
An official website of the United States government
The .gov means it’s official. Federal government websites often end in .gov or .mil. Before sharing sensitive information, make sure you’re on a federal government site.
The site is secure. The https:// ensures that you are connecting to the official website and that any information you provide is encrypted and transmitted securely.
- Publications
- Account settings
- My Bibliography
- Collections
- Citation manager

Save citation to file
Email citation, add to collections.
- Create a new collection
- Add to an existing collection
Add to My Bibliography
Your saved search, create a file for external citation management software, your rss feed.
- Search in PubMed
- Search in NLM Catalog
- Add to Search
Clinical presentations and epidemiology of vascular dementia
Affiliation.
- 1 Room 2941, Health Sciences Centre, University of Calgary, 3330 University Dr NW, Calgary, AB Canada T2N 2T9 [email protected].
- PMID: 28515342
- DOI: 10.1042/CS20160607
Cerebrovascular and cardiovascular diseases cause vascular brain injury that can lead to vascular cognitive impairment (VCI). VCI is the second most common neuropathology of dementia and mild cognitive impairment (MCI), accounting for up to one-third of the population risk. It is frequently present along with other age-related pathologies such as Alzheimer's disease (AD). Multiple etiology dementia with both VCI and AD is the single most common cause of later life dementia. There are two main clinical syndromes of VCI: post-stroke VCI in which cognitive impairment is the immediate consequence of a recent stroke and VCI without recent stroke in which cognitive impairment is the result of covert vascular brain injury detected only on neuroimaging or neuropathology. VCI is a syndrome that can result from any cause of infarction, hemorrhage, large artery disease, cardioembolism, small vessel disease, or other cerebrovascular or cardiovascular diseases. Secondary prevention of further vascular brain injury may improve outcomes in VCI.
Keywords: dementia; mild cognitive impairment; stroke; vascular dementia.
© 2017 The Author(s); published by Portland Press Limited on behalf of the Biochemical Society.
PubMed Disclaimer
Similar articles
- Diagnosis and management of vascular cognitive impairment and dementia. Erkinjuntti T. Erkinjuntti T. J Neural Transm Suppl. 2002;(63):91-109. doi: 10.1007/978-3-7091-6137-1_6. J Neural Transm Suppl. 2002. PMID: 12597611 Review.
- Neuropsychological assessment and cerebral vascular disease: the new standards. Godefroy O; GRECOG-VASC study group; Leclercq C, Bugnicourt JM, Roussel M, Moroni C, Quaglino V, Beaunieux H, Taillia H, Nédélec-Ciceri C, Bonnin C, Thomas-Anterion C, Varvat J, Aboulafia-Brakha T, Assal F. Godefroy O, et al. Rev Neurol (Paris). 2013 Oct;169(10):779-85. doi: 10.1016/j.neurol.2013.07.009. Epub 2013 Aug 30. Rev Neurol (Paris). 2013. PMID: 23999023 Review.
- Vascular cognitive disorder: a new diagnostic category updating vascular cognitive impairment and vascular dementia. Román GC, Sachdev P, Royall DR, Bullock RA, Orgogozo JM, López-Pousa S, Arizaga R, Wallin A. Román GC, et al. J Neurol Sci. 2004 Nov 15;226(1-2):81-7. doi: 10.1016/j.jns.2004.09.016. J Neurol Sci. 2004. PMID: 15537526 Review.
- Clinico-radiological predictors of vascular cognitive impairment (VCI) in patients with stroke: a prospective observational study. Chaudhari TS, Verma R, Garg RK, Singh MK, Malhotra HS, Sharma PK. Chaudhari TS, et al. J Neurol Sci. 2014 May 15;340(1-2):150-8. doi: 10.1016/j.jns.2014.03.018. Epub 2014 Mar 19. J Neurol Sci. 2014. PMID: 24680559
- Vascular Cognitive Impairment (VCI). Rundek T, Tolea M, Ariko T, Fagerli EA, Camargo CJ. Rundek T, et al. Neurotherapeutics. 2022 Jan;19(1):68-88. doi: 10.1007/s13311-021-01170-y. Epub 2021 Dec 22. Neurotherapeutics. 2022. PMID: 34939171 Free PMC article. Review.
- The Role of NRF2 in Cerebrovascular Protection: Implications for Vascular Cognitive Impairment and Dementia (VCID). Hu Y, Zhang F, Ikonomovic M, Yang T. Hu Y, et al. Int J Mol Sci. 2024 Mar 29;25(7):3833. doi: 10.3390/ijms25073833. Int J Mol Sci. 2024. PMID: 38612642 Free PMC article. Review.
- Gens PSD-95 and GSK-3β expression improved by hair follicular stem cells-conditioned medium enhances synaptic transmission and cognitive abilities in the rat model of vascular dementia. Ghobadi M, Akbari S, Bayat M, Moosavi SMS, Salehi MS, Pandamooz S, Azarpira N, Afshari A, Hooshmandi E, Haghani M. Ghobadi M, et al. Brain Behav. 2024 Jan;14(1):e3351. doi: 10.1002/brb3.3351. Brain Behav. 2024. PMID: 38376050 Free PMC article.
- The Luigi Sacco Hospital VAS-COG stroke care pathway: A five-year experience. Cova I, Mele F, Nicotra A, Maestri G, Cucumo V, Pomati S, Salvadori E, Pantoni L. Cova I, et al. Cereb Circ Cogn Behav. 2024 Feb 2;6:100210. doi: 10.1016/j.cccb.2024.100210. eCollection 2024. Cereb Circ Cogn Behav. 2024. PMID: 38357360 Free PMC article.
- The effect of acupuncture on oxidative stress in animal models of vascular dementia: a systematic review and meta-analysis. Bao QN, Xia MZ, Xiong J, Liu YW, Li YQ, Zhang XY, Chen ZH, Yao J, Wu KX, Zhong WQ, Xu SJ, Yin ZH, Liang FR. Bao QN, et al. Syst Rev. 2024 Feb 8;13(1):59. doi: 10.1186/s13643-024-02463-x. Syst Rev. 2024. PMID: 38331921 Free PMC article.
- Effect of non-invasive neuromodulation techniques on vascular cognitive impairment: A Bayesian network meta-analysis protocol. Yan L, Wu L, Li H, Qian Y, Wang M, Wang Y, Dou B, Yu T. Yan L, et al. PLoS One. 2024 Jan 4;19(1):e0284447. doi: 10.1371/journal.pone.0284447. eCollection 2024. PLoS One. 2024. PMID: 38175852 Free PMC article.
Publication types
- Search in MeSH
Related information
Linkout - more resources, full text sources.
- Silverchair Information Systems
Other Literature Sources
- scite Smart Citations

- Citation Manager
NCBI Literature Resources
MeSH PMC Bookshelf Disclaimer
The PubMed wordmark and PubMed logo are registered trademarks of the U.S. Department of Health and Human Services (HHS). Unauthorized use of these marks is strictly prohibited.
Call our 24 hours, seven days a week helpline at 800.272.3900

- Professionals

- Younger/Early-Onset Alzheimer's
- Is Alzheimer's Genetic?
- Women and Alzheimer's
- Creutzfeldt-Jakob Disease
- Dementia with Lewy Bodies
- Down Syndrome & Alzheimer's
- Frontotemporal Dementia
- Huntington's Disease
- Mixed Dementia
- Normal Pressure Hydrocephalus
- Posterior Cortical Atrophy
- Parkinson's Disease Dementia
- Vascular Dementia
- Korsakoff Syndrome
- Traumatic Brain Injury (TBI)
- Know the 10 Signs
- Difference Between Alzheimer's & Dementia
- 10 Steps to Approach Memory Concerns in Others
- Medical Tests for Diagnosing Alzheimer's
- Why Get Checked?
- Visiting Your Doctor
- Life After Diagnosis
- Stages of Alzheimer's
- Earlier Diagnosis
- Part the Cloud
- Research Momentum
- Our Commitment to Research
- TrialMatch: Find a Clinical Trial
- What Are Clinical Trials?
- How Clinical Trials Work
- When Clinical Trials End
- Why Participate?
- Talk to Your Doctor
- Clinical Trials: Myths vs. Facts
- Can Alzheimer's Disease Be Prevented?
- Brain Donation
- Navigating Treatment Options
- Aducanumab Discontinued as Alzheimer's Treatment
- Donanemab Approved for Treatment of Early Alzheimer's Disease
- Lecanemab Approved for Treatment of Early Alzheimer's Disease
- Medicare Treatment Coverage
- Questions for Your Doctor
- Medications for Memory, Cognition and Dementia-Related Behaviors
- Treatments for Behavior
- Treatments for Sleep Changes
- Alternative Treatments
- Facts and Figures
- Assessing Symptoms and Seeking Help
- Now is the Best Time to Talk about Alzheimer's Together
- Get Educated
- Just Diagnosed
- Sharing Your Diagnosis
- Changes in Relationships
- If You Live Alone
- Treatments and Research
- Legal Planning
- Financial Planning
- Building a Care Team
- End-of-Life Planning
- Programs and Support
- Overcoming Stigma
- Younger-Onset Alzheimer's
- Taking Care of Yourself
- Reducing Stress
- Tips for Daily Life
- Helping Family and Friends
- Leaving Your Legacy
- Live Well Online Resources
- Make a Difference
- Daily Care Plan
- Communication and Alzheimer's
- Food and Eating
- Art and Music
- Incontinence
- Dressing and Grooming
- Dental Care
- Working With the Doctor
- Medication Safety
- Accepting the Diagnosis
- Early-Stage Caregiving
- Middle-Stage Caregiving
- Late-Stage Caregiving
- Aggression and Anger
- Anxiety and Agitation
- Hallucinations
- Memory Loss and Confusion
- Sleep Issues and Sundowning
- Suspicions and Delusions
- In-Home Care
- Adult Day Centers
- Long-Term Care
- Respite Care
- Hospice Care
- Choosing Care Providers
- Finding a Memory Care-Certified Nursing Home or Assisted Living Community
- Changing Care Providers
- Working with Care Providers
- Creating Your Care Team
- Long-Distance Caregiving
- Community Resource Finder
- Be a Healthy Caregiver
- Caregiver Stress
- Caregiver Stress Check
- Caregiver Depression
- Changes to Your Relationship
- Grief and Loss as Alzheimer's Progresses
- Home Safety
- Dementia and Driving
- Technology 101
- Preparing for Emergencies
- Managing Money Online Program
- Planning for Care Costs
- Paying for Care
- Health Care Appeals for People with Alzheimer's and Other Dementias
- Social Security Disability
- Medicare Part D Benefits
- Tax Deductions and Credits
- Planning Ahead for Legal Matters
- Legal Documents
- ALZ Talks Virtual Events
- ALZNavigator™
- Veterans and Dementia
- The Knight Family Dementia Care Coordination Initiative
- Online Tools
- Asian Americans and Pacific Islanders and Alzheimer's
- Native Americans and Alzheimer's
- Black Americans and Alzheimer's
- Hispanic Americans and Alzheimer's
- LGBTQ+ Community Resources for Dementia
- Educational Programs and Dementia Care Resources
- Brain Facts
- 50 Activities
- For Parents and Teachers
- Resolving Family Conflicts
- Holiday Gift Guide for Caregivers and People Living with Dementia
- Trajectory Report
- Resource Lists
- Search Databases
- Publications
- Favorite Links
- 10 Healthy Habits for Your Brain
- Stay Physically Active
- Adopt a Healthy Diet
- Stay Mentally and Socially Active
- Online Community
- Support Groups
Find Your Local Chapter
- Any Given Moment
- New IDEAS Study
- Bruce T. Lamb, Ph.D., Chair
- Christopher van Dyck, M.D.
- Cynthia Lemere, Ph.D.
- David Knopman, M.D.
- Lee A. Jennings, M.D. MSHS
- Karen Bell, M.D.
- Lea Grinberg, M.D., Ph.D.
- Malú Tansey, Ph.D.
- Mary Sano, Ph.D.
- Oscar Lopez, M.D.
- Suzanne Craft, Ph.D.
- RFI Amyloid PET Depletion Following Treatment
- Criteria for Diagnosis and Staging
- About Our Grants
- Andrew Kiselica, Ph.D., ABPP-CN
- Arjun Masurkar, M.D., Ph.D.
- Benjamin Combs, Ph.D.
- Charles DeCarli, M.D.
- Damian Holsinger, Ph.D.
- David Soleimani-Meigooni, Ph.D.
- Donna M. Wilcock, Ph.D.
- Elizabeth Head, M.A, Ph.D.
- Fan Fan, M.D.
- Fayron Epps, Ph.D., R.N.
- Ganesh Babulal, Ph.D., OTD
- Hui Zheng, Ph.D.
- Jason D. Flatt, Ph.D., MPH
- Jennifer Manly, Ph.D.
- Joanna Jankowsky, Ph.D.
- Luis Medina, Ph.D.
- Marcello D’Amelio, Ph.D.
- Marcia N. Gordon, Ph.D.
- Margaret Pericak-Vance, Ph.D.
- María Llorens-Martín, Ph.D.
- Nancy Hodgson, Ph.D.
- Shana D. Stites, Psy.D., M.A., M.S.
- Walter Swardfager, Ph.D.
- ALZ WW-FNFP Grant
- Capacity Building in International Dementia Research Program
- ISTAART IGPCC
- Alzheimer’s Disease Strategic Fund: Endolysosomal Activity in Alzheimer’s (E2A) Grant Program
- Imaging Research in Alzheimer’s and Other Neurodegenerative Diseases
- Zenith Fellow Awards
- 2024 Part the Cloud Translational (PTC) Gene Targeting Challenge
- National Academy of Neuropsychology & Alzheimer’s Association Funding Opportunity
- Part the Cloud-Gates Partnership Grant Program: Bioenergetics and Inflammation
- Pilot Awards for Global Brain Health Leaders (Invitation Only)
- Robert W. Katzman, M.D., Clinical Research Training Scholarship
- Funded Studies
- How to Apply
- Portfolio Summaries
- Supporting Research in Health Disparities, Policy and Ethics in Alzheimer’s Disease and Dementia Research (HPE-ADRD)
- Annual Conference: AAIC
- Alzheimer's & Dementia
- Alzheimer's & Dementia: DADM
- Alzheimer's & Dementia: TRCI
- Professional Society: ISTAART
- International Network to Study SARS-CoV-2 Impact on Behavior and Cognition
- Alzheimer’s Association Business Consortium (AABC)
- Global Biomarker Standardization Consortium (GBSC)
- Global Alzheimer’s Association Interactive Network
- International Alzheimer's Disease Research Portfolio
- Alzheimer’s Disease Neuroimaging Initiative Private Partner Scientific Board (ADNI-PPSB)
- Research Roundtable
- About WW-ADNI
- North American ADNI
- European ADNI
- Australia ADNI
- Taiwan ADNI
- Argentina ADNI
- WW-ADNI Meetings
- Submit Study
- RFI Inclusive Language Guide
- Scientific Conferences
- AUC for Amyloid and Tau PET Imaging
- Make a Donation
- Walk to End Alzheimer's
- The Longest Day
- RivALZ to End ALZ
- Ride to End ALZ
- Donate Gold & Sterling Silver
- Tribute Pages
- Gift Options to Meet Your Goals
- Founders Society
- Fred Bernhardt
- Anjanette Kichline
- Lori A. Jacobson
- Pam and Bill Russell
- Gina Adelman
- Franz and Christa Boetsch
- Adrienne Edelstein
- For Professional Advisors
- Free Planning Guides
- Contact the Planned Giving Staff
- Workplace Giving
- Do Good to End ALZ
- Donate a Vehicle
- Donate Stock
- Donate Cryptocurrency
- Donor-Advised Funds
- Use of Funds
- Giving Societies
- Why We Advocate
- Ambassador Program
- About the Alzheimer’s Impact Movement
- Research Funding
- Improving Care
- Support for People Living With Dementia
- Public Policy Victories
- Planned Giving
- Community Educator
- Community Representative
- Support Group Facilitator or Mentor
- Faith Outreach Representative
- Early Stage Social Engagement Leaders
- Data Entry Volunteer
- Tech Support Volunteer
- Other Local Opportunities
- Visit the Program Volunteer Community to Learn More
- Become a Corporate Partner
- A Family Affair
- A Message from Elizabeth
- The Belin Family
- The Eliashar Family
- The Fremont Family
- The Freund Family
- Jeff and Randi Gillman
- Harold Matzner
- The Mendelson Family
- Patty and Arthur Newman
- The Ozer Family
- Salon Series
- No Shave November
- Other Philanthropic Activities
- Still Alice
- The Judy Fund E-blast Archive
- The Judy Fund in the News
- The Judy Fund Newsletter Archives
- Sigma Kappa Foundation
- Alpha Delta Kappa
- Parrot Heads in Paradise
- Tau Kappa Epsilon (TKE)
- Sigma Alpha Mu
- Alois Society Member Levels and Benefits
- Alois Society Member Resources
- Zenith Society
- Founder's Society
- Joel Berman
- JR and Emily Paterakis
- Legal Industry Leadership Council
- Accounting Industry Leadership Council
Find Local Resources
Let us connect you to professionals and support options near you. Please select an option below:
Use Current Location Use Map Selector
Search Alzheimer’s Association

