Physics Questions
- Otters Use Tools to Survive a Changing World
- Monogamy in Mice: Newly Evolved Type of Cell
- Sustainable Electronics, Doped With Air
Trending Topics
Strange & offbeat.
Identifiers
Linking ISSN (ISSN-L): 2641-3000
URL https://www.peertechz.com/journals/studies-on-stem-cells-research-and-therapy/archive
Google https://www.google.com/search?q=ISSN+%222641-3000%22
Bing https://www.bing.com/search?q=ISSN+%222641-3000%22
Yahoo https://search.yahoo.com/search?p=ISSN%20%222641-3000%22
Library of Congress https://catalog.loc.gov/vwebv/search?searchCode=STNO&searchArg=2641-3000&searchType=1&limitTo=none&fromYear=&toYear=&limitTo=LOCA%3Dall&limitTo=PLAC%3Dall&limitTo=TYPE%3Dall&limitTo=LANG%3Dall&recCount=25
Resource information
Archival status.

Title proper: Studies on stem cells research and therapy.
Other variant title: SSCRT
Country: United States
Medium: Online
Record information
Last modification date: 06/02/2021
Type of record: Confirmed
ISSN Center responsible of the record: ISSN National Centre for the USA
downloads requested
Discover all the features of the complete ISSN records
Display mode x.
Labelled view
MARC21 view
UNIMARC view
Suggestions or feedback?
MIT News | Massachusetts Institute of Technology
- Machine learning
- Social justice
- Black holes
- Classes and programs
Departments
- Aeronautics and Astronautics
- Brain and Cognitive Sciences
- Architecture
- Political Science
- Mechanical Engineering
Centers, Labs, & Programs
- Abdul Latif Jameel Poverty Action Lab (J-PAL)
- Picower Institute for Learning and Memory
- Lincoln Laboratory
- School of Architecture + Planning
- School of Engineering
- School of Humanities, Arts, and Social Sciences
- Sloan School of Management
- School of Science
- MIT Schwarzman College of Computing
New treatment could reverse hair loss caused by an autoimmune skin disease
Press contact :, media download.
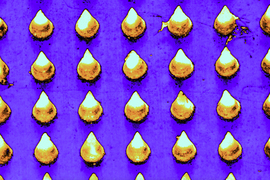
*Terms of Use:
Images for download on the MIT News office website are made available to non-commercial entities, press and the general public under a Creative Commons Attribution Non-Commercial No Derivatives license . You may not alter the images provided, other than to crop them to size. A credit line must be used when reproducing images; if one is not provided below, credit the images to "MIT."
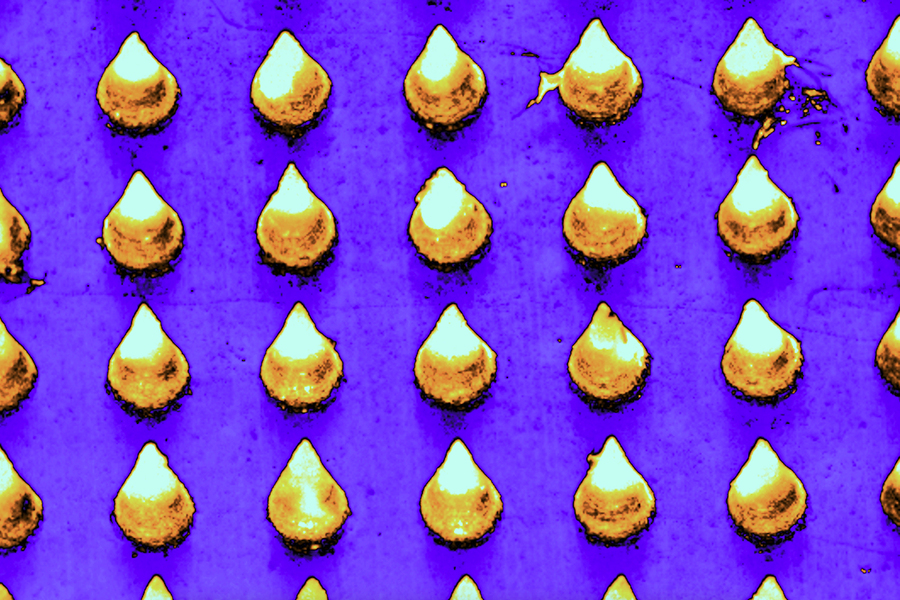
Previous image Next image
Researchers at MIT, Brigham and Women’s Hospital, and Harvard Medical School have developed a potential new treatment for alopecia areata, an autoimmune disorder that causes hair loss and affects people of all ages, including children.
For most patients with this type of hair loss, there is no effective treatment. The team developed a microneedle patch that can be painlessly applied to the scalp and releases drugs that help to rebalance the immune response at the site, halting the autoimmune attack.
In a study of mice, the researchers found that this treatment allowed hair to regrow and dramatically reduced inflammation at the treatment site, while avoiding systemic immune effects elsewhere in the body. This strategy could also be adapted to treat other autoimmune skin diseases such as vitiligo, atopic dermatitis, and psoriasis, the researchers say.
“This innovative approach marks a paradigm shift. Rather than suppressing the immune system, we’re now focusing on regulating it precisely at the site of antigen encounter to generate immune tolerance,” says Natalie Artzi, a principal research scientist in MIT’s Institute for Medical Engineering and Science, an associate professor of medicine at Harvard Medical School and Brigham and Women’s Hospital, and an associate faculty member at the Wyss Institute of Harvard University.
Artzi and Jamil R. Azzi, an associate professor of medicine at Harvard Medical School and Brigham and Women’s Hospital, are the senior authors of the new study , which appears in the journal Advanced Materials . Nour Younis, a Brigham and Women’s postdoc, and Nuria Puigmal, a Brigham and Women’s postdoc and former MIT research affiliate, are the lead authors of the paper.
The researchers are now working on launching a company to further develop the technology, led by Puigmal, who was recently awarded a Harvard Business School Blavatnik Fellowship.
Direct delivery
Alopecia areata, which affects more than 6 million Americans, occurs when the body’s own T cells attack hair follicles, leading the hair to fall out. The only treatment available to most patients — injections of immunosuppressant steroids into the scalp — is painful and patients often can’t tolerate it.
Some patients with alopecia areata and other autoimmune skin diseases can also be treated with immunosuppressant drugs that are given orally, but these drugs lead to widespread suppression of the immune system, which can have adverse side effects.
“This approach silences the entire immune system, offering relief from inflammation symptoms but leading to frequent recurrences. Moreover, it increases susceptibility to infections, cardiovascular diseases, and cancer,” Artzi says.
A few years ago, at a working group meeting in Washington, Artzi happened to be seated next to Azzi (the seating was alphabetical), an immunologist and transplant physican who was seeking new ways to deliver drugs directly to the skin to treat skin-related diseases.
Their conversation led to a new collaboration, and the two labs joined forces to work on a microneedle patch to deliver drugs to the skin. In 2021, they reported that such a patch can be used to prevent rejection following skin transplant. In the new study, they began applying this approach to autoimmune skin disorders.
“The skin is the only organ in our body that we can see and touch, and yet when it comes to drug delivery to the skin, we revert to systemic administration. We saw great potential in utilizing the microneedle patch to reprogram the immune system locally,” Azzi says.
The microneedle patches used in this study are made from hyaluronic acid crosslinked with polyethylene glycol (PEG), both of which are biocompatible and commonly used in medical applications. With this delivery method, drugs can pass through the tough outer layer of the epidermis, which can’t be penetrated by creams applied to the skin.
“This polymer formulation allows us to create highly durable needles capable of effectively penetrating the skin. Additionally, it gives us the flexibility to incorporate any desired drug,” Artzi says. For this study, the researchers loaded the patches with a combination of the cytokines IL-2 and CCL-22. Together, these immune molecules help to recruit regulatory T cells, which proliferate and help to tamp down inflammation. These cells also help the immune system learn to recognize that hair follicles are not foreign antigens, so that it will stop attacking them.
Hair regrowth
The researchers found that mice treated with this patch every other day for three weeks had many more regulatory T cells present at the site, along with a reduction in inflammation. Hair was able to regrow at those sites, and this growth was maintained for several weeks after the treatment ended. In these mice, there were no changes in the levels of regulatory T cells in the spleen or lymph nodes, suggesting that the treatment affected only the site where the patch was applied.
In another set of experiments, the researchers grafted human skin onto mice with a humanized immune system. In these mice, the microneedle treatment also induced proliferation of regulatory T cells and a reduction in inflammation.
The researchers designed the microneedle patches so that after releasing their drug payload, they can also collect samples that could be used to monitor the progress of the treatment. Hyaluronic acid causes the needles to swell about tenfold after entering the skin, which allows them to absorb interstitial fluid containing biomolecules and immune cells from the skin.
Following patch removal, researchers can analyze samples to measure levels of regulatory T cells and inflammation markers. This could prove valuable for monitoring future patients who may undergo this treatment.
The researchers now plan to further develop this approach for treating alopecia, and to expand into other autoimmune skin diseases.
The research was funded by the Ignite Fund and Shark Tank Fund awards from the Department of Medicine at Brigham and Women’s Hospital.
Share this news article on:
Press mentions, healthday news.
MIT researchers have developed microneedle patches that are capable of restoring hair growth in alopecia areata patients, reports Ernie Mundell for HealthDay . The team’s approach includes a, “patch containing myriad microneedles that is applied to the scalp,” writes Mundell. “It releases drugs to reset the immune system so it stops attacking follicles.”
Previous item Next item
Related Links
- Natalie Artzi
- Institute for Medical Engineering and Science
Related Topics
- Drug delivery
- Health sciences and technology
- Institute for Medical Engineering and Science (IMES)
Related Articles
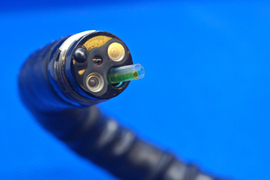
A sprayable gel could make minimally invasive surgeries simpler and safer
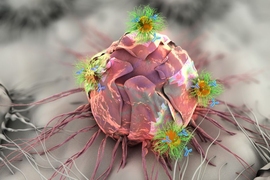
Patch that delivers drug, gene, and light-based therapy to tumor sites shows promising results
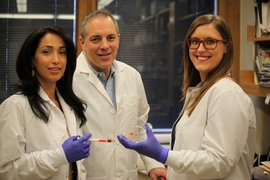
MIT researchers design tailored tissue adhesives
More mit news.
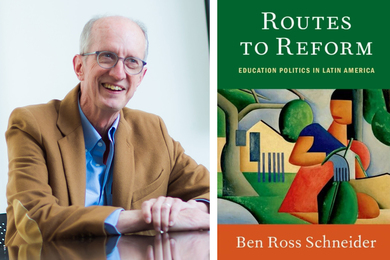
Trying to make the grade
Read full story →
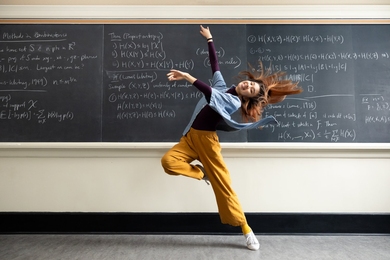

Janabel Xia: Algorithms, dance rhythms, and the drive to succeed
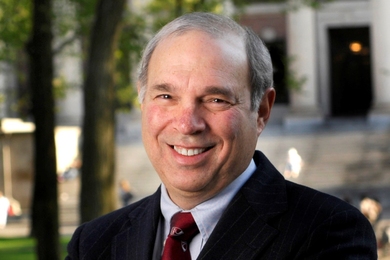
Jonathan Byrnes, MIT Center for Transportation and Logistics senior lecturer and visionary in supply chain management, dies at 75
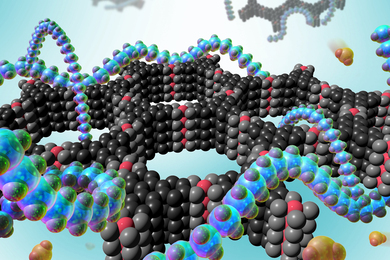
Researchers develop a detector for continuously monitoring toxic gases
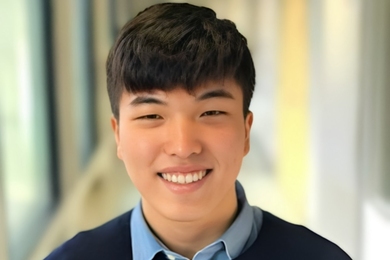
The beauty of biology
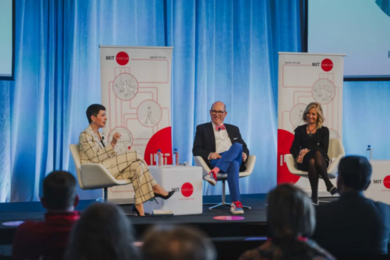
Navigating longevity with industry leaders at MIT AgeLab PLAN Forum
- More news on MIT News homepage →
Massachusetts Institute of Technology 77 Massachusetts Avenue, Cambridge, MA, USA
- Map (opens in new window)
- Events (opens in new window)
- People (opens in new window)
- Careers (opens in new window)
- Accessibility
- Social Media Hub
- MIT on Facebook
- MIT on YouTube
- MIT on Instagram
Thank you for visiting nature.com. You are using a browser version with limited support for CSS. To obtain the best experience, we recommend you use a more up to date browser (or turn off compatibility mode in Internet Explorer). In the meantime, to ensure continued support, we are displaying the site without styles and JavaScript.
- View all journals
- Explore content
- About the journal
- Publish with us
- Sign up for alerts
- Review Article
- Published: 14 May 2024
Challenges and perspectives of heart repair with pluripotent stem cell-derived cardiomyocytes
- Thomas Eschenhagen ORCID: orcid.org/0000-0003-1750-1170 1 , 2 &
- Florian Weinberger 1 , 2 , 3
Nature Cardiovascular Research volume 3 , pages 515–524 ( 2024 ) Cite this article
140 Accesses
8 Altmetric
Metrics details
- Cardiac regeneration
- Myocardial infarction
Here we aim at providing a concise but comprehensive overview of the perspectives and challenges of heart repair with pluripotent stem cell-derived cardiomyocytes. This Review comes at a time when consensus has been reached about the lack of relevant proliferative capacity of adult mammalian cardiomyocytes and the lack of new heart muscle formation with autologous cell sources. While alternatives to cell-based approaches will be shortly summarized, the focus lies on pluripotent stem cell-derived cardiomyocyte repair, which entered first clinical trials just 2 years ago. In the view of the authors, these early trials are important but have to be viewed as early proof-of-concept trials in humans that will hopefully provide first answers on feasibility, safety and the survival of allogeneic pluripotent stem cell-derived cardiomyocyte in the human heart. Better approaches have to be developed to make this approach clinically applicable.
This is a preview of subscription content, access via your institution
Access options
Subscribe to this journal
Receive 12 digital issues and online access to articles
111,21 € per year
only 9,27 € per issue
Buy this article
- Purchase on Springer Link
- Instant access to full article PDF
Prices may be subject to local taxes which are calculated during checkout
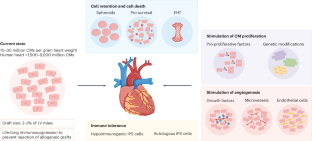
Similar content being viewed by others
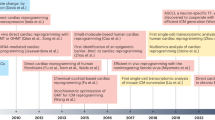
Recent advances and future prospects in direct cardiac reprogramming
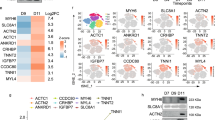
Pluripotent stem cell-derived committed cardiac progenitors remuscularize damaged ischemic hearts and improve their function in pigs
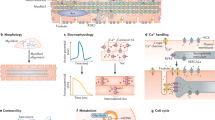
Cardiomyocyte maturation: advances in knowledge and implications for regenerative medicine
Nabel, E. G. & Braunwald, E. A tale of coronary artery disease and myocardial infarction. N. Engl. J. Med. 366 , 54–63 (2012).
Article CAS PubMed Google Scholar
McDonagh, T. A. et al. Group ESCSD. 2021 ESC guidelines for the diagnosis and treatment of acute and chronic heart failure. Eur. Heart J. 42 , 3599–3726 (2021).
Goldenberg, B. Ueber Atrophie und Hypertrophie der Muskelfasern des Herzens. Arch. Pathol. Anat. Physiol. Klin. Med. 103 , 88–130 (1886).
Article Google Scholar
Linzbach, A. J. Heart failure from the point of view of quantitative anatomy. Am. J. Cardiol. 5 , 370–382 (1960).
Linzbach, A. J. Hypertrophy, hyperplasia and structural dilatation of the human heart. Adv. Cardiol. 18 , 1–14 (1976).
Soonpaa, M. H., Kim, K. K., Pajak, L., Franklin, M. & Field, L. J. Cardiomyocyte DNA synthesis and binucleation during murine development. Am. J. Physiol. 271 , H2183–H2189 (1996).
CAS PubMed Google Scholar
Bergmann, O. et al. Evidence for cardiomyocyte renewal in humans. Science 324 , 98–102 (2009).
Article CAS PubMed PubMed Central Google Scholar
Olivetti, G. et al. Apoptosis in the failing human heart. N. Engl. J. Med. 336 , 1131–1141 (1997).
Quaini, F. et al. Chimerism of the transplanted heart. N. Engl. J. Med. 346 , 5–15 (2002).
Article PubMed Google Scholar
Orlic, D. et al. Bone marrow cells regenerate infarcted myocardium. Nature 410 , 701–705 (2001).
Jeyaraman, M. M. et al. Autologous bone marrow stem cell therapy in patients with st-elevation myocardial infarction: a systematic review and meta-analysis. Can. J. Cardiol. 33 , 1611–1623 (2017).
Blau, H. M., Brazelton, T. R. & Weimann, J. M. The evolving concept of a stem cell: entity or function? Cell 105 , 829–841 (2001).
Dimmeler, S., Zeiher, A. M. & Schneider, M. D. Unchain my heart: the scientific foundations of cardiac repair. J. Clin. Invest. 115 , 572–583 (2005).
Beltrami, A. P. et al. Adult cardiac stem cells are multipotent and support myocardial regeneration. Cell 114 , 763–776 (2003).
Oh, H. et al. Cardiac progenitor cells from adult myocardium: homing, differentiation, and fusion after infarction. Proc. Natl Acad. Sci. USA 100 , 12313–12318 (2003).
Laugwitz, K. L. et al. Postnatal isl1 + cardioblasts enter fully differentiated cardiomyocyte lineages. Nature. 433 , 647–653 (2005).
Planat-Benard, V. et al. Spontaneous cardiomyocyte differentiation from adipose tissue stroma cells. Circ. Res. 94 , 223–229 (2004).
Condorelli, G. et al. Cardiomyocytes induce endothelial cells to trans-differentiate into cardiac muscle: Implications for myocardium regeneration. Proc. Natl Acad. Sci. USA 98 , 10733–10738 (2001).
Li, Y. et al. Genetic lineage tracing of nonmyocyte population by dual recombinases. Circulation 138 , 793–805 (2018).
Murry, C. E. et al. Haematopoietic stem cells do not transdifferentiate into cardiac myocytes in myocardial infarcts. Nature 428 , 664–668 (2004).
Nygren, J. M. et al. Bone marrow-derived hematopoietic cells generate cardiomyocytes at a low frequency through cell fusion, but not transdifferentiation. Nat. Med. 10 , 494–501 (2004).
Laflamme, M. A., Myerson, D., Saffitz, J. E. & Murry, C. E. Evidence for cardiomyocyte repopulation by extracardiac progenitors in transplanted human hearts. Circ. Res. 90 , 634–640 (2002).
Hocht-Zeisberg, E. et al. Cellular repopulation of myocardial infarction in patients with sex-mismatched heart transplantation. Eur. Heart J. 25 , 749–758 (2004).
Partners HealthCare and Brigham and Women’s Hospital agree to pay $10 million to resolve research fraud allegations. US Attorney’s Office District of Massachusetts https://www.justice.gov/usao-ma/pr/partners-healthcare-and-brigham-and-women-s-hospital-agree-pay-10-million-resolve (2017).
Eschenhagen, T. et al. Cardiomyocyte regeneration: a consensus statement. Circulation 136 , 680–686 (2017).
Article PubMed PubMed Central Google Scholar
Senyo, S. E. et al. Mammalian heart renewal by pre-existing cardiomyocytes. Nature 493 , 433–436 (2013).
Vagnozzi, R. J. et al. An acute immune response underlies the benefit of cardiac stem cell therapy. Nature 577 , 405–409 (2020).
Sadek, H. & Olson, E. N. Toward the goal of human heart regeneration. Cell Stem Cell 26 , 7–16 (2020).
Giacca, M. Fulfilling the promise of rna therapies for cardiac repair and regeneration. Stem Cells Transl. Med. 12 , 527–535 (2023).
Yamada, Y., Sadahiro, T. & Ieda, M. Development of direct cardiac reprogramming for clinical applications. J. Mol. Cell Cardiol. 178 , 1–8 (2023).
Soonpaa, M. H., Koh, G. Y., Klug, M. G. & Field, L. J. Formation of nascent intercalated disks between grafted fetal cardiomyocytes and host myocardium. Science 264 , 98–101 (1994).
Thomson, J. A. et al. Embryonic stem cell lines derived from human blastocysts. Science 282 , 1145–1147 (1998).
Takahashi, K. et al. Induction of pluripotent stem cells from adult human fibroblasts by defined factors. Cell 131 , 861–872 (2007).
Kehat, I. et al. Human embryonic stem cells can differentiate into myocytes with structural and functional properties of cardiomyocytes. J. Clin. Invest. 108 , 407–414 (2001).
Kattman, S. J. et al. Stage-specific optimization of activin/nodal and bmp signaling promotes cardiac differentiation of mouse and human pluripotent stem cell lines. Cell Stem Cell. 8 , 228–240 (2011).
Burridge, P. W. & Zambidis, E. T. Highly efficient directed differentiation of human induced pluripotent stem cells into cardiomyocytes. Methods Mol. Biol. 997 , 149–161 (2013).
Breckwoldt, K. et al. Differentiation of cardiomyocytes and generation of human engineered heart tissue. Nat. Protoc. 12 , 1177–1197 (2017).
Halloin, C., Coffee, M., Manstein, F. & Zweigerdt, R. Production of cardiomyocytes from human pluripotent stem cells by bioreactor technologies. Methods Mol. Biol. 1994 , 55–70 (2019).
Buikema, J. W. et al. Wnt activation and reduced cell–cell contact synergistically induce massive expansion of functional human iPSC-derived cardiomyocytes. Cell Stem Cell 27 , 50–63 (2020).
Eschenhagen, T., Ridders, K. & Weinberger, F. How to repair a broken heart with pluripotent stem cell-derived cardiomyocytes. J Mol Cell Cardiol. 163 , 106–117 (2022).
Weinberger, F. & Eschenhagen, T. Cardiac regeneration: new hope for an old dream. Annu. Rev. Physiol. 83 , 59–81 (2021).
Shiba, Y. et al. Allogeneic transplantation of iPS cell-derived cardiomyocytes regenerates primate hearts. Nature 538 , 388–391 (2016).
Chong, J. J. et al. Human embryonic-stem-cell-derived cardiomyocytes regenerate non-human primate hearts. Nature 510 , 273–277 (2014).
Liu, Y. W. et al. Human embryonic stem cell-derived cardiomyocytes restore function in infarcted hearts of non-human primates. Nat. Biotechnol. 36 , 597–605 (2018).
Querdel, E. et al. Human engineered heart tissue patches remuscularize the injured heart in a dose-dependent manner. Circulation 143 , 1991–2006 (2021).
Riegler, J. et al. Human engineered heart muscles engraft and survive long term in a rodent myocardial infarction model. Circ. Res. 117 , 720–730 (2015).
Laflamme, M. A. et al. Cardiomyocytes derived from human embryonic stem cells in pro-survival factors enhance function of infarcted rat hearts. Nat. Biotechnol. 25 , 1015–1024 (2007).
Weinberger, F. et al. Cardiac repair in guinea pigs with human engineered heart tissue from induced pluripotent stem cells. Sci. Transl. Med. 8 , 363ra148 (2016).
Bergmann, O. et al. Dynamics of cell generation and turnover in the human heart. Cell 161 , 1566–1575 (2015).
Lou, X. et al. N -cadherin overexpression enhances the reparative potency of human-induced pluripotent stem cell-derived cardiac myocytes in infarcted mouse hearts. Cardiovasc. Res. 116 , 671–685 (2020).
Kobayashi, H. et al. Intracoronary transplantation of pluripotent stem cell-derived cardiomyocytes: inefficient procedure for cardiac regeneration. J. Mol. Cell Cardiol. 174 , 77–87 (2023).
Li, J. et al. All roads lead to rome (the heart): cell retention and outcomes from various delivery routes of cell therapy products to the heart. J. Am. Heart Assoc. 10 , e020402 (2021).
Dow, J., Simkhovich, B. Z., Kedes, L. & Kloner, R. A. Washout of transplanted cells from the heart: a potential new hurdle for cell transplantation therapy. Cardiovasc. Res. 67 , 301–307 (2005).
Harris, N. R. et al. The ebb and flow of cardiac lymphatics: a tidal wave of new discoveries. Physiol. Rev. 103 , 391–432 (2023).