Make Double the Impact Today
Causes and risks, about vascular dementia.
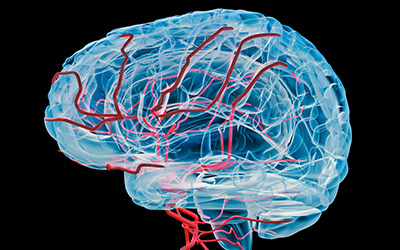
Other dementias share some common symptoms
Vascular changes that start in brain areas that play a key role in storing and retrieving information may cause memory loss that looks very much like Alzheimer's disease.
About 5% to 10% of people with dementia have vascular dementia alone. It is more common as a part of mixed dementia. Many experts believe that vascular dementia remains underdiagnosed — like Alzheimer's disease — even though it's recognized as common.
Learn more: Key Types of Dementia , Mixed Dementia
The impact of vascular conditions on thinking skills varies widely, depending on the severity of the blood vessel damage and the part of the brain it affects. Memory loss may or may not be a significant symptom depending on the specific brain areas where blood flow is reduced. Vascular damage that starts in the brain areas that play a key role in storing and retrieving information may cause memory loss that is very similar to Alzheimer’s disease. Symptoms may be most obvious when they happen soon after a major stroke. Sudden post-stroke changes in thinking and perception may include:
- Disorientation
- Trouble speaking or understanding speech
- Physical stroke symptoms, such as a sudden headache
- Difficulty walking
- Poor balance
- Numbness or paralysis on one side of the face or the body
Multiple small strokes or other conditions that affect blood vessels and nerve fibers deep inside the brain may cause more gradual thinking changes as damage accumulates. Common early signs of widespread small vessel disease include impaired planning and judgment, uncontrolled laughing and crying, declining ability to pay attention, impaired function in social situations, and difficulty finding the right words.
Learn more: Warning Signs from the American Stroke Association
Diagnostic guidelines for vascular dementia have used a range of definitions for dementia and various approaches to diagnosis. In 2011, the American Heart Association and American Stroke Association issued a joint scientific statement on vascular contributions to mild cognitive impairment (MCI) and dementia. The Alzheimer’s Association participated in developing the statement, which is also endorsed by the American Academy of Neurology. The goals of the statement, which include practice recommendations, are to raise awareness of the importance of vascular factors in cognitive change, increase diagnostic consistency and accelerate research. Under the diagnostic approach recommended in the 2011 statement, the following criteria suggest the greatest likelihood of mild cognitive impairment (MCI) or dementia is caused by vascular changes:
- The diagnosis of dementia or mild cognitive impairment is confirmed by neurocognitive testing, which involves several hours of written or computerized tests that provide detailed evaluation of specific thinking skills such as judgment, planning, problem-solving, reasoning and memory.
- A recent stroke, or
- Other vascular brain changes whose severity and pattern of affected tissue are consistent with the types of impairment documented in cognitive testing.
- There is no evidence that nonvascular factors may be contributing to cognitive decline.
The statement also details variations in these criteria that may suggest a possibility rather than a strong likelihood that cognitive change is due to vascular factors. Because vascular dementia often goes unrecognized, many experts recommend professional cognitive screening for everyone considered to be at high risk, including those who have had a stroke or a transient ischemic attack (TIA), also known as a ministroke, or who have risk factors for heart or blood vessel disease. Professional screening for depression is also recommended for high-risk groups. Depression commonly coexists with brain vascular disease and can contribute to cognitive impairment.
Learn more: AHA/ASA Scientific Statement: Vascular Contributions to Cognitive Impairment and Dementia, Mixed Dementia
Any condition that damages blood vessels anywhere in the body can cause brain changes linked to vascular dementia. As with Alzheimer’s disease, advancing age is a major risk factor. Additional risk factors for vascular dementia coincide with those that increase risk for heart disease, stroke and other conditions affecting blood vessels. Many of these factors are also linked to increased risk of Alzheimer’s. The following strategies may reduce the risk of developing diseases that affect the heart and blood vessels — and may help protect the brain:
- Don't smoke.
- Keep your blood pressure, cholesterol and blood sugar within recommended limits.
- Eat a healthy, balanced diet.
- Maintain a healthy weight.
- Limit alcohol consumption.
Learn more: Brain Health
Treatment and outcomes
The U.S. Food and Drug Administration (FDA) has not approved any drugs specifically to treat symptoms of vascular dementia, but there is evidence from clinical trials that drugs approved to treat Alzheimer’s symptoms may also offer a modest benefit in people with vascular dementia. Treatment primarily works to prevent the worsening of vascular dementia by treating the underlying disease, such as hypertension, hyperlipidemia or diabetes mellitus. Controlling risk factors that may increase the likelihood of further damage to the brain’s blood vessels is an important treatment strategy. There’s substantial evidence that treatment of risk factors may improve outcomes and help postpone or prevent further decline. Individuals should work with their physicians to develop the best treatment plan for their symptoms and circumstances.
Like other types of dementia, vascular dementia shortens life span. Some data suggest that those who develop dementia following a stroke survive an average of three years. As with other stroke symptoms, cognitive changes may sometimes improve during recovery and rehabilitation from the acute phase of a stroke as the brain generates new blood vessels and brain cells outside the damaged region take on new roles.
Help is available
- Call our 24/7 Helpline : 800.272.3900.
- Locate a support group in your community.
- Join our online community .
- Visit our Virtual Library .
Next Page: Financial Planning
Other pages in Types of Dementia
- Down Syndrome and Alzheimer's Disease
- Huntington's Disease
- Parkinson's Disease Dementia
- Related Conditions
Related Pages
The alzheimer’s association is in your community., learn how alzheimer’s disease affects the brain..
Take the Brain Tour
Don't just hope for a cure. Help us find one.
Keep up with alzheimer’s news and events.
When viewing this topic in a different language, you may notice some differences in the way the content is structured, but it still reflects the latest evidence-based guidance.
Vascular dementia
- Overview
- Theory
- Diagnosis
- Management
- Follow up
- Resources
Vascular dementia is characterised by a chronic progressive multifaceted impairment of cognitive function.
Loss of brain parenchyma is predominantly from cerebrovascular causes such as infarction and small-vessel changes.
Vascular dementia is the second most common cause of dementia in older people.
A large overlap exists with Alzheimer's dementia and many patients have a mixed form of dementia.
Early aggressive treatment of vascular risk factors is suggested in order to prevent further cerebrovascular disease. Supportive care and management of behavioural and psychological symptoms are also important. Treatments aimed at improving cognitive symptoms have shown no clear benefit.
Vascular dementia is a chronic progressive disease of the brain bringing about cognitive impairment. The executive functions of the brain such as planning are more prominently affected than memory. Motor and mood changes are often seen early. The underlying damage occurs to both grey matter and white matter from predominantly vascular causes: that is, infarction, leukoaraiosis, haemorrhage, and small-vessel changes. Mixed dementia (vascular dementia with comorbid Alzheimer's disease) is common. [1] Bowler JV. Modern concept of vascular cognitive impairment. Br Med Bull. 2007;83:291-305. http://www.ncbi.nlm.nih.gov/pubmed/17675645?tool=bestpractice.com
History and exam
Key diagnostic factors.
- history of stroke(s)
- difficulty solving problems
- disinhibition
- slowed processing of information
- poor attention
- retrieval memory deficit
- frontal release reflexes
- focal neurological signs
- impaired gait and balance
Risk factors
- age >60 years
- hypertension
- cigarette smoking
- diabetes mellitus
- hypercholesterolaemia
- alcohol misuse
Diagnostic investigations
1st investigations to order.
- erythrocyte sedimentation rate
- blood glucose level
- renal and liver function tests
- vitamin B12
- thyroid function
- CT or MRI brain
Investigations to consider
- syphilis serology
- lupus anticoagulant, antiphospholipid, and antinuclear testing
- neuropsychological testing
- carotid duplex ultrasound
- echocardiogram
Treatment algorithm
Atherosclerotic ischaemic disease, cardioembolic disease, contributors, peter passmore, bsc, mb, bch, bao, md, frcp (lond, glasg), frcpi.
Professor of Ageing and Geriatric Medicine
Queen's University Belfast
Disclosures
PP declares that he has no competing interests.
Acknowledgements
Dr Peter Passmore would like to gratefully acknowledge Dr David Wilson, Dr Grant Bateman, and Dr Velandai Srikanth, previous contributors to this topic.
DW has received educational grants from Shire. GB and VS declare that they have no competing interests.
Peer reviewers
Roy j. goldberg, md, facp, agsf, cmd.
Medical Director
Kings Harbor Multicare Center
RJG declares that he has no competing interests.
Craig N. Sawchuk, PhD
Affiliate Assistant Professor
Department of Psychiatry and Behavioral Sciences
University of Washington Medical Center
CNS declares that he has no competing interests.
Bryan Bernard, PhD
Assistant Professor and Clinical Neuropsychologist
Department of Neurological Sciences
Rush University Medical Center
BB declares that he has no competing interests.
Differentials
- Alzheimer's disease (AD)
- Mild cognitive impairment (MCI)
- Clinical guidance in neuropalliative care: an AAN position statement
- Ethical considerations in dementia diagnosis and care: an AAN position statement
Patient information
Alzheimer's disease and other kinds of dementia
Caring for someone with dementia
Use of this content is subject to our disclaimer
Log in or subscribe to access all of BMJ Best Practice
Log in to access all of bmj best practice, help us improve bmj best practice.
Please complete all fields.
I have some feedback on:
We will respond to all feedback.
For any urgent enquiries please contact our customer services team who are ready to help with any problems.
Phone: +44 (0) 207 111 1105
Email: [email protected]
Your feedback has been submitted successfully.
Masks Strongly Recommended but Not Required in Maryland, Starting Immediately
Due to the downward trend in respiratory viruses in Maryland, masking is no longer required but remains strongly recommended in Johns Hopkins Medicine clinical locations in Maryland. Read more .
- Vaccines
- Masking Guidelines
- Visitor Guidelines
Vascular Dementia
What is vascular dementia.
Vascular dementia is the second most common form of dementia after Alzheimer disease. It's caused when decreased blood flow damages brain tissue. Blood flow to brain tissue may be reduced. Or it may be completely blocked by a blood clot.
Symptoms of vascular dementia may develop slowly. Or they may develop after a stroke or major surgery, such as heart bypass surgery or abdominal surgery.
Dementia and other related diseases and conditions are hard to tell apart because they share similar symptoms. Vascular dementia is caused by problems with blood flow to the brain. But this blood flow problem can develop in different ways. Examples of vascular dementia include:
Mixed dementia. This type occurs when symptoms of both vascular dementia and Alzheimer exist.
Multi-infarct dementia. This occurs after repeated small, often "silent," blockages affect blood flow to certain parts of the brain. The changes that occur after each blockage may not be apparent. But over time, the combined effect starts to cause symptoms. This is also called vascular cognitive impairment.
The effect of decreased or no blood flow on the brain depends on the size and location of the area affected. If a small area in a part of the brain that controls memory is affected, you may be "forgetful." But this doesn't always change your ability to do normal activities. If a larger area is affected, you may have trouble thinking clearly or solving problems. These problems may change your ability to function normally.
Researchers think that vascular dementia will become more common in the next few decades because:
Vascular dementia is generally caused by conditions that occur most often in older people. These include hardening of the arteries (atherosclerosis), heart disease, and stroke.
The number of people older than age 65 is growing.
People are living longer with long-term (chronic) diseases, such as heart disease and diabetes.
What causes vascular dementia?
This disease is caused by a lack of blood flow to a part of the brain. Blood flow may be decreased or interrupted by:
Blood clots
Bleeding because of a ruptured blood vessel (such as from a stroke)
Damage to a blood vessel from atherosclerosis, infection, high blood pressure, or other causes, such as an autoimmune disorder
- CADASIL (cerebral autosomal dominant arteriopathy with sub-cortical infarcts and leukoencephalopathy) is a genetic disorder that generally leads to dementia of the vascular type. One parent with the gene for CADASIL passes it on to a child, which makes it an autosomal-dominant inheritance disorder. It affects the blood vessels in the white matter of the brain. Symptoms, such as migraine headaches, seizures, and severe depression, generally start when a person is in his or her mid-30s; but, symptoms may not appear until later in life.
Who is at risk for vascular dementia?
Risk factors for vascular dementia include risk factors for the conditions linked to it (heart disease, stroke, diabetes, and atherosclerosis):
High blood pressure
High cholesterol and triglyceride levels
Metabolic syndrome
Atrial fibrillation. This is fast and irregular heart rate of the upper 2 heart chambers.
High level of homocysteine in the blood. This can cause damage to blood vessels, heart disease, and blood clots.
Lack of physical activity
Birth control pills
Conditions that cause the blood to "thicken" (clot) more easily
Family history of dementia
Family history of CADASIL
What are the symptoms of vascular dementia?
The symptoms of vascular dementia depend on the location and amount of brain tissue involved. They may appear suddenly after a stroke, or slowly over time. Symptoms may get worse after another stroke, a heart attack, or major surgery. These are symptoms of vascular dementia:
Increased trouble doing normal daily activities because of problems with focus, communication, or inability to carry out directions
Memory problems. Short-term memory may not be affected.
Confusion, which may get worse at night (called sundown syndrome)
Stroke symptoms, such as sudden weakness and trouble with speech
Personality changes
Mood changes, such as depression or irritability
Stride changes when walking too fast or shuffling steps
Problems with movement, balance. or both
Urinary problems, such as urgency or incontinence
How is vascular dementia diagnosed?
Your healthcare provider will ask about your health history and do a physical exam. You may also need some of these tests:
Blood tests. These are done to look for other conditions causing your symptoms, such as vitamin deficiencies.
CT scan. This imaging test uses X-rays and a computer to make images of the brain. CT scans are more detailed than general X-rays.
FDG-PET scan . This is a PET scan of the brain that uses a special tracer to light up regions of the brain.
Electroencephalogram. This test measures electrical activity in the brain.
MRI . This test uses large magnets, radiofrequencies, and a computer to make detailed images of the brain.
Neuropsychological assessments. These tests can help sort out vascular dementia from other types of dementia and Alzheimer disease.
Lumbar puncture (Spinal tap). This is done to analyze cerebrospinal fluid for inflammation, infection, or other abnormalities. This can also be done to remove a large amount of fluid to see if there is any improvement.
Neuropsychiatric evaluation. This may be done to rule out a psychiatric condition that may look like dementia.
How is vascular dementia treated?
Vascular dementia can't be cured. The main goal is to treat the underlying conditions that affect the blood flow to the brain. This can help cut the risk for further damage to brain tissue.
Such treatments may include:
Medicines to manage blood pressure, cholesterol, triglycerides, diabetes, and problems with blood clotting
Lifestyle changes, such as a healthy diet, physical activity, quitting smoking, and stopping or decreasing alcohol use
Procedures to improve blood flow to the brain, such as carotid endarterectomy, angioplasty, and stenting. The carotid arteries are located in the neck and provide blood flow from the heart to the brain.
Medicines, such as cholinesterase inhibitors to treat the symptoms of dementia or antidepressants to help with depression or other symptoms
Living with vascular dementia
Vascular dementia gets worse over time and has no cure. But the rate at which the disease gets worse can vary. The disease can be stable for long periods of time. Some people with this disease may over time need a high level of care because of the loss of mental and physical abilities. Family members may be able to care for a person with vascular dementia early on. But if the disease progresses, the person may need more specialized care.
Respite programs, adult daycare programs, and other resources can help the caregiver get some time away from the demands of caring for a loved one with vascular dementia.
Long-term care facilities that specialize in caring for people with dementias, Alzheimer, and other related conditions are often available if a person affected by vascular dementia can no longer be cared for at home. Your healthcare provider can advise caregiver resources.
When should I call my healthcare provider?
People with vascular dementia and their caregivers should talk with their healthcare providers about when to call them. They will likely advise you to call if symptoms get worse. This can be obvious changes in behavior, personality, memory, or speech. You should also call if new symptoms appear. These can include sudden weakness, trouble speaking, or confusion.
Key points about vascular dementia
Vascular dementia is a disorder that damages brain tissue because of a lack of blood flow. Causes can include blood clots, ruptured blood vessels, or narrowing or hardening of blood vessels that supply the brain.
Symptoms can include problems with memory and focus, confusion, changes in personality and behavior, loss of speech and language skills, and sometimes physical symptoms, such as weakness or tremors.
Vascular dementia tends to get worse over time. Treatments can't cure the disease, but lifestyle changes and medicines to treat underlying causes might help slow its progress.
Surgery to improve blood flow to the brain can also be helpful. Other medicines might slow the progression of dementia or help with some of the symptoms it can cause.
A person with this disease may eventually need full-time nursing care or to stay in a long-term care facility.
Tips to help you get the most from a visit to your healthcare provider:
Know the reason for your visit and what you want to happen.
Before your visit, write down questions you want answered.
Bring someone with you to help you ask questions and remember what your provider tells you.
At the visit, write down the name of a new diagnosis, and any new medicines, treatments, or tests. Also write down any new directions your provider gives you.
Know why a new medicine or treatment is prescribed, and how it will help you. Also know what the side effects are.
Ask if your condition can be treated in other ways.
Know why a test or procedure is recommended and what the results could mean.
Know what to expect if you do not take the medicine or have the test or procedure.
If you have a follow-up appointment, write down the date, time, and purpose for that visit.
Know how you can contact your healthcare provider if you have questions, especially after office hours or on weekends.
Find a Doctor
Specializing In:
- Young-Onset Dementias
- Disorders of Social Cognition
- Cognitive Disorders
- Behavioral Disorders in Dementia
Find a Treatment Center
- Memory and Alzheimers Treatment Center
- Parkinson's Disease and Movement Disorders Center
Find Additional Treatment Centers at:
- Howard County Medical Center
- Sibley Memorial Hospital
- Suburban Hospital
Request an Appointment
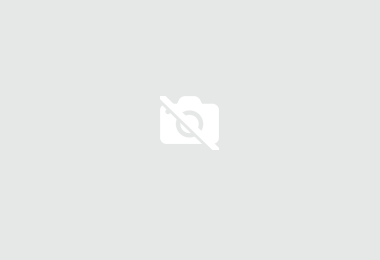
Lewy Body Dementia (LBD)
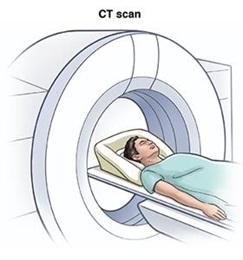
Frontotemporal Dementia
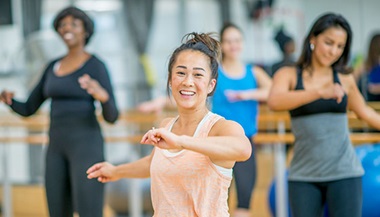
Dementia Prevention: Reduce Your Risk, Starting Now
Related Topics
Vascular dementia
On this page, coping and support, preparing for your appointment.
Doctors can nearly always determine that you have dementia, but there's no specific test that confirms you have vascular dementia. Your doctor will make a judgment about whether vascular dementia is the most likely cause of your symptoms based on the information you provide, your medical history for stroke or disorders of the heart and blood vessels, and results of tests that may help clarify your diagnosis.
If your medical record doesn't include recent values for key indicators of the health of your heart and blood vessels, your doctor will test your:
- Blood pressure
- Cholesterol
- Blood sugar
He or she may also order tests to rule out other potential causes of memory loss and confusion, such as:
- Thyroid disorders
- Vitamin deficiencies
Neurological exam
Your doctor is likely to check your overall neurological health by testing your:
- Muscle tone and strength, and how strength on one side of your body compares with the other side
- Ability to get up from a chair and walk across the room
- Sense of touch and sight
- Coordination
Brain imaging
Images of your brain can pinpoint visible abnormalities caused by strokes, blood vessel diseases, tumors or trauma that may cause changes in thinking and reasoning. A brain-imaging study can help your doctor zero in on more-likely causes for your symptoms and rule out other causes.
Brain-imaging procedures your doctor may recommend to help diagnose vascular dementia include:
Magnetic resonance imaging (MRI). An Magnetic resonance imaging (MRI) uses radio waves and a strong magnetic field to produce detailed images of your brain. You lie on a narrow table that slides into a tube-shaped MRI machine, which makes loud banging noises while it produces images.
MRI s are painless, but some people feel claustrophobic inside the machine and are disturbed by the noise. MRI s are generally the preferred imaging test because MRI s can provide even more detail than computed tomography (CT) scans about strokes, ministrokes and blood vessel abnormalities and is the test of choice for evaluating vascular dementia.
Computerized tomography (CT) scan. For a CT scan, you'll lie on a narrow table that slides into a small chamber. X-rays pass through your body from various angles, and a computer uses this information to create detailed cross-sectional images (slices) of your brain.
A CT scan can provide information about your brain's structure; tell whether any regions show shrinkage; and detect evidence of a stroke, ministroke (transient ischemic attacks), a change in blood vessels or a tumor.
Neuropsychological tests
This type of exam assesses your ability to:
- Speak, write and understand language
- Work with numbers
- Learn and remember information
- Develop a plan of attack and solve a problem
- Respond effectively to hypothetical situations
Neuropsychological tests sometimes show characteristic results for people with different types of dementia. People with vascular dementia may have an exceptionally hard time analyzing a problem and developing an effective solution.
They may be less likely to have trouble learning new information and remembering than are people with dementia due to Alzheimer's disease unless their blood vessel problems affect specific brain regions important for memory. However, there's often a lot of overlap in exam results for people with vascular dementia and people who also have the brain changes of Alzheimer's disease.
Overlap between vascular and Alzheimer's dementia
While much focus is placed on distinguishing Alzheimer's dementia from vascular dementia, it turns out there is usually substantial overlap. Most people diagnosed with Alzheimer's dementia have a vascular component and similarly most people with vascular dementia have some degree of coexisting Alzheimer's changes in their brain.
More Information
- Blood pressure test
- Carotid ultrasound
Treatment often focuses on managing the health conditions and risk factors that contribute to vascular dementia.
Controlling conditions that affect the underlying health of your heart and blood vessels can sometimes slow the rate at which vascular dementia gets worse, and may also sometimes prevent further decline. Depending on your individual situation, your doctor may prescribe medications to:
- Lower your blood pressure
- Reduce your cholesterol level
- Prevent your blood from clotting and keep your arteries clear
- Help control your blood sugar if you have diabetes
Clinical trials
Explore Mayo Clinic studies testing new treatments, interventions and tests as a means to prevent, detect, treat or manage this condition.
Though these haven't been proved to alter the course of vascular dementia, your doctor will likely recommend that you:
- Participate in regular physical activity
- Eat healthy
- Try to maintain a normal weight
- Engage in social activities
- Challenge your brain with games, puzzles and new activities, such as an art class or listening to new music
- Limit how much alcohol you drink
People with any type of dementia and their caregivers — whether it's vascular dementia or Alzheimer's disease — experience a mixture of emotions, including confusion, frustration, anger, fear, uncertainty, grief and depression.
Caring for someone with dementia
- Seek out support. Many people with dementia and their families benefit from counseling or local support services. Contact your local Alzheimer's Association affiliate to connect with support groups, resources and referrals, home care agencies, residential care facilities, a telephone help line, and educational seminars.
- Plan for the future. While your loved one is in the earlier stages of the disease, talk with a lawyer about the types of legal documents you'll need in the future, such as power of attorney, health care proxy and an advanced directive.
- Give encouragement. Care partners can help a person cope with vascular dementia by being there to listen, reassuring the person that life can still be enjoyed, providing encouragement, and doing their best to help the person retain dignity and self-respect.
- Provide a calm environment. A calm and predictable environment can help reduce worry and agitation. Establish a daily routine that includes enjoyable activities well within the comfort zone of the person with vascular dementia.
- Do activities together. Instead of dwelling on what's to come, try to find activities you both like to do, such as a painting class or a long walk.
- Respect independence as often as is safe. It may be a long time before your loved one has to give up living alone or driving. The early stages of dementia can last years, and your loved one may still be capable of doing many things on his or her own. If you and your loved one can't agree on when it's time to stop certain activities, such as driving, ask your loved one's doctor for his or her advice.
Caring for the caregiver or care partner
Providing care for and supporting a person with dementia is physically and emotionally demanding. Feelings of anger and guilt, frustration and discouragement, worry and grief, and social isolation are common. But paying attention to your own needs and well-being is one of the most important things you can do for yourself and for the person in your care.
If you're a caregiver or care partner:
- Learn as much about the disease as you can. Ask your primary care doctor or neurologist about good sources of information. Your local librarian also can help you find good resources.
- Ask questions of doctors, social workers and others involved in the care of your loved one.
- Call on friends and family members for help when you need it.
- Take a break every day.
- Take care of your health by seeing your own doctors on schedule, eating healthy meals and getting exercise.
- Make time for friends, and consider joining a support group.
If you've had a stroke, your first conversations about your symptoms and recovery will likely take place in the hospital. If you're noticing milder symptoms, you may decide that you want to talk to your doctor about changes in your thought processes, or you may seek care at the urging of a family member who arranges your appointment and goes with you.
You may start by seeing your primary care doctor, but he or she is likely to refer you to a doctor who specializes in disorders of the brain and nervous system (neurologist).
Because appointments can be brief, and there's often a lot of ground to cover, it's a good idea to be well prepared for your appointment. Here's some information to help you get ready and know what to expect from your doctor.
What you can do
- Be aware of any pre-appointment restrictions. When you make your appointment, ask if you need to fast for blood tests or if you need to do anything else to prepare for diagnostic tests.
- Write down all of your symptoms. Your doctor will want to know details about what's causing your concern about your memory or mental function. Make notes about some of the most important examples of forgetfulness, poor judgment or other lapses you want to mention. Try to remember when you first started to suspect that something might be wrong. If you think your difficulties are getting worse, be ready to describe them.
- Take along a family member or friend, if possible. Corroboration from a relative or trusted friend can play a key role in confirming that your difficulties are apparent to others. Having someone along can also help you recall all the information provided during your appointment.
- Make a list of your other medical conditions. Your doctor will want to know if you're currently being treated for diabetes, high blood pressure, heart disease, past strokes or any other conditions.
- Make a list of all your medications, including over-the-counter drugs and vitamins or supplements.
Writing down a list of questions ahead of time can help you remember your biggest concerns and allow you to make the most of your appointment. If you're seeing your doctor regarding concerns about vascular dementia, some questions to ask include:
- Do you think I have memory problems?
- Do you think my symptoms are due to circulation problems in my brain?
- What tests do I need?
- If I have vascular dementia, will you or another doctor manage my ongoing care? Can you help me get a plan in place to work with all my doctors?
- What treatments are available?
- Is there anything I can do that might help slow the progression of dementia?
- Are there any clinical trials of experimental treatments I should consider?
- What should I expect to happen over the long term? What steps do I need to take to prepare?
- Will my symptoms affect how I manage my other health conditions?
- Do you have any brochures or other printed material I can take home with me? What websites and support resources do you recommend?
In addition to the questions you've prepared ahead of time, don't hesitate to ask your doctor to clarify anything you don't understand.
What to expect from your doctor
Your doctor is also likely to have questions for you. Being ready to respond may free up time to focus on any points you want to talk about in-depth. Your doctor may ask:
- What kinds of thinking problems and mental lapses are you having? When did you first notice them?
- Are they steadily getting worse, or are they sometimes better and sometimes worse? Have they suddenly gotten worse?
- Has anyone close to you expressed concern about your thinking and reasoning?
- Have you started having problems with any long-standing activities or hobbies?
- Do you feel any sadder or more anxious than usual?
- Have you gotten lost lately on a driving route or in a situation that's usually familiar to you?
- Have you noticed any changes in the way you react to people or events?
- Do you have any change in your energy level?
- Are you currently being treated for high blood pressure, high cholesterol, diabetes, heart disease or stroke? Have you been treated for any of these in the past?
- What medications, vitamins or supplements are you taking?
- Do you drink alcohol or smoke? How much?
- Have you noticed any trembling or trouble walking?
- Are you having any trouble remembering your medical appointments or when to take your medication?
- Have you had your hearing and vision tested recently?
- Did anyone else in your family ever have trouble with thinking or remembering things as they got older? Was anyone ever diagnosed with Alzheimer's disease or dementia?
Jul 29, 2021
- Daroff RB, et al. Neuropsychology. In: Bradley's Neurology in Clinical Practice. 7th ed. Philadelphia, Pa.: Saunders Elsevier; 2016. https://www.clinicalkey.com. Accessed Feb. 16, 2018.
- AskMayoExpert. Vascular cognitive impairment. Rochester, Minn.: Mayo Foundation for Medical Education and Research; 2018.
- Wright CB. Etiology, clinical manifestations, and diagnosis of vascular dementia. https://www.uptodate.com/contents/search. Accessed Feb. 16, 2018.
- O'Brien JT, et al. Vascular dementia. The Lancet. 2015;386:1698.
- Dementia: Hope through research. National Institute of Neurological Disorders and Stroke. https://catalog.ninds.nih.gov/ninds/facet/Health-Topics/term/Dementia. Accessed Feb. 16, 2018.
- Wright CB. Treatment and prevention of vascular dementia. https://www.uptodate.com/contents/search. Accessed Feb. 16, 2018.
- Dichgans M, et al. Vascular cognitive impairment. Circulation. 2017;120:573.
- Medications for memory loss. Alzheimer's Association. https://www.alz.org/alzheimers_disease_standard_prescriptions.asp. Accessed Feb. 16, 2018.
- Early-stage caregiving. Alzheimer's Association. https://www.alz.org/care/alzheimers-early-mild-stage-caregiving.asp. Accessed Feb. 16, 2018.
- Graff-Radford J (expert opinion). Mayo Clinic. April 28, 2021.
- Symptoms & causes
- Doctors & departments
- Diseases & Conditions
- Vascular dementia diagnosis & treatment
News from Mayo Clinic
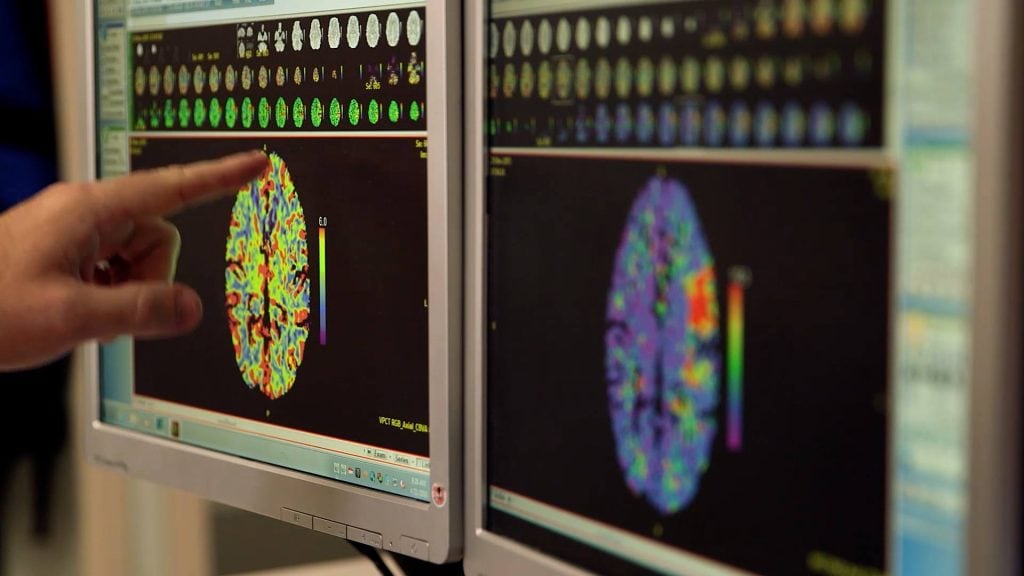
Associated Procedures
Products & services.
- A Book: Day to Day: Living With Dementia
- A Book: Mayo Clinic on Alzheimer's Disease
CON-XXXXXXXX
Help transform healthcare
Your donation can make a difference in the future of healthcare. Give now to support Mayo Clinic's research.

An official website of the United States government
The .gov means it’s official. Federal government websites often end in .gov or .mil. Before sharing sensitive information, make sure you’re on a federal government site.
The site is secure. The https:// ensures that you are connecting to the official website and that any information you provide is encrypted and transmitted securely.
- Publications
- Account settings
Preview improvements coming to the PMC website in October 2024. Learn More or Try it out now .
- Advanced Search
- Journal List
- Clin Interv Aging
- v.1(3); 2006 Sep
Vascular dementia: prevention and treatment
Background:.
Vascular dementia (VaD) is the most common cause of dementia in the elderly, second only to Alzheimer’s disease (AD). Between 1% and 4% of people of 65 years of age suffer from VaD and the prevalence appears to double every 5–10 years after the age of 65.
Prevention aims to reduce the disease by eliminating its cause or main risk factors, particularly hypertension as well as diabetes mellitus, atherosclerosis, coronary artery disease, smoking, lipid abnormalities, and hyperhomocystinemia. Initial studies of several agents for symptomatic treatment were disappointing. However, there is growing evidence for cholinergic involvement in VaD and recent studies with cholinesterase inhibitors have shown improvement in cognitive, global function, and activities of daily living as compared with placebo and have been well tolerated.
Conclusion:
VaD is a common condition and its prevalence is likely to increase. As physicians we need to be diligent with regards to recognition of risk factors and vigorous intervention. Promising results have been seen in several clinical trials of cholinesterase inhibitors and no safety of tolerability issues have been noted.
Introduction
Dementia is a syndrome of acquired intellectual deficit resulting in significant impairment of social or occupational functions. Vascular cognitive dementia (VaD) comprises dementias resulting from all types of vascular pathologies. The current classification of VaD includes: cortical vascular dementia; subcortical ischemic dementia; strategic-infarct dementia; hypoperfusion dementia; hemorrhagic dementia; and dementias resulting from specific arteriopathies ( O’Brien et al 2003 ).
The diagnosis requires the presence of cognitive decline (loss of memory and deficits in at least two other domains) resulting in impaired functional abilities. Evidence of cerebrovascular disease (CVD) must be confirmed by neuroimaging for diagnosis of probable VaD and dementia and CVD must be reasonably related in time or temporally. Several specific diagnostic criteria can be used to diagnose VaD including the Diagnostic Manual of Mental Disorders , 4th edition (DMS-IV) criteria, the International Classification of Diseases , 10th edition criteria, the National Institute of Neurological Disorders and Stroke Association International pour le Recherché at L’Enseignement en Neurosciences (NINDS-AIREN) criteria, the Alzheimer’s Disease Diagnostic and Treatment Centre criteria and the Hachinski Ischemic score.
The DSM-IV criteria have good sensitivity, but low specificity, but the NINDS-AIREN criteria are the most specific of all available criteria and are used most commonly in research (see Table Table1). 1 ). As not all patients fulfill the strict criteria for dementia and many may be significantly cognitively impaired without memory loss, the term vascular cognitive impairment (VCI) has been suggested. VCI includes VaD, but also encompasses mixed Alzheimer’s disease (AD) and VaD as well as vascular cognitive impairment without dementia and hereditary disorders. It is also becoming clear that there is an overlap between AD and CVD and the concept of mixed dementia has evolved. Patients will have clinical features of both AD and VaD and can share risk factors and pathogenic mechanisms ( Meyer et al 1999 ). Diagnosis is complex due to the current lack of appropriate clinical criteria and terminology ( Zekry et al 2002 ). However, in one recent Canadian cohort study (ACCORD), mixed dementia was found to represent as much as 33% of all dementias with “pure” VaD accounting for only 8.7% of dementia cases ( Feldman et al 2003 ).
NINDS-AIREN criteria for diagnosis of vascular dementia
Cerebrovascular disease: A relationship between the two manifested by one or more of the following: |
Abbreviations: CNS, central nervous system; CVD, cardiovascular disease; NINDS-AIREN, National Institute of Neurological Disorders and Stroke Association International pour le Recherché at L’Enseignement en Neurosciences.
VaD is historically considered the second most common cause of dementia in the elderly after AD ( Jorm 1991 ). Between 1% and 4% of people over 65 years suffer from VaD ( Hebert and Brayne 1995 ) and the prevalence appears to double every 5–10 years after the age of 65 years ( Hofman et al 1991 ). Post stroke dementia is extremely common and occurs in up to one third of patients with clinically evident ischemic stroke after 65 years ( Tatemicihi et al 1994 ; Pohjasvaara et al 1997 ). In a recent series ( Desmond et al 2000 ) dementia was reported in 26.3% of patients at three months after stroke. The subtypes were 57.1% with VaD, 38.7% with AD and stroke, and 4.2% with other causes.
Pohjasvaara and colleagues (2002) reported cognitive decline of any kind to be present in 61.7% of stroke patients ranging in age from 55 to 85 years. The prevalence of mixed dementia is much harder to measure as the concept is relatively new and most studies have used pathological evidence, however it is clear that CVD and AD frequently co-exist in the elderly population ( Snowdon et al 1997 ; Heyman et al 1998 ; MRCCFAS 2001 ). Autopsy series report that co-existing vascular pathology occurs in 24%–28% of AD cases ( Gearing et al 1995 ) and conversely half of patients with vascular disease who have become demented also have AD pathology ( O’Brien 1994 ).
Clinical features
Due to the variety of pathogenic mechanisms, the clinical manifestations of VaD can be varied and are determined by the size, location, and type of cerebral damage. Classically, the clinical features include an abrupt onset, stepwise deterioration, fluctuating course, and are often accompanied by focal motor and sensory abnormalities including early onset of urinary incontinence and gait disorders. However subcortical VaD can present with a gradual onset and deterioration like AD. Even within VaD, the clinical features can be further subdivided.
Cortical VaD
This is characterized by abrupt onset of unilateral sensorimotor changes along with aphasia, apraxia, or agnosia (cortical cognitive impairments). In particular, most patients have an element of executive dysfunctioning leading to difficulties in areas such as initiation, planning, and organization of activities. There may be day to day fluctuations in severity with long plateaus between events ( Erkinjuntti 1999 ).
Strategic infarct
Single strategic infarcts will produce cognitive and other deficits that entirely depend on the location of the infarct. Particular areas known to produce acute onset VaD include the thalamus, basal forebrain, and caudate ( Erkinjuntti 1999 ; O’Brien et al 2003 ). Cognitively, memory impairment, impaired executive function, confusion, and fluctuating levels of consciousness may occur. Behavioral changes include apathy, lack of spontaneity and perseveration ( Desmond et al 1999 ).
Subcortical VaD
Cerebrovascular lesions in the subcortical area tend to cause slow but episodic deterioration in executive functioning and abstract thought as well as mood changes including depression, personality changes, and emotional lability. Although memory deficits are less severe, the difficulties with complex tasks lead to decreased performance in activities of daily living (ADLs) ( Roman and Royall 1999 ).
With regard to the course of the disease as a whole, a recent study estimated median survival from dementia onset to death as 3.9 years for those with VaD as compared with 7.1 years for AD and 5.4 years for mixed dementia ( Fitzpatrick et al 2005 ).
Risk factors for VaD
Risk factors for VaD can be divided into two groups: modifiable and non-modifiable. The two most important non-modifiable risk factors are gender and age, followed by genetic predisposition, ethnicity, and a previous history of stroke. Both incidence and prevalence of VaD increase with age and tend to be higher in men ( Zhang 1990 ; Hofman et al 1991 ; Ruitenburg et al 2001). Dementia affects around 7% of the general population older than 65 years and 30% of people older than 80. As previously mentioned the prevalence doubles every 5–10 years after the age of 65 years ( Hofman et al 1991 ). Genetic defects for several monogenic disorders have been identified in the last decade. These include cerebral autosomal dominant arteriopathy with subcortical infarcts and leucoencephalopathy (CADASIL) which is a cause of small vessel disease and stroke leading to cognitive impairment up to 20 years after the onset of symptoms. Other genetic disorders include hereditary cerebral hemorrhage with amyloidosis which leads to cognitive impairment or dementia in the majority of patients with this disorder ( Thomas et al 2000 ).
Ethnicity appears to be of importance when one considers that previous studies suggest that VaD represents over 50% of dementias in Japan ( Ueda et al 1992 ) (however there may have been differences in ascertainment of cases with over-diagnosis of VaD). Conversely, in a recent study of four major regional centers in China, the prevalence of dementia subtypes in China was comparable with that in Western countries ( Zhang et al 2005 ).
There is a history of prior stroke in 76% of patients with vascular dementia and in 57% of those with vascular cognitive impairment as compared with only 5%–7% of people with AD ( Rockwood 1997 ).
The modifiable risk factors for VaD should be those that reduce CVD ie, hypertension, diabetes mellitus (DM), ischemic heart disease (IHD), peripheral vascular disease (PVD), white matter lesions, smoking, and hyperlipidemia ( Desmond et al 1993 ; Meyer et al 1995; Rockwood 1997 ).
Epidemiological data shows that hypertension is one of the most potent risk factors for VaD and it has been shown that control of hypertension can decrease the risk of VaD (In’t Veld et al 2001 ).
With regards to diabetes and the risk for VaD, Luchsinger and colleagues (2001) have shown that in a population of elderly subjects followed up for an average of 4.6 years, those with diabetes were over three times more likely to experience stroke-associated dementia.
White matter lesions seen on magnetic resonance imaging (MRI) scans of elderly patients with CVD strongly correlated with the development of dementia ( Liu et al 1992 ) implying that neuroimaging may prove a useful tool as a predictor of cognitive decline in post stroke patients.
Cognitive impairment is seen in 26% of patients discharged from hospital after treatment for heart failure, correlating with the degree of left ventricular dysfunction and systolic blood pressure levels below 130 mm Hg ( Cacciatore et al 1998 ).
Coronary artery bypass graft (CABG) surgery is increasingly common, with a particular increase in the older population. In this group, widespread atherosclerotic disease can predispose to vascular sequele leading to neurological dysfunction. This has been the subject of a recent review ( Royter et al 2005 ). The reported incidence of early cognitive disturbance ranges from 33% to 83%. Long term cognitive outcome seems to be more favourable for off-pump CABG, but late post-operative dementia is predicted by early cognitive deterioration. It has also been suggested that aggressive post-operative risk factor control could favorably impact on cognitive outcome ( Ross et al 1999 ).
Dyslipidemia, a well established risk factor for ischemic heart disease, has not yet been convincingly demonstrated as a factor associated with VaD or AD. However, a recent cross sectional and prospective study of 4316 patients in the US showed that elevated levels of non-high-density lipoprotein cholesterol (non-HDL-C), low-density lipoprotein cholesterol (LDL-C) and decreased levels of HDL-C were weak risk factors for VaD ( Reitz et al 2004 ).
The evidence for smoking and dementia is somewhat ambivalent however a recent study showed that current smokers had an increased risk of AD (relative risk [RR]=1.98; confidence index [CI]=1.63–5.42) and VaD (RR=1.98; CI=1.53–3.12) whereas past smokers did not have an increased risk of dementia ( Juan et al 2004 ) (Table (Table2 2 ).
Common risk factors for vascular dementia
Age |
Gender (male) |
Ethnicity |
Hypertension |
Atherosclerosis |
Hyperlipidemia |
Diabetes mellitus |
Smoking |
Atrial fibrillation |
Hyperhomocystinemia |
Primary prevention
Primary prevention aims to reduce the incidence of VaD by early detection and optimum treatment of known vascular factors for CVD and stroke ( O’Brien et al 2003 ). Targeting high risk groups (elderly patients, patients with hypertension, diabetes, atrial fibrillation, past transient ischemic attack or stroke, hypercholesterolemia, and smokers) affords the best chance of minimizing dementia in the population. As well as simple measures such as recommending lifestyle changes and optimizing control of diabetes, control of hypertension appears to be of paramount importance. Initially evidence for the effects of antihypertensive treatment on dementia was conflicting. Epidemiological data came from the Rotterdam study which was a longitudinal study of 7046 elderly and showed that the relative risk of vascular dementia was reduced by over one third over a mean of 2.2 years follow up in those who were receiving antihypertensives at baseline (In’t Veld et al 2001 ). Two subsequent prospective studies were less positive. The Medical Research Council (MRC) trial of hypertension in older adults with a diuretic/β blocker-based regimen showed no benefit in prevention of dementia ( MRC 1985 ) and the Systolic Hypertension in the Elderly Programme (SHEP) study showed no protective effect against dementia with treatment with diuretics +/−β blockers ( SHEP 1991 ). However further evaluation of this trial has revealed that cognitive and functional evaluations may have been biased toward the null effect by differential dropout, thus obscuring the appraisal of a protective effect of treatment on the cognitive and functional decline ( Di Bari et al 2001 ). More positive evidence came in the form of the Systolic Hypertension in Europe trial which demonstrated a 55% reduction in the incidence of dementia in the active treatment group (receiving a calcium channel blocker) over two years. However only small numbers of new cases of dementia were identified and therefore the results should be interpreted with caution ( Pahor et al 1999 ).
Secondary prevention
In secondary prevention, the target is stroke management and prevention of recurrent stroke. More recently, the Perindopril Protection Against Recurrent Stroke Study (PROGRESS) trial confirmed the benefits of blood pressure lowering in secondary prevention ( PROGRESS 2001 ). Although the primary outcome of PROGRESS was stroke incidence, dementia and cognitive function were secondary outcomes. The active treatment group received the long-acting angiotensin-converting enzyme (ACE) inhibitor perindopril combined with the diuretic indapamide. After four years of follow up in the entire population, the reduction in risk of dementia in the active treatment group was 12% (not significant). However, a significant reduction in dementia of 34% in the active treatment group was observed in patients with recurrent stroke (p=0.03). A similar pattern was seen for cognitive decline with an overall risk reduction of 19% with active treatment in the whole population (p=0.01) but a significant risk reduction of 45% in the group with prior stroke (p=0.001). Combination therapy induced a mean difference of blood pressure of 12/5 mm Hg and was more effective in reducing the risk of dementia than monotherapy, the difference of blood pressure on monotherapy being 5/3 mm Hg.
Among the patients without cognitive impairment at baseline, a 50% reduction in the risk of dementia was seen in those with prior stroke compared with a 16% reduction in those without stroke.
Further evidence was demonstrated in a PROGRESS sub study (MRI study). This looked at whether blood pressure lowering could arrest the progression of white matter hyperintensities (WMH). We know that WMH are associated with cognitive impairment or dementia ( Breteler et al 1994 ; Longstreth et al 1996 ; Vermeer et al 2003 ) and are often observed on brain MRI in elderly patients ( Bots et al 1993 ; Breteler et al 1994 ; Lindgren et al 1994 ; Longstreth et al 1996 ; Dufouil et al 2001 ) and those who have suffered a stroke ( Miyao et al 1992 ; Van Zagten et al 1996 ; Inzitari 2003 ). At 36 months the mean total volume of new WMHs was significantly reduced in the active treatment group receiving perindopril +/− indapamide (p=0.012), therefore the results indicated that an active blood-pressure lowering regimen stopped or delayed the progression of WMHs in patients with CVD ( Dufouil et al 2005 ).
The treatment of another risk factor, hypercholesterolemia, has also become topical. It is clear that the use of 3-hydroxy-3 methylglutaryl coenzyme A (HMG-CoA) reductase inhibitors (ie, statins) have a well defined role in the secondary prevention of stroke. What is less obvious is their role in preventing cognitive decline. Observational studies have suggested that treatment with statins lower the risk of developing dementia or cognitive decline however most of the available data does not distinguish between AD, VaD and other forms of dementia ( Jick et al 2000 ; Kivipelto et al 2001 ; Rockwood et al 2002 ). The Heart Protection Study which investigated the effects of simvastatin on vascular outcomes including stroke, showed no significant benefit of simvastatin on 5-year cognitive outcomes ( HPSCG 2002 ). However, the study design for this particular outcome could be criticized as there was no measure of baseline cognitive function. The Prospective Study of Pravastatin in the Elderly at Risk study (PROSPER) followed 6000 individuals aged 70 to 82 for 3.2 years. Pravastatin reduced the risk of coronary disease in elderly individuals but had no significant benefit on stroke, cognition, or ADL. It has been hypothesized though, that the studies involving statins have been of insufficient duration to detect cognitive benefits ( Shepherd et al 2002 ).
Other less studied strategies for prevention of VaD focus on prompt stroke diagnosis and treatment and good after care. They should impact on cognitive function at least through event reduction. These include: early intervention (within 3 hours) in selected patients with stroke to achieve reperfusion with thrombolytic agents, antiplatelet agents for patients with past transient ischemic attack (TIA) or non-hemorrhagic stroke, anticoagulants for atrial fibrillation as indicated, carotid endarterectomy for severe carotid stenosis and intensive rehabilitation after stroke ( Sachdev et al 1999 ).
Symptomatic treatment
Initial studies of several agents in VaD including vasodilators, nootropics, antithrombotics, ergot alkaloids, antioxidants, hyperbaric oxygen and thyrotropin-releasing hormone analogue have had mostly negative results ( Erkinjuntti 1999 ; Roman 2000 ). However the study designs were not ideal as they were based on small numbers, had short treatment periods and there were varying end points of the various trials.
Propentofylline is a glial modulator which has been studied in more detail. Several double blind, placebo-controlled randomized, parallel group trials carried out in Europe and Canada showed significant symptom improvement and long term efficacy in the cognitive subscale of the Alzheimer’s Disease Assessment Scale (ADAS-cog) and Clinician’s Interview Based Impression of Change (CIBIC-plus) up to 48 weeks compared with placebo ( Kittner et al 1997 ; Mielke et al 1998 ). The calcium antagonist nimodipine which is known to have specific effects on small vessels was studied in a double-blind, placebo controlled study in VaD. Although there was no overall evidence of efficacy in VaD, nimodipine had beneficial effects on attention and psychomotor performances in the subcortical group ( Pantoni et al 2000 ).However, the effects appear to be short term. Memantine, which is a moderate affinity noncompetitive N-methyl-D-aspartate receptor antagonist, is another treatment which shows promise and is well tolerated. It is licensed for use in moderate AD but a number of studies of memantine in VaD have shown improvement in mini-mental state examination (MMSE) as well as functional levels and reduced care dependency compared with placebo. Interestingly, the cognitive treatment effect for memantine was more pronounced in the small vessel group ( Gortelmeyer and Erbler 1992 ; Mobius and Stoffler 2002 ; Wilcock et al 2002 ).
There is growing evidence for cholinergic involvement in VaD. Autopsy patients with VaD have shown significantly reduced choline acetyl-transferase activity in several brain regions including the caudate and putamen, hippocampus and temporal lobe cortex ( Gottfries et al 1994 ). This equates to a 40% loss of cholinergic neurons as compared with 70% in AD cases ( Court et al 2002 ). Again, in rodent models such as the stroke-prone spontaneously-hypertensive rats, there is a significantly reduced level of cholinergic markers including acetylcholine in the neocortex, hippocampus, and cerebrospinal fluid that appears to correlate with impaired learning and memory ( Gottfries et al 1994 ; Kimura et al 2000 ). It is reasonable therefore to hypothesize that in a similar way to AD, enhancing cholinergic transmission may be a rational approach to treatment of VaD. As a result, three of the acetylcholinesterase inhibitors approved for use in AD: donepezil, rivastigmine, and galantamine have also been used in VaD. Rivastigmine is a second generation cholinesterase inhibitor with the capacity to inhibit both acetylcholinesterase and butyrylcholinesterase. In a randomized open label one year study, 208 patients with VaD were treated with rivastigmine. There was slight improvement in executive function (clock drawing tests) and in behavior ( Moretti et al 2003 ), however the results of a randomized double-blind trial with rivastigmine are awaited. The most positive results have been seen with donepezil and galantamine. Donepezil is a piperidine-based agent and is a noncompetitive, reversible antagonist of cholinesterase and is highly selective for acetyl-cholinesterase. Efficacy and safety has been shown in two large randomized placebo-controlled trials ( Black et al 2003 ; Wilkinson et al 2003 ) and confirmed in a recent Cochrane review ( Malouf and Birks 2004 ). Altogether 1219 patients with VaD, according to the NINDS-AIREN criteria, were recruited for 24 week trials. The patients were randomized to one of three groups: placebo, donepezil 5 mg per day, or donepezil 10 mg per day. From week 6 through week 24 both active treatment groups showed statistically significant improvement in cognitive, global function, and ADLs as compared with placebo and the drug was well tolerated. Galantamine is a cholinesterase inhibitor that also modulates central nicotinic receptors. In a multicenter, double-blind 6 month randomized control trial, patients diagnosed with probable VaD or AD combined with CVD received galantamine or placebo. Primary end points were cognition and global functioning and secondary end points included assessment of behavioral symptoms according to the Neuropsychiatric Inventory (NPI) and ADL using the Disability Assessment in Dementia (DAD) ( Cummings et al 1994 ). In analysis of both groups as a whole, galantamine demonstrated efficacy on all outcome measures. It showed greater efficacy than placebo on ADAS-cog (2.7 points; p<0.001) and CIBIC-plus (74% vs 59% of patients remained stable or improved; p<0.001). ADL and behavioral symptoms were also significantly improved compared with placebo (both p<0.05) ( Erkinjuntti et al 2002 ). Although the overall population showed improvement, the VaD subgroup changes did not achieve significance. There are two further large randomized controlled trials in VaD with galantamine awaiting publication. Preliminary results have shown that while benefits were seen for galantamine in executive dysfunction and cognition, the global outcomes were unaffected.
Vascular dementia is a common condition and its prevalence is likely to increase. As physicians we need to be diligent with regards to recognition of risk factors and vigorous intervention. Promising results have been seen in several clinical trials of cholinesterase inhibitors and no safety or tolerability issues have been noted.
- Black S, Roman GC, Geldmacher DS, et al.Donepezil 307 Vascular Dementia Study Group Efficacy and tolerability of donepezil in vascular dementia: positive results of a 24-week, multicenter, international, randomized, placebo-controlled clinical trial. Stroke. 2003; 34 :2323–30. [ PubMed ] [ Google Scholar ]
- Bots ML, van Swieten JC, Breteler MM, et al. Cerebral white matter lesions and atherosclerosis in the Rotterdam Study. Lancet. 1993; 341 :1232–7. [ PubMed ] [ Google Scholar ]
- Breteler MM, van Amerongen NM, van Swieten JC, et al. Cognitive correlates of ventricular enlargement and cerebral white matter lesions on magnetic resonance imaging. The Rotterdam Study. Stroke. 1994; 25 :1109–15. [ PubMed ] [ Google Scholar ]
- Breteler MM, van Swieten JC, Bots ML, et al. Cerebral white matter lesions, vascular risk factors, and cognitive function in a population-based study: the Rotterdam Study. Neurology. 1994; 44 :1246–52. [ PubMed ] [ Google Scholar ]
- Cacciatore F, Abete P, Ferrara N, et al. Congestive heart failure and cognitive impairment in an older population. Osservatorio Geriatrico Campano Study Group. J Am Geriatr Soc. 1998; 46 :1343–8. [ PubMed ] [ Google Scholar ]
- Court JA, Perry EK, Kalaria RN. Neurotransmitter control of the cerebral vasculature and abnormalities in vascular dementia. In: Erkinjuntti T, Gauthier S, editors. Vascular cognitive impairment. London: Martin Duniz Ltd; 2002. pp. 167–85. [ Google Scholar ]
- Cummings JL, Mega M, Gray K, et al. The neuropsychiatric inventory: comprehensive assessment of psychopathology in dementia. Neurology. 1994; 44 :2308–14. [ PubMed ] [ Google Scholar ]
- Desmond DW, Erkinjuntti T, Sano M, et al. The cognitive syndrome of vascular dementia: implications for clinical trials. Alzheimer Dis Assoc Disord. 1999; 13 (Suppl 3):S21–9. [ PubMed ] [ Google Scholar ]
- Desmond DW, Moroney JT, Paik MC, et al. Frequency and clinical determinants of dementia after ischemic stroke. Neurology. 2000; 54 :1124–31. [ PubMed ] [ Google Scholar ]
- Desmond DW, Tatemichi TK, Paik MC, et al. Risk factors for cerebrovascular disease as correlates of cognitive function in a stroke-free cohort. Arch Neurol. 1993; 50 :162–6. [ PubMed ] [ Google Scholar ]
- Di Bari M, Pahor M, Franse LV, et al. Dementia and disability outcomes in large hypertension trials: lessons learned from the systolic hypertension in the elderly program (SHEP) trial. Am J Epidemiol. 2001; 153 :72–8. [ PubMed ] [ Google Scholar ]
- Dufouil C, Chalmers J, Coskun O, et al.PROGRESS MRI Substudy Investigators Effects of blood pressure lowering on cerebral white matter hyperintensities in patients with stroke: the PROGRESS (Perindopril Protection Against Recurrent Stroke Study) Magnetic Resonance Imaging Substudy. Circulation. 2005; 112 :1644–50. [ PubMed ] [ Google Scholar ]
- Dufouil C, de Kersaint-Gilly A, Besancon V, et al. Longitudinal study of blood pressure and white matter hyperintensities: the EVA MRI Cohort. Neurology. 2001; 56 :921–6. [ PubMed ] [ Google Scholar ]
- Erkinjuntti T. Cerebrovascular dementia: pathophysiology, diagnosis and treatment. CNS Drugs. 1999; 12 :35–48. [ Google Scholar ]
- Erkinjuntti T, Kurz A, Gauthier S, et al. Efficacy of galantamine in probable vascular dementia and Alzheimer’s disease combined with cerebrovascular disease: a randomised trial. Lancet. 2002; 359 :1283–90. [ PubMed ] [ Google Scholar ]
- Feldman H, Levy AR, Hsiung GY, et al.ACCORD Study Group A Canadian cohort study of cognitive impairment and related dementias (ACCORD): study methods and baseline results. Neuroepidemiology. 2003; 22 :265–74. [ PubMed ] [ Google Scholar ]
- Fitzpatrick AL, Kuller LH, Lopez OL, et al. Survival following dementia onset: Alzheimer’s disease and vascular dementia. J Neurol Sci. 2005:229–30. 43–9. [ PubMed ] [ Google Scholar ]
- Gearing M, Mirra SS, Hedreen JC, et al. The consortium to establish a registry for Alzheimer’s disease (CERAD). Part X. Neuropathology confirmation of the clinical diagnosis of Alzheimer’s disease. Neurology. 1995; 45 :461–6. [ PubMed ] [ Google Scholar ]
- Gortelmeyer R, Erbler H. Memantine in the treatment of mild to moderate dementia syndrome. A double-blind placebo-controlled study. Arzneimittel-Forschung. 1992; 42 :904–13. [ PubMed ] [ Google Scholar ]
- Gottfries CG, Blennow K, Karlsson I, et al. The neurochemistry of vascular dementia. Dementia (Basel, Switzerland) 1994; 5 :163–7. [ PubMed ] [ Google Scholar ]
- [HPSCG] Heart Protection Study Collaborative Group MRC/BHF Heart Protection Study of cholesterol lowering with simvastatin in 20,536 high-risk individuals: a randomised placebo-controlled trial. Lancet. 2002; 360 :7–22. [ PMC free article ] [ PubMed ] [ Google Scholar ]
- Hebert R, Brayne C. Epidemiology of vascular dementia. Neuroepidemiology. 1995; 14 :240–57. [ PubMed ] [ Google Scholar ]
- Heyman A, Fillenbaum GG, Welsh-Bohmer KA, et al. Cerebral infarcts in patients with autopsy-proven Alzheimer’s disease: CERAD, part XVIII. Consortium to Establish a Registry for Alzheimer’s Disease. Neurology. 1998; 51 :159–62. [ PubMed ] [ Google Scholar ]
- Hofman A, Rocca WA, Brayne C, et al. The prevalence of dementia in Europe: a collaborative study of 1980–1990 findings. Eurodem Prevalence Research Group. Int J Epidemiol. 1991; 20 :736–48. [ PubMed ] [ Google Scholar ]
- in’t Veld BA, Ruitenberg A, Hofman A, et al. Antihypertensive drugs and incidence of dementia: the Rotterdam Study. Neurobiol Aging. 2001; 22 :407–12. [ PubMed ] [ Google Scholar ]
- Inzitari D. Leukoaraiosis: an independent risk factor for stroke? Stroke. 2003; 34 :2067–71. [ PubMed ] [ Google Scholar ]
- Jick H, Zornberg GL, Jick SS, et al. Statins and the risk of dementia. Lancet. 2000; 356 :1627–31. [ PubMed ] [ Google Scholar ]
- Jorm AF. Cross-national comparisons of the occurrence of Alzheimer’s and vascular dementias. Eur Arch Psychiatry Clin Neurosci. 1991; 240 :218–22. [ PubMed ] [ Google Scholar ]
- Juan D, Zhou DH, Li J, et al. A 2-year follow-up study of cigarette smoking and risk of dementia. Eur J Neurol. 2004; 11 :277–82. [ PubMed ] [ Google Scholar ]
- Kimura S, Saito H, Minami M, et al. Pathogenesis of vascular dementia in stroke-prone spontaneously hypertensive rats. Toxicology. 2000; 153 :167–78. [ PubMed ] [ Google Scholar ]
- Kittner B, Rossner M, Rother M. Clinical trials in dementia with propentofylline. Ann N Y Acad Sci. 1997; 826 :307–16. [ PubMed ] [ Google Scholar ]
- Kivipelto M, Helkala EL, Laakso MP, et al. Midlife vascular risk factors and Alzheimer’s disease in later life: longitudinal, population based study. BMJ. 2001; 322 :1447–51. [ PMC free article ] [ PubMed ] [ Google Scholar ]
- Lindgren A, Roijer A, Rudling O, et al. Cerebral lesions on magnetic resonance imaging, heart disease, and vascular risk factors in subjects without stroke. A population-based study. Stroke. 1994; 25 :929–34. [ PubMed ] [ Google Scholar ]
- Liu CK, Miller BL, Cummings JL, et al. A quantitative MRI study of vascular dementia” Neurology. 1992; 42 :138–43. [ PubMed ] [ Google Scholar ]
- Longstreth WT, Jr, Manolio TA, Arnold A, et al. Clinical correlates of white matter findings on cranial magnetic resonance imaging of 3301 elderly people. The Cardiovascular Health Study. Stroke. 1996; 27 :1274–82. [ PubMed ] [ Google Scholar ]
- Luchsinger JA, Tang MX, Stern Y, et al. Diabetes mellitus and risk of Alzheimer’s disease and dementia with stroke in a multiethnic cohort. Am J Epidemiol. 2001; 154 :635–41. [ PubMed ] [ Google Scholar ]
- Malouf R, Birks J. Donepezil for vascular cognitive impairment. Cochrane Database Syst Rev. 2004; 1 :CD004395. [ PubMed ] [ Google Scholar ]
- Meyer JS, Rauch GM, Crawford K, et al. Risk factors accelerating cerebral degenerative changes, cognitive decline and dementia. Int J Geriatr Psychiatry. 1999; 14 :1050–61. [ PubMed ] [ Google Scholar ]
- Mielke R, Moller HJ, Erkinjuntti T, et al. Propentofylline in the treatment of vascular dementia and Alzheimer-type dementia: overview of phase I and phase II clinical trials. Alzheimer Dis Assoc Disord. 1998; 12 (Suppl 2):S29–35. [ PubMed ] [ Google Scholar ]
- Miyao S, Takano A, Teramoto J, et al. Leukoaraiosis in relation to prognosis for patients with lacunar infarction. Stroke. 1992; 23 :1434–8. [ PubMed ] [ Google Scholar ]
- Mobius HJ, Stoffler A. New approaches to clinical trials in vascular dementia: memantine in small vessel disease. Cerebrovasc Dis. 2002; 13 (Suppl 2):61–6. [ PubMed ] [ Google Scholar ]
- Moretti R, Torre P, Antonello RM, et al. Rivastigmine in subcortical vascular dementia: a randomized, controlled, open 12-month study in 208 patients. Am J Alzheimers Dis Other Demen. 2003; 18 :265–72. [ PMC free article ] [ PubMed ] [ Google Scholar ]
- [MRC] Medical Research Council Working Party MRC trial of treatment of mild hypertension: principal results. Br Med J (Clin Res Ed) 1985; 291 :97–104. [ PMC free article ] [ PubMed ] [ Google Scholar ]
- [MRCCFAS] Neuropathology Group. Medical Research Council Cognitive Function and Aging Study Pathological correlates of late-onset dementia in a multicentre, community-based population in England and Wales. Neuropathology Group of the Medical Research Council Cognitive Function and Ageing Study (MRC CFAS) Lancet. 2001; 357 :169–75. [ PubMed ] [ Google Scholar ]
- O’Brien JT, Erkinjuntti T, Reisberg B, et al. Vascular cognitive impairment. Lancet Neurol. 2003; 2 :89–98. [ PubMed ] [ Google Scholar ]
- O’Brien MD. How does cerebrovascular disease cause dementia? Dementia. 1994; 5 :133–6. [ PubMed ] [ Google Scholar ]
- Pahor M, Somes GW, Franse LV, et al.1999 Prevention of dementia: Syst-Eur trial Lancet 353 235author reply 236–7 [ PubMed ] [ Google Scholar ]
- Pantoni L, Bianchi C, Beneke M, et al. The Scandinavian Multi-Infarct Dementia Trial: a double-blind, placebo-controlled trial on nimodipine in multi-infarct dementia. J Neurol Sci. 2000; 175 :116–23. [ PubMed ] [ Google Scholar ]
- Pohjasvaara T, Erkinjuntti T, Vataja R, et al. Dementia three months after stroke. Baseline frequency and effect of different definitions of dementia in the Helsinki Stroke Aging Memory Study (SAM) cohort. Stroke. 1997; 28 :785–92. [ PubMed ] [ Google Scholar ]
- [PROGRESS] PROGRESS Collaborative Group Randomised trial of a perindopril-based blood-pressure-lowering regimen among 6,105 individuals with previous stroke or transient ischaemic attack. Lancet. 2001; 358 :1033–41. [ PubMed ] [ Google Scholar ]
- Reitz C, Tang MX, Luchsinger J, et al. Relation of plasma lipids to Alzheimer disease and vascular dementia. Arch Neurol. 2004; 61 :705–14. [ PMC free article ] [ PubMed ] [ Google Scholar ]
- Rockwood K. Lessons from mixed dementia. Int Psychogeriatr. 1997; 9 :245–9. [ PubMed ] [ Google Scholar ]
- Rockwood K, Kirkland S, Hogan DB, et al. Use of lipid-lowering agents, indication bias, and the risk of dementia in community-dwelling elderly people. Arch Neurol. 2002; 59 :223–7. [ PubMed ] [ Google Scholar ]
- Roman G. Perspectives in the treatment of vascular dementia. Drugs Today. 2000; 36 :641–53. [ PubMed ] [ Google Scholar ]
- Roman GC, Royall DR. Executive control function: a rational basis for the diagnosis of vascular dementia. Alzheimer Dis Assoc Disord. 1999; 13 (Suppl 3):S69–80. [ PubMed ] [ Google Scholar ]
- Ross GW, Petrovitch H, White LR, et al. Characterization of risk factors for vascular dementia: the Honolulu-Asia Aging Study. Neurology. 1999; 53 :337–43. [ PubMed ] [ Google Scholar ]
- Royter V, M Bornstein N, Russell D. Coronary artery bypass grafting (CABG) and cognitive decline: a review. J Neurol Sci. 2005:229–230. 65–67. [ PubMed ] [ Google Scholar ]
- Ruitenberg A, Ott A, van Swieten JC, et al. Incidence of dementia: does gender make a difference? Neurobiol Aging. 2001; 22 :575–80. [ PubMed ] [ Google Scholar ]
- Sachdev PS, Brodaty H, Looi JC. Vascular dementia: diagnosis, management and possible prevention. Med J Aust. 1999; 170 :81–5. [ PubMed ] [ Google Scholar ]
- [SHEP] SHEP Cooperative Research Group Prevention of stroke by antihypertensive drug treatment in older persons with isolated systolic hypertension. Final results of the Systolic Hypertension in the Elderly Program (SHEP) JAMA. 1991; 265 :3255–64. [ PubMed ] [ Google Scholar ]
- Shepherd J, Blauw GJ, Murphy MB, et al.PROSPER study group. PROspective Study of Pravastatin in the Elderly at Risk Pravastatin in elderly individuals at risk of vascular disease (PROSPER): a randomised controlled trial. Lancet. 2002; 360 :1623–30. [ PubMed ] [ Google Scholar ]
- Snowdon DA, Greiner LH, Mortimer JA, et al. Brain infarction and the clinical expression of Alzheimer disease. The Nun Study. JAMA. 1997; 277 :813–17. [ PubMed ] [ Google Scholar ]
- Tatemichi TK, Desmond DW, Stern Y, et al. Cognitive impairment after stroke: frequency, patterns, and relationship to functional abilities. J Neurol Neurosurg Psychiatry. 1994; 57 :202–7. [ PMC free article ] [ PubMed ] [ Google Scholar ]
- Thomas NJ, Morris CM, Scaravilli F, et al. Hereditary vascular dementia linked to notch 3 mutations. CADASIL in British families. Ann N Y Acad Sci. 2000; 903 :293–8. [ PubMed ] [ Google Scholar ]
- Ueda K, Kawano H, Hasuo Y, et al. Prevalence and etiology of dementia in a Japanese community. Stroke. 1992; 23 :798–803. [ PubMed ] [ Google Scholar ]
- van Zagten M, Boiten J, Kessels F, et al. Significant progression of white matter lesions and small deep (lacunar) infarcts in patients with stroke. Arch Neurol. 1996; 53 :650–5. [ PubMed ] [ Google Scholar ]
- Vermeer SE, Prins ND, den Heijer T, et al. Silent brain infarcts and the risk of dementia and cognitive decline. New Engl J Med. 2003; 348 :1215–22. [ PubMed ] [ Google Scholar ]
- Wilcock G, Mobius HJ, Stoffler A, MMM 500 Group A double-blind, placebo-controlled multicentre study of memantine in mild to moderate vascular dementia (MMM500) Int Clin Psychopharmacol. 2002; 17 :297–305. [ PubMed ] [ Google Scholar ]
- Wilkinson D, Doody R, Helme R, et al.Donepezil 308 Study Group Donepezil in vascular dementia: a randomized, placebo-controlled study. Neurology. 2003; 61 :479–86. [ PubMed ] [ Google Scholar ]
- Zekry D, Hauw JJ, Gold G. Mixed dementia: epidemiology, diagnosis, and treatment. J Am Geriatr Soc. 2002; 50 :1431–8. [ PubMed ] [ Google Scholar ]
- Zhang M. Prevalence study on dementia and Alzheimer disease. Zhonghua yi xue za zhi. 1990; 70 :424–8. 30. [ PubMed ] [ Google Scholar ]
- Zhang ZX, Zahner GE, Roman GC, et al. Dementia subtypes in China: prevalence in Beijing, Xian, Shanghai, and Chengdu. Arch Neurol. 2005; 62 :447–53. [ PubMed ] [ Google Scholar ]