Kawaguchi, S. et al. Intramyocardial transplantation of human ips cell-derived cardiac spheroids improves cardiac function in heart failure animals. JACC Basic Transl. Sci. 6 , 239–254 (2021).
El-Nachef, D. et al. Engrafted human induced pluripotent stem cell-derived cardiomyocytes undergo clonal expansion in vivo. Circulation 143 , 1635–1638 (2021).
Laflamme, M. A. et al. Formation of human myocardium in the rat heart from human embryonic stem cells. Am. J. Pathol. 167 , 663–671 (2005).
Fernandes, S. et al. Human embryonic stem cell-derived cardiomyocytes engraft but do not alter cardiac remodeling after chronic infarction in rats. J. Mol. Cell Cardiol. 49 , 941–949 (2010).
Eschenhagen, T., Mummery, C. & Knollmann, B. C. Modelling sarcomeric cardiomyopathies in the dish: from human heart samples to iPSC cardiomyocytes. Cardiovasc. Res. 105 , 424–438 (2015).
Mitsutake, Y. et al. Improvement of local cell delivery using helix transendocardial delivery catheter in a porcine heart. Int. Heart J. 58 , 435–440 (2017).
Nakamura, K. et al. Pharmacologic therapy for engraftment arrhythmia induced by transplantation of human cardiomyocytes. Stem Cell Rep. 16 , 2473–2487 (2021).
Article CAS Google Scholar
Nakajima, K. et al. Gelatin hydrogel enhances the engraftment of transplanted cardiomyocytes and angiogenesis to ameliorate cardiac function after myocardial infarction. PLoS ONE 10 , e0133308 (2015).
Furuta, A. et al. Pulsatile cardiac tissue grafts using a novel three-dimensional cell sheet manipulation technique functionally integrates with the host heart, in vivo. Circ. Res. 98 , 705–712 (2006).
Kawamura, M. et al. Enhanced survival of transplanted human induced pluripotent stem cell-derived cardiomyocytes by the combination of cell sheets with the pedicled omental flap technique in a porcine heart. Circulation 128 , S87–S94 (2013).
Zimmermann, W. H. et al. Engineered heart tissue grafts improve systolic and diastolic function in infarcted rat hearts. Nat. Med. 12 , 452–458 (2006).
Zhu, W., Zhao, M., Mattapally, S., Chen, S. & Zhang, J. Ccnd2 overexpression enhances the regenerative potency of human induced pluripotent stem cell-derived cardiomyocytes: remuscularization of injured ventricle. Circ. Res. 122 , 88–96 (2018).
Zhao, M. et al. Cyclin d2 overexpression enhances the efficacy of human induced pluripotent stem cell-derived cardiomyocytes for myocardial repair in a swine model of myocardial infarction. Circulation 144 , 210–228 (2021).
Gabisonia, K. et al. Microrna therapy stimulates uncontrolled cardiac repair after myocardial infarction in pigs. Nature 569 , 418–422 (2019).
Gerbin, K. A., Mitzelfelt, K. A., Guan, X., Martinson, A. M. & Murry, C. E. Delta-1 functionalized hydrogel promotes hESC-cardiomyocyte graft proliferation and maintains heart function post-injury. Mol. Ther. Methods Clin. Dev. 17 , 986–998 (2020).
Shimizu, T. et al. Long-term survival and growth of pulsatile myocardial tissue grafts engineered by the layering of cardiomyocyte sheets. Tissue Eng. 12 , 499–507 (2006).
von Bibra, C. et al. Human engineered heart tissue transplantation in a guinea pig chronic injury model. J. Mol. Cell Cardiol. 166 , 1–10 (2022).
Munarin, F., Kant, R. J., Rupert, C. E., Khoo, A. & Coulombe, K. L. K. Engineered human myocardium with local release of angiogenic proteins improves vascularization and cardiac function in injured rat hearts. Biomaterials 251 , 120033 (2020).
Ai, X. et al. Transient secretion of VEGF protein from transplanted hiPSC-CMs enhances engraftment and improves rat heart function post MI. Mol. Ther. 31 , 211–229 (2023).
Cheng, Y. Y. et al. Metabolic changes associated with cardiomyocyte dedifferentiation enable adult mammalian cardiac regeneration. Circulation 146 , 1950–1967 (2022).
Sun, X. et al. Transplanted microvessels improve pluripotent stem cell-derived cardiomyocyte engraftment and cardiac function after infarction in rats. Sci. Transl. Med. 12 , eaax2992 (2020).
von Bibra, C. et al. Immature human engineered heart tissues engraft in a guinea pig chronic injury model. Dis. Model Mech. 16 , 049834 (2023).
Google Scholar
Dhahri, W. et al. In vitro matured human pluripotent stem cell-derived cardiomyocytes form grafts with enhanced structure and function in injured hearts. Circulation 145 , 1412–1426 (2022).
Poch, C. M. et al. Migratory and anti-fibrotic programmes define the regenerative potential of human cardiac progenitors. Nat. Cell Biol. 24 , 659–671 (2022).
Ottaviani, D., Ter Huurne, M., Elliott, D. A., Bellin, M. & Mummery, C. L. Maturing differentiated human pluripotent stem cells in vitro: methods and challenges. Development https://doi.org/10.1242/dev.201103 (2023).
Shiba, Y. et al. Electrical integration of human embryonic stem cell-derived cardiomyocytes in a guinea pig chronic infarct model. J. Cardiovasc. Pharmacol. Ther. 19 , 368–381 (2014).
Gao, L. et al. Large cardiac muscle patches engineered from human induced-pluripotent stem cell-derived cardiac cells improve recovery from myocardial infarction in swine. Circulation 137 , 1712–1730 (2018).
Zhu, K. et al. Lack of remuscularization following transplantation of human embryonic stem cell-derived cardiovascular progenitor cells in infarcted nonhuman primates. Circ. Res. 122 , 958–969 (2018).
Shiba, Y. et al. Human ES-cell-derived cardiomyocytes electrically couple and suppress arrhythmias in injured hearts. Nature 489 , 322–325 (2012).
Shadrin, I. Y. et al. Cardiopatch platform enables maturation and scale-up of human pluripotent stem cell-derived engineered heart tissues. Nat. Commun. 8 , 1825 (2017).
Studemann, T. et al. Contractile force of transplanted cardiomyocytes actively supports heart function after injury. Circulation 146 , 1159–1169 (2022).
Pecha, S. et al. Human iPS cell-derived engineered heart tissue does not affect ventricular arrhythmias in a guinea pig cryo-injury model. Sci. Rep. 9 , 9831 (2019).
Romagnuolo, R. et al. Human embryonic stem cell-derived cardiomyocytes regenerate the infarcted pig heart but induce ventricular tachyarrhythmias. Stem Cell Rep. 12 , 967–981 (2019).
Selvakumar, D. et al. Cellular heterogeneity of pluripotent stem cell-derived cardiomyocyte grafts is mechanistically linked to treatable arrhythmias. Nat. Cardiovasc. Res. 3 , 145–165 (2024).
Caspi, O. et al. Transplantation of human embryonic stem cell-derived cardiomyocytes improves myocardial performance in infarcted rat hearts. J. Am. Coll. Cardiol. 50 , 1884–1893 (2007).
Blin, G. et al. A purified population of multipotent cardiovascular progenitors derived from primate pluripotent stem cells engrafts in postmyocardial infarcted nonhuman primates. J. Clin. Invest. 120 , 1125–1139 (2010).
Itakura, G. et al. Fail-safe system against potential tumorigenicity after transplantation of ipsc derivatives. Stem Cell Rep. 8 , 673–684 (2017).
de Luzy, I. R. et al. Human stem cells harboring a suicide gene improve the safety and standardisation of neural transplants in parkinsonian rats. Nat. Commun. 12 , 3275 (2021).
Meissner, T. B., Schulze, H. S. & Dale, S. M. Immune editing: overcoming immune barriers in stem cell transplantation. Curr. Stem Cell Rep. 8 , 206–218 (2022).
Simpson, A., Hewitt, A. W. & Fairfax, K. A. Universal cell donor lines: a review of the current research. Stem Cell Rep. https://doi.org/10.1016/j.stemcr.2023.09.010 (2023).
Hu, X. et al. Hypoimmune induced pluripotent stem cells survive long term in fully immunocompetent, allogeneic rhesus macaques. Nat. Biotechnol . 42 , 413–423 (2023).
Eschenhagen, T. & Weinberger, F. Heart repair with myocytes. Circ. Res. 124 , 843–845 (2019).
Garreta, E., Sanchez, S., Lajara, J., Montserrat, N. & Belmonte, J. C. I. Roadblocks in the path of IPSC to the clinic. Curr. Transplant Rep. 5 , 14–18 (2018).
Bogomiakova, M. E. et al. iPSC-derived cells lack immune tolerance to autologous NK-cells due to imbalance in ligands for activating and inhibitory NK-cell receptors. Stem Cell Res. Ther. 14 , 77 (2023).
Deuse, T. et al. De novo mutations in mitochondrial DNA of iPSCs produce immunogenic neoepitopes in mice and humans. Nat. Biotechnol. 37 , 1137–1144 (2019).
Schweitzer, J. S. et al. Personalized iPSC-derived dopamine progenitor cells for Parkinson’s disease. N. Engl. J. Med. 382 , 1926–1932 (2020).
Download references
Acknowledgements
Our work on this topic was supported by a Late Translational Research Grant from the German Centre for Cardiovascular Research (DZHK), (81×2710153 to T.E.), the European Research Council (ERC-AG IndivuHeart to T.E.), the German Research Foundation (DFG, WE5620/3-1 to F.W. and T.E.) and the Werner Otto Stiftung (F.W.). Additionally, we have received funding from the European Union’s Horizon 2020 research and innovation program (874764 to T.E.) and the European Union’s Horizon 2020 FetOpen RIA (964800; to F.W.).
Author information
Authors and affiliations.
Institute of Experimental Pharmacology and Toxicology, University Medical Center Hamburg Eppendorf, Hamburg, Germany
Thomas Eschenhagen & Florian Weinberger
German Centre for Cardiovascular Research (DZHK), partner site Hamburg/Kiel/Lübeck, Hamburg, Germany
Cardiovascular Regeneration Program, Centro Nacional de Investigaciones Cardiovasculares, Madrid, Spain
Florian Weinberger
You can also search for this author in PubMed Google Scholar
Contributions
T.E. drafted the manuscript. F.W. provided substantial contributions to its content. T.E. and F.W. reviewed and edited the manuscript.
Corresponding author
Correspondence to Thomas Eschenhagen .
Ethics declarations
Competing interests.
T.E. and F.W. contribute in a structured partnership between Evotec AG and the University Medical Center Hamburg-Eppendorf (UKE) to originate a pluripotent stem cell-based remuscularization approach. They have no financial interest and did not obtain consultations fees.
Peer review
Peer review information.
Nature Cardiovascular Research thanks Mauro Giacca and Richard Lee for their contribution to the peer review of this work.
Additional information
Publisher’s note Springer Nature remains neutral with regard to jurisdictional claims in published maps and institutional affiliations.
Rights and permissions
Springer Nature or its licensor (e.g. a society or other partner) holds exclusive rights to this article under a publishing agreement with the author(s) or other rightsholder(s); author self-archiving of the accepted manuscript version of this article is solely governed by the terms of such publishing agreement and applicable law.
Reprints and permissions
About this article
Cite this article.
Eschenhagen, T., Weinberger, F. Challenges and perspectives of heart repair with pluripotent stem cell-derived cardiomyocytes. Nat Cardiovasc Res 3 , 515–524 (2024). https://doi.org/10.1038/s44161-024-00472-6
Download citation
Received : 30 October 2023
Accepted : 04 April 2024
Published : 14 May 2024
Issue Date : May 2024
DOI : https://doi.org/10.1038/s44161-024-00472-6
Share this article
Anyone you share the following link with will be able to read this content:
Sorry, a shareable link is not currently available for this article.
Provided by the Springer Nature SharedIt content-sharing initiative
Quick links
- Explore articles by subject
- Guide to authors
- Editorial policies
Sign up for the Nature Briefing newsletter — what matters in science, free to your inbox daily.

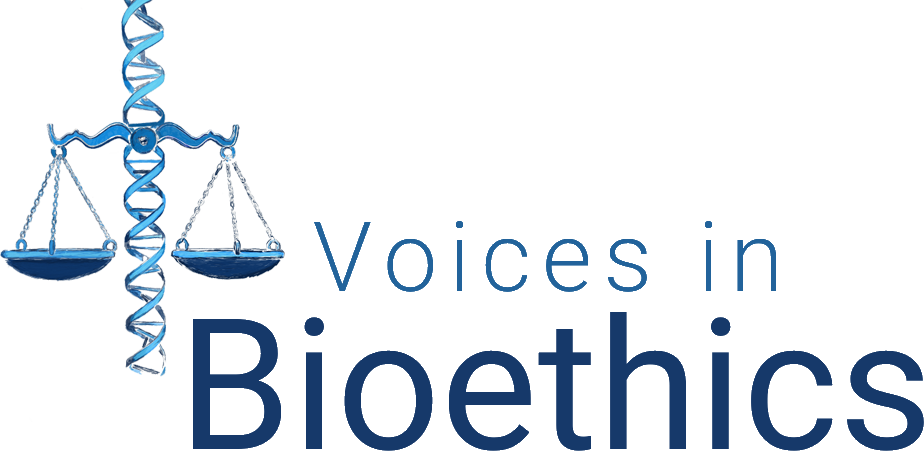
Cultural Relativity and Acceptance of Embryonic Stem Cell Research
Article sidebar.
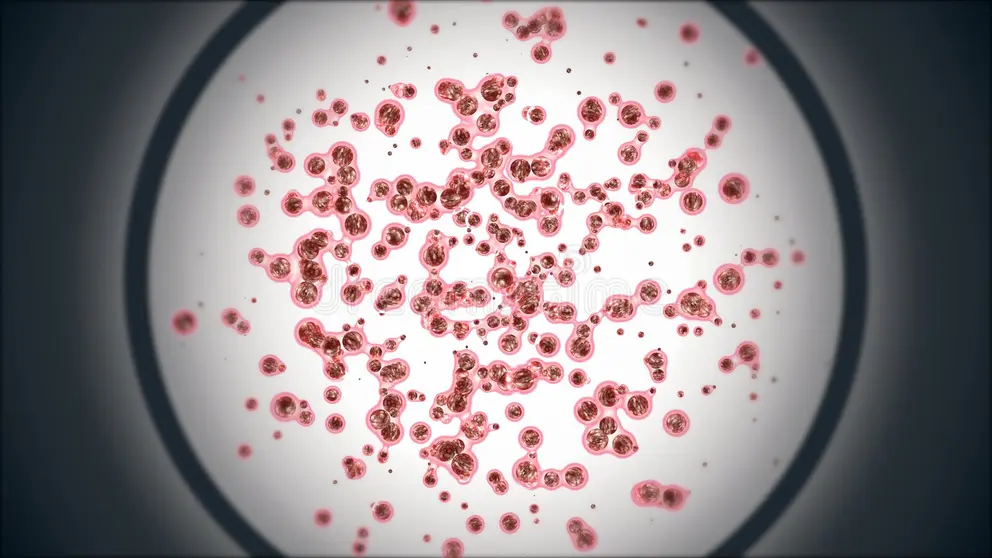
Main Article Content
There is a debate about the ethical implications of using human embryos in stem cell research, which can be influenced by cultural, moral, and social values. This paper argues for an adaptable framework to accommodate diverse cultural and religious perspectives. By using an adaptive ethics model, research protections can reflect various populations and foster growth in stem cell research possibilities.
INTRODUCTION
Stem cell research combines biology, medicine, and technology, promising to alter health care and the understanding of human development. Yet, ethical contention exists because of individuals’ perceptions of using human embryos based on their various cultural, moral, and social values. While these disagreements concerning policy, use, and general acceptance have prompted the development of an international ethics policy, such a uniform approach can overlook the nuanced ethical landscapes between cultures. With diverse viewpoints in public health, a single global policy, especially one reflecting Western ethics or the ethics prevalent in high-income countries, is impractical. This paper argues for a culturally sensitive, adaptable framework for the use of embryonic stem cells. Stem cell policy should accommodate varying ethical viewpoints and promote an effective global dialogue. With an extension of an ethics model that can adapt to various cultures, we recommend localized guidelines that reflect the moral views of the people those guidelines serve.
Stem cells, characterized by their unique ability to differentiate into various cell types, enable the repair or replacement of damaged tissues. Two primary types of stem cells are somatic stem cells (adult stem cells) and embryonic stem cells. Adult stem cells exist in developed tissues and maintain the body’s repair processes. [1] Embryonic stem cells (ESC) are remarkably pluripotent or versatile, making them valuable in research. [2] However, the use of ESCs has sparked ethics debates. Considering the potential of embryonic stem cells, research guidelines are essential. The International Society for Stem Cell Research (ISSCR) provides international stem cell research guidelines. They call for “public conversations touching on the scientific significance as well as the societal and ethical issues raised by ESC research.” [3] The ISSCR also publishes updates about culturing human embryos 14 days post fertilization, suggesting local policies and regulations should continue to evolve as ESC research develops. [4] Like the ISSCR, which calls for local law and policy to adapt to developing stem cell research given cultural acceptance, this paper highlights the importance of local social factors such as religion and culture.
I. Global Cultural Perspective of Embryonic Stem Cells
Views on ESCs vary throughout the world. Some countries readily embrace stem cell research and therapies, while others have stricter regulations due to ethical concerns surrounding embryonic stem cells and when an embryo becomes entitled to moral consideration. The philosophical issue of when the “someone” begins to be a human after fertilization, in the morally relevant sense, [5] impacts when an embryo becomes not just worthy of protection but morally entitled to it. The process of creating embryonic stem cell lines involves the destruction of the embryos for research. [6] Consequently, global engagement in ESC research depends on social-cultural acceptability.
a. US and Rights-Based Cultures
In the United States, attitudes toward stem cell therapies are diverse. The ethics and social approaches, which value individualism, [7] trigger debates regarding the destruction of human embryos, creating a complex regulatory environment. For example, the 1996 Dickey-Wicker Amendment prohibited federal funding for the creation of embryos for research and the destruction of embryos for “more than allowed for research on fetuses in utero.” [8] Following suit, in 2001, the Bush Administration heavily restricted stem cell lines for research. However, the Stem Cell Research Enhancement Act of 2005 was proposed to help develop ESC research but was ultimately vetoed. [9] Under the Obama administration, in 2009, an executive order lifted restrictions allowing for more development in this field. [10] The flux of research capacity and funding parallels the different cultural perceptions of human dignity of the embryo and how it is socially presented within the country’s research culture. [11]
b. Ubuntu and Collective Cultures
African bioethics differs from Western individualism because of the different traditions and values. African traditions, as described by individuals from South Africa and supported by some studies in other African countries, including Ghana and Kenya, follow the African moral philosophies of Ubuntu or Botho and Ukama , which “advocates for a form of wholeness that comes through one’s relationship and connectedness with other people in the society,” [12] making autonomy a socially collective concept. In this context, for the community to act autonomously, individuals would come together to decide what is best for the collective. Thus, stem cell research would require examining the value of the research to society as a whole and the use of the embryos as a collective societal resource. If society views the source as part of the collective whole, and opposes using stem cells, compromising the cultural values to pursue research may cause social detachment and stunt research growth. [13] Based on local culture and moral philosophy, the permissibility of stem cell research depends on how embryo, stem cell, and cell line therapies relate to the community as a whole . Ubuntu is the expression of humanness, with the person’s identity drawn from the “’I am because we are’” value. [14] The decision in a collectivistic culture becomes one born of cultural context, and individual decisions give deference to others in the society.
Consent differs in cultures where thought and moral philosophy are based on a collective paradigm. So, applying Western bioethical concepts is unrealistic. For one, Africa is a diverse continent with many countries with different belief systems, access to health care, and reliance on traditional or Western medicines. Where traditional medicine is the primary treatment, the “’restrictive focus on biomedically-related bioethics’” [is] problematic in African contexts because it neglects bioethical issues raised by traditional systems.” [15] No single approach applies in all areas or contexts. Rather than evaluating the permissibility of ESC research according to Western concepts such as the four principles approach, different ethics approaches should prevail.
Another consideration is the socio-economic standing of countries. In parts of South Africa, researchers have not focused heavily on contributing to the stem cell discourse, either because it is not considered health care or a health science priority or because resources are unavailable. [16] Each country’s priorities differ given different social, political, and economic factors. In South Africa, for instance, areas such as maternal mortality, non-communicable diseases, telemedicine, and the strength of health systems need improvement and require more focus. [17] Stem cell research could benefit the population, but it also could divert resources from basic medical care. Researchers in South Africa adhere to the National Health Act and Medicines Control Act in South Africa and international guidelines; however, the Act is not strictly enforced, and there is no clear legislation for research conduct or ethical guidelines. [18]
Some parts of Africa condemn stem cell research. For example, 98.2 percent of the Tunisian population is Muslim. [19] Tunisia does not permit stem cell research because of moral conflict with a Fatwa. Religion heavily saturates the regulation and direction of research. [20] Stem cell use became permissible for reproductive purposes only recently, with tight restrictions preventing cells from being used in any research other than procedures concerning ART/IVF. Their use is conditioned on consent, and available only to married couples. [21] The community's receptiveness to stem cell research depends on including communitarian African ethics.
c. Asia
Some Asian countries also have a collective model of ethics and decision making. [22] In China, the ethics model promotes a sincere respect for life or human dignity, [23] based on protective medicine. This model, influenced by Traditional Chinese Medicine (TCM), [24] recognizes Qi as the vital energy delivered via the meridians of the body; it connects illness to body systems, the body’s entire constitution, and the universe for a holistic bond of nature, health, and quality of life. [25] Following a protective ethics model, and traditional customs of wholeness, investment in stem cell research is heavily desired for its applications in regenerative therapies, disease modeling, and protective medicines. In a survey of medical students and healthcare practitioners, 30.8 percent considered stem cell research morally unacceptable while 63.5 percent accepted medical research using human embryonic stem cells. Of these individuals, 89.9 percent supported increased funding for stem cell research. [26] The scientific community might not reflect the overall population. From 1997 to 2019, China spent a total of $576 million (USD) on stem cell research at 8,050 stem cell programs, increased published presence from 0.6 percent to 14.01 percent of total global stem cell publications as of 2014, and made significant strides in cell-based therapies for various medical conditions. [27] However, while China has made substantial investments in stem cell research and achieved notable progress in clinical applications, concerns linger regarding ethical oversight and transparency. [28] For example, the China Biosecurity Law, promoted by the National Health Commission and China Hospital Association, attempted to mitigate risks by introducing an institutional review board (IRB) in the regulatory bodies. 5800 IRBs registered with the Chinese Clinical Trial Registry since 2021. [29] However, issues still need to be addressed in implementing effective IRB review and approval procedures.
The substantial government funding and focus on scientific advancement have sometimes overshadowed considerations of regional cultures, ethnic minorities, and individual perspectives, particularly evident during the one-child policy era. As government policy adapts to promote public stability, such as the change from the one-child to the two-child policy, [30] research ethics should also adapt to ensure respect for the values of its represented peoples.
Japan is also relatively supportive of stem cell research and therapies. Japan has a more transparent regulatory framework, allowing for faster approval of regenerative medicine products, which has led to several advanced clinical trials and therapies. [31] South Korea is also actively engaged in stem cell research and has a history of breakthroughs in cloning and embryonic stem cells. [32] However, the field is controversial, and there are issues of scientific integrity. For example, the Korean FDA fast-tracked products for approval, [33] and in another instance, the oocyte source was unclear and possibly violated ethical standards. [34] Trust is important in research, as it builds collaborative foundations between colleagues, trial participant comfort, open-mindedness for complicated and sensitive discussions, and supports regulatory procedures for stakeholders. There is a need to respect the culture’s interest, engagement, and for research and clinical trials to be transparent and have ethical oversight to promote global research discourse and trust.
d. Middle East
Countries in the Middle East have varying degrees of acceptance of or restrictions to policies related to using embryonic stem cells due to cultural and religious influences. Saudi Arabia has made significant contributions to stem cell research, and conducts research based on international guidelines for ethical conduct and under strict adherence to guidelines in accordance with Islamic principles. Specifically, the Saudi government and people require ESC research to adhere to Sharia law. In addition to umbilical and placental stem cells, [35] Saudi Arabia permits the use of embryonic stem cells as long as they come from miscarriages, therapeutic abortions permissible by Sharia law, or are left over from in vitro fertilization and donated to research. [36] Laws and ethical guidelines for stem cell research allow the development of research institutions such as the King Abdullah International Medical Research Center, which has a cord blood bank and a stem cell registry with nearly 10,000 donors. [37] Such volume and acceptance are due to the ethical ‘permissibility’ of the donor sources, which do not conflict with religious pillars. However, some researchers err on the side of caution, choosing not to use embryos or fetal tissue as they feel it is unethical to do so. [38]
Jordan has a positive research ethics culture. [39] However, there is a significant issue of lack of trust in researchers, with 45.23 percent (38.66 percent agreeing and 6.57 percent strongly agreeing) of Jordanians holding a low level of trust in researchers, compared to 81.34 percent of Jordanians agreeing that they feel safe to participate in a research trial. [40] Safety testifies to the feeling of confidence that adequate measures are in place to protect participants from harm, whereas trust in researchers could represent the confidence in researchers to act in the participants’ best interests, adhere to ethical guidelines, provide accurate information, and respect participants’ rights and dignity. One method to improve trust would be to address communication issues relevant to ESC. Legislation surrounding stem cell research has adopted specific language, especially concerning clarification “between ‘stem cells’ and ‘embryonic stem cells’” in translation. [41] Furthermore, legislation “mandates the creation of a national committee… laying out specific regulations for stem-cell banking in accordance with international standards.” [42] This broad regulation opens the door for future global engagement and maintains transparency. However, these regulations may also constrain the influence of research direction, pace, and accessibility of research outcomes.
e. Europe
In the European Union (EU), ethics is also principle-based, but the principles of autonomy, dignity, integrity, and vulnerability are interconnected. [43] As such, the opportunity for cohesion and concessions between individuals’ thoughts and ideals allows for a more adaptable ethics model due to the flexible principles that relate to the human experience The EU has put forth a framework in its Convention for the Protection of Human Rights and Dignity of the Human Being allowing member states to take different approaches. Each European state applies these principles to its specific conventions, leading to or reflecting different acceptance levels of stem cell research. [44]
For example, in Germany, Lebenzusammenhang , or the coherence of life, references integrity in the unity of human culture. Namely, the personal sphere “should not be subject to external intervention.” [45] Stem cell interventions could affect this concept of bodily completeness, leading to heavy restrictions. Under the Grundgesetz, human dignity and the right to life with physical integrity are paramount. [46] The Embryo Protection Act of 1991 made producing cell lines illegal. Cell lines can be imported if approved by the Central Ethics Commission for Stem Cell Research only if they were derived before May 2007. [47] Stem cell research respects the integrity of life for the embryo with heavy specifications and intense oversight. This is vastly different in Finland, where the regulatory bodies find research more permissible in IVF excess, but only up to 14 days after fertilization. [48] Spain’s approach differs still, with a comprehensive regulatory framework. [49] Thus, research regulation can be culture-specific due to variations in applied principles. Diverse cultures call for various approaches to ethical permissibility. [50] Only an adaptive-deliberative model can address the cultural constructions of self and achieve positive, culturally sensitive stem cell research practices. [51]
II. Religious Perspectives on ESC
Embryonic stem cell sources are the main consideration within religious contexts. While individuals may not regard their own religious texts as authoritative or factual, religion can shape their foundations or perspectives.