- Vascular dementia
- Report problem with article
- View revision history
Citation, DOI, disclosures and article data
At the time the article was created Jeremy Jones had no recorded disclosures.
At the time the article was last revised Francis Deng had no recorded disclosures.
- Multi-infarct dementia
- Vascular cognitive impairment
- Thalamic dementia
- Cortical vascular dementia
- Strategic infarct dementia
- Multiinfarct dementia
Vascular dementia , also known as vascular cognitive impairment , is the second most common cause of dementia after the far more common Alzheimer disease . It is primarily seen in patients with atherosclerosis and chronic hypertension and results from the accumulation of multiple white matter lesions or cortical infarcts, although cerebral hemorrhages can be variably included 4 .
On this page:
Terminology, epidemiology, clinical presentation, radiographic features, treatment and prognosis, history and etymology.
- Cases and figures
It is also possible to divide vascular dementia into subtypes, although no general agreement has been reached, and there is some overlap between them 4 .
- small vessel dementia (Binswanger disease)
- cortical vascular dementia roughly equivalent to multi-infarct dementia
- strategic infarct dementia
- thalamic dementia
Incidence has been variably reported but is strongly correlated with age, seen in only 1% of patients over the age of 55 years of age, but in over 4% of patients over 71 years of age 3 . To add to the confusion, given the prevalence of Alzheimer disease, the two are commonly seen together 4 . Additionally, some patients develop accelerated vascular changes due to specific underlying disease (e.g. CADASIL and Fabry disease ), and thus their demographics will be quite separate from the general population.
The presenting signs and symptoms are dependent on the areas affected and thus this is highly variable. Additionally, as these infarcts are accumulated in a stepwise fashion, deterioration is similarly stepwise rather than smoothly gradual.
Generally, cortical dementia and strategic infarct dementia, usually due to large vessel occlusion, have a definable time of onset and specific deficits related to the region affected 4 .
Subcortical or small vessel dementia, in contrast, has a more insidious onset and vaguer 'executive function' deficits 5 . The latter is therefore understandably more frequently in the differential list of other dementias.
Some authors have suggested that leukoaraiosis should involve at least 25% of the white matter in order to qualify for vascular dementia on imaging 5 . Moreover, increasing white matter lesions on imaging correlating with increasing cognitive decline is thought to be another feature in favor of vascular dementia 5 .
Both CT and MRI are able to provide evidence of ischemic damage, however, MRI is more sensitive, especially to white matter small vessel ischemic change as well as to microhemorrhages seen in cerebral amyloid angiopathy and chronic hypertensive encephalopathy .
It is beyond the scope of this article to describe all patterns of vascular damage, and the reader is referred to:
- cerebral infarction
- lacunar infarction
- intracerebral hemorrhage
As befits a heterogeneous group of conditions, no single treatment exists, with the majority of effort going into treating individual risk factors for ongoing vascular damage (e.g. hypertension, smoking, hypercoagulation, atherosclerosis).
Similarly, no single prognosis can be given, being largely dependent on the underlying cause and the degree to which successful treatment can be initiated.
Vladimir Hachinski ( fl. 2021) a Ukrainian-born Canadian neurologist, coined the terms leukoaraiosis and multi-infarct dementia and developed the Hachinski ischemic scale 6 .
- 1. Gorelick PB, Chatterjee A, Patel D et-al. Cranial computed tomographic observations in multi-infarct dementia. A controlled study. Stroke. 1992;23 (6): 804-11. Pubmed citation
- 2. Jayakumar PN, Taly AB, Shanmugam V et-al. Multi-infarct dementia: a computed tomographic study. Acta Neurol. Scand. 1989;79 (4): 292-5. Pubmed citation
- 3. van Straaten EC, Scheltens P, Knol DL et-al. Operational definitions for the NINDS-AIREN criteria for vascular dementia: an interobserver study. Stroke. 2003;34 (8): 1907-12. doi:10.1161/01.STR.0000083050.44441.10 - Pubmed citation
- 4. Iemolo F, Duro G, Rizzo C et-al. Pathophysiology of vascular dementia. Immun Ageing. 2009;6 (1): 13. doi:10.1186/1742-4933-6-13 - Free text at pubmed - Pubmed citation
- 5. Prins ND, Scheltens P. White matter hyperintensities, cognitive impairment and dementia: an update. (2015) Nature reviews. Neurology. 11 (3): 157-65. doi:10.1038/nrneurol.2015.10 - Pubmed
- 6. Goodman A. Vladimir Hachinski: a pioneer in the field of stroke. (2009) The Lancet. Neurology. 8 (1): 34. doi:10.1016/S1474-4422(08)70285-0 - Pubmed
Incoming Links
- Divry van Bogaert syndrome
- Normal pressure hydrocephalus
- Frontotemporal lobar degeneration
- Lacunar infarct
- Subcortical arteriosclerotic encephalopathy
- Cerebral autosomal recessive arteriopathy with subcortical infarcts and leukoencephalopathy (CARASIL)
- Cerebral small vessel disease
- Neurodegenerative protocol (MRI)
- Investigating altered consciousness (summary)
- Neurodegenerative MRI brain (an approach)
- Intracranial atherosclerotic disease
- Medical abbreviations and acronyms (V)
- Causes of dementia (mnemonic)
- Binswanger disease
Promoted articles (advertising)
ADVERTISEMENT: Supporters see fewer/no ads
By Section:
- Artificial Intelligence
- Classifications
- Imaging Technology
- Interventional Radiology
- Radiography
- Central Nervous System
- Gastrointestinal
- Gynaecology
- Haematology
- Head & Neck
- Hepatobiliary
- Interventional
- Musculoskeletal
- Paediatrics
- Not Applicable
Radiopaedia.org
- Feature Sponsor
- Expert advisers

- Overview & Symptoms
- Causes & Complications
- Diagnosis & Treatment
- Living With
- View Full Guide
Vascular Dementia

Vascular dementia , also known as multi-infarct dementia is the second most common cause of dementia in older people. Because it has a lower profile than Alzheimer's, many people don't suspect vascular dementia when forgetfulness becomes problematic. It's also difficult to diagnose so it's difficult to know exactly how many people suffer from vascular dementia. Current estimates attribute 15% to 20% of dementia cases in older adults to vascular dementia.
Determining the root cause can help determine the best action plan. If it's vascular dementia, certain lifestyle changes can help prevent further damage. WebMD takes a look at vascular dementia, its causes, symptoms, and prognosis.
What Is Vascular Dementia?
Compared to Alzheimer's disease , which happens when the brain 's nerve cells break down, vascular dementia happens when part of the brain doesn't get enough blood carrying the oxygen and nutrients it needs.
Though they happen in different ways, it is possible to have both vascular dementia and Alzheimer's disease . Discouraging as this sounds, there is ample reason to control the risk factors that contribute to vascular dementia. Allowing the condition to run its course without intervention can make Alzheimer's disease worse.
What Causes Vascular Dementia?
Vascular dementia occurs when vessels that supply blood to the brain become blocked or narrowed. Strokes take place when the supply of blood carrying oxygen to the brain is suddenly cut off. However, not all people with stroke will develop vascular dementia.
Vascular dementia can occur over time as "silent" strokes pile up. Quite often, vascular dementia draws attention to itself only when the impact of so many strokes adds up to significant disability. Avoiding and controlling risk factors such as diabetes, high blood pressure, smoking, and high cholesterol can help curb the risk of vascular dementia.
Catching the condition early also helps limit the impact and severity of vascular dementia. Early detection requires an awareness of risk factors and, more importantly, efforts to keep them under control. Anyone who suspects vascular dementia should talk with their doctor.

Symptoms of Vascular Dementia
Symptoms of vascular dementia depend on what part of the brain is affected and to what extent. Like Alzheimer's disease, the symptoms of vascular dementia are often mild for a long time. They may include:
- Problems with short-term memory
- Wandering or getting lost in familiar surroundings
- Laughing or crying at inappropriate times
- Trouble concentrating, planning, or following through on activities
- Trouble managing money
- Inability to follow instructions
- Loss of bladder or bowel control
- Hallucinations or delusions
Symptoms that suddenly get worse often signal a stroke . Doctors look for symptoms that progress in noticeable stages to diagnose vascular dementia. Alzheimer's, by comparison, progresses at a slow, steady pace. Another clue is impaired coordination or balance. In vascular dementia, problems walking or balancing can happen early. With Alzheimer's, these symptoms usually occur late in the disease.
Who Is at Risk for Vascular Dementia?
Some risk factors for vascular dementia can be managed; others, like age and gender, cannot. Among all factors, high blood pressure carries the greatest risk; vascular dementia almost never occurs without it.
Likewise, a high risk of stroke goes hand in hand with risk for vascular dementia. One-quarter to one-third of strokes are thought to result in some degree of dementia. People who smoke, consume excessive amounts of alcohol, have diabetes , or heart disease also have a higher rate of the condition.
Vascular dementia most commonly occurs in people between the ages of 60 and 75. Men seem to be more vulnerable than women, and the condition affects African-Americans more often than other races. People whose age, sex, or race puts them at increased risk of vascular dementia have that much more reason to manage risk factors within their control.
Vascular Dementia Treatment
Currently, no available treatments can repair the damage of vascular dementia once it's happened. Nonetheless, diagnosis provides important knowledge and the opportunity to prevent further damage.
Prevention typically involves bringing high blood pressure under control through exercise, diet, and medication . The same goes for diabetes if it exists. Patients should stop smoking and curb the use of alcohol.
Though medical options are limited, behavioral interventions such as cues and reminders can improve the quality of life for everyone involved. Family members and friends can place notes in visible locations around the house with daily plans and instructions for how to use basic items. Stepping up communication, reminding the person with vascular dementia what day it is, where they live, and what is going on in the family, can help keep them connected to the here and now.
Prognosis for People With Vascular Dementia
If the conditions that cause vascular dementia go untreated, the prognosis is not good. A person with vascular dementia may seem to improve for periods of time until another stroke takes away more brain function, memory, and independence. Eventually, untreated vascular dementia usually ends in death from stroke, heart disease , or infection.
Although vascular dementia is a serious condition, catching it early and preventing further damage are the best medicine. People with vascular dementia can work with their doctors and families to detect and manage the condition.
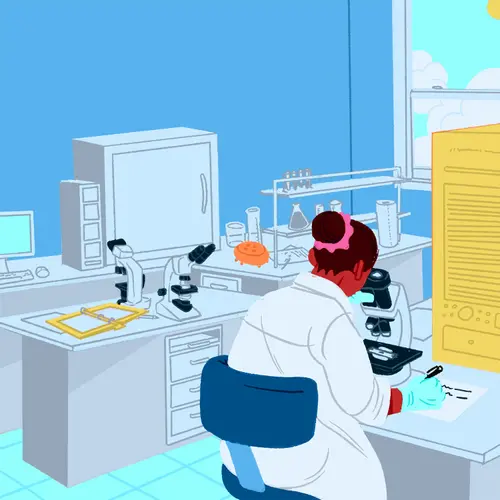
Top doctors in ,
Find more top doctors on, related links.
- Stroke News
- Stroke Reference
- Stroke Slideshows
- Stroke Videos
- Find a Neurologist
- Atrial Fibrillation
- Cholesterol Management
- Heart Disease
- Heart Failure
- High Blood Pressure
- Palliative Care
- My Medicine
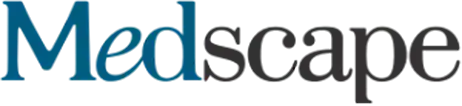
Neurological Manifestations of Vascular Dementia Clinical Presentation
- Author: Jasvinder Chawla, MD, MBA; Chief Editor: Niranjan N Singh, MBBS, MD, DM, FAHS, FAANEM more...
- Sections Neurological Manifestations of Vascular Dementia
- Practice Essentials
- Pathophysiology
- Epidemiology
- Laboratory Studies
- Imaging Studies
- Medical Care
- Behavioral Therapy
- Patient Education
- Questions & Answers
Criteria for the diagnosis of dementia require impairment in memory and at least 1 other cognitive domain (eg, orientation, language, praxis, executive functions, visuospatial abilities). These should be serious enough to affect activities of daily living and be consistently present to distinguish dementia from episodic impairments of consciousness such as delirium .
Currently, several sets of diagnostic criteria for vascular dementia exist. This list summarizes the main common criteria.
Vascular risk factors such as hypertension, coronary disease, and diabetes mellitus [ 17 ]
Specific evidence of cerebrovascular disease, eg, strokes and transient ischemic attacks: The cerebrovascular insult should precede (by no more than 3 months) or coincide with the onset or worsening of cognitive abnormalities.
Neuroimaging evidence of strokes
Lateralizing neurologic signs
Psychiatric disturbances (eg, emotional lability, depression, apathy)
Depression is a common comorbidity in patients with cerebrovascular disease and vascular dementia.
Medications should be reviewed because of the potential of drugs to interfere with alertness and cognition.
Differences between the cognitive disturbances in vascular dementia and Alzheimer disease are of limited value in discriminating vascular dementia from Alzheimer disease in a clinical setting.
Vascular dementia is thought to be associated with less significant memory dysfunction than Alzheimer disease. [ 3 ]
Frontal dysfunction due to widespread involvement of subcortical structures in vascular dementia is thought to lead to a dysexecutive syndrome with abulia and apathy.
A cognitively impaired patient with vascular risks factors but no history of cerebrovascular disease is most likely to have Alzheimer disease. Patients with dementia and vascular disease frequently have mixed pathology (ie, both Alzheimer disease and vascular dementia).
The physical examination should be focused on the cardiovascular system and neurologic localizing signs.
The temporal arteries may show decreased pulsatility, local tenderness, and thickening associated with giant cell arteritis. [ 5 ]
Funduscopic examination provides important information regarding end-organ effects of hypertension and diabetes mellitus.
Cardiac auscultation may detect rhythmic and valvular abnormalities.
Low scores on a standardized instrument (eg, Mini Mental Status Examination, Short Blessed questionnaire) can provide corroborating evidence of a cognitive disturbance.
Spasticity, hemiparesis, visual field defects, pseudobulbar palsy, and extrapyramidal signs confirm focal pathology.
Vascular dementia and cerebrovascular disease share risk factors, including age, male sex, diabetes mellitus, hypertension, cardiomyopathy, and possibly homocysteine levels.
So far, no relationship between cholesterol, serum lipoproteins, and the risk of vascular dementia is clearly indicated.
Evidence for tobacco consumption as a risk factor for vascular dementia is conflicting. However, a multiethnic, population-based study published in 2010 suggested that the brain is not immune to long-term consequences of heavy smoking. In fact, 21,123 heavy midlife smokers were followed for a mean of 23 years and were found more than 2 decades later to have a greater than 100% increased risk of dementia, Alzheimer disease, and vascular dementia. [ 18 ]
Limited alcohol consumption may be protective. [ 19 ]
Jellinger KA. Morphologic diagnosis of "vascular dementia" - a critical update. J Neurol Sci . 2008 Jul 15. 270(1-2):1-12. [QxMD MEDLINE Link] .
Jellinger KA. The pathology of "vascular dementia": a critical update. J Alzheimers Dis . 2008 May. 14(1):107-23. [QxMD MEDLINE Link] .
Schneck MJ. Vascular dementia. Top Stroke Rehabil . 2008 Jan-Feb. 15(1):22-6. [QxMD MEDLINE Link] .
Srinivasan V, Braidy N, Chan EK, Xu YH, Chan DK. Genetic and Environmental Factors in Vascular Dementia: an update of blood brain barrier dysfunction. Clin Exp Pharmacol Physiol . 2016 Feb 9. [QxMD MEDLINE Link] .
Solans-Laque R, Bosch-Gil JA, Molina-Catenario CA, Ortega-Aznar A, Alvarez-Sabin J, Vilardell-Tarres M. Stroke and multi-infarct dementia as presenting symptoms of giant cell arteritis: report of 7 cases and review of the literature. Medicine (Baltimore) . 2008 Nov. 87(6):335-44. [QxMD MEDLINE Link] .
Anderson P. 6 Steps to nondrug management of dementia. Medscape Medical News. December 27, 2012. [Full Text] .
Gitlin LN, Kales HC, Lyketsos CG. Nonpharmacologic management of behavioral symptoms in dementia. JAMA . 2012 Nov 21. 308(19):2020-9. [QxMD MEDLINE Link] .
Bousser MG. Stroke prevention: an update. Front Med . 2012 Mar. 6(1):22-34. [QxMD MEDLINE Link] .
Ancelin ML, Carrière I, Barberger-Gateau P, Auriacombe S, Rouaud O, Fourlanos S, et al. Lipid Lowering Agents, Cognitive Decline, and Dementia: The Three-City Study. J Alzheimers Dis . 2012 Mar 26. [QxMD MEDLINE Link] .
Fischer P, Krampla W, Mostafaie N, Zehetmayer S, Rainer M, Jungwirth S, et al. VITA study: white matter hyperintensities of vascular and degenerative origin in the elderly. J Neural Transm Suppl . 2007. 181-8. [QxMD MEDLINE Link] .
Chen C, Homma A, Mok VC, Krishnamoorthy E, Alladi S, Meguro K, et al. Alzheimer's disease with cerebrovascular disease: current status in the Asia-Pacific region. J Intern Med . 2016 Mar 16. [QxMD MEDLINE Link] .
Roach GW, Kanchuger M, Mangano CM. Adverse cerebral outcomes after coronary bypass surgery. Multicenter Study of Perioperative Ischemia Research Group and the Ischemia Research and Education Foundation Investigators. N Engl J Med . 1996 Dec 19. 335(25):1857-63. [QxMD MEDLINE Link] .
Ruchoux MM, Brulin P, Brillault J. Lessons from CADASIL. Ann N Y Acad Sci . 2002 Nov. 977:224-31. [QxMD MEDLINE Link] .
Qian L, Ding L, Cheng L, Zhu X, Zhao H, Jin J, et al. Early biomarkers for post-stroke cognitive impairment. J Neurol . 2012 Mar 10. [QxMD MEDLINE Link] .
Perrotta M, Lembo G, Carnevale D. Hypertension and Dementia: Epidemiological and Experimental Evidence Revealing a Detrimental Relationship. Int J Mol Sci . 2016 Mar 8. 17 (3): [QxMD MEDLINE Link] .
Knopman DS, Rocca WA, Cha RH. Incidence of vascular dementia in Rochester, Minn, 1985-1989. Arch Neurol . 2002 Oct. 59(10):1605-10. [QxMD MEDLINE Link] .
Erkinjuntti T. Vascular cognitive deterioration and stroke. Cerebrovasc Dis . 2007. 24 Suppl 1:189-94. [QxMD MEDLINE Link] .
Rusanen M, Kivipelto M, Quesenberry CP Jr, Zhou J, Whitmer RA. Heavy Smoking in Midlife and Long-term Risk of Alzheimer Disease and Vascular Dementia. Arch Intern Med . 2010 Oct 25. [QxMD MEDLINE Link] .
Mukamal KJ, Kuller LH, Fitzpatrick AL. Prospective study of alcohol consumption and risk of dementia in older adults. JAMA . 2003 Mar 19. 289(11):1405-13. [QxMD MEDLINE Link] .
Smith E. Vascular Cognitive Impairment. Continuum (Minneap Minn) . 2016 Apr. 22 (2 Dementia):490-509. [QxMD MEDLINE Link] .
Bowler JV. Acetylcholinesterase inhibitors for vascular dementia and Alzheimer's disease combined with cerebrovascular disease. Stroke . 2003 Feb. 34(2):584-6. [QxMD MEDLINE Link] .
Bowler JV. Modern concept of vascular cognitive impairment. Br Med Bull . 2007. 83:291-305. [QxMD MEDLINE Link] .
de Groot JC, de Leeuw FE, Oudkerk M. Cerebral white matter lesions and cognitive function: the Rotterdam Scan Study. Ann Neurol . 2000 Feb. 47(2):145-51. [QxMD MEDLINE Link] .
Deramecourt V, Slade JY, Oakley AE, Perry RH, Ince PG, Maurage CA, et al. Staging and natural history of cerebrovascular pathology in dementia. Neurology . 2012 Feb 29. [QxMD MEDLINE Link] .
Engelhart MJ, Geerlings MI, Ruitenberg A. Diet and risk of dementia: Does fat matter?: The Rotterdam Study. Neurology . 2002 Dec 24. 59(12):1915-21. [QxMD MEDLINE Link] .
Freels S, Nyenhuis DL, Gorelick PB. Predictors of survival in African American patients with AD, VaD, or stroke without dementia. Neurology . 2002 Oct 22. 59(8):1146-53. [QxMD MEDLINE Link] .
Iadecola C, Gorelick PB. Converging pathogenic mechanisms in vascular and neurodegenerative dementia. Stroke . 2003 Feb. 34(2):335-7. [QxMD MEDLINE Link] .
Knopman DS. Cerebrovascular pathology in cognitive impairment: New (in)sights. Neurology . 2012 Feb 29. [QxMD MEDLINE Link] .
Knopman DS, Rocca WA, Cha RH. Survival study of vascular dementia in Rochester, Minnesota. Arch Neurol . 2003 Jan. 60(1):85-90. [QxMD MEDLINE Link] .
Meguro K, Ishii H, Yamaguchi S. Prevalence of dementia and dementing diseases in Japan: the Tajiri project. Arch Neurol . 2002 Jul. 59(7):1109-14. [QxMD MEDLINE Link] .
Mok VC, Liu T, Lam WW, Wong A, Hu X, Guo L, et al. Neuroimaging predictors of cognitive impairment in confluent white matter lesion: volumetric analyses of 99 brain regions. Dement Geriatr Cogn Disord . 2008. 25(1):67-73. [QxMD MEDLINE Link] .
Mok VC, Wong A, Lam WW. Cognitive impairment and functional outcome after stroke associated with small vessel disease. J Neurol Neurosurg Psychiatry . 2004 Apr. 75(4):560-6. [QxMD MEDLINE Link] .
Moretti R, Torre P, Antonello RM, Cazzato G, Pizzolato G. Different responses to rivastigmine in subcortical vascular dementia and multi-infarct dementia. Am J Alzheimers Dis Other Demen . 2008 Apr-May. 23(2):167-76. [QxMD MEDLINE Link] .
Morris JC. The nosology of dementia. Neurol Clin . 2000 Nov. 18(4):773-88. [QxMD MEDLINE Link] .
Orgogozo JM, Rigaud AS, Stoffler A. Efficacy and safety of memantine in patients with mild to moderate vascular dementia: a randomized, placebo-controlled trial (MMM 300). Stroke . 2002 Jul. 33(7):1834-9. [QxMD MEDLINE Link] .
Polidori MC, Pientka L. A brief update on dementia prevention. Z Gerontol Geriatr . 2012 Jan. 45(1):7-10. [QxMD MEDLINE Link] .
Quinn J. Vascular dementia. J Am Med Dir Assoc . 2003 Nov-Dec. 4(6 Suppl):S155-61. [QxMD MEDLINE Link] .
Rao R. The role of carotid stenosis in vascular cognitive impairment. J Neurol Sci . 2002 Nov 15. 203-204:103-7. [QxMD MEDLINE Link] .
Roman GC, Tatemichi TK, Erkinjuntti T. Vascular dementia: diagnostic criteria for research studies. Report of the NINDS-AIREN International Workshop. Neurology . 1993 Feb. 43(2):250-60. [QxMD MEDLINE Link] .
Sha MC, Callahan CM. The efficacy of pentoxifylline in the treatment of vascular dementia: a systematic review. Alzheimer Dis Assoc Disord . 2003 Jan-Mar. 17(1):46-54. [QxMD MEDLINE Link] .
Vermeer SE, Prins ND, den Heijer T. Silent brain infarcts and the risk of dementia and cognitive decline. N Engl J Med . 2003 Mar 27. 348(13):1215-22. [QxMD MEDLINE Link] .
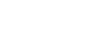
Contributor Information and Disclosures
Jasvinder Chawla, MD, MBA Chief of Neurology, Hines Veterans Affairs Hospital; Professor of Neurology, Loyola University Medical Center Jasvinder Chawla, MD, MBA is a member of the following medical societies: American Academy of Neurology , American Association of Neuromuscular and Electrodiagnostic Medicine , American Clinical Neurophysiology Society , American Medical Association Disclosure: Nothing to disclose.
Jaime Grutzendler, MD Assistant Professor, Department of Neurology and Physiology, Northwestern University School of Medicine Jaime Grutzendler, MD is a member of the following medical societies: American Academy of Neurology , Society for Neuroscience Disclosure: Nothing to disclose.
Giovanni d'Avossa, MD Lecturer, School of Psychology, Bangor University; NHS Honorary Consultant in Neurology Disclosure: Nothing to disclose.
Fredy J Revilla, MD, FAAN, FANA Clinical Professor, University of South Carolina School of Medicine, Greenville; Chief, Division of Neurology, UMG Neuroscience Associates, Prisma Health-Upstate Fredy J Revilla, MD, FAAN, FANA is a member of the following medical societies: American Academy of Neurology , American Neurological Association , Huntington Study Group, International Parkinson and Movement Disorder Society , Parkinson Study Group, Society for Neuroscience Disclosure: Nothing to disclose.
Francisco Talavera, PharmD, PhD Adjunct Assistant Professor, University of Nebraska Medical Center College of Pharmacy; Editor-in-Chief, Medscape Drug Reference Disclosure: Received salary from Medscape for employment. for: Medscape.
Jorge C Kattah, MD Head, Associate Program Director, Professor, Department of Neurology, University of Illinois College of Medicine at Peoria Jorge C Kattah, MD is a member of the following medical societies: American Academy of Neurology , American Neurological Association , New York Academy of Sciences Disclosure: Nothing to disclose.
Niranjan N Singh, MBBS, MD, DM, FAHS, FAANEM Adjunct Associate Professor of Neurology, University of Missouri-Columbia School of Medicine; Medical Director of St Mary's Stroke Program, SSM Neurosciences Institute, SSM Health Niranjan N Singh, MBBS, MD, DM, FAHS, FAANEM is a member of the following medical societies: American Academy of Neurology , American Association of Neuromuscular and Electrodiagnostic Medicine , American Headache Society Disclosure: Nothing to disclose.
Christopher Luzzio, MD Clinical Assistant Professor, Department of Neurology, University of Wisconsin at Madison School of Medicine and Public Health Christopher Luzzio, MD is a member of the following medical societies: American Academy of Neurology Disclosure: Nothing to disclose.
What would you like to print?
- Print this section
- Print the entire contents of
- Print the entire contents of article
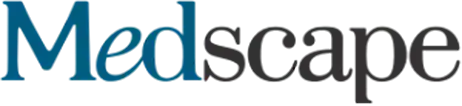
- Alzheimer Disease
- Alzheimer Disease Imaging
- Alzheimer Disease and APOE e4
- Alzheimer Disease in Down Syndrome
- Dementia Pathology
- Emerging Therapies for Alzheimer's Disease
- Early Diagnosis and Treatment in Alzheimer's Disease
- Position Statement Addresses Thyroid Disease and Menopause
- Is Anxiety a Prodromal Feature of Parkinson's Disease?
- Huntington Disease: 5 Things to Know
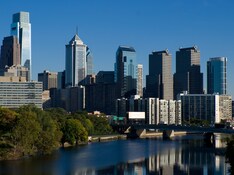
- Drug Interaction Checker
- Pill Identifier
- Calculators
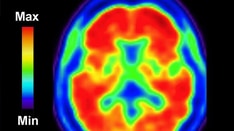
- 2010/recap/1000252 Managing Noncognitive Symptoms of Alzheimer's Disease
Overview - Vascular dementia
Vascular dementia is a common type of dementia caused by reduced blood flow to the brain. It's estimated to affect around 180,000 people in the UK.
Dementia is the name for problems with mental abilities caused by gradual changes and damage in the brain. It's rare in people under 65.
Vascular dementia tends to get worse over time, although it's sometimes possible to slow it down.
Symptoms of vascular dementia
Vascular dementia can start suddenly or begin slowly over time.
Symptoms include:
- slowness of thought
- difficulty with planning and understanding
- problems with concentration
- changes to your mood, personality or behaviour
- feeling disoriented and confused
- difficulty walking and keeping balance
- symptoms of Alzheimer's disease , such as problems with memory and language (many people with vascular dementia also have Alzheimer's disease)
These problems can make daily activities increasingly difficult and someone with the condition may eventually be unable to look after themselves.
Getting medical advice
See a GP if you think you have early symptoms of dementia, especially if you're over 65 years of age.
If it's found at an early stage, treatment may be able to stop vascular dementia getting worse, or at least slow it down.
If you're worried about someone else, encourage them to make an appointment with a GP and perhaps suggest that you go with them.
Your GP can do some simple checks to try to find the cause of your symptoms. They can refer you to a memory clinic or another specialist for further tests if needed.
Find out more about how to get a dementia diagnosis .
Tests for vascular dementia
There's no single test for vascular dementia.
The tests that are needed to make a diagnosis include:
- an assessment of symptoms – for example, whether these are typical symptoms of vascular dementia
- a full medical history, including asking about a history of conditions related to vascular dementia, such as strokes or high blood pressure
- an assessment of mental abilities – this will usually involve several tasks and questions
- a brain scan, such as an MRI scan or CT scan , to look for any changes that have happened in your brain
Find out more about the tests used to diagnose dementia .
Treatments for vascular dementia
There's currently no cure for vascular dementia and there's no way to reverse any loss of brain cells that happened before the condition was diagnosed.
But treatment can sometimes help slow down vascular dementia.
Treatment aims to tackle the underlying cause, which may reduce the speed at which brain cells are lost.
This will often involve:
- eating a healthy, balanced diet
- losing weight if you're overweight
- stopping smoking
- getting fit
- cutting down on alcohol
- taking medicines, such as those used to treat high blood pressure , lower cholesterol or prevent blood clots
Other treatments, including physiotherapy , occupational therapy , dementia activities (such as memory cafes) and psychological therapies , can help reduce the impact of any existing problems.
Outlook for vascular dementia
Vascular dementia will usually get worse over time. This can happen in sudden steps, with periods in between where the symptoms do not change much, but it's difficult to predict when this will happen.
Home-based help will usually be needed, and some people will eventually need care in a nursing home .
Although treatment can help, vascular dementia can significantly shorten life expectancy.
But this is highly variable, and many people live for several years with the condition, or die from some other cause.
If you or a loved one has been diagnosed with dementia, remember that you're not alone. The NHS and social services , as well as voluntary organisations, can provide advice and support for you and your family.
Causes of vascular dementia
Vascular dementia is caused by reduced blood flow to the brain, which damages and eventually kills brain cells.
This can happen as a result of:
- narrowing and blockage of the small blood vessels inside the brain
- a single stroke , where the blood supply to part of the brain is suddenly cut off
- lots of "mini strokes" (also called transient ischaemic attacks, or TIAs) that cause tiny but widespread damage to the brain
In many cases, these problems are linked to underlying conditions, such as high blood pressure and diabetes , and lifestyle factors, such as smoking and being overweight.
Tackling these might reduce your risk of vascular dementia in later life, although it's not yet clear exactly how much your risk of dementia can be reduced.
More information
Living with dementia.
- Living well with dementia
- Staying independent with dementia
- Dementia activities
- Looking after someone with dementia
- Dementia and your relationships
- Communicating with someone with dementia
- Coping with dementia behaviour changes
Care and support
- Sources of help and support
- Dementia UK: nurse helpline
- Dementia and care homes
- Dementia, social services and the NHS
- Dementia and managing money
- Managing legal affairs for someone with dementia
- End of life planning
How you can help
- Dementia Friends
- Alzheimer's Society: Dementia forum
Social care and support guide
- need help with day-to-day living because of illness or disability
- care for someone regularly because they're ill, elderly or disabled, including family members
Our guide to care and support explains your options and where you can get support.
Page last reviewed: 08 June 2023 Next review due: 08 June 2026
Help us improve our website
Can you answer a 5 minute survey about your visit today?