The Qur'an states:
“And indeed We created man from a quintessence of clay. Then We placed within him a small quantity of nutfa (sperm to fertilize) in a safe place. Then We have fashioned the nutfa into an ‘alaqa (clinging clot or cell cluster), then We developed the ‘alaqa into mudgha (a lump of flesh), and We made mudgha into bones, and clothed the bones with flesh, then We brought it into being as a new creation. So Blessed is Allah, the Best of Creators.” [52]
Many scholars of Islam estimate the time of soul installment, marked by the angel breathing in the soul to bring the individual into creation, as 120 days from conception. [53] Personhood begins at this point, and the value of life would prohibit research or experimentation that could harm the individual. If the fetus is more than 120 days old, the time ensoulment is interpreted to occur according to Islamic law, abortion is no longer permissible. [54] There are a few opposing opinions about early embryos in Islamic traditions. According to some Islamic theologians, there is no ensoulment of the early embryo, which is the source of stem cells for ESC research. [55]
In Buddhism, the stance on stem cell research is not settled. The main tenets, the prohibition against harming or destroying others (ahimsa) and the pursuit of knowledge (prajña) and compassion (karuna), leave Buddhist scholars and communities divided. [56] Some scholars argue stem cell research is in accordance with the Buddhist tenet of seeking knowledge and ending human suffering. Others feel it violates the principle of not harming others. Finding the balance between these two points relies on the karmic burden of Buddhist morality. In trying to prevent ahimsa towards the embryo, Buddhist scholars suggest that to comply with Buddhist tenets, research cannot be done as the embryo has personhood at the moment of conception and would reincarnate immediately, harming the individual's ability to build their karmic burden. [57] On the other hand, the Bodhisattvas, those considered to be on the path to enlightenment or Nirvana, have given organs and flesh to others to help alleviate grieving and to benefit all. [58] Acceptance varies on applied beliefs and interpretations.
Catholicism does not support embryonic stem cell research, as it entails creation or destruction of human embryos. This destruction conflicts with the belief in the sanctity of life. For example, in the Old Testament, Genesis describes humanity as being created in God’s image and multiplying on the Earth, referencing the sacred rights to human conception and the purpose of development and life. In the Ten Commandments, the tenet that one should not kill has numerous interpretations where killing could mean murder or shedding of the sanctity of life, demonstrating the high value of human personhood. In other books, the theological conception of when life begins is interpreted as in utero, [59] highlighting the inviolability of life and its formation in vivo to make a religious point for accepting such research as relatively limited, if at all. [60] The Vatican has released ethical directives to help apply a theological basis to modern-day conflicts. The Magisterium of the Church states that “unless there is a moral certainty of not causing harm,” experimentation on fetuses, fertilized cells, stem cells, or embryos constitutes a crime. [61] Such procedures would not respect the human person who exists at these stages, according to Catholicism. Damages to the embryo are considered gravely immoral and illicit. [62] Although the Catholic Church officially opposes abortion, surveys demonstrate that many Catholic people hold pro-choice views, whether due to the context of conception, stage of pregnancy, threat to the mother’s life, or for other reasons, demonstrating that practicing members can also accept some but not all tenets. [63]
Some major Jewish denominations, such as the Reform, Conservative, and Reconstructionist movements, are open to supporting ESC use or research as long as it is for saving a life. [64] Within Judaism, the Talmud, or study, gives personhood to the child at birth and emphasizes that life does not begin at conception: [65]
“If she is found pregnant, until the fortieth day it is mere fluid,” [66]
Whereas most religions prioritize the status of human embryos, the Halakah (Jewish religious law) states that to save one life, most other religious laws can be ignored because it is in pursuit of preservation. [67] Stem cell research is accepted due to application of these religious laws.
We recognize that all religions contain subsets and sects. The variety of environmental and cultural differences within religious groups requires further analysis to respect the flexibility of religious thoughts and practices. We make no presumptions that all cultures require notions of autonomy or morality as under the common morality theory , which asserts a set of universal moral norms that all individuals share provides moral reasoning and guides ethical decisions. [68] We only wish to show that the interaction with morality varies between cultures and countries.
III. A Flexible Ethical Approach
The plurality of different moral approaches described above demonstrates that there can be no universally acceptable uniform law for ESC on a global scale. Instead of developing one standard, flexible ethical applications must be continued. We recommend local guidelines that incorporate important cultural and ethical priorities.
While the Declaration of Helsinki is more relevant to people in clinical trials receiving ESC products, in keeping with the tradition of protections for research subjects, consent of the donor is an ethical requirement for ESC donation in many jurisdictions including the US, Canada, and Europe. [69] The Declaration of Helsinki provides a reference point for regulatory standards and could potentially be used as a universal baseline for obtaining consent prior to gamete or embryo donation.
For instance, in Columbia University’s egg donor program for stem cell research, donors followed standard screening protocols and “underwent counseling sessions that included information as to the purpose of oocyte donation for research, what the oocytes would be used for, the risks and benefits of donation, and process of oocyte stimulation” to ensure transparency for consent. [70] The program helped advance stem cell research and provided clear and safe research methods with paid participants. Though paid participation or covering costs of incidental expenses may not be socially acceptable in every culture or context, [71] and creating embryos for ESC research is illegal in many jurisdictions, Columbia’s program was effective because of the clear and honest communications with donors, IRBs, and related stakeholders. This example demonstrates that cultural acceptance of scientific research and of the idea that an egg or embryo does not have personhood is likely behind societal acceptance of donating eggs for ESC research. As noted, many countries do not permit the creation of embryos for research.
Proper communication and education regarding the process and purpose of stem cell research may bolster comprehension and garner more acceptance. “Given the sensitive subject material, a complete consent process can support voluntary participation through trust, understanding, and ethical norms from the cultures and morals participants value. This can be hard for researchers entering countries of different socioeconomic stability, with different languages and different societal values. [72]
An adequate moral foundation in medical ethics is derived from the cultural and religious basis that informs knowledge and actions. [73] Understanding local cultural and religious values and their impact on research could help researchers develop humility and promote inclusion.
IV. Concerns
Some may argue that if researchers all adhere to one ethics standard, protection will be satisfied across all borders, and the global public will trust researchers. However, defining what needs to be protected and how to define such research standards is very specific to the people to which standards are applied. We suggest that applying one uniform guide cannot accurately protect each individual because we all possess our own perceptions and interpretations of social values. [74] Therefore, the issue of not adjusting to the moral pluralism between peoples in applying one standard of ethics can be resolved by building out ethics models that can be adapted to different cultures and religions.
Other concerns include medical tourism, which may promote health inequities. [75] Some countries may develop and approve products derived from ESC research before others, compromising research ethics or drug approval processes. There are also concerns about the sale of unauthorized stem cell treatments, for example, those without FDA approval in the United States. Countries with robust research infrastructures may be tempted to attract medical tourists, and some customers will have false hopes based on aggressive publicity of unproven treatments. [76]
For example, in China, stem cell clinics can market to foreign clients who are not protected under the regulatory regimes. Companies employ a marketing strategy of “ethically friendly” therapies. Specifically, in the case of Beike, China’s leading stem cell tourism company and sprouting network, ethical oversight of administrators or health bureaus at one site has “the unintended consequence of shifting questionable activities to another node in Beike's diffuse network.” [77] In contrast, Jordan is aware of stem cell research’s potential abuse and its own status as a “health-care hub.” Jordan’s expanded regulations include preserving the interests of individuals in clinical trials and banning private companies from ESC research to preserve transparency and the integrity of research practices. [78]
The social priorities of the community are also a concern. The ISSCR explicitly states that guidelines “should be periodically revised to accommodate scientific advances, new challenges, and evolving social priorities.” [79] The adaptable ethics model extends this consideration further by addressing whether research is warranted given the varying degrees of socioeconomic conditions, political stability, and healthcare accessibilities and limitations. An ethical approach would require discussion about resource allocation and appropriate distribution of funds. [80]
While some religions emphasize the sanctity of life from conception, which may lead to public opposition to ESC research, others encourage ESC research due to its potential for healing and alleviating human pain. Many countries have special regulations that balance local views on embryonic personhood, the benefits of research as individual or societal goods, and the protection of human research subjects. To foster understanding and constructive dialogue, global policy frameworks should prioritize the protection of universal human rights, transparency, and informed consent. In addition to these foundational global policies, we recommend tailoring local guidelines to reflect the diverse cultural and religious perspectives of the populations they govern. Ethics models should be adapted to local populations to effectively establish research protections, growth, and possibilities of stem cell research.
For example, in countries with strong beliefs in the moral sanctity of embryos or heavy religious restrictions, an adaptive model can allow for discussion instead of immediate rejection. In countries with limited individual rights and voice in science policy, an adaptive model ensures cultural, moral, and religious views are taken into consideration, thereby building social inclusion. While this ethical consideration by the government may not give a complete voice to every individual, it will help balance policies and maintain the diverse perspectives of those it affects. Embracing an adaptive ethics model of ESC research promotes open-minded dialogue and respect for the importance of human belief and tradition. By actively engaging with cultural and religious values, researchers can better handle disagreements and promote ethical research practices that benefit each society.
This brief exploration of the religious and cultural differences that impact ESC research reveals the nuances of relative ethics and highlights a need for local policymakers to apply a more intense adaptive model.
[1] Poliwoda, S., Noor, N., Downs, E., Schaaf, A., Cantwell, A., Ganti, L., Kaye, A. D., Mosel, L. I., Carroll, C. B., Viswanath, O., & Urits, I. (2022). Stem cells: a comprehensive review of origins and emerging clinical roles in medical practice. Orthopedic reviews , 14 (3), 37498. https://doi.org/10.52965/001c.37498
[2] Poliwoda, S., Noor, N., Downs, E., Schaaf, A., Cantwell, A., Ganti, L., Kaye, A. D., Mosel, L. I., Carroll, C. B., Viswanath, O., & Urits, I. (2022). Stem cells: a comprehensive review of origins and emerging clinical roles in medical practice. Orthopedic reviews , 14 (3), 37498. https://doi.org/10.52965/001c.37498
[3] International Society for Stem Cell Research. (2023). Laboratory-based human embryonic stem cell research, embryo research, and related research activities . International Society for Stem Cell Research. https://www.isscr.org/guidelines/blog-post-title-one-ed2td-6fcdk ; Kimmelman, J., Hyun, I., Benvenisty, N. et al. Policy: Global standards for stem-cell research. Nature 533 , 311–313 (2016). https://doi.org/10.1038/533311a
[4] International Society for Stem Cell Research. (2023). Laboratory-based human embryonic stem cell research, embryo research, and related research activities . International Society for Stem Cell Research. https://www.isscr.org/guidelines/blog-post-title-one-ed2td-6fcdk
[5] Concerning the moral philosophies of stem cell research, our paper does not posit a personal moral stance nor delve into the “when” of human life begins. To read further about the philosophical debate, consider the following sources:
Sandel M. J. (2004). Embryo ethics--the moral logic of stem-cell research. The New England journal of medicine , 351 (3), 207–209. https://doi.org/10.1056/NEJMp048145 ; George, R. P., & Lee, P. (2020, September 26). Acorns and Embryos . The New Atlantis. https://www.thenewatlantis.com/publications/acorns-and-embryos ; Sagan, A., & Singer, P. (2007). The moral status of stem cells. Metaphilosophy , 38 (2/3), 264–284. http://www.jstor.org/stable/24439776 ; McHugh P. R. (2004). Zygote and "clonote"--the ethical use of embryonic stem cells. The New England journal of medicine , 351 (3), 209–211. https://doi.org/10.1056/NEJMp048147 ; Kurjak, A., & Tripalo, A. (2004). The facts and doubts about beginning of the human life and personality. Bosnian journal of basic medical sciences , 4 (1), 5–14. https://doi.org/10.17305/bjbms.2004.3453
[6] Vazin, T., & Freed, W. J. (2010). Human embryonic stem cells: derivation, culture, and differentiation: a review. Restorative neurology and neuroscience , 28 (4), 589–603. https://doi.org/10.3233/RNN-2010-0543
[7] Socially, at its core, the Western approach to ethics is widely principle-based, autonomy being one of the key factors to ensure a fundamental respect for persons within research. For information regarding autonomy in research, see: Department of Health, Education, and Welfare, & National Commission for the Protection of Human Subjects of Biomedical and Behavioral Research (1978). The Belmont Report. Ethical principles and guidelines for the protection of human subjects of research.; For a more in-depth review of autonomy within the US, see: Beauchamp, T. L., & Childress, J. F. (1994). Principles of Biomedical Ethics . Oxford University Press.
[8] Sherley v. Sebelius , 644 F.3d 388 (D.C. Cir. 2011), citing 45 C.F.R. 46.204(b) and [42 U.S.C. § 289g(b)]. https://www.cadc.uscourts.gov/internet/opinions.nsf/6c690438a9b43dd685257a64004ebf99/$file/11-5241-1391178.pdf
[9] Stem Cell Research Enhancement Act of 2005, H. R. 810, 109 th Cong. (2001). https://www.govtrack.us/congress/bills/109/hr810/text ; Bush, G. W. (2006, July 19). Message to the House of Representatives . National Archives and Records Administration. https://georgewbush-whitehouse.archives.gov/news/releases/2006/07/20060719-5.html
[10] National Archives and Records Administration. (2009, March 9). Executive order 13505 -- removing barriers to responsible scientific research involving human stem cells . National Archives and Records Administration. https://obamawhitehouse.archives.gov/the-press-office/removing-barriers-responsible-scientific-research-involving-human-stem-cells
[11] Hurlbut, W. B. (2006). Science, Religion, and the Politics of Stem Cells. Social Research , 73 (3), 819–834. http://www.jstor.org/stable/40971854
[12] Akpa-Inyang, Francis & Chima, Sylvester. (2021). South African traditional values and beliefs regarding informed consent and limitations of the principle of respect for autonomy in African communities: a cross-cultural qualitative study. BMC Medical Ethics . 22. 10.1186/s12910-021-00678-4.
[13] Source for further reading: Tangwa G. B. (2007). Moral status of embryonic stem cells: perspective of an African villager. Bioethics , 21(8), 449–457. https://doi.org/10.1111/j.1467-8519.2007.00582.x , see also Mnisi, F. M. (2020). An African analysis based on ethics of Ubuntu - are human embryonic stem cell patents morally justifiable? African Insight , 49 (4).
[14] Jecker, N. S., & Atuire, C. (2021). Bioethics in Africa: A contextually enlightened analysis of three cases. Developing World Bioethics , 22 (2), 112–122. https://doi.org/10.1111/dewb.12324
[15] Jecker, N. S., & Atuire, C. (2021). Bioethics in Africa: A contextually enlightened analysis of three cases. Developing World Bioethics, 22(2), 112–122. https://doi.org/10.1111/dewb.12324
[16] Jackson, C.S., Pepper, M.S. Opportunities and barriers to establishing a cell therapy programme in South Africa. Stem Cell Res Ther 4 , 54 (2013). https://doi.org/10.1186/scrt204 ; Pew Research Center. (2014, May 1). Public health a major priority in African nations . Pew Research Center’s Global Attitudes Project. https://www.pewresearch.org/global/2014/05/01/public-health-a-major-priority-in-african-nations/
[17] Department of Health Republic of South Africa. (2021). Health Research Priorities (revised) for South Africa 2021-2024 . National Health Research Strategy. https://www.health.gov.za/wp-content/uploads/2022/05/National-Health-Research-Priorities-2021-2024.pdf
[18] Oosthuizen, H. (2013). Legal and Ethical Issues in Stem Cell Research in South Africa. In: Beran, R. (eds) Legal and Forensic Medicine. Springer, Berlin, Heidelberg. https://doi.org/10.1007/978-3-642-32338-6_80 , see also: Gaobotse G (2018) Stem Cell Research in Africa: Legislation and Challenges. J Regen Med 7:1. doi: 10.4172/2325-9620.1000142
[19] United States Bureau of Citizenship and Immigration Services. (1998). Tunisia: Information on the status of Christian conversions in Tunisia . UNHCR Web Archive. https://webarchive.archive.unhcr.org/20230522142618/https://www.refworld.org/docid/3df0be9a2.html
[20] Gaobotse, G. (2018) Stem Cell Research in Africa: Legislation and Challenges. J Regen Med 7:1. doi: 10.4172/2325-9620.1000142
[21] Kooli, C. Review of assisted reproduction techniques, laws, and regulations in Muslim countries. Middle East Fertil Soc J 24 , 8 (2020). https://doi.org/10.1186/s43043-019-0011-0 ; Gaobotse, G. (2018) Stem Cell Research in Africa: Legislation and Challenges. J Regen Med 7:1. doi: 10.4172/2325-9620.1000142
[22] Pang M. C. (1999). Protective truthfulness: the Chinese way of safeguarding patients in informed treatment decisions. Journal of medical ethics , 25(3), 247–253. https://doi.org/10.1136/jme.25.3.247
[23] Wang, L., Wang, F., & Zhang, W. (2021). Bioethics in China’s biosecurity law: Forms, effects, and unsettled issues. Journal of law and the biosciences , 8(1). https://doi.org/10.1093/jlb/lsab019 https://academic.oup.com/jlb/article/8/1/lsab019/6299199
[24] Wang, Y., Xue, Y., & Guo, H. D. (2022). Intervention effects of traditional Chinese medicine on stem cell therapy of myocardial infarction. Frontiers in pharmacology , 13 , 1013740. https://doi.org/10.3389/fphar.2022.1013740
[25] Li, X.-T., & Zhao, J. (2012). Chapter 4: An Approach to the Nature of Qi in TCM- Qi and Bioenergy. In Recent Advances in Theories and Practice of Chinese Medicine (p. 79). InTech.
[26] Luo, D., Xu, Z., Wang, Z., & Ran, W. (2021). China's Stem Cell Research and Knowledge Levels of Medical Practitioners and Students. Stem cells international , 2021 , 6667743. https://doi.org/10.1155/2021/6667743
[27] Luo, D., Xu, Z., Wang, Z., & Ran, W. (2021). China's Stem Cell Research and Knowledge Levels of Medical Practitioners and Students. Stem cells international , 2021 , 6667743. https://doi.org/10.1155/2021/6667743
[28] Zhang, J. Y. (2017). Lost in translation? accountability and governance of Clinical Stem Cell Research in China. Regenerative Medicine , 12 (6), 647–656. https://doi.org/10.2217/rme-2017-0035
[29] Wang, L., Wang, F., & Zhang, W. (2021). Bioethics in China’s biosecurity law: Forms, effects, and unsettled issues. Journal of law and the biosciences , 8(1). https://doi.org/10.1093/jlb/lsab019 https://academic.oup.com/jlb/article/8/1/lsab019/6299199
[30] Chen, H., Wei, T., Wang, H. et al. Association of China’s two-child policy with changes in number of births and birth defects rate, 2008–2017. BMC Public Health 22 , 434 (2022). https://doi.org/10.1186/s12889-022-12839-0
[31] Azuma, K. Regulatory Landscape of Regenerative Medicine in Japan. Curr Stem Cell Rep 1 , 118–128 (2015). https://doi.org/10.1007/s40778-015-0012-6
[32] Harris, R. (2005, May 19). Researchers Report Advance in Stem Cell Production . NPR. https://www.npr.org/2005/05/19/4658967/researchers-report-advance-in-stem-cell-production
[33] Park, S. (2012). South Korea steps up stem-cell work. Nature . https://doi.org/10.1038/nature.2012.10565
[34] Resnik, D. B., Shamoo, A. E., & Krimsky, S. (2006). Fraudulent human embryonic stem cell research in South Korea: lessons learned. Accountability in research , 13 (1), 101–109. https://doi.org/10.1080/08989620600634193 .
[35] Alahmad, G., Aljohani, S., & Najjar, M. F. (2020). Ethical challenges regarding the use of stem cells: interviews with researchers from Saudi Arabia. BMC medical ethics, 21(1), 35. https://doi.org/10.1186/s12910-020-00482-6
[36] Association for the Advancement of Blood and Biotherapies. https://www.aabb.org/regulatory-and-advocacy/regulatory-affairs/regulatory-for-cellular-therapies/international-competent-authorities/saudi-arabia
[37] Alahmad, G., Aljohani, S., & Najjar, M. F. (2020). Ethical challenges regarding the use of stem cells: Interviews with researchers from Saudi Arabia. BMC medical ethics , 21 (1), 35. https://doi.org/10.1186/s12910-020-00482-6
[38] Alahmad, G., Aljohani, S., & Najjar, M. F. (2020). Ethical challenges regarding the use of stem cells: Interviews with researchers from Saudi Arabia. BMC medical ethics , 21(1), 35. https://doi.org/10.1186/s12910-020-00482-6
Culturally, autonomy practices follow a relational autonomy approach based on a paternalistic deontological health care model. The adherence to strict international research policies and religious pillars within the regulatory environment is a great foundation for research ethics. However, there is a need to develop locally targeted ethics approaches for research (as called for in Alahmad, G., Aljohani, S., & Najjar, M. F. (2020). Ethical challenges regarding the use of stem cells: interviews with researchers from Saudi Arabia. BMC medical ethics, 21(1), 35. https://doi.org/10.1186/s12910-020-00482-6), this decision-making approach may help advise a research decision model. For more on the clinical cultural autonomy approaches, see: Alabdullah, Y. Y., Alzaid, E., Alsaad, S., Alamri, T., Alolayan, S. W., Bah, S., & Aljoudi, A. S. (2022). Autonomy and paternalism in Shared decision‐making in a Saudi Arabian tertiary hospital: A cross‐sectional study. Developing World Bioethics , 23 (3), 260–268. https://doi.org/10.1111/dewb.12355 ; Bukhari, A. A. (2017). Universal Principles of Bioethics and Patient Rights in Saudi Arabia (Doctoral dissertation, Duquesne University). https://dsc.duq.edu/etd/124; Ladha, S., Nakshawani, S. A., Alzaidy, A., & Tarab, B. (2023, October 26). Islam and Bioethics: What We All Need to Know . Columbia University School of Professional Studies. https://sps.columbia.edu/events/islam-and-bioethics-what-we-all-need-know
[39] Ababneh, M. A., Al-Azzam, S. I., Alzoubi, K., Rababa’h, A., & Al Demour, S. (2021). Understanding and attitudes of the Jordanian public about clinical research ethics. Research Ethics , 17 (2), 228-241. https://doi.org/10.1177/1747016120966779
[40] Ababneh, M. A., Al-Azzam, S. I., Alzoubi, K., Rababa’h, A., & Al Demour, S. (2021). Understanding and attitudes of the Jordanian public about clinical research ethics. Research Ethics , 17 (2), 228-241. https://doi.org/10.1177/1747016120966779
[41] Dajani, R. (2014). Jordan’s stem-cell law can guide the Middle East. Nature 510, 189. https://doi.org/10.1038/510189a
[42] Dajani, R. (2014). Jordan’s stem-cell law can guide the Middle East. Nature 510, 189. https://doi.org/10.1038/510189a
[43] The EU’s definition of autonomy relates to the capacity for creating ideas, moral insight, decisions, and actions without constraint, personal responsibility, and informed consent. However, the EU views autonomy as not completely able to protect individuals and depends on other principles, such as dignity, which “expresses the intrinsic worth and fundamental equality of all human beings.” Rendtorff, J.D., Kemp, P. (2019). Four Ethical Principles in European Bioethics and Biolaw: Autonomy, Dignity, Integrity and Vulnerability. In: Valdés, E., Lecaros, J. (eds) Biolaw and Policy in the Twenty-First Century. International Library of Ethics, Law, and the New Medicine, vol 78. Springer, Cham. https://doi.org/10.1007/978-3-030-05903-3_3
[44] Council of Europe. Convention for the protection of Human Rights and Dignity of the Human Being with regard to the Application of Biology and Medicine: Convention on Human Rights and Biomedicine (ETS No. 164) https://www.coe.int/en/web/conventions/full-list?module=treaty-detail&treatynum=164 (forbidding the creation of embryos for research purposes only, and suggests embryos in vitro have protections.); Also see Drabiak-Syed B. K. (2013). New President, New Human Embryonic Stem Cell Research Policy: Comparative International Perspectives and Embryonic Stem Cell Research Laws in France. Biotechnology Law Report , 32 (6), 349–356. https://doi.org/10.1089/blr.2013.9865
[45] Rendtorff, J.D., Kemp, P. (2019). Four Ethical Principles in European Bioethics and Biolaw: Autonomy, Dignity, Integrity and Vulnerability. In: Valdés, E., Lecaros, J. (eds) Biolaw and Policy in the Twenty-First Century. International Library of Ethics, Law, and the New Medicine, vol 78. Springer, Cham. https://doi.org/10.1007/978-3-030-05903-3_3
[46] Tomuschat, C., Currie, D. P., Kommers, D. P., & Kerr, R. (Trans.). (1949, May 23). Basic law for the Federal Republic of Germany. https://www.btg-bestellservice.de/pdf/80201000.pdf
[47] Regulation of Stem Cell Research in Germany . Eurostemcell. (2017, April 26). https://www.eurostemcell.org/regulation-stem-cell-research-germany
[48] Regulation of Stem Cell Research in Finland . Eurostemcell. (2017, April 26). https://www.eurostemcell.org/regulation-stem-cell-research-finland
[49] Regulation of Stem Cell Research in Spain . Eurostemcell. (2017, April 26). https://www.eurostemcell.org/regulation-stem-cell-research-spain
[50] Some sources to consider regarding ethics models or regulatory oversights of other cultures not covered:
Kara MA. Applicability of the principle of respect for autonomy: the perspective of Turkey. J Med Ethics. 2007 Nov;33(11):627-30. doi: 10.1136/jme.2006.017400. PMID: 17971462; PMCID: PMC2598110.