An official website of the United States government
Here's how you know
The .gov means it’s official. Federal government websites often end in .gov or .mil. Before sharing sensitive information, make sure you’re on a federal government site.
The site is secure. The https:// ensures that you are connecting to the official website and that any information you provide is encrypted and transmitted securely.
Vascular dementia
Vascular dementia: causes, symptoms, and treatments.

nia.nih.gov
An official website of the National Institutes of Health
- Type 2 Diabetes
- Heart Disease
- Digestive Health
- Multiple Sclerosis
- Diet & Nutrition
- Supplements
- Health Insurance
- Public Health
- Patient Rights
- Caregivers & Loved Ones
- End of Life Concerns
- Health News
- Thyroid Test Analyzer
- Doctor Discussion Guides
- Hemoglobin A1c Test Analyzer
- Lipid Test Analyzer
- Complete Blood Count (CBC) Analyzer
- What to Buy
- Editorial Process
- Meet Our Medical Expert Board
What's the Difference Between Alzheimer's and Vascular Dementia?
Risk factors, disease progression.
Alzheimer’s disease and vascular dementia (sometimes called vascular cognitive impairment or vascular neurocognitive disorder) are both types of dementia . They have several symptoms and characteristics that overlap, but there are also some clear differences between the two.
If you or a loved one is diagnosed with either condition, this broad overview of the main differences between the two can help you get a grasp on the differences. Keep reading for more detailed information.
Vascular dementia : Statistics vary widely as to the prevalence of vascular dementia, but it’s estimated it accounts for close to 10% of dementia cases. Among older people with dementia, about 50% have signs of vascular dementia.
Alzheimer’s : Alzheimer's disease is by far the most common kind of dementia. There are more than 5 million Americans with Alzheimer’s disease.
Vascular dementia : Vascular dementia is often caused by an acute, specific event such as a stroke or transient ischemic attack in which blood flow to the brain has been interrupted. It also can develop more gradually over time from very small blockages or the slowing of blood flow.
Alzheimer’s : While there are several ways to decrease the chance of developing Alzheimer’s, including exercise and maintaining an active mind , what causes Alzheimer’s to develop isn't fully understood. There appear to be many contributing components, such as genetics, lifestyle, and other environmental factors.
Vascular dementia : Common risk factors include coronary heart disease , peripheral artery disease , diabetes mellitus , high blood pressure , and high cholesterol .
Alzheimer’s : Risk factors include age, genetics (heredity), and general health.
The two conditions have some differences in cognitive symptoms and physical symptoms.
Vascular dementia : Cognitive abilities often seem to decline suddenly in relation to an event like a stroke or a transient ischemic attack (TIA) and then remain stable for a time. These changes are often described as step-like since in between them, brain functioning may hold steady.
Alzheimer’s : While cognition can vary somewhat in Alzheimer’s, the person’s ability to think and use his memory gradually declines over time. There is not usually a sudden, significant change from one day to the next.
In contrast to the step-like decline in vascular dementia, Alzheimer's is typically more like a slight, downward slope of a road over time.
Walking and Physical Movement
Vascular dementia : Vascular dementia is often accompanied by some physical challenge. If a person has a stroke, they may have limited movement on one side of her body. Both the cognitive and physical impairments related to vascular dementia usually develop at the same time since they are often the result of a sudden condition like a stroke.
Alzheimer’s: Often, mental abilities like memory or judgment decline initially, and then as Alzheimer's progresses into the middle stages , physical abilities like balance or walking show some deterioration.
Vascular dementia: Several tests can help evaluate your loved one's memory , judgment, communication and general cognitive ability. Along with those tests, an MRI can often clearly identify a specific area in the brain where a stroke affected the brain.
Alzheimer’s : Similar cognitive tests are used to evaluate brain functioning, but Alzheimer's is often diagnosed by ruling out other causes, rather than being able to pinpoint the diagnosis through a brain scan.
There's no test to diagnose Alzheimer's at this time, so physicians generally eliminate other reversible causes of confusion such as vitamin B12 deficiency and normal pressure hydrocephalus , as well as other types of dementia or delirium .
Vascular dementia : Since there is such a variety of causes and different amounts of damage, it's difficult to predict survival time for vascular dementia. Progression of vascular dementia depends on a number of factors including the extent of the damage in the brain, in addition to your overall health condition.
Alzheimer’s : Indivduals with Alzheimer's disease have a median age at death of 84.6 years old. The survival after the beginning of symptoms is an average of 8.4 years.
A Word From Verywell
Learning the differences between vascular dementia and Alzheimer's disease can help you better understand what to expect from a diagnosis.
Additionally, while there are clear differences between the two diseases, research has found that some similar strategies can be used to reduce their risk. These include a heart-healthy diet and physical activity .
Alzheimer's Association. 2014 Alzheimer's disease facts and figures. Alzheimers Dement . 2014;10(2):e47-92. doi:10.1016/j.jalz.2014.02.001
National Institute on Aging. Alzheimer’s disease fact sheet . 2019.
Smith EE. Clinical presentations and epidemiology of vascular dementia. Clin Sci . 2017;131(11):1059-1068. doi:10.1042/CS20160607
Saito S, Yamamoto Y, Ihara M. Development of a multicomponent intervention to prevent Alzheimer's disease. Front Neurol. 2019;10:490. doi:10.3389/fneur.2019.00490
Appleton JP, Scutt P, Sprigg N, Bath PM. Hypercholesterolaemia and vascular dementia. Clin Sci . 2017;131(14):1561-1578. doi:10.1042/CS20160382
Mayeux R, Stern Y. Epidemiology of Alzheimer disease. Cold Spring Harb Perspect Med . 2012;2(8). doi:10.1101/cshperspect.a006239
Jahn H. Memory loss in Alzheimer's disease. Dialogues Clin Neurosci. 2013;15(4):445-54.
Tolea MI, Morris JC, Galvin JE. Trajectory of mobility decline by type of dementia. Alzheimer Dis Assoc Disord . 2016;30(1):60-6. doi:10.1097/WAD.0000000000000091
Barnes J, Dickerson BC, Frost C, Jiskoot LC, Wolk D, Van der flier WM. Alzheimer's disease first symptoms are age dependent: Evidence from the NACC dataset. Alzheimers Dement . 2015;11(11):1349-57. doi:10.1016/j.jalz.2014.12.007
The appropriate use of neuroimaging in the diagnostic work-up of dementia: an evidence-based analysis. Ont Health Technol Assess Ser. 2014;14(1):1-64.
Morris MC, Schneider JA, Tangney CC. Thoughts on B-vitamins and dementia. J Alzheimers Dis . 2006;9(4):429-33.
Lee AY. Vascular dementia. Chonnam Med J . 2011;47(2):66-71. doi:10.4068/cmj.2011.47.2.66
Williams MM, Xiong C, Morris JC, Galvin JE. Survival and mortality differences between dementia with Lewy bodies vs Alzheimer disease. Neurology . 2006;67(11):1935-41. doi:10.1212/01.wnl.0000247041.63081.98
Alzheimer's Association. Risk factors.
Alzheimer’s Association. Vascular dementia .
Ozbabalık, D, Arslantaş, A and Elmacı, NT. The Epidemiology of Vascular Dementia . 2012. ISBN: 978-953-51-0080-5, InTech.
O’Brien, J.T., Markus, H.S. Vascular risk factors and Alzheimer’s disease . BMC Med. 2014;12, 218. doi:10.1186/s12916-014-0218-y
By Esther Heerema, MSW Esther Heerema, MSW, shares practical tips gained from working with hundreds of people whose lives are touched by Alzheimer's disease and other kinds of dementia.
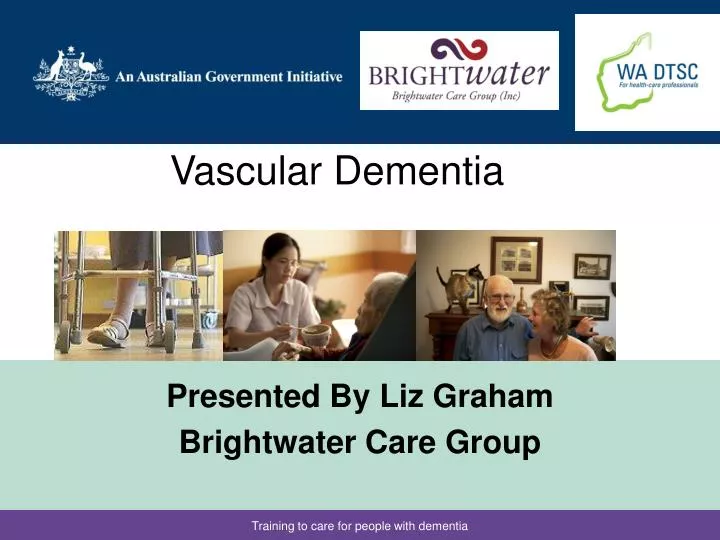
Vascular Dementia
Aug 10, 2014
470 likes | 1.92k Views
Vascular Dementia. Presented By Liz Graham Brightwater Care Group. Objectives. To increase knowledge and understanding of Vascular Dementia To demonstrate the progression of Vascular Dementia To discuss the importance of team management of the person with Vascular Dementia.
Share Presentation
- entertainment tia non
- dementia training
- jim family rn speech
- vascular dementia eg aricept
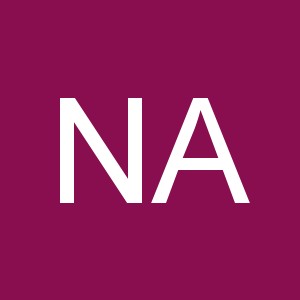
Presentation Transcript
Vascular Dementia Presented By Liz Graham Brightwater Care Group Training to care for people with dementia
Objectives • To increase knowledge and understanding of Vascular Dementia • To demonstrate the progression of Vascular Dementia • To discuss the importance of team management of the person with Vascular Dementia Training to care for people with dementia
Types of Dementia • Creutzfelt-Jacob Disease (CJD) • Progressive Supranuclear Palsy • Downs Syndrome • AIDS related • Encephalitis • Head Injury • And Others (over 80 causes) • Alzheimer’s Disease • Vascular Dementia • Dementia with Lewy bodies • Frontal lobe Dementia • Korsakoff’s Syndrome • Huntington’s Disease • Substance abuse Training to care for people with dementia
What is Vascular Dementia? • Second most common form of dementia after Alzheimer’s disease. • Occurs when the blood supply to the brain is interrupted by a blocked or diseased vascular system. Training to care for people with dementia
A Little Bit of History • Arteriosclerosis and senile dementia described as different syndromes as early as 1899. • Mayer-Gross et al in 1969 reported hypertension to be the cause in 50% of patients. • Hachinski in 1974 used the term multi-infarct dementia. • In 1985 the term vascular dementia was used by Loeb. Training to care for people with dementia
Different Types 0f Vascular Dementia • Mild vascular cognitive impairment • Multi-infarct dementia • Vascular dementia due to a strategic single infarct. • Vascular dementia due to lacunar lesions • Vascular dementia due to haemorrhagic lesions • Binswanger disease • Subcortical vascular dementia • Mixed dementia (combination of AD and Vascular) Article by Kannayiram Alagiakrishnam Training to care for people with dementia
Multi-infarct dementia • Most common form • Caused by a series of small strokes or TIA. • Damage caused to the cortex of the brain • Area associated with learning, memory and language. • Can be temporary but over time with repeated incidents become permanent • Symptoms include severe depression, mood swings and epilepsy Training to care for people with dementia
Binswanger’s Disease(or Subcortical vascular dementia) • Associated with stroke-related changes. • Damage to tiny blood vessels of the white matter, deep within the brain. • Symptoms develop more gradually and include • Slowness, lethargy, difficulty walking, emotional ups and downs, lack of bladder control. Training to care for people with dementia
Physical signs/symptoms Memory problems, forgetfulness Dizziness Leg or arm weakness Lack of concentration Moving with rapid, shuffling steps Loss of bladder or bowel control Signs and Symptoms of Vascular Dementia Training to care for people with dementia
Behavioural signs/symptoms • Depression • Slurred speech • Language problems • Abnormal behaviour • Wandering/getting lost • Laughing/crying inappropriately • Difficulty following instructions • Problems handling money Training to care for people with dementia
Risk Factors that increase risk of developing Vascular Dementia Training to care for people with dementia
Risk Factors • High blood pressure • Smoking • Diabetes • High Cholesterol • History of mild warning strokes • Evidence of arterial disease elsewhere • Heart rhythm abnormalities • Lack of physical activity • Fatty diet • Gender – Men are at a slightly higher risk. • Age – Usually between 60 and 75, incidence increases with age. Training to care for people with dementia
GOOD NEWSUnlike Alzheimer’s Disease, there are ways to prevent and reduce the severity of vascular dementia. Training to care for people with dementia
Medical management of: • High blood pressure • High Cholesterol • Heart disease • Diabetes • Administering medication to prevent clots forming eg Aspirin • Drugs to relieve restlessness or depression • Recent research indicates that cholinesterase inhibitor medications which are helpful with Alzheimer’s disease may be of benefit in Vascular Dementia eg Aricept, Reminyl. • In some cases surgery such as carotid endarterectomy may be indicated. Training to care for people with dementia
Receiving Rehabilitative Support: Physiotherapy Occupational Therapy Speech Therapy To help the person regain lost functions. Unlike Alzheimer’s Disease, there are ways to prevent and reduce the severity of vascular dementia. • A Healthier Lifestyle: • A healthy diet • Regular Exercise • Stop smoking • Moderate intake of alcohol Training to care for people with dementia
Progression of Vascular Dementia Onset gradual or dramatic Stabilise TIA/Stroke Stabilise TIA/Stroke Typically Vascular Dementia progresses gradually in a stepwise fashion in which a person’s abilities deteriorate after a stroke, then stabilise until the next stroke. Training to care for people with dementia
Mortality/Morbidity • In community-based studies in Australia, the prevalence rate for vascular and mixed dementia is 13% and 28%, respectively. • In patients with dementia who have had a stroke, the increase in mortality is significant. The 5 year survival rate is 39% for patients with vascular dementia compares with 75% age matched controls. • Vascular dementia is associated with higher mortality rate than AD, presumably because of the coexistence of other atherosclerotic diseases. (Alagiakrishnan) Training to care for people with dementia
Case Study • 74 year old male admitted to High Care from CAP unit • Diagnosis – Dementia • History of Hypertension, TIAs. • Supportive family, wife and 2 daughters • Transfer information - Communication poor. - Mobility: Non-ambulant, hoist transfer. • ADLs: Full assistance, resistive in showering/dressing. • Doubly incontinent. - Full assistance with meals. Training to care for people with dementia
Personhood • “A standing or status that is bestowed upon one human being, by others, in the context of relationship and social being. It implies recognition, respect and trust” • (Kitwood 1997) Training to care for people with dementia
Philosophy and Principles of Person Centred Care • Attend to the whole person • See each person as unique and special • Give respect to the past • Focus on the positives • Stay in communication • Nourish attachments • Create community • Maximise freedom – minimise controls • Maintain environment of trust and value Training to care for people with dementia
Team assessment and planning of care GP OCCUPATIONAL THERAPIST CARER JIM FAMILY RN SPEECH PATHOLOGIST PHYSIOTHERAPIST Training to care for people with dementia
From Assessment • GP – Reviews and monitors medication for hypertension and administers aspirin. • RN – Monitors health status and specifically blood pressure. Works with team to implement care strategies. • Occupational Therapist – Establishes grabbing and gripping is behavioural. Implements strategies to assist during washing/dressing including good communication, time, use of smiling, careful handling. • Carer– Works with Jim implementing strategies and feeding back responses. • Family– Guided by all staff in understanding and supporting Jim’s needs. Involved during mealtimes, activities to support Jim. Training to care for people with dementia
From assessment Contd. • Physio – Works to promote effective transfers and walking with assistance of 2, 20 metres. Works with OT to ensure active upper limb range present for washing /dressing. Works with team to implement care strategies. • Speech Pathologist – Establishes dysphagia status and assistance for meals (along with OT). Soft diet, promoting assistance to initiate ability to feed himself especially with finger foods. Communication requires one stage commands, time to respond and friendly smiling face. Works with team to implement care strategies Training to care for people with dementia
Example of Jim’s Progress Walks 20m with 2, Feeds himself with help, Strategies assist during washing. Gross motor activity, Entertainment TIA Non-Ambulant, Smiles, Whistles, Resistive, Assisted with meals Stand transfers, non-ambulant, Distracter to hold during washing Smiles, Whistles, Gross motor TIA Standing Hoist transfers, Active-Assisted exercises. Assistance with vitamised meals. Continue Distractor use. Smiles but no whistling. Assess Implement Evaluate Review Training to care for people with dementia
Take Home Message The take home message is simple: By reducing your risk for stroke, you also reduce your risk for vascular dementia. Training to care for people with dementia
References • www.brad.ac.uk/acad/health/dementia • www.helpguide.org/elder/vascular_dementia.htm • www.alzheimers.org.uk • www.nia.nih.gov/Alzheimers/Publication/dementia.htm • www.memorylossonline.com/pastissues/summer2000/ vasculardementia.html • www.alzheimers.org.au • www.emedicine.com/MED/topic3150.htm Training to care for people with dementia
- More by User
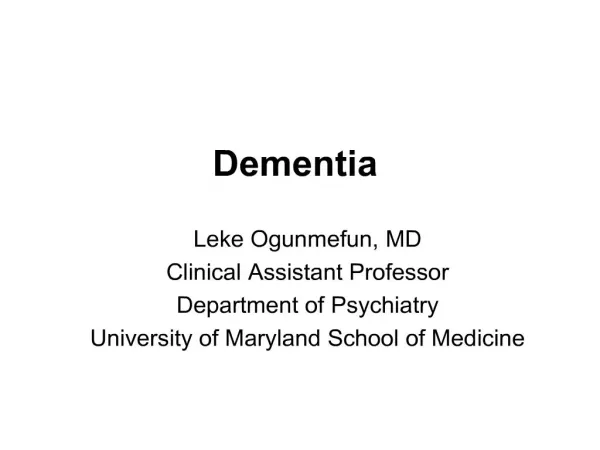
Definitions. Dementia is used as an umbrella term to group all diseases in which there is some form of memory loss.Symptoms of dementia emerge slowly, worsen over time and restrict your ability to function.Because depression can sometimes affect memory and cognition, it is often difficult to clearly differentiate it from dementia..
1.04k views • 25 slides
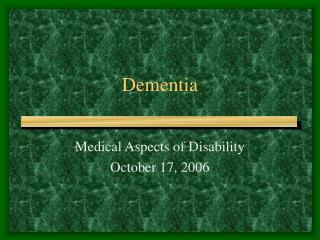
Dementia. Medical Aspects of Disability October 17, 2006. DEMENTIA. DEFINITION: Group of symptoms that can be caused by over 60-70 disorders.
901 views • 46 slides
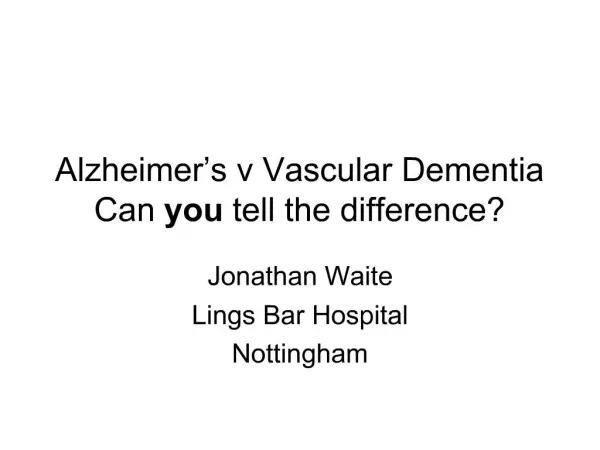
Alzheimer s v Vascular Dementia Can you tell the difference
Alzheimer's v Vascular. PlaquesTanglesInsidious onsetProgressive deterioration. Infarction Sudden onsetStepwise deterioration Focal signs and symptomsPathological emotionalism. Hachinski Score. Abrupt onset 2Stepwise deterioration1Fluctuating course2Nocturnal confusion 1Personality preservation1Depression1Somatic complaints1 .
542 views • 19 slides
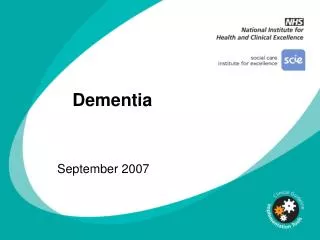
Dementia. September 2007 . This presentation covers:. Background Key recommendations Interventions Implementation. National Institute for Health and Clinical Excellence.
994 views • 39 slides
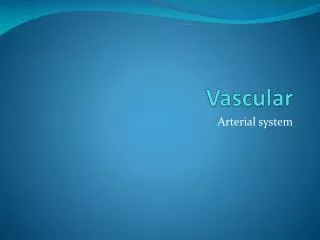
Vascular. Arterial system. Aneurysmal Vascular Disease. Defined as a permanent localized enlargement of an artery to more than 1.5 times its expected diameter .
999 views • 51 slides
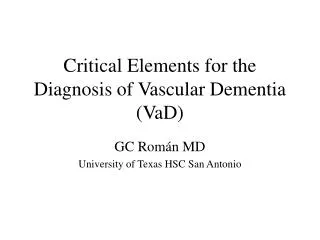
Critical Elements for the Diagnosis of Vascular Dementia (VaD)
Critical Elements for the Diagnosis of Vascular Dementia (VaD). GC Román MD University of Texas HSC San Antonio. Research Definition of VaD. A) Dementia B) Cerebrovascular disease C) A and B must be reasonably related.
426 views • 10 slides
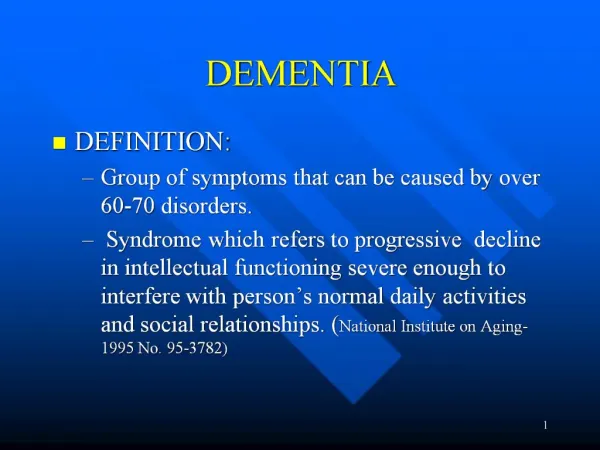
12/10/2012. 2. Dementia. Marked by progressive, irreversible declines in memory. visual-spatial relationshipsperformance of routine tasks language and communication skillsabstract thinkingability to learn and carry out mathematical calculations.. 12/10/2012. 3. Dementia. Two Types:Reversible
698 views • 22 slides
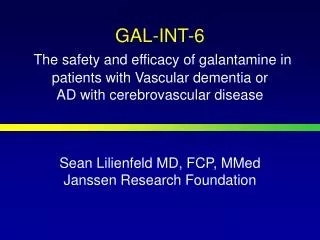
Interactions between vascular dementia and Alzheimer’s disease
GAL-INT-6 The safety and efficacy of galantamine in patients with Vascular dementia or AD with cerebrovascular disease Sean Lilienfeld MD, FCP, MMed Janssen Research Foundation. Interactions between vascular dementia and Alzheimer’s disease. VaD. AD. Mixed. Erkinjuntti T.
510 views • 21 slides
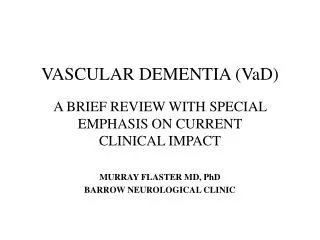
VASCULAR DEMENTIA (VaD)
VASCULAR DEMENTIA (VaD). A BRIEF REVIEW WITH SPECIAL EMPHASIS ON CURRENT CLINICAL IMPACT MURRAY FLASTER MD, PhD BARROW NEUROLOGICAL CLINIC. OVERVIEW. HISTORICAL PERSPECTIVE PATHOPHYSIOLOGIC BASIS CLINICAL IMPACT. OTTO BINSWANGER 1894 ALOIS ALZHEIMER 1895, 1907 PIERRE MARIE 1901
464 views • 17 slides
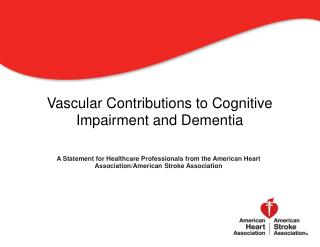
Vascular Contributions to Cognitive Impairment and Dementia
Vascular Contributions to Cognitive Impairment and Dementia. A Statement for Healthcare Professionals from the American Heart Association/American Stroke Association. Statement Authors.
1.55k views • 107 slides
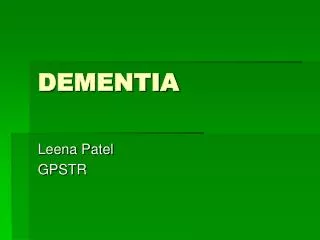
DEMENTIA. Leena Patel GPSTR. Overview. Causes of dementia Differential diagnosis Dementia and QOF. Degenerative diseases. Alzheimer’s Lewy body dementia Vascular Mixed (e.g. Alzheimer's & vascular) Parkinson’s disease Huntington’s chorea CJD Picks disease.
720 views • 12 slides
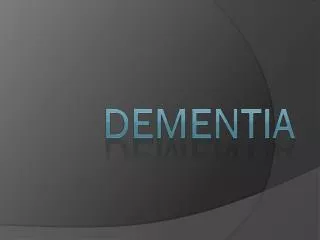
Dementia. Dementia is a progressive impairment of cognitive functions occurring in clear consciousness. The major defect in dementia is Memory loss (antrograde or retrograde).
818 views • 27 slides
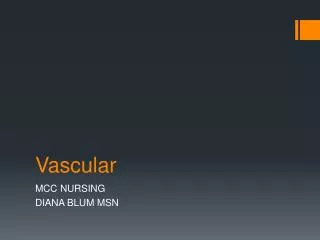
Vascular . MCC NURSING DIANA BLUM MSN. hormones. C reactive protein is a marker for cardiac inflammation Increases mean: risk of damage
749 views • 56 slides
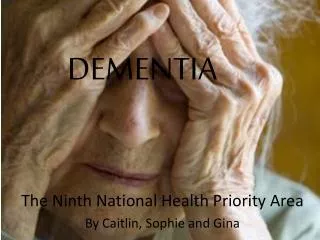
DEMENTIA. The Ninth National Health Priority Area By Caitlin, Sophie and Gina. What is it?. What is it?. Most recent addition to the NHPA inititiative being included in 2012
465 views • 20 slides
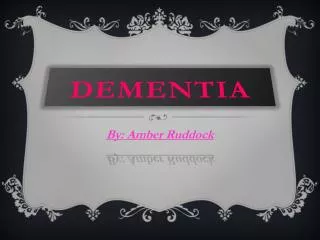
DEMENTIA. By: Amber Ruddock. WHAT IS IT?. Dementia- is a loss of brain function that occurs with certain diseases. Types of dementia. Cortial Dementia- memory, thinking, behavior (brain’s cortex) Subcortial Dementia - changes in emotions & movements (below the cortex of brain)
547 views • 8 slides
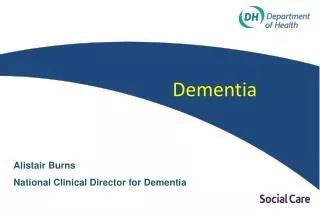
Dementia . Alistair Burns National Clinical Director for Dementia. Dementia dementia.dh.gov.uk. Early diagnosis and intervention in primary care Dementia in the General Hospital Dementia in Care homes Reduction of antipsychotics Support for carers Ten outcomes statements.
678 views • 14 slides
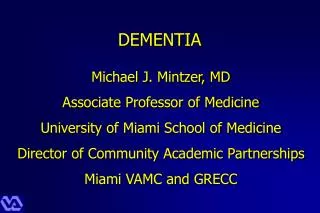
DEMENTIA. Michael J. Mintzer, MD Associate Professor of Medicine University of Miami School of Medicine Director of Community Academic Partnerships Miami VAMC and GRECC. Dementia. Learning Objectives: Define dementia Describe the common dementias in the elderly
545 views • 21 slides
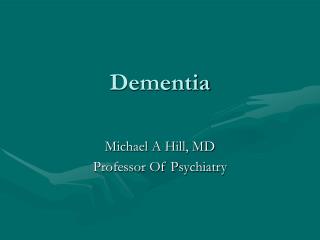
Dementia. Michael A Hill, MD Professor Of Psychiatry. Dementia An acquired syndrome characterized by:. Short-term memory impairment (i.e. learning) AND At least one of the following: Aphasia - language memory impairments Apraxia - motor memory impairments
1.64k views • 70 slides
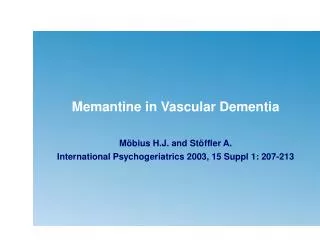
Memantine in Vascular Dementia: Subgroup Analyses
Memantine in Vascular Dementia Möbius H.J. and Stöffler A. International Psychogeriatrics 2003, 15 Suppl 1: 207-213. Memantine in Vascular Dementia: Subgroup Analyses. Analyses based on studies in patients with mild to moderate vascular dementia: Orgogozo et al. (2002) (MMM300)
256 views • 6 slides
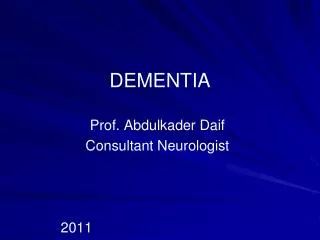
DEMENTIA. Prof. Abdulkader Daif Consultant Neurologist 2011. Cognitive Disorders (DSM-IV). 1. Dementia 2. Delirium 3. Amnesic Disorder. Cognition. Brain functions including: Attention Initiation Memory Language Calculation Praxis Executive functions
1.2k views • 57 slides
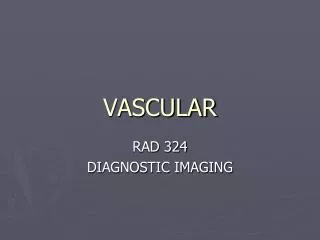
VASCULAR. RAD 324 DIAGNOSTIC IMAGING. Vascular Radiography. Case Number 1422. A. A. C. F. Vascular Radiography. Case Number 4412. 7. 5. 8. 9. 6. 4. 3. 2. 1. 10. Vascular Radiography. Case Number 6204. E. F 2. 5. F 1. Vascular Radiography. Case Number 6208.
619 views • 39 slides
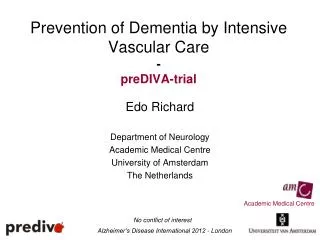
Prevention of Dementia by Intensive Vascular Care - preDIVA-trial
Prevention of Dementia by Intensive Vascular Care - preDIVA-trial. Edo Richard Department of Neurology Academic Medical Centre University of Amsterdam The Netherlands. Academic Medical Centre. No conflict of interest. Alzheimer’s Disease International 2012 - London. Epidemiology.
223 views • 11 slides
- Research article
- Open access
- Published: 06 August 2024
Network proteomics of the Lewy body dementia brain reveals presynaptic signatures distinct from Alzheimer’s disease
- Anantharaman Shantaraman 1 , 2 ,
- Eric B. Dammer 1 , 2 ,
- Obiadada Ugochukwu 1 ,
- Duc M. Duong 1 , 2 ,
- Luming Yin 2 ,
- E. Kathleen Carter 1 , 2 , 3 ,
- Marla Gearing 1 , 3 , 4 ,
- Alice Chen-Plotkin 5 ,
- Edward B. Lee 6 ,
- John Q. Trojanowski 6 ,
- David A. Bennett 7 ,
- James J. Lah 1 , 3 ,
- Allan I. Levey 1 , 3 ,
- Nicholas T. Seyfried 1 , 2 , 3 &
- Lenora Higginbotham ORCID: orcid.org/0000-0002-8694-9211 1 , 3
Molecular Neurodegeneration volume 19 , Article number: 60 ( 2024 ) Cite this article
427 Accesses
15 Altmetric
Metrics details
Lewy body dementia (LBD), a class of disorders comprising Parkinson’s disease dementia (PDD) and dementia with Lewy bodies (DLB), features substantial clinical and pathological overlap with Alzheimer’s disease (AD). The identification of biomarkers unique to LBD pathophysiology could meaningfully advance its diagnosis, monitoring, and treatment. Using quantitative mass spectrometry (MS), we measured over 9,000 proteins across 138 dorsolateral prefrontal cortex (DLPFC) tissues from a University of Pennsylvania autopsy collection comprising control, Parkinson’s disease (PD), PDD, and DLB diagnoses. We then analyzed co-expression network protein alterations in those with LBD, validated these disease signatures in two independent LBD datasets, and compared these findings to those observed in network analyses of AD cases. The LBD network revealed numerous groups or “modules” of co-expressed proteins significantly altered in PDD and DLB, representing synaptic, metabolic, and inflammatory pathophysiology. A comparison of validated LBD signatures to those of AD identified distinct differences between the two diseases. Notably, synuclein-associated presynaptic modules were elevated in LBD but decreased in AD relative to controls. We also found that glial-associated matrisome signatures consistently elevated in AD were more variably altered in LBD, ultimately stratifying those LBD cases with low versus high burdens of concurrent beta-amyloid deposition. In conclusion, unbiased network proteomic analysis revealed diverse pathophysiological changes in the LBD frontal cortex distinct from alterations in AD. These results highlight the LBD brain network proteome as a promising source of biomarkers that could enhance clinical recognition and management.
Introduction
Lewy body dementia (LBD), a class of disorders comprising Parkinson’s disease dementia (PDD) and dementia with Lewy bodies (DLB), is the second most common cause of dementia worldwide and is currently without cure or effective mitigating therapies [ 1 ]. The identification of reliable biomarkers of its aggressive cognitive and neuropsychiatric symptoms is critical to advancing the clinical management of LBD. Yet, LBD biomarker discovery has proven challenging, in large part due to the complexity and often overlapping pathophysiology driving its dementia, psychosis, and mood disturbances. Pathological evidence has linked the cognitive and neuropsychiatric manifestations of LBD to the diffuse deposition of α-synuclein-rich Lewy bodies (LBs) throughout the limbic and neocortex [ 2 , 3 , 4 , 5 , 6 ]. However, a growing number of studies across multiple disciplines, including genetic, clinicopathological, imaging, and biofluid analyses, suggests LBD pathophysiology encompasses a diverse array of corticolimbic processes extending beyond synuclein accumulation and neuronal loss. Among these implicated mechanisms are mitochondrial dysfunction, neuroinflammation, aberrant cholinergic and other neurotransmitter activity, and synaptic dysregulation [ 1 , 7 , 8 , 9 ]. This increasingly complex pathophysiological landscape indicates multiple molecular signatures may be necessary for effective LBD biomarker development.
The genetic and molecular overlap LBD shares with Alzheimer’s disease (AD) and other neurodegenerative disorders further complicates the ability to unravel its key pathophysiological signatures. Genome-wide association studies (GWAS) have identified a significant amount of polygenic overlap between LBD and AD [ 7 ]. Meanwhile, according to certain clinicopathological estimates, over 50% of those with LBD feature concurrent accumulation of the extracellular amyloid-beta (Aβ) plaques and tau neurofibrillary tangles (NFTs) that comprise the core of AD neuropathology [ 10 ]. Furthermore, limbic-predominant TAR DNA-binding protein 43 (TDP-43) and other pathological inclusions are frequently detected in both LBD and AD [ 11 ]. This overlapping pathology has a marked impact on diagnostic accuracy, clinical trial stratification, disease prognosis, and therapeutic development [ 10 , 11 , 12 , 13 ]. Thus, research strategies designed to better define not only unique signatures of LBD pathophysiology but also its degree of overlap with other neurodegenerative diseases are critical to the discovery of biomarkers that meaningfully advance its diagnosis, monitoring, and treatment.
Network-based proteomics, which quantifies global pathophysiological changes in complex biological samples [ 14 ], is a tool designed to address many of these challenges. This data-driven approach organizes large proteomic datasets into groups or “modules” of proteins with similar expression patterns across individual samples. These co-expression modules are often enriched with markers specific to certain cell types, molecular functions, and organelles, providing insights into the diverse pathophysiological alterations reflected in the disease specimen. We have previously used this approach to define and characterize the network of complex protein pathophysiology within the brain tissues of those with pathologically defined AD [ 15 , 16 , 17 , 18 , 19 , 20 , 21 , 22 ]. These AD-associated modules and their hub proteins have proven highly reproducible across different tissue cohorts and brain regions, allowing us to generate a large consensus AD brain network across hundreds of corticolimbic tissues [ 17 ]. In sum, our consensus findings have 1) enhanced understanding of neuronal and non-neuronal pathophysiology in the AD brain; 2) provided a strong molecular framework for network-level comparison to other neurodegenerative diseases, and 3) served as a strong foundation for panel-based biomarker discovery in cerebrospinal fluid and plasma [ 23 , 24 ]. Furthermore, these proteomic networks have revealed significant disease-related alterations not reflected at the transcriptomic level [ 15 , 16 , 17 , 20 ].
In the current study, we apply an unbiased co-expression network proteomic approach to the study of corticolimbic alterations in the brains of those with pathologically defined LBD, establishing a global systems-based framework of the protein-level changes underlying neurodegeneration in these tissues. In addition, we perform a network-level comparison of the LBD and AD brain proteomes. Our results reveal protein co-expression alterations throughout a diverse range of pathophysiological systems in the LBD brain, including presynaptic signatures distinct from those observed in the AD proteomic network. We also demonstrate how α-synuclein (SNCA) plays a critical “bottleneck” role in the network-level communication among these synaptic signatures. Finally, we underscore the utility of proteomic network analysis in examining not only divergent changes but also overlapping features of LBD and AD, highlighting signatures capable of stratifying LBD cases with low versus high burdens of amyloid co-pathology. Overall, this approach offers a systems-based foundation for the discovery of protein biomarkers that reflect the unique and complex pathophysiology of LBD.
Differential expression analysis demonstrates robust protein alterations in the LBD brain
The main objective of this study was to perform unbiased co-expression network analysis of LBD brain tissues to better define global pathophysiological alterations in cortical regions and compare these findings to the AD brain network proteome. We began our analyses with brain tissues derived from a pathologically well-characterized autopsy collection within the University of Pennsylvania (UPenn) Alzheimer’s Disease Research Center (ADRC). We examined the dorsolateral prefrontal cortex (DLPFC) as it is often affected in the diffuse corticolimbic LB accumulation found in LBD and is routinely scored in its neuropathological diagnosis [ 25 ]. In addition, frontal executive deficits are commonly among the first symptoms observed in LBD [ 26 ], suggesting this region is at the forefront of LB-mediated cognitive changes. We analyzed a total of 354 UPenn DLPFC tissues of various neurological diagnoses by tandem mass tag mass spectrometry (TMT-MS) but focused our analyses on those with LB disease for this study (Fig. 1 A). MS analysis of the total cohort quantified 9,661 proteins, each present in at least 50% of samples. Technical variance was minimized using a tunable median polish approach (TAMPOR) (Fig. S1A) [ 27 ]. To prioritize alterations driven by disease, the data was then regressed for variability due to age, sex, and post-mortem interval (PMI) (Fig. S1B).

Differential expression and network analysis of UPenn LBD tissues. A Study approach for analyzing differential expression and co-expression across UPenn LB DLPFC tissues, including control ( n = 47), PD ( n = 33), PDD ( n = 47), and DLB ( n = 11) cases. B Volcano plots displaying the log 2 fold change (x-axis) against the -log 10 statistical p value (y-axis) for proteins differentially expressed between pairwise comparisons of each disease to controls. All p values across pairwise comparisons were derived by ANOVA with Tukey post-hoc correction. C Boxplots of MS-measured APP and SNCA levels and their correlations to neuropathology measures of global amyloid plaque (CERAD) and frontal LB deposition, respectively. ANOVA p values are provided for each MS abundance plot (*, p < 0.05; **, p < 0.01; ***, p < 0.001), while the Pearson correlation coefficient with associated p value is provided for each correlation analysis. D Co-expression network generated by WGCNA across control and LB cases, consisting of 33 modules each labeled with a number and color. Module relatedness is shown in the dendrogram. E Neuropathological trait correlations, cell type marker enrichment, and principal gene ontology for each module. Module abundances were correlated to disease diagnosis and measures of pathological burden with positive correlations indicated in red and negative correlations in blue. The cell type nature of each module was assessed by module protein overlap with cell type-specific marker lists of astrocytes, microglia, neurons, oligodendrocytes, and endothelia. Gene ontology analysis was used to identify the primary biology reflected by each module. Asterisks in each heat map indicate the degree of statistical significance of trait correlations and cell type marker enrichment (*, p < 0.05; **, p < 0.01; ***, p < 0.001). Abbreviations: CTL, control; PD, Parkinson’s disease; PDD, Parkinson’s disease dementia; DLB, Dementia with Lewy bodies; DLPFC, dorsolateral prefrontal cortex; TMT-MS, Tandem mass tag mass spectrometry; APP, amyloid precursor protein; SNCA, α-synuclein
Differential expression and network analyses were performed on a subset of these UPenn tissues, including 47 control, 33 Parkinson’s disease (PD), 47 PDD, and 11 DLB cases. The LB cases were slightly older and more male compared to controls (Table S1-S2). All groups featured similar post-mortem intervals. Pathological traits available for these brain tissues included levels of global Aβ neuritic plaque and tau NFT deposition as measured by the Consortium to Establish a Registry for Alzheimer’s Disease (CERAD) criteria and the Braak staging system, respectively [ 28 , 29 ]. Severity levels of LB deposition in the frontal cortex were measured on a semi-quantitative severity scale of 0 to 3. Nearly all 58 dementia cases harbored LBs in the frontal cortex, ranging from mild to severe. Robust amyloid co-pathology was common among the LBD cases. Yet, most harbored only mild to moderate NFT levels (Braak I-IV), increasing the likelihood that their cognitive symptoms were driven by LB deposition as opposed to AD-associated neuropathological change [ 26 ]. Only 7 PDD and 2 DLB cases featured severe NFT levels (Braak V-IV).
Prior to building a co-expression network, we examined the differential expression in each disease compared to controls (Fig. 1 B, Table S3). All three LB disorders featured > 1000 significantly altered proteins ( p > 0.05) compared to controls. Isobaric labeling strategies like TMT-MS tend to compress quantitative data [ 30 ]. Thus, most of these differentially expressed proteins featured log-transformed fold changes (log 2 Δ) between -1 and 1, consistent with the fold-change magnitudes we have previously observed in TMT-MS analyses of the human brain [ 17 ]. Amyloid precursor protein (APP), which has historically correlated strongly to Aβ accumulation in our proteomic datasets [ 20 ], was significantly elevated in both PDD ( p = 0.0038, log 2 Δ = 0.18) and DLB ( p = 4.15E-05, log 2 Δ = 0.39) but largely unaltered in PD ( p = 0.98, log 2 Δ = 0.024), aligning with the moderate to severe CERAD scores of the dementia cases. SNCA was notably increased in all three LB disorders, reaching significance in PD ( p = 0.00021, log 2 Δ = 0.15) and PDD ( p = 1.51E-06, log 2 Δ = 0.17) and approaching significance in DLB ( p = 0.089, log 2 Δ = 0.12). In contrast, microtubule associated protein tau (MAPT) levels were not significantly altered in any of the disease groups compared to controls. APP and SNCA levels correlated significantly with neuropathology measures of neuritic plaque and LB deposition, respectively (Fig. 1 C).
Proteins most highly increased in dementia cases included phospholipid transporter TMEM30A, calcium channel modulator ORAI2, and various muscarinic cholinergic receptors (CHRM1, CHRM3). Meanwhile, both dementia groups featured starkly decreased levels of known neuroprotective markers VGF and NPTX2, which are consistently decreased in the brains of those with neurodegeneration [ 31 , 32 , 33 , 34 , 35 , 36 , 37 , 38 , 39 ]. Neuritin 1 (NRN1), a synaptic protein linked to cognitive resilience in AD, was also significantly decreased in both PDD and DLB [ 40 , 41 ]. Cholinergic disruption was further evidenced by exceptional decreases in the ion channel transporter SLC5A7 across all three diseases, as large as fivefold lower in DLB compared to controls. This protein, also known as CHT1, mediates presynaptic high-affinity choline uptake in cholinergic neurons for acetylcholine (ACh) synthesis [ 42 ], and its dysfunction has been linked to AD in animal models [ 43 , 44 ]. Yet, several of the most decreased LBD markers had less well-described links to neurodegeneration, such as the serotonin synthesizing enzyme TPH2. Overall, these results highlighted significant differential expression across numerous proteins in all three LB diseases, including changes in known markers of neurodegeneration.
Network analysis of the LBD brain reveals alterations across diverse cell types and molecular functions
To examine global systems-based alterations in LBD, we applied Weighted Gene Co-Expression Network Analysis (WGCNA), which organizes complex proteomic datasets into groups or modules (M) of proteins with similar expression patterns across individual cases [ 20 ]. Our resultant LBD co-expression network comprised 8,517 of the total 9,661 quantified proteins. Approximately 12% of the dataset ( n = 1,144 proteins) did not map strongly to a particular module, consistent with the proportions of unassigned proteins we have encountered in previous network analyses [ 15 , 16 , 17 , 18 , 19 , 20 ]. These 8,517 proteins were clustered into 33 UPenn (P) co-expression modules, with P-M1 representing the largest module ( n proteins = 601) and P-M33 the smallest module ( n proteins = 39) (Fig. 1 D, Table S4). The weighted expression profile, or eigenprotein, of each module was correlated to disease diagnosis and various clinicopathological traits (Fig. 1 E, Table S5). In addition, each module was further characterized by cell type enrichment and gene ontology (GO) analyses (Fig. 1 E, Table S6), as previously described [ 16 , 17 , 18 ].
Over two-thirds of the 33 modules correlated significantly to PDD, DLB, or both. These LBD-associated modules reflected a variety of cell type associations, biological ontologies, and cellular compartments. Modules with strongly negative correlations to disease included those linked to mitochondria (P-M1), Golgi transport (P-M15), ribosome biogenesis / function (P-M2, P-M25, P-M27, P-M33), and the postsynaptic density (P-M6, P-M8). In contrast, LBD-associated modules with highly positive disease correlations reflected myelination (P-M4), matrisome / cell adhesion (P-M10), telomere maintenance (P-M11), RNA binding / splicing (P-M13), presynaptic vesicular transport / signaling (P-M17, P-M19, P-M26), sugar metabolism (P-M18), and proteasome function (P-M21) (Fig. 1 E). Module abundance plots across diagnostic groups supported these correlation analyses, with LBD displaying significantly increased levels of positively correlated modules and significantly decreased levels of negatively correlated modules (Fig. 2 A-H). Our pre-network regression for protein variation due to age, sex, and PMI resulted in very few notable module correlations to these traits. Yet, because our LB cohorts tended to skew older and more male compared to controls, it was difficult to eliminate all age- and sex-related variance from the data and select modules, such as P-M1, did appear to significantly correlate with both disease and these demographics (Table S5).
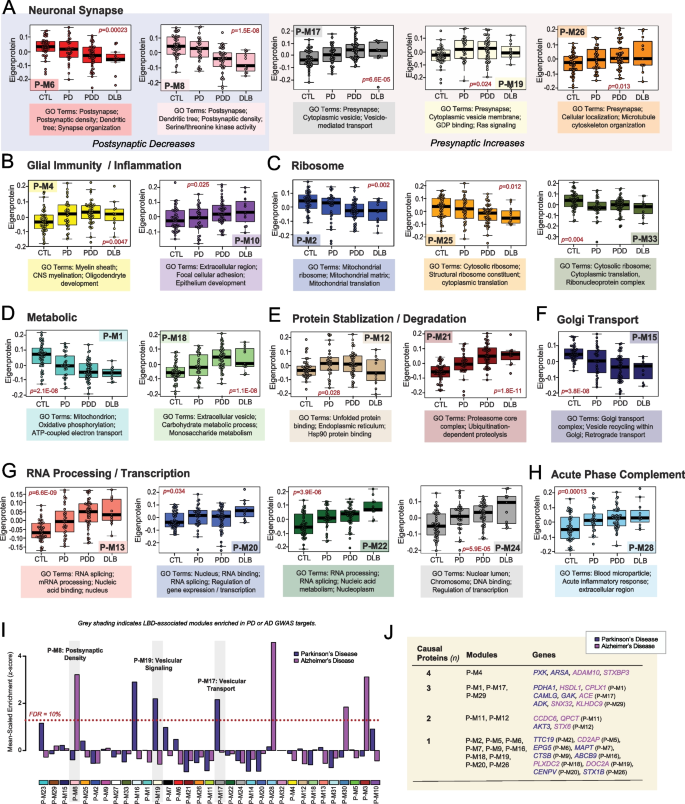
Module abundances of UPenn LBD network reflect significant disease-associated alterations across diverse biological ontologies. A-H Abundance levels (eigenproteins) of select modules across control and disease groups with their top associated biological ontologies. ANOVA p values are provided for each abundance plot. All modules depicted were significantly altered ( p < 0.05) across the four groups. Box plots represent the median and interquartile range between the 25th and 75th percentiles, while data points up to 1.5 times the interquartile range from the box hinge define the extent of error bar whiskers. I Module enrichment of PD (dark blue) and AD (purple) genetic risk factor proteins identified by GWAS. The dashed red line indicates a z score of 1.28, above which enrichment was significant ( p = 0.05) with an FDR of < 10%. Gray shading indicates those modules with significant LBD-associated alterations in disease that were also significantly enriched with GWAS targets. Modules are ordered by relatedness as shown in Fig. 1 D. J Table highlighting modules containing proteins identified by integrative multi-omic analysis as maintaining a causal role in PD (dark blue) and AD (purple). Abbreviations: FDR, false discovery rate
Most LBD-associated modules were also highly correlated to one or more of the core neuropathologies (Fig. 1 E). A few modules stood out for their selectively strong correlations to LB burden, most notably two synaptic modules (P-M17, P-M26) that featured strong positive correlations to LB deposition over other neuropathological traits (Fig. 1 E). These two modules, as well as a third synaptic module (P-M19), also displayed interesting abundance trends. In our prior AD networks, synaptic modules were uniformly decreased in disease [ 17 , 18 , 20 ]. Yet, these three synaptic modules demonstrated significant increases in both PDD and DLB (Fig. 2 A). The presynaptic compartment (vesicular signaling / transport, cell localization) was most strongly reflected among the top GO terms for these elevated modules (Table S6). Accordingly, P-M19 contained SNCA among its module members, a protein that has been repeatedly linked to presynaptic signaling and membrane trafficking [ 45 ]. There was a fourth presynaptic module (P-M7) that was not significantly altered in LBD, suggesting some selectivity in the upregulation of presynaptic proteins. Neuronal modules associated with the postsynaptic compartment (P-M6, P-M8) were significantly decreased in PDD and DLB, aligning more with our previous observations in AD (Fig. 2 A). These postsynaptic modules featured strong negative correlations to neuritic plaque, tau, and LB pathology levels (Fig. 1 E). In sum, these results suggested that network-level changes among synuclein-associated presynaptic proteins may diverge between LBD and AD.
LBD-associated modules are enriched in genetic risk targets
To investigate causal relationships to disease among our LBD-associated modules, we analyzed each for enrichment of specific disease associated GWAS targets. This analysis was performed using a gene and gene-set analysis tool called MAGMA [ 46 ]. Given LBD shares a significant number of genetic risk factors with both PD and AD [ 47 , 48 ], we utilized GWAS targets for these two disorders. Two LBD-associated pre-synaptic modules (P-M17, P-M19) were significantly enriched in PD GWAS targets (Fig. 2 I, Table S7). P-M16 was also enriched in PD targets, but this axonal module was not significantly altered in our LBD network. Post-synaptic P-M8 was the only LBD-associated module enriched with AD GWAS targets (Fig. 2 I, Table S8). To complement this GWAS enrichment analysis, we also examined our modules for the inclusion of proteins identified in a recent integrative multi-omic analysis as maintaining a pleiotropic or causal role in neuropsychiatric disease, including PD and AD [ 49 ]. Of these 48 causal proteins, 27 mapped to a module in our LBD network (Fig. 2 J). Again, synaptic modules were highly represented among these causal proteins, most notably P-M17 which harbored PD causal proteins calcium modulating ligand (CAMLG) and cyclin G associated kinase (GAK) and the AD causal protein angiotensin 1 converting enzyme (ACE). The remaining presynaptic modules (P-M7, P-M19, P-M26) each featured one causal protein each. Among postsynaptic modules, P-M6 harbored the PD causal protein ectopic P-granules 5 autophagy tethering factor (EPG5). Other LBD-associated modules linked to causal proteins included P-M1 mitochondrion, P-M4 myelination, P-M11 telomere maintenance, and P-M12 unfolded protein response. In summary, these findings highlighted modules with potential causative relationships to disease with select synaptic modules once again emerging as interesting given their enrichment with genetic risk targets.
Alpha-synuclein protein serves as a bottleneck to LBD-associated presynaptic modules
In co-expression protein networks, there are two predominant categories of centrally important molecules: 1) hub proteins and 2) bottleneck proteins. A hub protein is critical to the structure of its assigned module, harboring large numbers of interactors within its community of co-expressed proteins. Thus, the deletion or removal of a hub from a network is often lethal to cells [ 50 , 51 ]. In contrast, bottleneck proteins mediate the flow of information between modules, representing key connectors across different communities of co-expressed proteins. The disruption of a protein so critical to module communication could partition the network and similarly cause significant harm to the cell [ 50 , 51 ]. Given its central neuropathological role in LBD, we were interested in whether SNCA played one or more of these key roles in our proteomic co-expression network.
WGCNA assigns each protein to only one module based on the strength of its correlation to the module eigenprotein [ 52 ]. This correlation metric (kME) can also be used to identify module hubs, which are often defined as those proteins ranking among the top 20% of module members based on kME [ 50 , 52 ]. Using these criteria, SNCA was not a hub of its assigned module (P-M19). While it featured a moderately strong correlation to P-M19 (kME = 0.6838), SNCA ranked 95 among its 242 module members and fell well outside of hub status (Fig. 3 A). Yet, we noticed that SNCA harbored kMEs of similar strength to other modules, including P-M7 (kME = 0.6245), P-M17 (kME = 0.5820), and P-M26 (kME = 0.5688) (Table S4). Like P-M19, all three of these modules featured strong links to presynaptic gene ontologies. Furthermore, P-M17 and P-M26 were also highly correlated to LB deposition, as opposed to neuritic plaque and NFT accumulation. These observations indicated that while it was not a hub of its assigned module, SNCA may play more of a central role in the communication between this group of closely related presynaptic modules.