Ugarte, O. N., & Acioly, M. A. (2014). The principle of autonomy in Brazil: one needs to discuss it ... Revista do Colegio Brasileiro de Cirurgioes , 41 (5), 374–377. https://doi.org/10.1590/0100-69912014005013
Bharadwaj, A., & Glasner, P. E. (2012). Local cells, global science: The rise of embryonic stem cell research in India . Routledge.
For further research on specific European countries regarding ethical and regulatory framework, we recommend this database: Regulation of Stem Cell Research in Europe . Eurostemcell. (2017, April 26). https://www.eurostemcell.org/regulation-stem-cell-research-europe
[51] Klitzman, R. (2006). Complications of culture in obtaining informed consent. The American Journal of Bioethics, 6(1), 20–21. https://doi.org/10.1080/15265160500394671 see also: Ekmekci, P. E., & Arda, B. (2017). Interculturalism and Informed Consent: Respecting Cultural Differences without Breaching Human Rights. Cultura (Iasi, Romania) , 14 (2), 159–172.; For why trust is important in research, see also: Gray, B., Hilder, J., Macdonald, L., Tester, R., Dowell, A., & Stubbe, M. (2017). Are research ethics guidelines culturally competent? Research Ethics , 13 (1), 23-41. https://doi.org/10.1177/1747016116650235
[52] The Qur'an (M. Khattab, Trans.). (1965). Al-Mu’minun, 23: 12-14. https://quran.com/23
[53] Lenfest, Y. (2017, December 8). Islam and the beginning of human life . Bill of Health. https://blog.petrieflom.law.harvard.edu/2017/12/08/islam-and-the-beginning-of-human-life/
[54] Aksoy, S. (2005). Making regulations and drawing up legislation in Islamic countries under conditions of uncertainty, with special reference to embryonic stem cell research. Journal of Medical Ethics , 31: 399-403.; see also: Mahmoud, Azza. "Islamic Bioethics: National Regulations and Guidelines of Human Stem Cell Research in the Muslim World." Master's thesis, Chapman University, 2022. https://doi.org/10.36837/ chapman.000386
[55] Rashid, R. (2022). When does Ensoulment occur in the Human Foetus. Journal of the British Islamic Medical Association , 12 (4). ISSN 2634 8071. https://www.jbima.com/wp-content/uploads/2023/01/2-Ethics-3_-Ensoulment_Rafaqat.pdf.
[56] Sivaraman, M. & Noor, S. (2017). Ethics of embryonic stem cell research according to Buddhist, Hindu, Catholic, and Islamic religions: perspective from Malaysia. Asian Biomedicine,8(1) 43-52. https://doi.org/10.5372/1905-7415.0801.260
[57] Jafari, M., Elahi, F., Ozyurt, S. & Wrigley, T. (2007). 4. Religious Perspectives on Embryonic Stem Cell Research. In K. Monroe, R. Miller & J. Tobis (Ed.), Fundamentals of the Stem Cell Debate: The Scientific, Religious, Ethical, and Political Issues (pp. 79-94). Berkeley: University of California Press. https://escholarship.org/content/qt9rj0k7s3/qt9rj0k7s3_noSplash_f9aca2e02c3777c7fb76ea768ba458f0.pdf https://doi.org/10.1525/9780520940994-005
[58] Lecso, P. A. (1991). The Bodhisattva Ideal and Organ Transplantation. Journal of Religion and Health , 30 (1), 35–41. http://www.jstor.org/stable/27510629 ; Bodhisattva, S. (n.d.). The Key of Becoming a Bodhisattva . A Guide to the Bodhisattva Way of Life. http://www.buddhism.org/Sutras/2/BodhisattvaWay.htm
[59] There is no explicit religious reference to when life begins or how to conduct research that interacts with the concept of life. However, these are relevant verses pertaining to how the fetus is viewed. (( King James Bible . (1999). Oxford University Press. (original work published 1769))
Jerimiah 1: 5 “Before I formed thee in the belly I knew thee; and before thou camest forth out of the womb I sanctified thee…”
In prophet Jerimiah’s insight, God set him apart as a person known before childbirth, a theme carried within the Psalm of David.
Psalm 139: 13-14 “…Thou hast covered me in my mother's womb. I will praise thee; for I am fearfully and wonderfully made…”
These verses demonstrate David’s respect for God as an entity that would know of all man’s thoughts and doings even before birth.
[60] It should be noted that abortion is not supported as well.
[61] The Vatican. (1987, February 22). Instruction on Respect for Human Life in Its Origin and on the Dignity of Procreation Replies to Certain Questions of the Day . Congregation For the Doctrine of the Faith. https://www.vatican.va/roman_curia/congregations/cfaith/documents/rc_con_cfaith_doc_19870222_respect-for-human-life_en.html
[62] The Vatican. (2000, August 25). Declaration On the Production and the Scientific and Therapeutic Use of Human Embryonic Stem Cells . Pontifical Academy for Life. https://www.vatican.va/roman_curia/pontifical_academies/acdlife/documents/rc_pa_acdlife_doc_20000824_cellule-staminali_en.html ; Ohara, N. (2003). Ethical Consideration of Experimentation Using Living Human Embryos: The Catholic Church’s Position on Human Embryonic Stem Cell Research and Human Cloning. Department of Obstetrics and Gynecology . Retrieved from https://article.imrpress.com/journal/CEOG/30/2-3/pii/2003018/77-81.pdf.
[63] Smith, G. A. (2022, May 23). Like Americans overall, Catholics vary in their abortion views, with regular mass attenders most opposed . Pew Research Center. https://www.pewresearch.org/short-reads/2022/05/23/like-americans-overall-catholics-vary-in-their-abortion-views-with-regular-mass-attenders-most-opposed/
[64] Rosner, F., & Reichman, E. (2002). Embryonic stem cell research in Jewish law. Journal of halacha and contemporary society , (43), 49–68.; Jafari, M., Elahi, F., Ozyurt, S. & Wrigley, T. (2007). 4. Religious Perspectives on Embryonic Stem Cell Research. In K. Monroe, R. Miller & J. Tobis (Ed.), Fundamentals of the Stem Cell Debate: The Scientific, Religious, Ethical, and Political Issues (pp. 79-94). Berkeley: University of California Press. https://escholarship.org/content/qt9rj0k7s3/qt9rj0k7s3_noSplash_f9aca2e02c3777c7fb76ea768ba458f0.pdf https://doi.org/10.1525/9780520940994-005
[65] Schenker J. G. (2008). The beginning of human life: status of embryo. Perspectives in Halakha (Jewish Religious Law). Journal of assisted reproduction and genetics , 25 (6), 271–276. https://doi.org/10.1007/s10815-008-9221-6
[66] Ruttenberg, D. (2020, May 5). The Torah of Abortion Justice (annotated source sheet) . Sefaria. https://www.sefaria.org/sheets/234926.7?lang=bi&with=all&lang2=en
[67] Jafari, M., Elahi, F., Ozyurt, S. & Wrigley, T. (2007). 4. Religious Perspectives on Embryonic Stem Cell Research. In K. Monroe, R. Miller & J. Tobis (Ed.), Fundamentals of the Stem Cell Debate: The Scientific, Religious, Ethical, and Political Issues (pp. 79-94). Berkeley: University of California Press. https://escholarship.org/content/qt9rj0k7s3/qt9rj0k7s3_noSplash_f9aca2e02c3777c7fb76ea768ba458f0.pdf https://doi.org/10.1525/9780520940994-005
[68] Gert, B. (2007). Common morality: Deciding what to do . Oxford Univ. Press.
[69] World Medical Association (2013). World Medical Association Declaration of Helsinki: ethical principles for medical research involving human subjects. JAMA , 310(20), 2191–2194. https://doi.org/10.1001/jama.2013.281053 Declaration of Helsinki – WMA – The World Medical Association .; see also: National Commission for the Protection of Human Subjects of Biomedical and Behavioral Research. (1979). The Belmont report: Ethical principles and guidelines for the protection of human subjects of research . U.S. Department of Health and Human Services. https://www.hhs.gov/ohrp/regulations-and-policy/belmont-report/read-the-belmont-report/index.html
[70] Zakarin Safier, L., Gumer, A., Kline, M., Egli, D., & Sauer, M. V. (2018). Compensating human subjects providing oocytes for stem cell research: 9-year experience and outcomes. Journal of assisted reproduction and genetics , 35 (7), 1219–1225. https://doi.org/10.1007/s10815-018-1171-z https://www.ncbi.nlm.nih.gov/pmc/articles/PMC6063839/ see also: Riordan, N. H., & Paz Rodríguez, J. (2021). Addressing concerns regarding associated costs, transparency, and integrity of research in recent stem cell trial. Stem Cells Translational Medicine , 10 (12), 1715–1716. https://doi.org/10.1002/sctm.21-0234
[71] Klitzman, R., & Sauer, M. V. (2009). Payment of egg donors in stem cell research in the USA. Reproductive biomedicine online , 18 (5), 603–608. https://doi.org/10.1016/s1472-6483(10)60002-8
[72] Krosin, M. T., Klitzman, R., Levin, B., Cheng, J., & Ranney, M. L. (2006). Problems in comprehension of informed consent in rural and peri-urban Mali, West Africa. Clinical trials (London, England) , 3 (3), 306–313. https://doi.org/10.1191/1740774506cn150oa
[73] Veatch, Robert M. Hippocratic, Religious, and Secular Medical Ethics: The Points of Conflict . Georgetown University Press, 2012.
[74] Msoroka, M. S., & Amundsen, D. (2018). One size fits not quite all: Universal research ethics with diversity. Research Ethics , 14 (3), 1-17. https://doi.org/10.1177/1747016117739939
[75] Pirzada, N. (2022). The Expansion of Turkey’s Medical Tourism Industry. Voices in Bioethics , 8 . https://doi.org/10.52214/vib.v8i.9894
[76] Stem Cell Tourism: False Hope for Real Money . Harvard Stem Cell Institute (HSCI). (2023). https://hsci.harvard.edu/stem-cell-tourism , See also: Bissassar, M. (2017). Transnational Stem Cell Tourism: An ethical analysis. Voices in Bioethics , 3 . https://doi.org/10.7916/vib.v3i.6027
[77] Song, P. (2011) The proliferation of stem cell therapies in post-Mao China: problematizing ethical regulation, New Genetics and Society , 30:2, 141-153, DOI: 10.1080/14636778.2011.574375
[78] Dajani, R. (2014). Jordan’s stem-cell law can guide the Middle East. Nature 510, 189. https://doi.org/10.1038/510189a
[79] International Society for Stem Cell Research. (2024). Standards in stem cell research . International Society for Stem Cell Research. https://www.isscr.org/guidelines/5-standards-in-stem-cell-research
[80] Benjamin, R. (2013). People’s science bodies and rights on the Stem Cell Frontier . Stanford University Press.
Mifrah Hayath
SM Candidate Harvard Medical School, MS Biotechnology Johns Hopkins University
Olivia Bowers
MS Bioethics Columbia University (Disclosure: affiliated with Voices in Bioethics)
Article Details

This work is licensed under a Creative Commons Attribution 4.0 International License .

An official website of the United States government
The .gov means it’s official. Federal government websites often end in .gov or .mil. Before sharing sensitive information, make sure you’re on a federal government site.
The site is secure. The https:// ensures that you are connecting to the official website and that any information you provide is encrypted and transmitted securely.
- Publications
- Account settings
Preview improvements coming to the PMC website in October 2024. Learn More or Try it out now .
- Advanced Search
- Journal List
- Stem Cell Res Ther
- v.5(4); 2014

Ethical issues in stem cell research and therapy
Nancy mp king.
1 Department of Social Sciences and Health Policy, Wake Forest School of Medicine, Medical Center Blvd, Winston-Salem, NC 27157, USA
Jacob Perrin
2 Center for Bioethics, Health, and Society, Wake Forest University, 1834 Wake Forest Rd, Winston-Salem, NC 27106, USA
Rapid progress in biotechnology has introduced a host of pressing ethical and policy issues pertaining to stem cell research. In this review, we provide an overview of the most significant issues with which the stem cell research community should be familiar. We draw on a sample of the bioethics and scientific literatures to address issues that are specific to stem cell research and therapy, as well as issues that are important for stem cell research and therapy but also for translational research in related fields, and issues that apply to all clinical research and therapy. Although debate about the moral status of the embryo in human embryonic stem cell research continues to have relevance, the discovery of other highly multipotent stem cell types and alternative methods of isolating and creating highly multipotent stem cells has raised new questions and concerns. Induced pluripotent stem cells hold great promise, but care is needed to ensure their safety in translational clinical trials, despite the temptation to move quickly from bench to bedside. A variety of highly multipotent stem cells - such as mesenchymal stem/stromal cells and stem cells derived from amniotic fluid, umbilical cord blood, adipose tissue, or urine - present the opportunity for widespread biobanking and increased access. With these increased opportunities, however, come pressing policy issues of consent, control, and justice. The imperatives to minimize risks of harm, obtain informed consent, reduce the likelihood of the therapeutic misconception, and facilitate sound translation from bench to bedside are not unique to stem cell research; their application to stem cell research and therapy nonetheless merits particular attention. Because stem cell research is both scientifically promising and ethically challenging, both the application of existing ethical frameworks and careful consideration of new ethical implications are necessary as this broad and diverse field moves forward.
Introduction
As every reader of this journal knows, ‘stem cell research’ is a category of enormous breadth and complexity. Current and potential therapeutic applications for stem cells are numerous. Stem cell researchers may be engaged in many different endeavors, including but not limited to seeking new sources of highly multipotent stem cells and methods of perpetuating them; creating induced pluripotent stem cell (iPSC) lines to study genetic disorders or explore pharmacogenomics; conducting animal or early-phase human studies of experimental stem cell interventions; or working with stem cells and biomaterials to develop organoids and other products for use in regenerative medicine, to name only a few possibilities.
In this review of selected major ethical issues in stem cell research and therapy, we briefly describe and discuss the most significant ethical implications of this wide-ranging and fast-moving field. Our discussion addresses research oversight in the historical context of human embryonic stem cell (hESC) research; clinical translation and uncertainty; the profound tension between the desire for clinical progress and the need for scientific caution; and issues of consent, control, commercialization, and justice arising from stem cell banking, disease modeling, and drug discovery. We seek to make stem cell scientists more aware of the need for clarity of discussion and to improve professional and public understanding of the ethical and policy issues affecting this important but early research. A review this brief is necessarily general; our hope is that researchers can use this discussion as a starting point for more in-depth identification and analysis of issues pertinent to specific translational research projects [ 1 - 3 ].
Stem cell research: oversight and clinical translation
The basic system of regulation and review of research involving humans and animals as subjects [ 4 , 5 ] is familiar to investigators. Recently, however, the term ‘translational’ has come to describe a line of research inquiry intended to stretch from bench to bedside and beyond. This has helped to emphasize that thinking about ethical issues should begin at the earliest stages of preclinical research. Ethics in both research and clinical settings is most effective when it is preventive.
In this respect, stem cell research is not unique; stem cell researchers should ask themselves the same questions about the trajectory of their translational research as would any other biomedical researcher [ 6 ]. Oversight of cell-based interventions does, however, include additional features that, while adding complexity to the regulatory process, also make it easier to take a long view, by requiring attention to the use of stem cells at all research stages. Increasing pressures for the rapid clinical translation and commercialization of stem cell products underscore the value of this long view [ 7 - 14 ].
The ethical issues that all researchers face during clinical translation begin with the need to ask a meaningful question, the answer to which has both scientific and social value and can be reached by the study as designed when properly conducted [ 6 , 15 ]. The risks of harm and the potential benefits to society from the development of generalizable knowledge (and, sometimes, potential direct benefit to patient-subjects) must be weighed and balanced at each stage of the research. Sound justification is necessary to support moving from the laboratory into animal studies, and from animals into human subjects, as well as through relevant phases of research with humans [ 15 - 18 ]. Minimizing the risks of harm, selecting and recruiting appropriate patient-subjects, facilitating informed decision making through the consent form and process, and avoiding the ‘therapeutic misconception’, whereby unduly high expectations affect all interested parties to a clinical trial, are all significant research ethics considerations, especially in first-in-human and other early-phase studies [ 19 - 26 ]. To many researchers, these considerations are simply requirements of sound and responsible study design, as exemplified, for example, in US Food and Drug Administration (FDA) guidance documents and investigational new drug requirements [ 27 ]. It should come as no surprise, however, that research design and research ethics are closely intertwined [ 1 , 6 , 15 ].
Stem cell research may give rise to heightened concern in several of these areas. One such concern is clarity of language. The term ‘stem cell’ by itself is broad and non-specific enough to be confusing; it can refer to hESCs, to iPSCs, to other types of multipotent and highly multipotent stem cells (including but not limited to stem cells derived from amniotic fluid, umbilical cord blood, adipose tissue, or urine), or to determined or adult stem cells like hematopoietic stem cells (HSCs), which have long been used in standard therapies. Patients, science reporters, and the public, on hearing the term ‘stem cell’, may thus find it difficult to distinguish between experimental stem cell interventions and proven stem cell therapies of long standing, such as treatments involving autologous or allogeneic HSC transplantation. The commercial availability worldwide of unproven ‘stem cell therapies’ that have not been studied in translational research adds to this confusion [ 12 , 14 , 24 - 26 , 28 , 29 ].
Human embryonic stem cells and embryonic stem cell research oversight committees
Hopes that the ethical controversy surrounding hESCs would become irrelevant when new sources of highly multipotent stem cells became available have proven somewhat premature. hESCs remain scientifically promising and continue to have important uses, even as research with iPSCs and other highly multipotent stem cells gains momentum [ 30 - 32 ]. A brief discussion thus seems warranted.
The first hESC line was derived in 1998, ushering in one of the most public, spirited, and intractable debates in research ethics: the moral status of the embryo from which hESCs are derived. To harvest hESCs, it is first necessary to destroy the 5-day-old preimplantation embryo. Opponents of hESC research argue that because the embryo is capable of developing into a human being, it has significant moral standing; therefore, its destruction is unethical. Some proponents of hESC research deny that the embryo has any moral status; others grant it limited moral status but argue that the value of this limited status is far outweighed by the potential benefits that can result from hESC research [ 24 , 33 ].
The ethical implications of hESC research in the US have been reflected in federal funding policy and in research oversight. In 2003, the National Academy of Sciences (NAS) established a committee to develop guidelines for institutions and investigators conducting hESC research [ 9 ]. The NAS Guidelines for Human Embryonic Stem Cell Research , most recently amended in 2010 [ 34 ], comprehensively address permissible and impermissible categories of hESC research and recommend the establishment of embryonic stem cell research oversight committees (ESCROs) to assist in research review. They also incorporate National Institutes of Health guidelines promulgated after a 2009 federal funding expansion, recommend oversight of research with human pluripotent stem cells, and address questions of consent from all donors of biomaterials, creation and use of embryos for research purposes, and animal-human chimeras.
Many research institutions have created ESCROs or ‘SCROs’ to review hESC and iPSC research; others rely on their institutional review boards or their animal care and use committees or both. As stem cell research diversifies, its ethical oversight also becomes more diverse, and questions have been raised regarding the ongoing need for specialized committees like ESCROs and SCROs [ 9 , 10 ]. The NAS Guidelines are nonetheless likely to continue providing guidance for a variety of oversight bodies reviewing stem cell research [ 9 , 10 , 32 ].
Induced pluripotent stem cells on the translational pathway
Controversy about the derivation and use of hESCs led investigators to seek less ethically fraught but maximally useful types of stem cells [ 31 ]. The history of iPSCs is one of seeking efficient ways to induce pluripotency that minimize the risk of teratoma development [ 35 ]. Although the rapidly developing science has reduced risks of harm and has increased the efficiency of pluripotent cell line creation to some extent, safety and efficacy concerns remain [ 36 ]. Indeed, the most recent advance in inducing pluripotency - stimulus-triggered acquisition of pluripotency, or STAP [ 37 ] - was widely heralded [ 38 ] but has since been called into question [ 39 ]. Obokata and colleagues [ 37 ] presented data suggesting that subjecting somatic cells to various stresses could quickly and safely produce iPSCs, but their results have not proven reproducible.
In research with iPSCs as well as with other types of stem cells, it is essential that preclinical studies in animal models and other media be sufficient to justify the progression to clinical trials. Toxicity and the risk of tumorigenicity must be assessed for all stem cell-based products, especially when genetically modified, in order to minimize the risks of harm as far as feasible before moving to humans [ 11 , 12 , 16 , 17 , 26 , 40 ].
Concern about the research use of animals - especially non-human primates - in preclinical research, including iPSC research, is growing and must be addressed; at the same time, researchers are increasingly aware that good animal models are often unavailable or inadequate to predict effects in humans. Thus, considerable uncertainty continues to surround first-in-human trials and other early-phase studies using stem cells, even as the rapid pace and apparently improving safety of iPSC creation tempt the field to move rapidly into clinical research and even therapeutic applications [ 5 , 25 , 41 ].
Clinical trials: uncertainty and human subjects
Clinical trials of iPSCs and other highly pluripotent stem cell interventions generally enroll patients as subjects at all trial stages, as using healthy volunteers may raise safety concerns or compromise the value of the data. All clinical trials must, of course, be carefully designed, rigorously justified, and properly conducted in order to protect the rights, interests, and welfare of trial subjects and contribute to generalizable knowledge [ 11 , 12 , 15 - 17 , 25 , 26 , 35 , 40 ]. Stem cell researchers can and should benefit from the lessons learned by gene transfer researchers: rapid transition to clinical applications without sufficient understanding of the mechanisms of effect is both inefficient and unwise [ 11 , 12 , 25 , 42 ].
The Geron trial provides just one instructive example. In late January 2009, the FDA approved the first clinical trial of an hESC-based experimental intervention for spinal cord injury. The product, oligodendrocyte progenitor cells (OPCs), is thought to remyelinate spinal cord axons. The trial was to enroll a small number of patient-subjects with recent serious spinal cord lesions. It was placed on hold once by the FDA to ensure the purity and safety of the OPCs and ultimately was halted by the sponsor, Geron Corporation (Menlo Park, CA, USA), for reasons of cost, after only four patient-subjects had received the intervention.
Both the trial’s design and its ultimate discontinuation were controversial. Its design caused controversy because the subjects were enrolled very soon after a serious injury, making understanding and consent challenging in this first-in-human trial and in addition making it potentially difficult to distinguish between spontaneous recovery of function and remyelination attributable to the intervention. Patients with older lesions, though very probably in a better position to make decisions about trial participation, have scar tissue that makes remyelination unlikely or impossible. The sponsor’s premature discontinuation of the trial was problematic because data insufficiency renders worthless not only its own investment but also those made by patient-subjects and investigators. The outcome had the potential to discourage pioneering stem cell research in the future [ 25 , 43 , 44 ]. Nonetheless, identifying the optimal time for post-injury intervention, both to maximize the potential for assessing effects on remyelination and to promote an optimal decision-making process by patient-subjects, is of ongoing concern to spinal cord injury researchers studying cell-based interventions [ 45 ]. More recently, discussions of ethical and design issues in particular stem cell trials (for example, macular degeneration [ 2 ] and cardiovascular disease [ 3 ]) highlight the difficult balance between the imperatives of caution and progress for first-in-human trials in high-profile areas like stem cell intervention research.
Disclosure and discussion of uncertainty with potential subjects in stem cell trials are essential in order to reduce the incidence of therapeutic misconception, whereby research subjects and also investigators and oversight bodies view research as a treatment modality or significantly overestimate the likelihood of direct benefit or both [ 19 - 22 , 41 ]. This information transparency also helps protect the integrity of the research process and the safety of patients in the face of increasing global availability of unapproved and unproven stem cell ‘treatments’ [ 11 , 12 , 16 , 24 - 26 ].
Many types of multipotent and highly multipotent stem cells have been identified as potentially suitable for clinical applications. Some of the most significant challenges faced in clinical application include how quickly to move forward in the face of great promise, great uncertainty, and great clinical need; how to regard research with investigational interventions that are difficult to standardize and impossible to undo; and how to define and describe these uncertainties in the consent process. A growing number of prestigious academics from both science and bioethics are calling attention to these challenges [ 2 , 24 , 26 , 42 ].
One prominent scientist commentator compares the current state of stem cell research with the histories of HSC transplantation and gene transfer research, citing several principles: risks of harm should be commensurate with the severity of the condition under study, preclinical animal models remain critically important, and gaining insight into therapeutic mechanisms is essential to the success of a line of clinical research. He advocates ‘a conservative approach to clinical translation of stem cell therapies’ at present, not because of risks of harm, ‘but rather because our understanding of the mechanisms by which stem cells might prove useful, and in which diseases, remains primitive’ [ 25 ]. Similarly, in an international survey of stem cell scientists and scholars of ethical issues in stem cell research, a prolific bioethics research group has identified increasing concerns arising from pressures for clinical translation, commercialization, and oversight of new stem cell technologies [ 14 ].
Highly multipotent stem cells: biobanking, disease modeling, and drug discovery
Some applications of stem cell research, such as disease modeling, drug discovery and testing, cell line banking, and commercialization of stem cell therapies, also give rise to ethical considerations specific to the field [ 11 , 12 , 14 , 16 , 24 - 26 , 28 , 29 ]. iPSCs and other highly multipotent stem cells have many additional important uses outside the typical clinical research trajectory. The creation and use of disease-specific iPSC lines, both alone and in combination with regenerative medicine products (for example, to produce ex vivo organoids), are essential components of disease modeling and drug discovery. ‘Body-on-a-chip’ types of three-dimensional organoid arrays hold great promise for improving drug development, disease modeling, and pharmacogenomic research, by lowering costs, speeding results, and increasing the safety and potential efficacy signaling of first-in-human trials, and considerable research is under way [ 46 ]. That promise is as yet unrealized, but questions of consent and control arise even at the bench. Because iPSC lines are derived from the somatic cells of identifiable individuals, disclosing to those individuals the planned and envisioned uses of iPSCs derived from the cells they have donated and obtaining consent from them are critical for the creation and sharing of cell line research libraries and the future uses of biomaterials derived from previously donated biospecimens [ 24 , 26 , 47 - 50 ].
As potentially therapeutic applications proliferate for different highly multipotent stem cell types and as technical barriers to the collection and perpetuation of cell lines continue to fall, proposed research and treatment uses abound for both autologous and allogeneic stem cells. In particular, the development of public and other broadly accessible biobanking models for stem cells derived from umbilical cord blood, amniotic fluid and placental tissue, urine, and adipose tissue holds promise for easy collection of good allograft matches for a large percentage of the population but also requires attention to ethical and policy issues [ 26 , 51 ].
Justice in stem cell research and treatment
Justice is a necessary but neglected consideration in all scientific research. Like many novel biotechnologies, gene- and cell-based and regenerative medicine interventions and products can be extraordinarily costly and time- and labor-intensive to develop and use. Justice thus requires attention to the costs of developing stem cell therapies and making them available, with the goal of reducing unfair disparities in access. Cost is a standard distributive justice concern. Less commonly discussed is the effect of research funding decisions on health disparities - both priority-setting within research and priority between research funding and funding for medical care, public health, and other public goods [ 19 - 21 ].
Justice considerations are addressed in stem cell research and therapy in several ways. The first is biobanking policy and practice. The rationale for public stem cell banking is to provide a resource for transplantation of blood-forming HSCs to virtually anyone. Ideally, large-scale banking efforts could store enough different lines of broadly multipotent and pluripotent stem cells, suitable for use in regenerative medicine applications, to provide good matches for nearly the entire population of the US. Comprehensive systems for the collection, storage, and use of stem cells of different types are, however, still in the early stages of technological and policy development. Scientific, practical, and ethical challenges include ensuring broad availability of matches for those in need, determining access for both research and therapy, refining consent forms and processes, and protecting confidentiality in labeling and information linkage [ 11 , 12 , 16 , 26 , 51 , 52 ]. Thus, large-scale biobanking of stem cell lines holds the potential to greatly increase access to stem cell therapies and reduce costs, but because available allogeneic matches may not be perfect, balancing the harms and benefits of biobanking remains critical.
The second justice-promoting feature of stem cell research and therapy has some similarities. Attempts to standardize and streamline production are more prevalent in stem cell-related research than elsewhere - especially in development of cell-based products and in regenerative medicine. In other new technologies like gene transfer, standardization, the development of platform technologies, and attempts at large-scale, cost-reducing production are in their infancy. This production perspective is an important step in reducing time, labor, and costs and thus increasing access, but it could also have interesting ethical implications. Autologous or individually ‘compounded’ cell-based interventions will certainly be more costly and less readily available - and will take more time to produce - than allogeneic and other ‘mass-produced’ cell-based interventions, which may provide a less-than-perfect match or fit for a given patient. Such differences could have efficacy implications that must be monitored and balanced against cost savings and access gains [ 51 ].
A final justice consideration that is heightened in the stem cell context is the simple reality that important work dedicated to improving the health of the public takes place in a market system with its attendant pressures of competition and commercialization. The attempt to ensure that hope does not become hype and that hype does not become fraud is a matter of justice. Thus, sound practice in clinical translation, careful discussion in the media, and even seeking balance between scientific transparency and data-sharing and the intellectual property interests of industry all have important justice implications [ 13 , 16 , 24 - 29 , 42 , 53 ]. As research funding shrinks and competitive pressures grow, it may become increasingly difficult to move deliberately toward clinical translation and to allocate research resources wisely. This is especially likely as more is learned about how to reduce the risks of harm from the creation and use of iPSCs and as the costs of careful progress continue to increase. The fewer resources we have, the more important it is to allocate funds to maximize the likelihood of knowledge development in areas of greatest promise and clinical need [ 14 , 21 ].
Individual researchers may at first regard justice considerations as somewhat removed from their daily work at bench or bedside. The goals of advancing knowledge and, ultimately, improving human health are nonetheless social goals, not merely scientific goals. Researchers make vital contributions to societal views about the value of - and best directions for - scientific progress. For this reason alone, it is worthwhile for researchers to keep in mind the population-level applications of stem cell research as well as the effects of stem cell therapy on individual health.
Summary and conclusions
As our discussion has shown, many of the ethical and policy issues that are most significant for stem cell research and therapy are similar to those arising in other novel biotechnologies. Consideration of these issues in both scientific and bioethics literatures addresses many common themes: the minimization of risks of harm; the importance of information disclosure and informed consent; the potential for overpromising, overexpectations, and the therapeutic misconception; and the pressure from disease constituencies and commercial entities to move quickly into the clinic, too often at the expense of understanding basic mechanisms. In the realm of clinical translation, trial-specific examinations of ethical issues continue to provide important guidance, not only with regard to the trials specifically considered but also as models for investigators starting down new translational pathways.
Although the creation and use of hESCs have long been the unique focus of stem cell ethics, more current controversies include the creation, for research use, of human embryos, human-animal chimeras, and gametes. Yet these marquee controversies are, in the long run, less important for the field as a whole than are more mundane, justice-oriented concerns like the creation and use of stem cell banks for research and therapy, facilitation of ‘off-the-shelf’ stem cell applications that could be less costly though perhaps less than perfect, and questions of consent, provenance, and policy. Finally, moving forward with the right blend of creativity and caution is essential, in the interest of both science and patients. In all areas of stem cell research and therapy, nuanced consideration and discussion of the best translational pathways, as viewed by ethics as well as science, will play a vital role in balancing hope and hype now and in the future, as the field continues its rapid progress.
Abbreviations
ESCRO: embryonic stem cell research oversight (committee); FDA: US Food and Drug Administration; hESC: human embryonic stem cell; HSC: hematopoietic stem cell; iPSC: induced pluripotent stem cell; NAS: National Academy of Sciences; OPC: oligodendrocyte progenitor cell; SCRO: stem cell research oversight (committee).
Competing interests
The authors declare that they have no competing interests.
- Lo B, Parham L. Resolving ethical issues in stem cell clinical trials: the example of Parkinson disease. J Law Med Ethics. 2010; 38 :257–266. doi: 10.1111/j.1748-720X.2010.00486.x. [ PubMed ] [ CrossRef ] [ Google Scholar ]
- Habets MG, van Delden JJ, Bredenoord AL. The inherent ethical challenge of first-in-human pluripotent stem cell trials. Regen Med. 2014; 9 :1–3. doi: 10.2217/rme.13.83. [ PubMed ] [ CrossRef ] [ Google Scholar ]
- Niemansburg SL, Teraa M, Hesam H, van Delden JJ, Verhaar MC, Bredenoord AL. Stem cell trials for cardiovascular medicine: ethical rationale. Tiss Eng Part A. 2013. [Epub ahead of print] [ PMC free article ] [ PubMed ]
- Levine R. Ethics and Regulation of Clinical Research. New York: Yale University Press; 1988. [ Google Scholar ]
- Gilbert S, Kaebnick GE, Murray TH. Special Report: Animal research ethics: evolving views and practices. Hastings Center Rep. 2012; 42 :S1–S39. [ Google Scholar ]
- Joffe S, Miller FG. Bench to bedside: mapping the moral terrain of clinical research. Hastings Center Rep. 2008; 38 :30–42. [ PubMed ] [ Google Scholar ]
- Arcidiacono JA, Blair JW, Benton KA. US Food and Drug Administration international collaborations for cellular therapy product regulation. Stem Cell Res Ther. 2012; 3 :38–42. doi: 10.1186/scrt129. [ PMC free article ] [ PubMed ] [ CrossRef ] [ Google Scholar ]
- Caulfield T, Zarzeczny A, McCormick J, Bubela T, Critchley C, Einsiedel E, Galipeau J, Harmon S, Huynh M, Hyun I, Illes J, Isasi R, Joly Y, Laurie G, Lomax G, Longstaff H, McDonald M, Murdoch C, Ogbogu U, Owen-Smith J, Pattinson S, Premji S, von Tigerstrom B, Winickoff DE. The stem cell research environment: a patchwork of patchworks. Stem Cell Rev. 2009; 5 :82–88. doi: 10.1007/s12015-009-9071-3. [ PubMed ] [ CrossRef ] [ Google Scholar ]
- Greely H. Assessing ESCROs: yesterday and tomorrow. Am J Bioeth. 2013; 13 :44–52. [ PubMed ] [ Google Scholar ]
- Lomax GP, Peckman SR. Stem cell policy exceptionalism: proceed with caution. Stem Cell Rev Rep. 2012; 8 :299–304. doi: 10.1007/s12015-011-9305-z. [ PubMed ] [ CrossRef ] [ Google Scholar ]
- Hyun I, Lindvall O, Ahrlund-Richter L, Cattaneo E, Cavazzana-Calvo M, Cossu G, De Luca M, Fox IJ, Gerstle C, Goldstein RA, Hermeren G, High KA, Kim HO, Lee HP, Levy-Lahad E, Li L, Lo B, Marshak DR, McNab A, Munsie M, Nakauchi H, Rao M, Rooke HM, Valles CS, Srivastava A, Sugarman J, Taylor PL, Veiga A, Wong AL, Zoloth L, Daley GQ. New ISSCR guidelines underscore major principles for responsible translational stem cell research. Cell Stem Cell. 2008; 3 :607–609. doi: 10.1016/j.stem.2008.11.009. [ PubMed ] [ CrossRef ] [ Google Scholar ]
- International Society for Stem Cell Research. Guidelines for the clinical translation of stem cells. [ http://www.isscr.org/docs/guidelines/isscrglclinicaltrans.pdf ]
- Caulfield T. Stem cell research and economic promises. J Law Med Ethics. 2010; 38 :303–313. doi: 10.1111/j.1748-720X.2010.00490.x. [ PubMed ] [ CrossRef ] [ Google Scholar ]
- Caulfield T, Rachul C, Zarzeczny A. The evolution of policy issues in stem cell research: an international survey. Stem Cell Rev Rep. 2012; 8 :1037–1042. doi: 10.1007/s12015-012-9404-5. [ PubMed ] [ CrossRef ] [ Google Scholar ]
- Emanuel EJ, Wendler D, Grady C. What makes clinical research ethical? JAMA. 2000; 283 :2701–2711. doi: 10.1001/jama.283.20.2701. [ PubMed ] [ CrossRef ] [ Google Scholar ]
- Kato K, Kimmelman J, Robert J, Sipp D, Sugarman J. Ethical and policy issues in the clinical translation of stem cells: report of a focus session at the ISSCR annual meeting. Cell Stem Cell. 2012; 11 :765–767. doi: 10.1016/j.stem.2012.11.004. [ PubMed ] [ CrossRef ] [ Google Scholar ]
- London AJ, Kimmelman J, Emborg ME. Beyond access vs. protection in trials of innovative therapies. Science. 2010; 328 :829–830. doi: 10.1126/science.1189369. [ PMC free article ] [ PubMed ] [ CrossRef ] [ Google Scholar ]
- King NM, Cohen-Haguenauer O. En route to ethical recommendations for gene transfer clinical trials. Mol Ther. 2008; 16 :432–438. doi: 10.1038/mt.2008.13. [ PubMed ] [ CrossRef ] [ Google Scholar ]
- Dresser R. First-in-human trial participants: not a vulnerable population, but vulnerable nonetheless. J Law Med Ethics. 2009; 37 :38–50. [ PMC free article ] [ PubMed ] [ Google Scholar ]
- Dresser R. Stem cell research as innovation: expanding the ethical and policy conversation. J Law Med Ethics. 2010; 38 :332–341. doi: 10.1111/j.1748-720X.2010.00492.x. [ PMC free article ] [ PubMed ] [ CrossRef ] [ Google Scholar ]
- Dresser R. Alive and well: the research imperative. J Law Med Ethics. 2012; 40 :915–921. [ PubMed ] [ Google Scholar ]
- Dresser R. The ubiquity and utility of the therapeutic misconception. Soc Philos Policy. 2002; 19 :271–294. doi: 10.1017/S0265052502192119. [ PubMed ] [ CrossRef ] [ Google Scholar ]
- King NM, Henderson GE, Churchill LR, Davis AM, Hull SC, Nelson DK, Parham-Vetter PC, Rothschild BB, Easter MM, Wilfond BS. Consent forms and the therapeutic misconception: the example of gene transfer research. IRB. 2005; 27 :1. [ PubMed ] [ Google Scholar ]
- Hyun I. The bioethics of stem cell research and therapy. J Clin Invest. 2010; 120 :71–75. doi: 10.1172/JCI40435. [ PMC free article ] [ PubMed ] [ CrossRef ] [ Google Scholar ]
- Daley GQ. The promise and perils of stem cell therapeutics. Cell Stem Cell. 2012; 10 :740–749. doi: 10.1016/j.stem.2012.05.010. [ PMC free article ] [ PubMed ] [ CrossRef ] [ Google Scholar ]
- Sugarman J. Human stem cell ethics: beyond the embryo. Cell Stem Cell. 2008; 2 :529–533. doi: 10.1016/j.stem.2008.05.005. [ PubMed ] [ CrossRef ] [ Google Scholar ]
- Department of Health and Human Services, Food and Drug Administration, Center for Biologics Evaluation and Research. Guidance for industry: considerations for the design and conduct of early-phase clinical trials of cellular and gene therapy products (DRAFT) 2013. [ http://www.fda.gov/downloads/BiologicsBloodVaccines/GuidanceComplianceRegulatoryInformation/Guidances/CellularandGeneTherapy/UCM359073.pdf ]
- Sipp D. Direct-to-consumer stem cell marketing and regulatory responses. Stem Cells Translational Med. 2013; 2 :638–640. doi: 10.5966/sctm.2013-0040. [ PMC free article ] [ PubMed ] [ CrossRef ] [ Google Scholar ]
- Weissman I. Stem cell therapies could change medicine if they get the chance. Cell Stem Cell. 2012; 10 :663–665. doi: 10.1016/j.stem.2012.05.014. [ PubMed ] [ CrossRef ] [ Google Scholar ]
- Hyun I, Hochedlinger K, Jaenish R, Yamanaka S. New advances in iPS cell research do not obviate the need for human embryonic stem cells. Cell Stem Cell. 2007; 4 :367–368. [ PubMed ] [ Google Scholar ]
- King NM, Coughlin CN, Atala A. Pluripotent stem cells: the search for the ‘perfect’ source. Minn J Law Sci Technol. 2011; 12 :715–730. [ Google Scholar ]
- Ishii T, Pera RA, Greely HT. Ethical and legal issues arising in research on inducing human germ cells from pluripotent stem cells. Cell Stem Cell. 2013; 13 :145–148. doi: 10.1016/j.stem.2013.07.005. [ PubMed ] [ CrossRef ] [ Google Scholar ]
- Cohen CB. Renewing the Stuff of Life: Stem Cells, Ethics, and Public Policy. New York: Oxford University Press; 2007. [ Google Scholar ]
- Human Embryonic Stem Cell Research Advisory Committee, The National Academies. Final Report and 2010 Amendments to the National Academies’ Guidelines for Human Embryonic Stem Cell Research. Washington, DC: National Academies Press; 2010. [ PubMed ] [ Google Scholar ]
- Yamanaka S. Induced pluripotent stem cells: past, present, and future. Cell Stem Cell. 2012; 10 :678–684. doi: 10.1016/j.stem.2012.05.005. [ PubMed ] [ CrossRef ] [ Google Scholar ]
- Pera MF. Stem cells: the dark side of induced pluripotency. Nature. 2011; 471 :46–47. doi: 10.1038/471046a. [ PubMed ] [ CrossRef ] [ Google Scholar ]
- Obokata H, Wakayama T, Sasai Y, Kojima K, Vacanti MP, Niwa H, Yamato M, Vacanti CA. Stimulus-triggered fate conversion of somatic cells into pluripotency. Nature. 2014; 505 :641–647. doi: 10.1038/nature12968. [ PubMed ] [ CrossRef ] [ Google Scholar ]
- Cyranoski D. Acid bath offers easy path to stem cells. Nature. 2014; 505 :596. doi: 10.1038/505596a. [ PubMed ] [ CrossRef ] [ Google Scholar ]
- Cyranoski D. Acid-bath stem-cell study under investigation. Nature. 2014. [ http://www.nature.com/news/acid-bath-stem-cell-study-under-investigation-1.14738 ]
- Kimmelman J, Baylis F, Glass KG. Stem cell trials: lessons from gene transfer research. Hastings Cent Rep. 2006; 36 :23–26. [ PubMed ] [ Google Scholar ]
- Hyun I. Allowing innovative stem cell based therapies outside of clinical trials: ethical and policy challenges. J Law Med Ethics. 2010; 38 :277–285. doi: 10.1111/j.1748-720X.2010.00488.x. [ PubMed ] [ CrossRef ] [ Google Scholar ]
- Wilson JM. A history lesson for stem cells. Science. 2009; 324 :727–728. doi: 10.1126/science.1174935. [ PubMed ] [ CrossRef ] [ Google Scholar ]
- Bretzner F, Gilbert F, Baylis F, Brownstone RM. Target populations for first-in-human embryonic stem cell research in spinal cords. Cell Stem Cell. 2011; 8 :468–475. doi: 10.1016/j.stem.2011.04.012. [ PubMed ] [ CrossRef ] [ Google Scholar ]
- Lukovic D, Stojkovic M, Moreno-Manzano V, Bhattacharya SS, Erceg S. Perspectives and future directions of human pluripotent stem cell-based therapies: lessons from Geron’s clinical trial for spinal cord injury. Stem Cells Dev. 2014; 23 :1–4. doi: 10.1089/scd.2013.0266. [ PubMed ] [ CrossRef ] [ Google Scholar ]
- Illes J, Reimer C, Kwon BK. Stem cell clinical trials for spinal cord injury: readiness, reluctance, redefinition. Stem Cell Rev. 2011; 7 :997–1005. doi: 10.1007/s12015-011-9259-1. [ PubMed ] [ CrossRef ] [ Google Scholar ]
- Esch MB, King TL, Shuler ML. The role of body-on-a-chip devices in drug and toxicity studies. Ann Rev Biomed Eng. 2011; 13 :55–72. doi: 10.1146/annurev-bioeng-071910-124629. [ PubMed ] [ CrossRef ] [ Google Scholar ]
- Lowenthal J, Lipnick S, Rao M, Hull SC. Specimen collection for induced pluripotent stem cell research: harmonizing the approach to informed consent. Stem Cells Translational Med. 2012; 1 :409–421. doi: 10.5966/sctm.2012-0029. [ PMC free article ] [ PubMed ] [ CrossRef ] [ Google Scholar ]
- Lomax GP, Hull SC, Lowenthal J, Rao M, Isasi R. The DISCUSS project: induced pluripotent stem cell lines from previously collected research biospecimens and informed consent: points to consider. Stem Cells Translational Med. 2013; 2 :727–730. doi: 10.5966/sctm.2013-0099. [ PMC free article ] [ PubMed ] [ CrossRef ] [ Google Scholar ]
- Lomax GP, Shepard KA. Return of results in translational iPS cell research: considerations for donor informed consent. Stem Cell Res Ther. 2013; 4 :6–7. doi: 10.1186/scrt154. [ PMC free article ] [ PubMed ] [ CrossRef ] [ Google Scholar ]
- Hyun I. The bioethics of iPS cell based drug discovery. Clin Pharmacol Ther. 2011; 89 :646–647. doi: 10.1038/clpt.2010.308. [ PubMed ] [ CrossRef ] [ Google Scholar ]
- King NM, Coughlin CN, Furth M. Ethical issues in regenerative medicine. Wake Forest Intellectual Property Law J. 2009; 9 :216–238. [ Google Scholar ]
- Faden RR, Dawson L, Bateman-House AS, Agnew DM, Bok H, Brock DW, Chakravarti A, Gao XJ, Greene M, Hansen JA, King PA, O’Brien SJ, Sachs DH, Schill KE, Siegel A, Solter D, Suter SM, Verfaillie CM, Walters LB, Gearhart JD. Public stem cell banks: considerations of justice in stem cell research and therapy. Hastings Center Rep. 2003; 33 :13–27. [ PubMed ] [ Google Scholar ]
- Caulfield T, Rachul C. Science spin: iPSC research in the news. Clin Pharmacol Ther. 2011; 89 :644–646. doi: 10.1038/clpt.2010.309. [ PubMed ] [ CrossRef ] [ Google Scholar ]
- Open access
- Published: 12 May 2024
Multipotent/pluripotent stem cell populations in stromal tissues and peripheral blood: exploring diversity, potential, and therapeutic applications
- Domenico Aprile 1 ,
- Deanira Patrone 1 ,
- Gianfranco Peluso 2 &
- Umberto Galderisi ORCID: orcid.org/0000-0003-0909-7403 1 , 3 , 4
Stem Cell Research & Therapy volume 15 , Article number: 139 ( 2024 ) Cite this article
292 Accesses
Metrics details
The concept of “stemness” incorporates the molecular mechanisms that regulate the unlimited self-regenerative potential typical of undifferentiated primitive cells. These cells possess the unique ability to navigate the cell cycle, transitioning in and out of the quiescent G0 phase, and hold the capacity to generate diverse cell phenotypes. Stem cells, as undifferentiated precursors endow with extraordinary regenerative capabilities, exhibit a heterogeneous and tissue-specific distribution throughout the human body. The identification and characterization of distinct stem cell populations across various tissues have revolutionized our understanding of tissue homeostasis and regeneration. From the hematopoietic to the nervous and musculoskeletal systems, the presence of tissue-specific stem cells underlines the complex adaptability of multicellular organisms. Recent investigations have revealed a diverse cohort of non-hematopoietic stem cells (non-HSC), primarily within bone marrow and other stromal tissue, alongside established hematopoietic stem cells (HSC). Among these non-HSC, a rare subset exhibits pluripotent characteristics. In vitro and in vivo studies have demonstrated the remarkable differentiation potential of these putative stem cells, known by various names including multipotent adult progenitor cells (MAPC), marrow-isolated adult multilineage inducible cells (MIAMI), small blood stem cells (SBSC), very small embryonic-like stem cells (VSELs), and multilineage differentiating stress enduring cells (MUSE). The diverse nomenclatures assigned to these primitive stem cell populations may arise from different origins or varied experimental methodologies. This review aims to present a comprehensive comparison of various subpopulations of multipotent/pluripotent stem cells derived from stromal tissues. By analysing isolation techniques and surface marker expression associated with these populations, we aim to delineate the similarities and distinctions among stromal tissue-derived stem cells. Understanding the nuances of these tissue-specific stem cells is critical for unlocking their therapeutic potential and advancing regenerative medicine. The future of stem cells research should prioritize the standardization of methodologies and collaborative investigations in shared laboratory environments. This approach could mitigate variability in research outcomes and foster scientific partnerships to fully exploit the therapeutic potential of pluripotent stem cells.
The term “stemness” refers to the molecular unlimited self-regenerative process, triggered by undifferentiated primitive cells that have the ability to enter and exit the G0 phase of the cell cycle and the power to generate one or more cell phenotypes. Stem cells, undifferentiated progenitors endowed with remarkable regenerative potential, display a diverse and tissue-specific distribution throughout the human body. The identification and characterization of distinct stem cell populations across various tissues have transformed our comprehension of tissue homeostasis and regeneration [ 1 ]. From the hematopoietic system to the nervous and musculoskeletal systems, the presence of tissue-specific stem cells underscores the complexity and adaptability of multicellular organisms. Notably, accumulating evidence emphasizes that different tissues harbor unique types of stem cells, each equipped with specialized functions tailored to the specific microenvironment of their residing niche. Understanding the intricate traits of tissue-specific stem cells is vital for propelling regenerative medicine forward and unlocking their full therapeutic capabilities [ 2 ].
Stem cells are characterized by their unique ability to undergo continuous division (self-renewal) and, upon specific cues, differentiate into various specialized cell types [ 3 ]. Classification of stem cells is based on their differentiation potential, encompassing totipotent and pluripotent stem cells associated with early embryo development, as well as multipotent, oligopotent, and unipotent stem cells prevalent in adult tissues, each displaying varying degrees of differentiation capacity. Totipotent cells are present in the earliest developmental stages, whereas pluripotent cells are located in the inner cell mass of the blastocyst, capable of giving rise to a diverse array of cell types representing the three germ layers but not extraembryonic tissues. Multipotent stem cells, such as mesenchymal and hematopoietic stem cells, can differentiate into various cell types within the same embryonic germ layer. Oligopotent and unipotent stem cells, often referred to as progenitor cells, have limited differentiation potential [ 4 ].
The classification of stem cells based on their potency often arises from in vitro experiments demonstrating their differentiation potential. However, for some stem cells, the in vitro differentiation potential may not accurately reflect their in vivo differentiation capacities. Mesenchymal stromal cells (MSCs) encompass a stem cell population capable of in vivo differentiation into mesodermal derivatives, such as osteocytes, adipocytes, and chondrocytes. Nevertheless, certain in vitro studies have indicated that these cells may also differentiate into neurons and other ectodermal and/or endodermal cell phenotypes.
Discrepancies between in vitro and in vivo physiological differentiation potential, along with variations in in vitro properties depending on isolation and cultivation procedures, may lead to the misclassification of stem cells [ 5 ]. In this scenario, it is important to emphasize that the in vitro biological properties of stem cells may not necessarily reflect their physiological functions in the body. The classification of stem cells for investigations aimed at isolating and cultivating stem cells for therapeutic purposes must not be confused with studies devoted to dissecting the physiological role of stem cells in tissue renewal and organismal homeostasis.
Recent discoveries suggest the existence of a diverse group of non-hematopoietic stem cells (non-HSC) primarily within bone marrow stromal tissue, as well as in the stromal components of other tissues, alongside well-established hematopoietic stem cells (HSC). Moreover, it has been theorized that among these non-HSC, a particularly rare subset exhibits several characteristics of pluripotent stem cells (PSC). In vitro studies have demonstrated the capacity of these potential PSC to differentiate into cells representing all three germ layers, and they have been identified in the scientific literature under various names, including i) multipotent adult progenitor cells (MAPC), ii) marrow-isolated adult multilineage inducible (MIAMI) cells, iii) small blood stem cell (SBSC), iv) very small embryonic-like stem cells (VSELs), and v) Multilineage differentiating stress enduring (MUSE) cells. The assignment of different nomenclatures to similar or overlapping populations of primitive stem cells within the BM may stem from diverse experimental approaches [ 3 , 4 , 5 , 6 ].
This review aims to provide a comprehensive report and comparison of various subpopulations of multipotent/pluripotent stem cells derived from stromal tissues. Through an analysis of the isolation methods and surface marker expression associated with these diverse populations, the goal is to develop a thorough understanding of the commonalities and differences among stem cells found in stromal tissues. This is to ascertain whether stem cells isolated using different methods and subsequently labeled with distinct names may refer to a singular core population of stem cells. In this scenario, the diverse biological properties of the various non-HSC stem cells may depend on the percentage of stem cells and progenitors present in the samples isolated using different procedures. It should be noted that even stem cells isolated at the 'purest' grade contain several subpopulations, including both stem cells and lineage-committed cells at various degrees of maturation [ 7 , 8 ].
Stromal cell population
The term “stromal cells” encompasses a diverse group of connective tissue cells forming the structural framework for organs and playing crucial roles in health and disease. Coexisting with parenchymal cells that define organ-specific functions, stromal cell populations include fibroblasts, pericytes, and telocytes found across various organ systems, alongside with heterogenous cell populations such as bone-marrow-derived mesenchymal stem/stromal cells (MSCs) and adipose tissue-derived stem/stromal cells (ASCs), which contain stem cells, progenitor cells and other stromal cell types [ 9 , 10 , 11 ]. Recent research has unveiled the molecular underpinnings of stromal cell contributions to processes such as tissue development, homeostasis, regeneration, immune responses, cancer, and disease [ 12 ]. While stromal cells shape their microenvironment, their functions are profoundly influenced by tissue context.
The intricate landscape of stromal cells unveils a diverse array of subpopulations, including MUSE cells, MIAMI cells, MAPCs and VSELs, each holding unique characteristics within the realm of adult multipotent/pluripotent stem cells. These distinct entities, nestled within the stromal tissues, add a layer of complexity to our understanding of the physiological regenerative potential of our tissues and organs [ 13 ]. The recognition and exploration of these specific multipotent/pluripotent subpopulations within stromal tissues broaden the horizons of regenerative medicine, offering promising avenues for therapeutic applications. Harnessing the regenerative capabilities of MUSE cells, MIAMI cells, MAPCs and VSELs, from stromal environments could pave the way for innovative approaches in tissue repair and disease treatment, elevating the role of stromal cells as crucial players in the pursuit of regenerative medicine breakthroughs.
Multilineage-differentiating stress enduring (MUSE) cells
In 2010, Professor Dezawa's research group at the University of Sendai, Japan, successfully isolated a distinctive stem cell population termed Multilineage-differentiating Stress Enduring cells (MUSE) from the mononuclear cell fraction of the bone marrow [ 14 ]. These endogenous, stress-resistant stem cells express pluripotency master genes and feature specific surface markers like SSEA-3 [ 15 , 16 ]. The utilization of FACS and MACS in isolation protocols ensures the dependable purification of MUSE cells.
MUSE cells were initially identified in humans and mice, and subsequently in other species, including rat, rabbit, sheep, and monkey, demonstrating evolutionary conservation and broad applicability [ 17 , 18 ]. These cells are found in various tissues, such as fibroblasts, adipose tissue and bone marrow MSCs, and peripheral blood, showcasing their versatile accessibility for therapeutic purposes [ 19 ].
In vitro, MUSE cells exhibit extensive trilineage differentiation into hepatocytes, neural/neuronal-lineage cells, cardiomyocytes, skeletal muscle, and glomerular cells, highlighting their pluripotent nature. Their distinctive ability for clonal in vitro trilineage differentiation, as confirmed by RT-qPCR and ICC analyses, underscores their unique pluripotent characteristics, crucial for controlled and specific differentiation in therapeutic applications. In vivo, MUSE cells differentiate into diverse cell types, emphasizing their potential in regenerative medicine.
MUSE cells, present in connective tissues, remain quiescent but activate under stress conditions [ 20 ]. Their homing ability to damaged tissues and resistance to stressors like H 2 O 2 and UV make them advantageous for therapeutic applications [ 21 ]. MUSE cells grow as single cells in suspension and form clusters positive for both MSC marker CD105 and SSEA-3. Upon transfer to an adhesion system, single cells express markers of mesoderm, ectoderm, and endoderm, indicating trilinear differentiation and self-renewal ability through multiple culture cycles (Table 1 , Fig. 1 ).
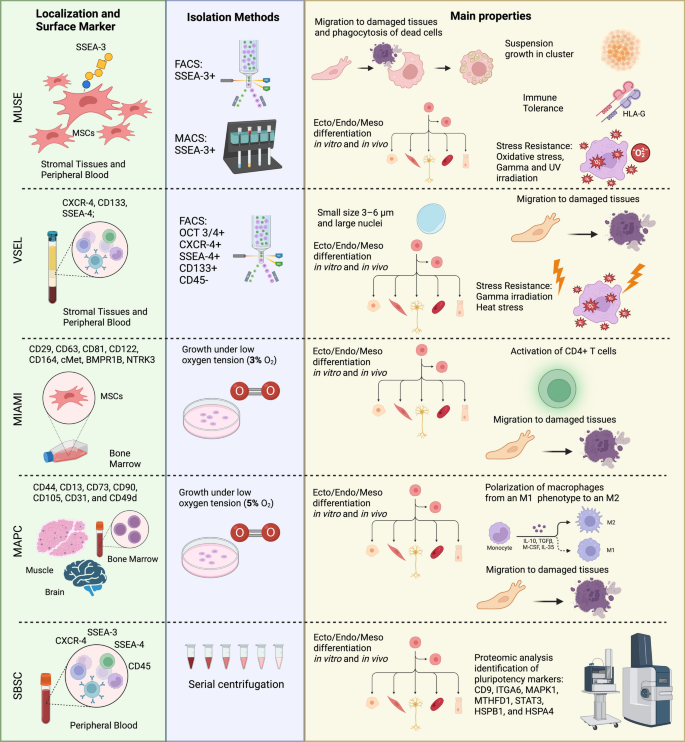
Key Properties of Stromal and Peripheral Blood Stem Cell Populations (MUSE, VSEL, SSBC, MIAMI, MAPC). Localization, surface markers, isolation methods, and main properties of stem cells have been reported
Comprehensive analyses of MUSE cells have included investigations into their transcriptomics and proteomics, identifying specific factors within the secretome that significantly contribute to stress resistance and tissue repair mechanisms [ 22 ]. The secretome of MUSE cells reveals a unique protein profile, including Serpins and 14-3-3 proteins, which contribute to stress tolerance and apoptosis inhibition [ 23 ]. Notably, in severe tissue damage, MUSE cells migrate from the bone marrow to peripheral blood, guided by the sphingosine-1-phosphate (S1P) pathway, particularly the S1PR2 receptor. In injured areas, MUSE cells persist and phagocyte apoptotic differentiated cells using distinct phagocytic receptor subsets compared to macrophages. The phagocyted contents from the differentiated cells, such as transcription factors, were promptly discharged into the cytoplasm, moved into the nucleus, and attached to the promoter regions of the stem cell genomes. Within 2 days, the MUSE cells exhibited lineage-specific markers associated with the phagocyted differentiated cells [ 21 , 24 ]. MUSE cells exhibit immunomodulatory capacity, activating regulatory T cells, suppressing dendritic cell differentiation, and expressing HLA-G, suggesting potential in immune tolerance [ 25 ].
MUSE cells possess significant regenerative potential, demonstrated by their safety, accessibility, in vivo differentiation, and therapeutic effects in various models, including lacunar infarction, acute myocardial infarction, amyotrophic lateral sclerosis, and liver fibrosis [ 26 , 27 , 28 , 29 , 30 , 31 ]. Clinical trials for conditions like acute myocardial infarction, ischemic stroke, epidermolysis bullosa, spinal cord injuries, ALS, cerebral palsy, and COVID-19-related acute respiratory distress syndrome are underway in Japan. The absence of tumorigenesis post-injection and their applicability without gene manipulation or cytokine treatment further underscore MUSE cells' promise in regenerative medicine.
In summary, MUSE cells present a unique pluripotent profile, making them versatile candidates for regenerative medicine with applications in diverse biological contexts.
Very small embryonic-like (VSEL) stem cells
Discovered in 2006, Very Small Embryonic-Like (VSEL) stem cells have emerged as a unique subset within the realm of stem cell research, holding great promise for regenerative medicine [ 32 ]. Unlike conventional stem cell populations, VSEL cells present distinct characteristics that set them apart and offer an ethical alternative to embryonic stem cells. VSEL cells, remarkably small in size (3–6 μm), lack certain lineage markers (CD45–, lin–), and express specific surface antigens like Sca-1 and CXCR4. They also exhibit pluripotency markers, including Oct-4, SSEA-3, Nanog, and Rex1, commonly associated with embryonic stem cells [ 33 ]. VSELs have been identified in both mice and humans, displaying remarkable differentiation potential and widespread localization in tissues such as bone marrow, umbilical cord blood, and peripheral blood. The sorting method involves the use of beads of predefined sizes, isolating Oct-4 + CXCR4 + SSEA-1 + Sca-1 + CD45 – lin – cells in mice and Oct-4 + CXCR4 + SSEA-4 + CD133 + CD45 – lin – cells in humans [ 34 , 35 ]. These sorted cells display large nuclei with unorganized chromatin (euchroma). Approximately 5–10% of isolated VSELs, when cultured, can form VSEL-Derived Sphere (DS), resembling embryoid bodies. These structures, observed in young mice, exhibit features such as immature cells with large nuclei, and express pluripotency markers. VSEL-DS, when replated, can generate new secondary or tertiary spheres and, under conducive conditions, differentiate into various cell types [ 36 , 37 ]. These cells exhibit not only in vitro trilineage differentiation but also contribute to diverse in vivo outcomes, including the development of bone-like structures, endothelial cells, cardiomyocytes, hepatocytes, and pancreatic cells. VSELs demonstrate migratory capabilities, responding to specific tissues during migration assays and showing a prompt response to tissue damage. Moreover, they exhibit stress resistance, surviving high doses of gamma-irradiation and extrinsic heat stress, showcasing their robust nature in adverse conditions (Table 1 , Fig. 1 ).
Comprehensive analyses have been conducted on the proteome and transcriptome of VSELs, revealing dynamic profiles that contribute to their functional diversity. Researchers have diligently investigated the molecular characteristics of VSELs to understand their developmental origin. By studying highly purified double-sorted VSELs from murine bone marrow under steady-state conditions, it has been observed that these cells express genes associated with both epiblast specification (e.g., Stella, Prdm14, Fragilis, Blimp1, Nanos3, and Dnd1) and primordial germ cells (PGCs). Notably, PGC-specific genes like Dppa2, Dppa4, and Mvh, which are characteristic of late-migratory PGCs, were also expressed. Authors hypothesized that VSELs maintain quiescence in adult tissues through mechanisms similar to those governing PGC quiescence, involving epigenetic modifications of paternally imprinted genes [ 38 ]. These findings suggest that VSELs modify the imprinting of early-development imprinted gene loci (e.g., Igf2–H19), rendering them resistant to insulin-like growth factor signaling. These insights strengthen the link between PGCs and VSELs as potential precursors for long-term tissue regeneration.
Some studies suggest that VSELs may possess immunomodulatory capabilities, indicating their potential interactions with the immune system. Claims have been made that VSELs could regulate the immune response, potentially enhancing it in cases of infections or injuries and attenuating it in conditions of autoimmune hyperactivity. However, these findings have been reported primarily on the websites of private regenerative medicine centers, lacking validation through scientific evidence.
On the other hand, several clinical trials suggest that VSELs might exhibit immune-privileged properties, potentially enabling transplantation across histocompatibility barriers without triggering an immune response. Nevertheless, further research is necessary to comprehensively understand the underlying mechanisms and to develop effective therapies utilizing VSELs [ 39 ].
VSELs are currently being investigated in clinical trials for various applications [ 40 , 41 ]. In the context of knee osteoarthritis, researchers are exploring the safety and efficacy of autologous VSELs by injecting them into the affected knee. Additionally, VSELs are being evaluated for facial skin antiaging, where they are injected into specific areas. Furthermore, these stem cells are being studied for their potential role in addressing organic erectile dysfunction. Overall, VSELs hold promise as a fascinating avenue for tissue regeneration and aging-related therapies.
A significant application of VSEL cells has been explored in diabetes repair. VSELs, isolated from mouse bone marrow, were analyzed for specific markers and demonstrated the ability to differentiate into various cell types. Upon intravenous injection into mice with pancreas damage, VSELs migrated to the pancreas, survived, and resulted in a significant decrease in blood glucose levels for at least two months. The mice also experienced gradual weight gain [ 42 ]. This groundbreaking research suggests that VSELs could be a promising strategy for treating diabetes and other regenerative diseases, offering a viable alternative to traditional stem cell therapies [ 35 , 43 ].
In summary, VSELs present a unique combination of migratory, stress-resistant, and differentiation features, positioning them as resilient contributors to tissue regeneration and repair. This makes them promising candidates for therapeutic applications in regenerative medicine, akin to MUSE cells, showcasing distinct characteristics in diverse biological contexts.
Marrow isolated adult multilineage inducible (MIAMI) cells
Marrow Isolated Adult Multilineage Inducible (MIAMI) cells were discovered in 2004 by Gianluca D'Ippolito and his team [ 47 ]. These cells have sparked significant interest in the field of regenerative medicine due to their distinctive characteristics and promising therapeutic potential. The process of isolating MIAMI cells involves a specialized expansion and selection procedure that emulates the in vivo microenvironment of primitive stem cells in the bone marrow. This process entails co-culturing adherent and non-adherent marrow cells on fibronectin under low oxygen conditions (3%) [ 48 ]. Compared to human mesenchymal stem cells, which exhibit a typical fibroblastic morphology with few long and thin cellular processes, MIAMI cells appear smaller, with a more compact and rounded cytoplasm. The remarkable ability of MIAMI cells to differentiate into various lineages—mesodermal, ectodermal, and endodermal—makes them versatile for addressing diverse tissue regeneration needs. Surface marker analysis plays a key role in identifying and characterizing these cells. They express specific markers such as CD29, CD63, CD81, CD122, CD164, cMet, BMPR1B, and NTRK3, while significantly lacking expression of markers like CD34, CD36, CD45, cKit, and HLA-DR. This distinctive marker profile contributes to their unique identity. Additionally, MIAMI cells express typical embryonic stem cell markers such as Sox2, Nanog, Oct-4, and Rex-1, along with strong telomerase expression, indicating their pluripotent characteristics, despite exhibiting a rounded and compact morphology with a high nucleus-to-cytoplasm ratio [ 47 ]. MIAMI cells isolated from various donors' bone marrow show consistently similar genetic expression, regardless of age and sex, and appear to share more proteins with human embryonic stem cells than with mesenchymal stem cells, but without the potential to form teratomas, as they have been demonstrated to be non-cancerogenic [ 48 ]. Additionally, the expression level of distinctive markers of MIAMI cells remains constant regardless of age and gender. Furthermore, although the proportion of MIAMI cells compared to the total marrow nucleated cells decreases from 0.01% at the age of 3 to 0.0018% at the age of 45, their overall number remains stable after the age of 45.
Due to these characteristics, MIAMI cells are currently under investigation in various clinical applications, including tissue regeneration [ 49 ]. MIAMI cells express numerous markers similar to embryonic stem cells, they do not possess complete self-renewal capacity; however, they appear to respond to specific molecular signals in the right environmental conditions to induce self-renewal. This can be exploited to manipulate these cells to become more stable, maintain their pluripotency, and support their immunoregulatory properties for longer periods. Importantly, MIAMI cells show rapid proliferation without signs of senescence, ensuring the maintenance of their differentiation potential during prolonged culture periods, which is crucial for scalability and potential clinical applications. MIAMI cells demonstrate migratory capabilities to damaged tissues and immunomodulatory capacity, suggesting their potential in tissue repair and immunomodulation [ 50 , 51 ]. In therapeutic applications, MIAMI cells have shown promise in various pathologies, such as in the treatment of peripheral vascular disease (PVD). Studies using a murine model of critical limb ischemia have demonstrated that the combination of MIAMI cells with a bilayer electrospun gelatin B nanofiber construct (BIC) significantly improved limb recovery compared to single treatments [ 52 ]. This combined approach led to improved blood flow restoration, reduced ischemia and necrosis, and prevention of intermuscular adipose tissue infiltration (IMAT). However, further research and clinical studies are essential to unveil the full therapeutic potential of MIAMI cells and establish their role in treating a variety of diseases and conditions.
Multipotent adult progenitor cells (MAPCs)
The multipotent adult progenitor cells (MAPCs) were first identified in human bone marrow and subsequently confirmed in animal models, such as mice and rats. MAPCs have demonstrated a remarkable ability to differentiate into a variety of cell lineages, including mesodermal, ectodermal, and endodermal lineages. These cells can be isolated from various tissue sources, including bone marrow, brain, muscle and bone tissue [ 53 , 54 ]. However, isolating MAPCs from bone marrow has been one of the most common and widely studied methods to date. The process of isolating MAPCs from bone marrow involves several key steps that have been developed and optimized over the years. One distinctive feature of this process is the use of low-oxygen conditions, typically around 5%, during cell isolation. This hypoxic environment mimics the physiological conditions present in the bone marrow and promotes the maintenance of the unique properties of MAPCs. Once a critical mass of cells is reached in culture, MAPCs can then be selected using specific cell surface markers through flow cytometry techniques, allowing for the separation of MAPCs based on their expression of specific markers such as CD44, CD13, CD73, CD90, CD105, CD31, and CD49d [ 53 ]. Despite MAPCs may be present in a population of MSCs, the crucial points that define MAPCs compared to MSCs essentially lie in their different origins, not only mesodermal, and surface marker expressions. These differences define distinct potentials, such as a broader differentiative capacity, a more pronounced immunomodulatory capacity, and better performance in cell therapy. One of the most remarkable features of MAPCs is their ability to surpass the differentiation potential of traditional MSCs, which are also frequently used in the field of regenerative medicine. Therefore, not only do they possess the ability to differentiate into a variety of cell types, like MSCs, but they also exhibit exceptional plasticity and adaptability, allowing them to cross lineage barriers more completely and efficiently. MAPCs demonstrate immunomodulatory properties that go beyond those of MSCs. They can modulate immune responses, playing a role in regulating inflammation and promoting a favorable environment for tissue healing. This immunomodulatory behavior of MAPCs makes them particularly interesting for application as universal donors, as they can be transplanted into patients without the risk of immunological rejection. The option to use MAPCs as universal donors is highly appealing in regenerative medicine, as it reduces the need to find a matching donor and the risk of tissue compatibility complications. The clinical applications of multipotent adult progenitor cells (MAPCs) are extremely broad and promising, with various pieces of evidence confirming their efficacy in crucial therapeutic contexts. One of the most interesting aspects is the use of MAPC secretome, known as MAPC-conditioned medium (MAPC-CM), as a therapeutic agent for wound healing. This secretome contains a rich mixture of growth factors, cytokines, and other bioactive molecules that influence a series of key processes in tissue repair. Studies conducted on animal models with excisional wounds have shown that the application of MAPC-CM can promote cell migration, stimulate cell proliferation, promote collagen deposition, and enhance the formation of new blood vessels, known as angiogenesis. These combined effects contribute to the rapid and effective healing of damaged tissues. Furthermore, clinical studies have demonstrated that MAPCs can have a significant impact on reducing myocardial scars in patients who have suffered from a myocardial infarction [ 55 ]. This is particularly relevant considering that myocardial scars can compromise long-term cardiac function and increase the risk of cardiovascular complications. MAPCs, due to their ability to differentiate into cardiac cells and their modulating effect on the surrounding microenvironment, can contribute to the regeneration of damaged cardiac tissue and the reduction of scars, thereby improving cardiac function and reducing the risk of complications. In the context of stroke recovery, the MASTERS study has highlighted that MAPCs, particularly the Multistem type, can offer significant benefits if administered early within the first 36 h after the stroke [ 55 ]. This underscores the crucial importance of optimized timing in stem cell therapies. MAPCs can act by reducing inflammation, promoting the regeneration of damaged brain tissues, and improving neurological function, thereby contributing to the recovery process after a stroke. Transcriptomic analyses have also been performed, providing important insights into the differences in differentiation potential between MAPCs and traditional MSCs [ 56 ]. These analyses have revealed that MAPCs show a greater inclination towards endothelial differentiation, namely the formation of cells that comprise blood and lymphatic vessels. This characteristic has been supported by in vitro experiments, such as Matrigel plug tests, which simulate the formation of blood vessels in a three-dimensional environment. MAPCs thus appear to express genes that are involved in angiogenesis, the process through which new blood vessels are formed from pre-existing ones, promoting tissue growth and repair [ 57 , 58 ]. On the other hand, MSCs seem to show a greater propensity towards differentiation into cartilage (chondrogenic) and bone (osteogenic) tissue cells. In summary, transcriptomic analyses have highlighted that MAPCs and MSCs present significant differences in their differentiation potential, with the former showing a greater inclination towards blood vessel formation and the latter towards the formation of cartilage and bone tissues. These differences can have crucial implications in the context of regenerative medicine, allowing for the targeted use of each cell type based on the specific needs of the patient and the pathological condition to be treated. In conclusion, MAPCs exhibit exceptional characteristics that make them valuable in regenerative medicine. Their ability to differentiate into a wide range of cell lineages, together with their immunomodulatory properties and distinct transcriptomic profiles, makes them versatile players in the treatment of a variety of pathologies. Research efforts continue to fully explore and exploit the therapeutic potential of MAPCs, with the aim of improving the health and quality of life of patients suffering from chronic diseases and severe injuries.
Small blood stem cells (SBSCs)
Identified in human peripheral blood, Small Blood Stem Cells (SBSCs), as reported by Filidou et al. [ 44 ], exhibit specific characteristics and differentiation potential, solidifying their significance in the landscape of stem cell biology. SBSCs showed bulk in vitro trilineage differentiation as demonstrated by RT-qPCR and ICC, while specific details about clonal in vitro trilineage differentiation and bulk in vivo differentiation are not provided (Table 1 , Fig. 1 ).
The population of SBSCs is distinguished by its unique expression profile, encompassing pluripotent embryonic markers, hematopoietic markers, and mesenchymal markers. This heterogeneity suggests a multifaceted nature of SBSCs, contributing to their potential in various biological processes.
Notable markers and factors associated with SBSCs encompass pluripotent and embryonic markers, exemplified by the expression of NANOG, SSEA-3, SSEA-4 and CXCR4 highlighting their potential for multilineage differentiation. SBSCs exhibit a distinctive co-expression of hematopoietic markers (CD45) and mesenchymal markers (CD90, CD29, CD105, PTH1R), suggesting their association with both blood cell development and mesenchymal lineage differentiation (Table 1 ). Quantitative proteomic profiling of SBSCs has identified diverse stem cell markers, including CD9, ITGA6, MAPK1, MTHFD1, STAT3, HSPB1, and HSPA4, enriching the understanding of their molecular composition (Fig. 1 ). Moreover, SBSCs harbor transcriptional regulatory complex factors like STAT5B, PDLIM1, ANXA2, ATF6, and CAMK1, contributing to their regulatory capabilities. This comprehensive expression profile underscores the heterogeneity and versatility of SBSCs, positioning them as a unique subset of stem cells with the potential to play a pivotal role in diverse biological processes. The isolation of SBSCs involves a protocol with serial centrifugation, facilitating their separation and collection from peripheral blood [ 45 ]. Specific details about the immunomodulatory capacity of SBSCs are not provided, highlighting avenues for further exploration to comprehensively understand their therapeutic potential in regenerative medicine. This characterization emphasizes the importance of various attributes for the evaluation and potential application of these stem cell populations.
Anyway, recent findings investigate the safety and tolerability of SBSC in dental implantation for patients with severe bone defects. Nine patients received different doses of SB cells, and evaluations were conducted through computed tomography (CT) scans and comprehensive chemistry panel testing. The trial, spanning six months, revealed no severe adverse effects, with observed improvements in bone mineral density (BMD) and stress levels. Elevations in specific cytokines and chemokines indicated SBSC-triggered responses for local tissue repair [ 46 ]. The findings support the well-tolerated use of SB cells in dental implantation, suggesting their potential for accelerating osseointegration in high-risk patients.
Coordinating embryonic, hematopoietic, and mesenchymal markers, along with the presence of various stem cell-related factors, accentuates the intriguing nature of SBSCs, warranting further investigation in the realm of stem cell research.
Markers and methodologies used for isolation
Analyzing the surface markers of the five considered stem cell populations and isolation methods, SSEA-3 emerges as a key marker extensively used in the isolation process. SSEA-3 plays a pivotal role in identifying and purifying these stem cell populations [ 16 ].
The chemokine receptor CXCR4 has been identified as a relevant marker associated with VSELs. CXCR4, also known as C-X-C chemokine receptor type 4, plays a role in the migration and homing of stem cells. CXCR4 is expressed on the surface of VSELs, and its interaction with its ligand, SDF-1 (stromal cell-derived factor 1), is considered crucial for the mobilization and homing of VSELs in various tissues [ 32 ]. This interaction is implicated in the trafficking of VSELs to areas of tissue damage or injury, where they may contribute to regenerative processes. The presence of CXCR4 on VSELs is a notable characteristic and contributes to the understanding of the homing and migration mechanisms that these stem cells employ.
CD133, also known as prominin-1, is a surface marker associated with VSELs. CD133 is a glycoprotein and is often utilized as one of the distinctive markers for isolating and characterizing VSELs.
In addition to SSEA-3 and CXCR, several other markers have been mentioned for isolating specific populations of stem cells. For instance, CD45 and CD90 have been identified as co-expressed markers in some SBSCs, emphasizing their association with blood cell development. CD29, CD105, and PTH1R have been recognized as mesenchymal markers expressed by SBSCs, indicating their ability to differentiate into mesenchymal lineages.
For MIAMI cells, CD122, CD29, CD63, CD81, CD164, CD90, and SSEA-4 have been cited as surface markers. These markers provide a distinctive profile for the identification and characterization of MIAMI cells. In the context of MAPCs, markers such as CD44, CD13, CD73, CD90, CD105, CD31, CD49d have been indicated as distinguishing elements.
Notably, methods such as Fluorescence-Activated Cell Sorting (FACS) and Magnetic-Activated Cell Sorting (MACS) have been employed for their precision in isolating cells expressing specific markers, like SSEA-3 [ 17 ]. These techniques enable the attainment of a more homogenous cell population, concentrating solely on those cells expressing the targeted marker. In contrast, the isolation method involving bone marrow under low oxygen tension (3% O2) emphasizes a distinct approach, suggesting the importance of the microenvironment in which stem cells reside.
FACS and MACS are particularly advantageous in achieving a higher degree of purity in isolated populations, ensuring that the isolated cells predominantly express the desired surface markers. This precision is crucial for subsequent analyses and applications, enhancing the reliability of research outcomes. On the other hand, methods like isolation from bone marrow under low oxygen tension might yield more heterogeneous populations, potentially capturing a broader range of stem cells with diverse characteristics.
The choice of isolation method significantly influences the purity and homogeneity of the obtained stem cell populations. While FACS and MACS offer a more defined and targeted approach, other methods might capture a broader spectrum of stem cell phenotypes.
Role of cell cycle phases in the isolation of pluripotent stem cells
The dynamic nature of stem cells, as they undergo cycling, implies a constantly changing phenotype. This characteristic serves as a protective mechanism, preventing catastrophic toxicity by allowing stem cells to exhibit different phenotypes at relatively short intervals during the cell cycle. The concept of a "stem cell calculus" is proposed, wherein changes in phenotype throughout the cell cycle represent individual components, and the overall outcome is an integration of these changes. Various studies on different stem cell types align with this model. Notably, research on highly purified LRH stem cells, even when isolated at different cell cycle points, has revealed significant heterogeneity. This observed heterogeneity, while present at the cellular level, still demonstrates overall patterns of differentiation. An analogy is drawn to the decay of radioactive substances, where individual atomic behavior appears random, but when observed as a whole, it follows a predictable pattern. This suggests that the regulation of the stem cell population as a whole, rather than individual cells, involves control mechanisms influencing birth and death probabilities [ 8 , 59 , 60 , 61 ].
This perspective raises questions about the previously-dismissed significance of the stem cell assay CFU-s (Colony-forming Unit Spleen), as it did not correlate with studies on purified stem cells. Given the observed heterogeneity in purified stem cells, the importance of CFU-s as a stem cell assay may need reconsideration. Recent emphasis on single-cell RNA analysis has revealed heterogeneity in different cell populations, including murine hematopoietic stem cells. Even in highly purified cells, small cell cycle progressions likely contribute to observed heterogeneity [ 62 ].
The proposed model suggests the existence of a universal stem cell encompassing hematopoietic LT-HSC (long-term hematopoietic stem cells) and various non-hematopoietic stem and progenitor cells, forming a continuum related to the cell cycle [ 8 ]. The differentiation of this stem cell relies on cell cycle-related changes in differentiation potential, illustrated by marker expressions like B220 and Gr-1, along with data on megakaryocyte development. The stem cell's tissue residence, modulated by extracellular vesicles, plays a crucial role, allowing transformation into non-hematopoietic tissue-specific stem cells. This model accounts for constant heterogeneity in different stem and progenitor cell classes, attributing it to continual phenotypic changes as the stem cell progresses through the cell cycle. The model is conceptualized as a stem cell calculus, where cycle-related phenotype changes represent derivatives, and the overall population outcome is the integral. While previous studies have provided valuable insights into purified LT-HSCs at specific cell cycle phases, future progress in the field involves characterizing the entire stem cell population.
This may lead to stem cell misclassification and identification, see the discussion.
The analysis of the five stem cell populations highlights several common features that are of particular interest in the context of regenerative medicine. Firstly, Multilineage-differentiating Stress Enduring (MUSE) cells, Very Small Embryonic-Like (VSEL) Cells, Small Blood Stem Cells (SBSC), Marrow Isolated Adult Multilineage Inducible (MIAMI) Cells, and Multipotent Adult Progenitor Cells (MAPC) share the presence of pluripotent embryonic markers and the ability to differentiate into a wide range of cell types, spanning the three germ layers. The notable similarity in differentiative potential suggests a possible common origin, even if contrasting data are present in literature on this subject.
MUSE cells are the most extensively studied cell types among the five above reported stem cells and hold promise for regenerative medicine. These well-characterized cells exhibit extraordinary potential for repairing damaged tissues. Similarly, VSELs have also been the focus of intense research and possess equally intriguing characteristics. Both cell types share several key features: MUSE cells and VSELs express the SSEA-3 antigen [ 63 , 64 ], which plays a role in cellular functions and differentiation; both cell types can migrate to damaged tissues, contributing to tissue repair; these cells demonstrate remarkable resilience to environmental stressors and adverse conditions. However, there are some notable differences between them. MUSE cells are mainly derived from mesenchymal tissues, while VSELs are believed to be derived from primordial germ cells or other embryonic precursors [ 65 ]. VSELs are exceptionally small, with diameters ranging from 3 to 5 µm, whereas MUSE cells are reported to be larger.
The extremely small size reported for VSEL, ranging from 3 to 5 µm, raises questions and triggers skepticism within the scientific community [ 37 ]. Cells of such diminutive dimensions are seldom encountered, and the presence of cells this small within the context of VSELs has been a subject of debate. The rarity of cells with such minuscule sizes in the cellular landscape introduces a level of skepticism regarding their actual existence and biological potential. Concerns have been voiced within the scientific community regarding the possibility that measurements of VSEL sizes may be influenced by various analytical techniques, emphasizing the need for further characterization of phenotype and isolation methodologies to more conclusively establish the true nature and size of these particular cells.
Despite these differences, both MUSE and VSEL cells offer exciting avenues for regenerative therapies, and ongoing research aims to harness their potential for treating various diseases and injuries.
In a recent paper by Oguma et al. [ 66 ], a comprehensive analysis of the transcriptome of MUSE cells at the single-cell level was conducted, drawing a comparative assessment with the transcriptome of MSCs. The study focused also on evaluating the expression profiles of various markers associated with VSELs within both MUSE cells and MSCs. VSELs, as defined by Shin et al. (2012), are characterized by their positivity for CXCR4, along with the expression of epiblast-related markers (GBX2, FGF5, NODAL) and primordial germ cell-related markers (DPPA3 [Stella], PRDM1 [Blimp1], PRDM14), while being negative for PTPRC (CD45). Intriguingly, neither MUSE nor MSCs expressed these specific marker genes, with the exception of FGF5, which exhibited higher expression levels in MSCs compared to MUSE [ 66 ].
VSELs also express additional markers, such as SSEA-4, and share similarities with germ cells, expressing markers like DDX4/VASA and PRDM14. In vitro, these VSELs remain quiescent, except in ascites, and become highly activated after exposure to valproic acid and follicle-stimulating hormone (FSH). VSELs spontaneously form aggregates resembling tumor-like structures or grow into larger cells resembling oocytes. Several studies propose a germinal origin for VSELs, while MUSE cells are found in both stromal tissues like bone marrow and tissues from the umbilical cord. While certain conditions may lead to uncontrolled proliferation of VSELs, resulting in tumor formation, numerous studies have demonstrated the non-tumorigenic nature of MUSE cells [ 26 , 33 ]. This stark contrast in tumorigenicity further underscores the distinctive characteristics between VSELs and MUSE cells, emphasizing the importance of comprehending their unique molecular profiles and biological behaviors for their potential applications in regenerative medicine.
This differential expression pattern provides valuable insights into the distinctive molecular profiles of MUSE cells and VSEL, emphasizing the need for a nuanced understanding of their biological characteristics and potential applications in regenerative medicine.
SBSC exhibit considerable overlap in characteristics with VSELs, except for the expression of CD45. This commonality suggests a shared profile related to pluripotency and regenerative potential. Furthermore, a comparative analysis with MUSE cells reveals intriguing parallels, as both SBSC and MUSE cells express SSEA-3. Interestingly, SBSC isolated from peripheral blood also exhibit CD45 expression, aligning them with MUSE cells in this aspect. However, a notable distinction remains in terms of cell size. These findings contribute to a nuanced understanding of the unique features and potential applications of SB cells, positioning them within the broader landscape of stem cell populations and highlighting both shared and distinct attributes.
MAPCs have attracted attention in biomedical research due to their remarkable capacity to differentiate into various cell types derived from the three embryonic germ layers: endoderm, mesoderm, and ectoderm. MUSE, VSEL, and MAPC stem cells can be isolated from stromal tissues, but MAPC cells uniquely possess the ability to be isolated from several organs. Recent studies indicate the potential presence of MAPCs in other adult tissues, such as the liver and brain, expanding their possible sources of isolation. Molecular distinctions between MAPC and other stem cells, including the differential expression of pluripotent and surface markers, may reflect the diverse origins and differentiation potentials of these cell populations. Both MUSE and MAPCs have demonstrated the ability to differentiate into a wide range of cell types; however, their effectiveness or differentiation potential may vary in specific experimental contexts or in relation to the preferred cell types. For instance, MAPCs might exhibit a greater predisposition to differentiate into specific cell types compared to MUSE cells, or vice versa, depending on experimental conditions or the cellular environment [ 58 ]. Concerning tumorigenicity, MUSE cells have been consistently described as non-tumorigenic in various studies, making them promising for clinical applications without the risk of tumor formation. In contrast, MAPCs may show tumorigenic potential in certain contexts or if not adequately controlled during culture, raising concerns about their therapeutic use.
MAPCs have emerged as a subject of controversy within the scientific community, primarily due to challenges associated with their reproducibility. The reliability and consistency of research findings related to MAPCs have been called into question, leading to the retraction of several studies that initially reported on these cells. The inherent difficulty in replicating experimental outcomes with MAPCs has raised concerns about the robustness and validity of the scientific evidence surrounding their properties and potential applications. These uncertainties underscore the importance of rigorous experimental design, standardization of methodologies, and comprehensive validation processes to address the reproducibility issues associated with MAPCs and establish a more reliable foundation for their characterization and therapeutic exploration. In summary, although MAPCs share some characteristics with the other population of stem cells, such as the ability to differentiate into different cell types, there are significant differences in their tissue origins, surface markers, tumorigenic potential, and other biological characteristics, which make them distinctive and potentially useful for different applications in regenerative medicine.
MIAMI cells are adult cells with interesting therapeutic potential but distinctive characteristics. Indeed, the isolation of MIAMI cells, to date, occurs solely from the bone marrow aspirates, while MUSE cells and VSEL can be isolated from a variety of stromal tissues. Nonetheless, these cells have sparked significant interest in the field of regenerative medicine due to their versatility and potential in treating bone lesions and musculoskeletal disorders. Unlike MUSE stem cells, known for their remarkable resistance to stress, MIAMI are not generally considered stress-resistant. This may impact their utility in clinical contexts where stress conditions are a significant factor. However, MIAMI cells have demonstrated to promote blood vessel formation and reduce inflammation and necrosis in ischemic tissues. This is attributable to the secreted factors they release into the surrounding environment, as identified by secretome analysis. Regarding surface markers, both for MIAMI and MAPC cells, the expression of SSEA-3 still needs to be evaluated.
In-depth studies on the proteome and transcriptome of these cell subpopulations could be pivotal to better understand the molecular basis of their unique characteristics and potential common origins. Identifying key expressed genes and proteins could shed light on the regulation of molecular pathways involved in pluripotency and cell differentiation.
The cell cycle plays a predominant role in influencing the differentiation capabilities of stem cells and the expression of various stem cell surface markers. In the context of the proposed universal stem cell hypothesis, a comprehensive investigation into the cell cycle phases of pluripotent stem cells isolated from stromal tissues becomes crucial. It is conceivable that the expression of specific markers in pluripotent stem cells might vary across different cell cycle phases. For instance, certain markers may be expressed during the G1 phase but not in other phases like S phase. Analyzing marker expression at distinct cell cycle stages could provide valuable insights into the regulatory mechanisms governing pluripotent stem cells. Correlating these cell cycle phases with the differentiation capacities across the three embryonic germ layers further enhances our understanding. This detailed analysis is instrumental in uncovering both similarities and differences within these stem cell populations. Ultimately, exploring the expression dynamics of stem cell markers throughout the cell cycle holds great promise for unraveling the intricacies of pluripotent stem cell behavior and refining our comprehension of their unique characteristics.
However, it is worth noting that the high variability in results across different laboratories could be attributed to technical differences in the isolation and culture procedures of these stem cells. Standardizing work methodologies could help reduce this variability and provide more consistent results, thereby facilitating a more accurate understanding of each population's intrinsic characteristics.
Taking into consideration the above-reported findings, the differences in biological properties among the various stromal stem cell populations so far described may be due to the fact that they are truly distinct cell populations (Fig. 2 ). Alternatively, it must be remembered that stem cells are inherently heterogeneous. This implies that the stem cell niche hosts different subpopulations of stem cells, each presenting subtle differences in stemness and lineage potential [ 67 ]. The isolation and cultivation procedures of the aforementioned stromal stem cells may have selected a specific stem cell subpopulation from the larger population of stromal stem cells.
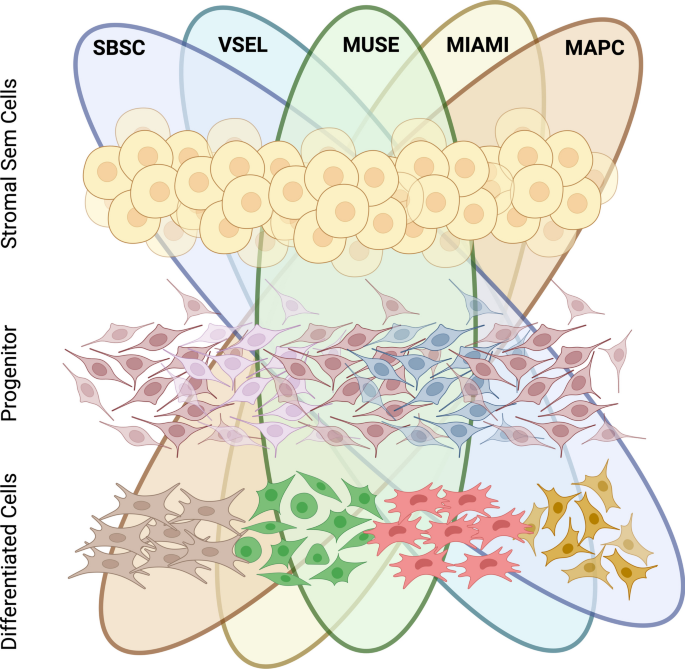
Correlation Hypothesis Among Different Stromal/Stem Cell Lines: within stromal tissues and peripheral blood, various populations of multipotent and pluripotent stem cells (including MUSE cells, VSEL, MAPC, MIAMI, SBSC) exist. These populations share numerous common characteristics, suggesting potential interrelations or overlaps. Factors such as different isolation protocols, distinct cell cycle stages, or diverse niches could contribute to the isolation of these subpopulations. Each selected subpopulation (MUSE, VSEL, MAPC, MIAMI, SBSC) possesses specific progenitors and differentiation potential. Given the overlapping nature of stem cell populations, it is plausible that these features exhibit commonalities. However, further investigation is needed to determine whether stromal tissues harbor distinct subsets of stem cells with overlapping features or contains a single stem population, with subtypes, which can be isolated based on the aforementioned factors
This scenario is further complicated by the fact that every single cell population may exhibit differences according to the cell cycle stage of its components. Finally, confounding issues may also arise from differences in the evaluation of biological properties, either in vitro or in vivo .
Conclusions and future perspectives
In conclusion, multipotent/pluripotent adult stem cell (PSC) populations represent an extraordinary resource for regenerative medicine, offering therapeutic possibilities for a broad spectrum of pathologies. However, the confusion arising from the diversity of PSC types and their unique characteristics emphasizes the need to isolate and characterize these populations in common laboratories. Only through detailed analyses of the proteome, secretome, and transcriptome can we clarify the overlaps and differences between these stem cells, contributing to a deeper understanding of their origins and therapeutic potential.
The future of PSC research should focus on standardizing methodologies and conducting in-depth analyses in shared laboratories. This approach could not only reduce variability in results but also facilitate scientific collaboration to maximize the therapeutic potential of pluripotent stem cells. Furthermore, ongoing research into optimizing the timing of therapies underscores the need for further investigations to refine treatment strategies across different clinical conditions.
Abbreviations
Adipose tissue-derived stem/stromal cells
Fluorescence-activated cell sorting
Hematopoietic stem cells
Magnetic-activated cell sorting
Multipotent adult progenitor cells
Marrow-isolated adult multilineage inducible cells
Mesenchymal stem/stromal cells
Multilineage differentiating stress enduring cells
Primordial germ cells
Pluripotent stem cells
Sphingosine-1-phosphate
Small blood stem cells
Very small embryonic-like stem cells
Clevers H. Stem cells, asymmetric division and cancer. Nat Genet. 2005;37(10):1027–8.
Article CAS PubMed Google Scholar
Labusca L, Mashayekhi K. Human adult pluripotency: Facts and questions. World J Stem Cells. 2019;11(1):1–12.
Article PubMed PubMed Central Google Scholar
Shahriyari L, Komarova NL. Symmetric vs asymmetric stem cell divisions: an adaptation against cancer? PLoS ONE. 2013;8(10):e76195.
Article CAS PubMed PubMed Central Google Scholar
Zhang L, Hu J, Athanasiou KA. The role of tissue engineering in articular cartilage repair and regeneration. Crit Rev Biomed Eng. 2009;37(1–2):1–57.
Quinn C, Flake AW. In vivo differentiation potential of mesenchymal stem cells: prenatal and postnatal model systems. Transfus Med Hemotherapy. 2008;35(3):239–47.
Article Google Scholar
Bhartiya D. Pluripotent stem cells in adult tissues: struggling to be acknowledged over two decades. Stem Cell Rev Rep. 2017;13(6):713–24.
Galderisi U, Giordano A. The gap between the physiological and therapeutic roles of mesenchymal stem cells. Med Res Rev. 2014;34(5):1100–26.
Quesenberry PJ, Wen S, Goldberg LR, Dooner MS. The universal stem cell. Leukemia. 2022;36(12):2784–92.
Squillaro T, Peluso G, Galderisi U. Clinical trials with mesenchymal stem cells: an update. Cell Transpl. 2016;25(5):829–48.
Di Maio G, Alessio N, Peluso G, Perrotta S, Monda M, Di Bernardo G. Molecular and physiological effects of browning agents on white adipocytes from bone marrow mesenchymal stromal cells. Int J Mol Sci. 2022;23(20):12151.
Di Maio G, Alessio N, Demirsoy IH, Peluso G, Perrotta S, Monda M, et al. Evaluation of browning agents on the white adipogenesis of bone marrow mesenchymal stromal cells: a contribution to fighting obesity. Cells. 2021;10(2):403.
Manetti M. Molecular morphology and function of stromal cells. Int J Mol Sci. 2021;22(24):13422.
Luzzani C, Neiman G, Garate X, Questa M, Solari C, Fernandez Espinosa D, et al. A therapy-grade protocol for differentiation of pluripotent stem cells into mesenchymal stem cells using platelet lysate as supplement. Stem Cell Res Ther. 2015;6(1):6.
Kuroda Y, Kitada M, Wakao S, Nishikawa K, Tanimura Y, Makinoshima H, et al. Unique multipotent cells in adult human mesenchymal cell populations. Proc Natl Acad Sci. 2010;107(19):8639–43.
Dezawa M. Muse cells provide the pluripotency of mesenchymal stem cells: direct contribution of muse cells to tissue regeneration. Cell Transpl. 2016;25(5):849–61.
Aprile D, Alessio N, Squillaro T, Di Bernardo G, Peluso G, Galderisi U. Role of glycosphingolipid SSEA‐3 and FGF2 in the stemness and lineage commitment of multilineage differentiating stress enduring (MUSE) cells. Cell Prolif. 2023 56(1).
Aprile D, Alessio N, Demirsoy IH, Squillaro T, Peluso G, Di Bernardo G, et al. MUSE stem cells can be isolated from stromal compartment of mouse bone marrow, adipose tissue, and ear connective tissue: a comparative study of their in vitro properties. Cells. 2021;10(4):761.
Wakao S, Kitada M, Kuroda Y, Shigemoto T, Matsuse D, Akashi H, et al. Multilineage-differentiating stress-enduring (Muse) cells are a primary source of induced pluripotent stem cells in human fibroblasts. Proc Natl Acad Sci. 2011;108(24):9875–80.
Sato T, Wakao S, Kushida Y, Tatsumi K, Kitada M, Abe T, et al. A novel type of stem cells double-positive for SSEA-3 and CD45 in human peripheral blood. Cell Transpl. 2020;1(29):096368972092357.
Alessio N, Squillaro T, Özcan S, Di Bernardo G, Venditti M, Melone M, et al. Stress and stem cells: adult Muse cells tolerate extensive genotoxic stimuli better than mesenchymal stromal cells. Oncotarget. 2018;9(27):19328–41.
Yamada Y, Wakao S, Kushida Y, Minatoguchi S, Mikami A, Higashi K, et al. S1P–S1PR2 axis mediates homing of muse cells into damaged heart for long-lasting tissue repair and functional recovery after acute myocardial infarction. Circ Res. 2018;122(8):1069–83.
Acar MB, Aprile D, Ayaz-Guner S, Guner H, Tez C, Di Bernardo G, et al. Why do muse stem cells present an enduring stress capacity? Hints from a comparative proteome analysis. Int J Mol Sci. 2021;22(4):2064.
Alessio N, Özcan S, Tatsumi K, Murat A, Peluso G, Dezawa M, et al. The secretome of MUSE cells contains factors that may play a role in regulation of stemness, apoptosis and immunomodulation. Cell Cycle. 2017;16(1):33–44.
Wakao S, Oguma Y, Kushida Y, Kuroda Y, Tatsumi K, Dezawa M. Phagocytosing differentiated cell-fragments is a novel mechanism for controlling somatic stem cell differentiation within a short time frame. Cell Mol Life Sci. 2022;79(11):542.
Uchida H, Morita T, Niizuma K, Kushida Y, Kuroda Y, Wakao S, et al. Transplantation of unique subpopulation of fibroblasts, muse cells, ameliorates experimental stroke possibly via robust neuronal differentiation. Stem Cells. 2016;34(1):160–73.
Iseki M, Kushida Y, Wakao S, Akimoto T, Mizuma M, Motoi F, et al. Human muse cells, nontumorigenic phiripotent-like stem cells, have liver regeneration capacity through specific homing and cell replacement in a mouse model of liver fibrosis. Cell Transpl. 2017;26(5):821–40.
Yamashita T, Kushida Y, Wakao S, Tadokoro K, Nomura E, Omote Y, et al. Therapeutic benefit of Muse cells in a mouse model of amyotrophic lateral sclerosis. Sci Rep. 2020;10(1):17102.
Yamada Y, Minatoguchi S, Baba S, Shibata S, Takashima S, Wakao S, et al. Human Muse cells reduce myocardial infarct size and improve cardiac function without causing arrythmias in a swine model of acute myocardial infarction. PLoS ONE. 2022;17(3): e0265347.
Uchida H, Niizuma K, Kushida Y, Wakao S, Tominaga T, Borlongan CV, et al. Human muse cells reconstruct neuronal circuitry in subacute lacunar stroke model. Stroke. 2017;48(2):428–35.
Article PubMed Google Scholar
Al Sammarraie SHA, Aprile D, Meloni I, Alessio N, Mari F, Manata M, et al. An example of neuro-glial commitment and differentiation of muse stem cells obtained from patients with IQSEC2-related neural disorder: a possible new cell-based disease model. Cells. 2023;12(7):977.
Amin M, Kushida Y, Wakao S, Kitada M, Tatsumi K, Dezawa M. Cardiotrophic growth factor-driven induction of human muse cells into cardiomyocyte-like phenotype. Cell Transpl. 2018;27(2):285–98.
Kucia M, Reca R, Campbell FR, Zuba-Surma E, Majka M, Ratajczak J, et al. A population of very small embryonic-like (VSEL) CXCR4+SSEA-1+Oct-4+ stem cells identified in adult bone marrow. Leukemia. 2006;20(5):857–69.
Ratajczak MZ, Zuba-Surma EK, Wysoczynski M, Ratajczak J, Kucia M. Very small embryonic-like stem cells: characterization, developmental origin, and biological significance. Exp Hematol. 2008;36(6):742–51.
Kuruca SE, Çelik DD, Özerkan D, Erdemir G. Characterization and isolation of very small embryonic-like (VSEL) stem cells obtained from various human hematopoietic cell sources. Stem Cell Rev Rep. 2019;15(5):730–42.
Halasa M, Baskiewicz-Masiuk M, Dabkowska E, Machalinski B. An efficient two-step method to purify very small embryonic-like (VSEL) stem cells from umbilical cord blood (UCB). Folia Histochem Cytobiol. 2008 Jun 4;46(2).
Ratajczak MZ, Ratajczak J, Suszynska M, Miller DM, Kucia M, Shin DM. A novel view of the adult stem cell compartment from the perspective of a quiescent population of very small embryonic-like stem cells. Circ Res. 2017;120(1):166–78.
Ratajczak MZ, Ratajczak J, Kucia M. Very small embryonic-like stem cells (VSELs). Circ Res. 2019;124(2):208–10.
Chumak V, Sielatycka K, Ciechanowicz A, Bujko K, Ratajczak MZ, Kucia M. Proteomic analysis of murine bone marrow very small embryonic-like stem cells at steady-state conditions and after in vivo stimulation by nicotinamide and follicle-stimulating factor reflects their germ-lineage origin and multi germ layer differentiation potential. Stem Cell Rev Rep. 2023;19(1):120–32.
Anwar S, Abbas Z, Numan A. Safety and efficacy of regenerative medicine (PRP and PBD-VSEL stem cell therapy) for Parkinson’s disease. J Neurol Sci. 2023;455: 122595.
Zuba-Surma EK, Wojakowski W, Ratajczak MZ, Dawn B. Very small embryonic-like stem cells: biology and therapeutic potential for heart repair. Antioxid Redox Signal. 2011;15(7):1821–34.
Reginia A, Samochowiec J, Jabłoński M, Ferensztajn-Rochowiak E, Rybakowski JK, Telesiński A, et al. Markers of regenerative processes in patients with bipolar disorder: a case-control study. Brain Sci. 2020;10(7):408.
Abouzaripour M, Ragerdi Kashani I, Pasbakhsh P, Atlasy N. Intravenous transplantation of very small embryonic like stem cells in treatment of diabetes mellitus. Avicenna J Med Biotechnol. 2015;7(1):22–31.
PubMed PubMed Central Google Scholar
Bhartiya D, Jha N, Tripathi A, Tripathi A. Very small embryonic-like stem cells have the potential to win the three-front war on tissue damage, cancer, and aging. Front Cell Dev Biol. 2023;4:10.
Google Scholar
Filidou E, Kandilogiannakis L, Tarapatzi G, Spathakis M, Su C, Rai A, et al. A simplified and effective approach for the isolation of small pluripotent stem cells derived from human peripheral blood. Biomedicines. 2023;11(3):787.
Gargiulo C, Pham VH, Thuy Hai N, Nguyen KCD, Van PP, Abe K, et al. Isolation and characterization of multipotent and pluripotent stem cells from human peripheral blood. Stem Cell Discov. 2015;05(03):19–32.
Article CAS Google Scholar
Feng SW, Su YH, Lin YK, Wu YC, Huang YH, Yang FH, et al. Small blood stem cells for enhancing early osseointegration formation on dental implants: a human phase I safety study. Stem Cell Res Ther. 2021;12(1):380.
D’Ippolito G, Diabira S, Howard GA, Menei P, Roos BA, Schiller PC. Marrow-isolated adult multilineage inducible (MIAMI) cells, a unique population of postnatal young and old human cells with extensive expansion and differentiation potential. J Cell Sci. 2004;117(14):2971–81.
D’Ippolito G, Howard GA, Roos BA, Schiller PC. Isolation and characterization of marrow-isolated adult multilineage inducible (MIAMI) cells. Exp Hematol. 2006;34(11):1608–10.
Grau-Monge C, Delcroix GJR, Bonnin-Marquez A, Valdes M, Awadallah ELM, Quevedo DF, et al. Marrow-isolated adult multilineage inducible cells embedded within a biologically-inspired construct promote recovery in a mouse model of peripheral vascular disease. Biomed Mater. 2017;12(1): 015024.
Banga R, Banga V, Eltalla A, Shahin L, Parag S, Naim M, et al. Effects of autophagy modulators tamoxifen and chloroquine on the expression profiles of long non-coding RNAs in MIAMI cells exposed to IFNγ. PLoS ONE. 2022;17(4): e0266179.
Rossi F, Noren H, Sarria L, Schiller PC, Nathanson L, Beljanski V. Combination therapies enhance immunoregulatory properties of MIAMI cells. Stem Cell Res Ther. 2019;10(1):395.
Rahnemai-Azar A, D’Ippolito G, Gomez LA, Reiner T, Vazquez-Padron RI, Perez-Stable C, et al. Human marrow-isolated adult multilineage-inducible (MIAMI) cells protect against peripheral vascular ischemia in a mouse model. Cytotherapy. 2011;13(2):179–92.
Jiang Y, Vaessen B, Lenvik T, Blackstad M, Reyes M, Verfaillie CM. Multipotent progenitor cells can be isolated from postnatal murine bone marrow, muscle, and brain. Exp Hematol. 2002;30(8):896–904.
Toma JG, Akhavan M, Fernandes KJL, Barnabé-Heider F, Sadikot A, Kaplan DR, et al. Isolation of multipotent adult stem cells from the dermis of mammalian skin. Nat Cell Biol. 2001;3(9):778–84.
Aranguren XL, Luttun A, Clavel C, Moreno C, Abizanda G, Barajas MA, et al. In vitro and in vivo arterial differentiation of human multipotent adult progenitor cells. Blood. 2007;109(6):2634–42.
Ulloa-Montoya F, Kidder BL, Pauwelyn KA, Chase LG, Luttun A, Crabbe A, et al. Comparative transcriptome analysis of embryonic and adult stem cells with extended and limited differentiation capacity. Genome Biol. 2007;8(8):R163.
Ahangar P, Mills SJ, Smith LE, Strudwick XL, Ting AE, Vaes B, et al. Human multipotent adult progenitor cell-conditioned medium improves wound healing through modulating inflammation and angiogenesis in mice. Stem Cell Res Ther. 2020;11(1):299.
Khan RS, Newsome PN. A comparison of phenotypic and functional properties of mesenchymal stromal cells and multipotent adult progenitor cells. Front Immunol. 2019;28:10.
Till JE, McCulloch EA, Siminovitch L. A stochastic model of stem cell proliferation, based on the growth of spleen colony-forming cells. Proc Natl Acad Sci. 1964;51(1):29–36.
Colvin GA, Berz D, Liu L, Dooner MS, Dooner G, Pascual S, et al. Heterogeneity of non-cycling and cycling synchronized murine hematopoietic stem/progenitor cells. J Cell Physiol. 2010;222(1):57–65.
Morita Y, Ema H, Nakauchi H. Heterogeneity and hierarchy within the most primitive hematopoietic stem cell compartment. J Exp Med. 2010;207(6):1173–82.
Zhang P, Li X, Pan C, Zheng X, Hu B, Xie R, et al. Single-cell RNA sequencing to track novel perspectives in HSC heterogeneity. Stem Cell Res Ther. 2022;13(1):39.
Liang YJ, Kuo HH, Lin CH, Chen YY, Yang BC, Cheng YY, et al. Switching of the core structures of glycosphingolipids from globo- and lacto- to ganglio-series upon human embryonic stem cell differentiation. Proc Natl Acad Sci. 2010;107(52):22564–9.
Liu Q, Zhi ZR, Li D, Cheng S, Hua YY, Tian T, et al. Muse cells, a new type of pluripotent stem cell derived from human fibroblasts. Cell Reprogram. 2016;18(2):67–77.
Mierzejewska K, Borkowska S, Suszynska E, Suszynska M, Poniewierska-Baran A, Maj M, et al. Hematopoietic stem/progenitor cells express several functional sex hormone receptors—novel evidence for a potential developmental link between hematopoiesis and primordial germ cells. Stem Cells Dev. 2015;24(8):927–37.
Oguma Y, Kuroda Y, Wakao S, Kushida Y, Dezawa M. Single-cell RNA sequencing reveals different signatures of mesenchymal stromal cell pluripotent-like and multipotent populations. Science. 2022;25(11):105395.
CAS Google Scholar
Muraglia A, Cancedda R, Quarto R. Clonal mesenchymal progenitors from human bone marrow differentiate in vitro according to a hierarchical model. J Cell Sci. 2000;113(7):1161–6.
Wakao S, Kushida Y, Dezawa M. Basic characteristics of muse cells. In 2018. p. 13–41.
Ogura F, Wakao S, Kuroda Y, Tsuchiyama K, Bagheri M, Heneidi S, et al. Human adipose tissue possesses a unique population of pluripotent stem cells with nontumorigenic and low telomerase activities: potential implications in regenerative medicine. Stem Cells Dev. 2014;23(7):717–28.
Alekseenko LL, Shilina MA, Lyublinskaya OG, Kornienko JS, Anatskaya OV, Vinogradov AE, et al. Quiescent human mesenchymal stem cells are more resistant to heat stress than cycling cells. Stem Cells Int. 2018;24(2018):1–15.
Burrows GG, Van’t Hof W, Newell LF, Reddy A, Wilmarth PA, David LL, et al. Dissection of the human multipotent adult progenitor cell secretome by proteomic analysis. Stem Cells Transl Med. 2013;2(10):745–57.
Lo Nigro A, Geraerts M, Notelaers T, Roobrouck VD, Muijtjens M, Eggermont K, et al. MAPC culture conditions support the derivation of cells with nascent hypoblast features from bone marrow and blastocysts. J Mol Cell Biol. 2012;4(6):423–6.
Fischer UM, Harting MT, Jimenez F, Monzon-Posadas WO, Xue H, Savitz SI, et al. Pulmonary passage is a major obstacle for intravenous stem cell delivery: the pulmonary first-pass effect. Stem Cells Dev. 2009;18(5):683–92.
Yasuhara T, Matsukawa N, Yu G, Xu L, Mays RW, Kovach J, et al. Transplantation of cryopreserved human bone marrowderived multipotent adult progenitor cells for neonatal hypoxie-ischemic injury: targeting the hippocampus. Rev Neurosci. 2006 Jan;17(1–2).
Wakao S, Kitada M, Kuroda Y, Dezawa M. Isolation of adult human pluripotent stem cells from mesenchymal cell populations and their application to liver damages. In 2012. p. 89–102.
Taichman RS, Wang Z, Shiozawa Y, Jung Y, Song J, Balduino A, et al. Prospective identification and skeletal localization of cells capable of multilineage differentiation in vivo. Stem Cells Dev. 2010;19(10):1557–70.
Smadja DM. Vasculogenic Stem and progenitor cells in human: future cell therapy product or liquid biopsy for vascular disease. In 2019. p. 215–37.
Chen ZH, Lv X, Dai H, Liu C, Lou D, Chen R, et al. Hepatic regenerative potential of mouse bone marrow very small embryonic-like stem cells. J Cell Physiol. 2015;230(8):1852–61.
Shin DM, Liu R, Wu W, Waigel SJ, Zacharias W, Ratajczak MZ, et al. Global gene expression analysis of very small embryonic-like stem cells reveals that the Ezh2 -dependent bivalent domain mechanism contributes to their pluripotent state. Stem Cells Dev. 2012;21(10):1639–52.
Roche S, D’Ippolito G, Gomez LA, Bouckenooghe T, Lehmann S, Montero-Menei CN, et al. Comparative analysis of protein expression of three stem cell populations: models of cytokine delivery system in vivo. Int J Pharm. 2013;440(1):72–82.
Reyes M, Dudek A, Jahagirdar B, Koodie L, Marker PH, Verfaillie CM. Origin of endothelial progenitors in human postnatal bone marrow. J Clin Investig. 2002;109(3):337–46.
Serafini M, Dylla SJ, Oki M, Heremans Y, Tolar J, Jiang Y, et al. Hematopoietic reconstitution by multipotent adult progenitor cells: precursors to long-term hematopoietic stem cells. J Exp Med. 2007;204(1):129–39.
Download references
Acknowledgements
Figures 1 and 2 contain images generated with Biorender licensed to U.G.
The work presented herein was partly supported by grants from “fondo per la crescita sostenibile (FCS)” project MICROPOLI, n. F/200004/02/X45 and PRIN n. 2022MN7M2M. Salary of Assistant Professor for D.A. was obtained from MNESYS PNRR grant.
Author information
Authors and affiliations.
Department of Experimental Medicine, Luigi Vanvitelli Campania University, Naples, Italy
Domenico Aprile, Deanira Patrone & Umberto Galderisi
Faculty of Medicine and Surgery, Saint Camillus International, University of Health Sciences, Rome, Italy
Gianfranco Peluso
Genome and Stem Cell Center (GENKÖK), Erciyes University, Kayseri, Turkey
Umberto Galderisi
Center for Biotechnology, Sbarro Institute for Cancer Research and Molecular Medicine Temple University, Philadelphia, PA, USA
You can also search for this author in PubMed Google Scholar
Contributions
UG and DA conceptualized research; UG, DA and DP drafted the manuscript and prepared the tables, and figures. UG, GP, DP and DA revised the manuscript, tables and figures. All authors approved the final manuscript before submission to the journal.
Corresponding author
Correspondence to Umberto Galderisi .
Ethics declarations
Competing interests.
The authors declare that they have no competing interests.
Additional information
Publisher's note.
Springer Nature remains neutral with regard to jurisdictional claims in published maps and institutional affiliations.
Rights and permissions
Open Access This article is licensed under a Creative Commons Attribution 4.0 International License, which permits use, sharing, adaptation, distribution and reproduction in any medium or format, as long as you give appropriate credit to the original author(s) and the source, provide a link to the Creative Commons licence, and indicate if changes were made. The images or other third party material in this article are included in the article's Creative Commons licence, unless indicated otherwise in a credit line to the material. If material is not included in the article's Creative Commons licence and your intended use is not permitted by statutory regulation or exceeds the permitted use, you will need to obtain permission directly from the copyright holder. To view a copy of this licence, visit http://creativecommons.org/licenses/by/4.0/ . The Creative Commons Public Domain Dedication waiver ( http://creativecommons.org/publicdomain/zero/1.0/ ) applies to the data made available in this article, unless otherwise stated in a credit line to the data.
Reprints and permissions
About this article
Cite this article.
Aprile, D., Patrone, D., Peluso, G. et al. Multipotent/pluripotent stem cell populations in stromal tissues and peripheral blood: exploring diversity, potential, and therapeutic applications. Stem Cell Res Ther 15 , 139 (2024). https://doi.org/10.1186/s13287-024-03752-x
Download citation
Received : 04 April 2024
Accepted : 02 May 2024
Published : 12 May 2024
DOI : https://doi.org/10.1186/s13287-024-03752-x
Share this article
Anyone you share the following link with will be able to read this content:
Sorry, a shareable link is not currently available for this article.
Provided by the Springer Nature SharedIt content-sharing initiative
- MIAMI cells
- Pluripotency
Stem Cell Research & Therapy
ISSN: 1757-6512
- Submission enquiries: Access here and click Contact Us
- General enquiries: [email protected]

COMMENTS
Stem Cell Research & Therapy is the major forum for translational research into stem cell therapies. An international peer-reviewed journal, it publishes high-quality open access research articles with a special emphasis on basic, translational and clinical research into stem cell therapeutics and regenerative therapies, including animal models and clinical trials.
Stem cell-based therapies. Stem cell-based therapies are defined as any treatment for a disease or a medical condition that fundamentally involves the use of any type of viable human stem cells including embryonic stem cells (ESCs), iPSCs and adult stem cells for autologous and allogeneic therapies ().Stem cells offer the perfect solution when there is a need for tissue and organ ...
In the last 50 years, more than 40,000 research papers have focused on stem-cell-based therapies. In this review study, we present a general overview of the translation of stem cell therapy from scientific ideas to clinical applications. ... Review and perspective of cell dosage and routes of administration from preclinical and clinical studies ...
Stem cell-based therapy: the history and cell source. a The timeline of major discoveries and advances in basic research and clinical applications of stem cell-based therapy. The term "stem ...
Stem Cell Research & Therapy (2023) Despite many reports of putative stem-cell-based treatments in genetic and degenerative disorders or severe injuries, the number of proven stem cell therapies ...
The Role of Various Factors in Neural Differentiation of Human Umbilical Cord Mesenchymal Stem Cells with a Special Focus on the Physical Stimulants, Current Stem Cell Research & Therapy, 19, 2 ...
Stem cell therapies are a type of cell therapy in which the cells used are either stem cells (as in the case of bone marrow transplantation) or are derived from stem cells, as is this case with ...
Cell therapy is a significant treatment that has been applied in a variety of diseases, including lung [33,34,35,36,37], cardiovascular [38,39,40], liver [41, 42], kidney [43, 44] and other diseases [45,46,47,48].Stem cells are primitive cells with self-renewal ability and multidirectional differentiation potential and can differentiate into a variety of functional cells or tissues.
Interview with Dr. Helen Blau on stem cells in the treatment of disease. 9m 12s Download. The derivation of induced pluripotent stem cells (iPSCs) has revolutionized stem-cell research (see the ...
Stem cells: The body's master cells. Stem cells are a special type of cells that have two important properties. They are able to make more cells like themselves. That is, they self-renew. And they can become other cells that do different things in a process known as differentiation. Stem cells are found in almost all tissues of the body.
FBLN5 was Regulated by PRDM9, and Promoted Senescence and Osteogenic Differentiation of Human Periodontal Ligament Stem Cells. Mengyao Zhao, ... Jianpeng Zhang. Read latest issue. Read the latest articles of Current Stem Cell Research & Therapy at ScienceDirect.com, Elsevier's leading platform of peer-reviewed scholarly literature.
Recent advancements in stem cell technology open a new door for patients suffering from diseases and disorders that have yet to be treated. Stem cell-based therapy, including human pluripotent stem cells (hPSCs) and multipotent mesenchymal stem cells (MSCs), has recently emerged as a key player in regenerative medicine. hPSCs are defined as self-renewable cell types conferring the ability to ...
Her research, learning more about the most fundamental aspects of stem cell biology, helps all stem cell researchers better understand the cells they work with. Jennifer Elisseeff, of the Department of Biomedical Engineering, studies the differences between embryonic stem cells and adult stem cells. She has found that embryonic stem cells are ...
Stem Cell Research & Therapy. Search within journal. Search. Volumes and issues. Volume 15 December 2024. December 2024, issue 1; Volume 14 December 2023. December 2023, issue 1; ... Stem cells on bioengineered microphysiological platforms for disease modeling and drug testing. This is a supplement. September 2013, issue 3; June 2013, issue 2;
Embryonic stem cells have been utilized in the past for research, but ethical concerns have led to them being replaced largely by stem cells derived from other origins. 12 Common tissues from which adult oligopotent and unipotent stem cells are isolated include bone marrow, adipose tissue, and trabecular bone. 13 Bone marrow has traditionally ...
Stem-cell research is the area of research that studies the properties of stem cells and their potential use in medicine. As stem cells are the source of all tissues, understanding their ...
In recent years, stem cell therapy has become a very promising and advanced scientific research topic. The development of treatment methods has evoked great expectations. This paper is a review focused on the discovery of different stem cells and the potential therapies based on these cells. The genesis of stem cells is followed by laboratory ...
Dr. Florian Halbritter, from St. Anna Children's Cancer Research Institute and second lead author of the study, said: "This was an impressive team effort, breaching geographic and disciplinary ...
Status Publisher Keeper From To Updated Extent of archive; Preserved. Peertechz Publications Pvt. Ltd. Portico. 2015. 2016. 14/05/2024
The study, which tested the safety and efficacy of intra-articular injections of autologous haMPCs to reduce inflammation and repair damaged joint cartilage, showed knee pain was significantly reduced and knee mobility was improved. 40 And the journal Stem Cell Research & Therapy reported that researchers at the University of Adelaide in ...
CAR T-cell immunotherapy changes these collected T cells to produce chimeric antigen receptors (or CARs) that adhere to tumors to destroy them. A recent study published in the New England Journal of Medicine, suggests CAR T-cell therapy could become a highly effective treatment for SLE patients who do not respond to current lupus therapeutics.
Caption: The microneedle patches used in this study are made from hyaluronic acid crosslinked with polyethylene glycol (PEG), both of which are biocompatible and commonly used in medical applications. The researchers designed the microneedle patches so that after releasing their drug payload, they can also collect samples that could be used to monitor the progress of the treatment.
Almost all studies have been done in a xenogenic approach (hPS cells in rats, guinea pigs, rabbits, pigs and nonhuman primates) and only one study allogeneically (cynomolgus monkey iPS cell ...
The Embryo Protection Act of 1991 made producing cell lines illegal. Cell lines can be imported if approved by the Central Ethics Commission for Stem Cell Research only if they were derived before May 2007.[47] Stem cell research respects the integrity of life for the embryo with heavy specifications and intense oversight.
Human embryonic stem cells and embryonic stem cell research oversight committees. Hopes that the ethical controversy surrounding hESCs would become irrelevant when new sources of highly multipotent stem cells became available have proven somewhat premature. hESCs remain scientifically promising and continue to have important uses, even as research with iPSCs and other highly multipotent stem ...
The future of stem cells research should prioritize the standardization of methodologies and collaborative investigations in shared laboratory environments. ... Various studies on different stem cell types align with this model. Notably, research on highly purified LRH stem cells, even when isolated at different cell cycle points, has revealed ...