Alpha-synuclein serves as a bottleneck protein between presynaptic UPenn modules. A Graphical representation of SNCA module membership (kME) relative to four neuronal modules in the UPenn co-expression network. While assigned to P-M19, SNCA was not a hub of this module. In addition, SNCA maintained moderately strong correlations to P-M7, P-M17, and P-M26 with kME values approaching or exceeding 0.6. All four modules were linked to presynaptic biological ontologies. The top hub proteins for P-M19 are shown. B Graphical representation of the bottleneck analysis performed among the four presynaptic modules of interest. Top bottlenecks for P-M19 across these modules are shown based on measures of betweenness centrality ( g ). SNCA featured the highest g value of P-M19, indicating its central role in information flow between the four modules. C Volcano plot displaying the biweight midcorrelation (bicor) to SNCA abundance (x-axis) against the -log 10 statistical p value (y-axis) for all proteins quantified in the UPenn dataset. Proteins are shaded according to color of module membership. There were 2,394 proteins with statistically significant ( p < 0.05) negative correlations and 2222 proteins with significant positive correlations to SNCA abundance. Presynaptic modules were among those most highly represented among proteins with the strongest positive SNCA correlations. SNCA -log 10 p value was set from > 200 to 25 to keep plot scale. D Abundance plots for select individual proteins with significant positive correlations to SNCA abundance, highlighting presynaptic modules P-M17, P-M19, and P-M26. ANOVA p values are provided for each abundance plot (*, p < 0.05; **, p < 0.01; ***, p < 0.001). Module eigenprotein box plots represent the median and 25th and 75th percentiles, while data points up to 1.5 times the interquartile range from each box hinge define the extent of error bar whiskers. Abbreviations: SNCA, α-synuclein
We thus investigated whether SNCA might serve as a bottleneck protein between these four modules. In network theory, bottleneck status is typically measured using the metric “betweenness centrality ( g )”, which in WGCNA can be calculated utilizing the topological overlap matrix generated with each network [ 52 , 53 ]. Applying this method, we were thus able to measure and rank the top bottleneck proteins of P-M19 among the presynaptic modules of interest. SNCA was identified as the strongest bottleneck in this analysis ( g = 0.003089), highlighting this protein as central to flow of information between the four modules (Fig. 3 B, Table S9). This result underscored the importance of SNCA within the UPenn LBD network and further bolstered the links we previously observed between these presynaptic modules and neuropathological LB burden.
Accordingly, the same presynaptic modules were also highly represented among individual proteins with strong positive correlations to SNCA abundance levels (Fig. 3 C-D, Table S10). These highly correlated proteins included known SNCA interactors, such as beta-synuclein (SNCB) of P-M26 and synaptobrevin-2 (VAMP2) of P-M19 [ 45 , 54 , 55 ]. P-M19 and P-M26 also harbored numerous other SNCA-correlated cell surface proteins, such as L1 cell adhesion molecule (L1CAM), neural cell adhesion molecule 1 (NCAM1), and ankyrin 2 (ANK2). Synuclein levels in L1CAM-positive plasma-derived exosomes have emerged recently as a possible prodromal PD biomarker, supporting the strong association between these proteins [ 56 , 57 ]. The highly positive correlation between SNCA and cholinergic receptor muscarinic 1 (CHRM1) of P-M26 suggested synuclein-mediated cholinergic dysfunction may play a key role in LBD pathophysiology. Many signaling molecules from P-M17 were also among those proteins highly correlated to SNCA, including various members of the G protein family (GNAO1, GNB1, GNB2, GRM5). Select SNCA-correlated proteins among these presynaptic modules appeared more strongly linked to protein transport and targeting, such as tumor protein D52 (TPD52) of P-M17 and NEDD8 ubiquitin like modifier (NEDD8) of P-M26. TPD52 localizes primarily to endoplasmic reticulum (ER), highlighting the complex interactions between the ER and synaptic compartments. Indeed, the ER of neurons is known to mediate several aspects of synaptic transmission, including calcium signaling / homeostasis and vesicular trafficking [ 58 , 59 , 60 ].
In sum, we established that SNCA serves as a key bottleneck node to modules with strong links to presynaptic ontologies, robust elevations in LBD, and positive correlations to LB pathology. Numerous individual proteins across these modules demonstrated strong correlations to SNCA abundance and together reflected a wide range of synapse-associated processes.
LBD-associated network alterations are preserved in replication analyses
Emory replication analysis.
To examine the validity of our LBD-associated network findings, we analyzed the proteome of a separate cohort of DLPFC tissues derived from the Emory University ADRC brain bank. These cases included tissues with neuropathologically confirmed diagnoses of control ( n = 15), PDD ( n = 10), and DLB ( n = 19). Like the UPenn cohort, Emory cases with dementia were on average in their mid-70 s (PDD = 75.3 ± 10.7, DLB = 74.6 ± 7.9) and predominantly male (Tables S11-12). Neuritic plaque deposition was common among the Emory LBD cases. Nearly all Emory DLB cases ( n = 17) and half of the PDD cases ( n = 5) featured moderate to severe levels of plaque deposition (CERAD 2–3). NFT tau levels were overall milder. Yet, 10 of the 19 DLB cases featured severe NFT deposition (Braak V-VI), increasing the likelihood that both AD and LBD pathology were contributing to cognitive decline within this group. All Emory LBD cases featured some degree of LB deposition throughout the frontal cortex. Almost all DLB cases harbored frequent frontal LB inclusions, while those with PDD generally maintained lower burdens often ranging from sparse to moderate.
TMT-MS analysis across all 44 Emory cases quantified 8,213 proteins (Fig. 4 A), including only those proteins quantified in at least 50% of samples. Like the UPenn cohort, TAMPOR was used to minimize technical variance and the protein abundance data was regressed for variance due to age, sex, and PMI [ 17 , 18 , 27 ]. We then used WGCNA to build a co-expression network on the dataset. The resultant network comprised 7,829 proteins organized into 39 Emory (E) modules with the largest (E-M1) featuring 431 proteins and the smallest (E-M39) harboring 90 proteins (Fig. 4 B, Table S13). Module eigenprotein correlations were similar to those observed in the UPenn LBD network. This included strongly negative disease and pathological correlations among modules linked to postsynaptic (E-M3, E-M10, E-M25), ribosomal (E-M23, E-M36), mitochondrial (E-M2, E-M5, E-M19), and cilia function (E-M6, E-M33) (Table S14-S15). Highly positive disease and pathological correlations among modules linked to the matrisome / cell adhesion (E-M8), proteasome (E-M11), transcription / RNA localization (E-M12), and ER / synaptic signaling (E-M24) also mirrored our UPenn findings. Module preservation analysis revealed that all 39 Emory modules were highly preserved (z score > 10) in the UPenn network (Fig. 4 C). To directly compare the expression trends of preserved modules across the two LBD networks, we examined the weighted module abundance of the top 20% of proteins by kME in each Emory module within the UPenn dataset. These UPenn “synthetic eigenproteins” revealed strong concordance between abundance trends in both networks across a variety of biological ontologies (Fig. 4 D).

LBD-associated network alterations are replicated in an Emory tissue cohort. A Study approach for analyzing co-expression across the Emory DLPFC tissues and comparing these network-level alterations to the UPenn dataset. TMT-MS resulted in the quantification of 8,213 proteins across all cases, which included 15 controls, 10 PDD, and 19 DLB tissues. The Emory and UPenn networks were compared using module preservation and overlap analyses. B Co-expression network generated by WGCNA across all Emory cases, consisting of 39 modules each labeled with a number and color. Module relatedness is shown in the dendrogram. As in the UPenn network, module abundances were correlated to each disease diagnosis and measures of pathological burden with positive correlations indicated in red and negative correlations in blue. Gene ontology analysis was used to identify the primary biology reflected by each module. Asterisks in each heat map indicate the statistical significance of the trait correlation (*, p < 0.05; **, p < 0.01; ***, p < 0.001). C Module preservation analysis of the Emory network into the UPenn network. Modules with a Z summary score of greater than or equal to 1.96 ( q = 0.05, blue dotted line) were considered preserved, while modules with Z summary scores of greater than or equal to 10 ( q = 1.0E-23, red dotted line) were considered highly preserved. D Select Emory network module eigenproteins with their corresponding synthetic eigenproteins in the UPenn network. The UPenn synthetic eigenproteins reflected the weighted module abundance of the top 20% of proteins by kME comprising each Emory module. All Emory module eigenproteins shown were significantly altered ( p < 0.05) across groups with synthetic eigenproteins that replicated in the UPenn network. ANOVA p values are provided for each eigenprotein plot. Box plots represent the median and 25th and 75th percentiles, while data points up to 1.5 times the interquartile range from the box hinge define the extent of error bar whiskers. E Graphical representation of individual proteins in the E-24 module arranged by kME with strong hubs at the center. SNCA is designated in bold. F Plots of individual protein abundances across groups for members of the E24 protein module. ANOVA p values are provided for each abundance plot (*, p < 0.05; **, p < 0.01; ***, p < 0.001). Abbreviations: CTL, control; PDD, Parkinson’s disease dementia; DLB, Dementia with Lewy bodies; SNCA, α-synuclein; ER, endoplasmic reticulum
A hypergeometric Fisher’s exact test (FET) revealed a closely related cluster of Emory modules (E-M24, E-M26, E-M29) that overlapped strongly with the SNCA-associated presynaptic modules in the UPenn network (Fig. S2). Accordingly, synaptic signaling ontologies were featured among the top GO terms for these Emory modules (Table S15). E-M24 was also strongly linked to ER function, again highlighting the close associations between the synaptic and ER compartments. E-M24 was particularly interesting among this module cluster, as it featured significant increases in LBD and highly positive correlations to frontal LB deposition compared to amyloid and tau. Furthermore, E-M24 harbored SNCA among its module members (Fig. 4 E, Table S13). As in the UPenn network, SNCA was not a strong hub of E-M24 (kME = 0.71). Its kME ranking of 32 within this relatively small module of 161 proteins placed it at the edge of the top 20th percentile. SNCA also maintained similar kME values relative to other synapse-associated Emory modules, including E-M26 (kME = 0.6481) and E-M29 (kME = 0.6210). This suggested a preserved bottleneck role for SNCA within the Emory network. In addition to SNCA, E-M24 also featured stark elevations in many of the same synapse-associated proteins also increased in the UPenn network, including L1CAM, ANK2, TPD52, and NEDD8 (Fig. 4 F). Overall, these results from our Emory cases validated many of the observations from the UPenn network, including increases in proteins associated with SNCA and presynaptic functions.
ROSMAP replication analysis
As a second replication study, we examined the proteomes of DLPFC tissues derived from the Religious Orders Study or Rush Memory and Aging Project (ROSMAP) cohorts [ 61 , 62 , 63 ]. While the UPenn and Emory datasets were newly generated for these LBD analyses, this ROSMAP replication was performed using a previously published TMT-MS dataset comprising 610 total tissues [ 64 ]. We used available clinical and pathological traits to identify three groups of interest among these cases: control ( n = 42), asymptomatic LB pathology (AsymLB, n = 21), and Lewy body dementia (LBD, n = 40) (Tables S16-S17). Control cases comprised those with no cognitive impairment or corticolimbic LB deposition at death. AsymLB was defined as cases with corticolimbic LB deposition but normal cognition at death. Finally, LBD cases included those with clinical dementia and corticolimbic LB pathology present at death. Control cases featured minimal to no AD pathology, while AsymLB and LBD cases were restricted to those with only mild to moderate NFT deposition. These criteria helped ensure a high likelihood that LB deposition, as opposed to AD pathology, was the primary contributor to cognitive decline in our LBD cases [ 26 ].
Using TMT-MS, we quantified a total of 7,801 proteins across these cases. All quantified data was regressed for age, sex, and PMI. We then built a co-expression network on this ROSMAP data for comparison with the UPenn LBD network. We found that the co-expression observed in the UPenn data remained consistent among these ROSMAP cases. All 33 UPenn modules were significantly preserved in the ROSMAP dataset with the majority surpassing preservation z scores of 10 (Fig. S3A). Synthetic eigenproteins of UPenn module members within the ROSMAP dataset demonstrated concordance between the two cohorts across multiple modules, including the presynaptic UPenn modules of interest (P-M17, P-M19, P-M26) (Fig. S3B). Interestingly, among these presynaptic modules, increased protein levels were also observed in those with AsymLB, suggesting these alterations occur early in preclinical LB deposition. In sum, these results provided additional validation of the disease-associated alterations observed in our discovery UPenn cohort.
Network level proteome comparison reveals LBD presynaptic co-expression signatures distinct from AD
Unique pathophysiological signatures of LBD that distinguish it from AD and other related dementias are necessary to advance diagnostic and therapeutic target development. Our LBD network observations suggested key differences in co-expression patterns between LBD and our previous AD networks [ 15 , 16 , 17 , 18 , 19 , 20 , 21 , 22 ] that could inform biomarker discovery. To further compare network-level changes between LBD and AD, we performed a series of overlap analyses with our UPenn LBD network and two separate AD co-expression networks (Fig. 5 A). The first AD network was derived from our UPenn DLPFC tissues, comprising 49 pathologically defined AD cases and the same 47 control cases analyzed in the LBD network (Table S1-S2). The second AD network was a previously published consensus analysis comprising 516 control, asymptomatic AD (AsymAD), and AD DLPFC cases from the Banner Sun Health Research Institute and ROSMAP cohorts [ 17 ]. AsymAD cases were defined as those with an Aβ and NFT burden similar to pathologically defined AD cases but without significant cognitive impairment close to death [ 17 ]. Thus, these tissues represented an early preclinical phase of AD [ 65 ]. We first analyzed the preservation of the 33 modules in our LBD network in these two AD networks (Fig. 5 B). Nearly all LBD modules were highly preserved (Z summary > 10) in both AD networks, indicating that the framework of protein co-expression was consistent across all three datasets.

Network level proteome comparison between LBD and AD reveals divergent signatures. A Study approach for comparing network-level alterations between the UPenn LBD network and two independent AD networks. The first AD network comprised DLPFC UPenn tissues, including 49 AD cases and the same 47 control cases analyzed in the initial UPenn LBD network. The second AD network was a previously published consensus analysis comprising > 500 control, asymptomatic AD (AsymAD), and AD DLPFC cases from the Banner Sun Health Research Institute and ROSMAP cohorts. Module preservation and overlap analyses were used to compare these networks. B Module preservation analyses of the UPenn LBD network into the UPenn and Consensus AD networks. Modules with a Z summary score of greater than or equal to 1.96 ( q = 0.05, blue dotted line) were considered preserved, while modules with Z summary scores of greater than or equal to 10 ( q = 1.0E-23, red dotted line) were considered highly preserved. C-D UPenn LBD network module eigenproteins associated with the postsynaptic ( C ) and presynaptic ( D ) compartments with their corresponding synthetic eigenproteins in the two AD networks. The AD synthetic eigenproteins reflected the weighted module abundance of the top 20% of proteins by kME comprising each LBD module. ANOVA p values are provided for each eigenprotein plot. Box plots represent the median and 25th and 75th percentiles, while data points up to 1.5 times the interquartile range from the box hinge define the extent of error bar whiskers. E Heat maps depicting the fold change magnitude of select presynaptic proteins across UPenn LBD and AD cases. Increases in protein levels are indicated in red, while decreases are in blue. Asterisks in each heat map indicate the statistical significance of the fold change (*, p < 0.05; **, p < 0.01; ***, p < 0.001). Abbreviations: CTL, control; AsymAD, Asymptomatic Alzheimer’s disease; AD, Alzheimer’s disease; PDD, Parkinson’s disease dementia; DLB, Dementia with Lewy bodies
To compare the direction of expression of preserved modules across networks, we examined the synthetic eigenproteins of the LBD modules in each AD network. As with our above replication analyses, these AD synthetic eigenproteins reflected the weighted expression profiles of the top 20% of proteins in each UPenn LBD module. As expected, synaptic modules significantly decreased in LBD were also significantly decreased in AD (Fig. 5 C). This included P-M6 and P-M8, which were both associated with the postsynaptic density. In contrast, modules associated with presynaptic functions (P-M7, P-M17, P-M19, P-M26) showed much less concordance between LBD and AD (Fig. 5 D). P-M19 and P-M26 were significantly increased in LBD but decreased in AD, while P-M17 featured significant increases in LBD but remained largely unchanged in AD. Finally, P-M7 was unchanged in LBD but significantly decreased in AD. Individual proteins with the starkest divergence in expression trends between LBD and AD are highlighted in Fig. 5 E. These differentially expressed markers included SNCA and several co-expressed proteins of interest, such as L1CAM, ANK2, NEDD8, and CEP68. These findings supported our observations that the LBD frontal cortex harbored expression trends among synaptic proteins that diverged from those found in AD. These results also suggested that these two diseases may feature marked differences in presynaptic protein pathophysiology.
Matrisome-associated protein levels differentiate LBD cases with high levels of amyloid co-pathology
Overlapping AD pathology is extremely frequent in both DLB and PDD with as many as 90% of cases harboring accumulation of extracellular amyloid-beta (Aβ) plaques [ 10 ]. The presence of this concurrent pathology can also influence clinical progression and disease severity. Therefore, we were interested in examining protein expression trends across cases with low and high AD pathology burden. In our UPenn cohort, nearly half of the 58 LBD cases harbored minimal to no Aβ plaque pathology (CERAD 0–1), including 26 PDD and 2 DLB cases. The remaining cases, including 21 PDD and 9 DLB, featured moderate to severe plaque pathology (CERAD 2–3). Differential expression analysis of these low- and high-amyloid LBD cases revealed > 700 proteins significantly altered between these two groups (Fig. 6 A, Table S18). As expected, this included APP and several additional members of P-M13 (NXPH1, OSTM1), which were significantly elevated in the high-amyloid cases compared to those with low amyloid levels. Yet, most altered were numerous members of P-M10 (MDK, NTN1, SMOC1, CTHRC1), a glial and endothelial enriched module highly linked to the extracellular matrix (i.e., matrisome) and cell adhesion. Compared to low-amyloid LBD cases, these P-M10 markers demonstrated large, highly significant fold-change elevations in those with high-amyloid LBD and were even more elevated in the UPenn AD cases (Fig. 6 B-C). These results aligned well with our previous observations in the AD brain network, in which these matrisome proteins serve as hubs of a highly preserved plaque-associated module consistently elevated in AD [ 17 , 18 ]. Interestingly, certain matrisome markers were significantly decreased in low-amyloid LBD compared to controls (Fig. 6 B-C), suggesting a separate physiological process in this cohort in which the expression of these plaque-associated proteins is actively suppressed. Such markers included midkine (MDK), a heparin-binding growth factor involved in cell growth and angiogenesis, and collagen triple helix repeat containing 1 (CTHRC1), a protein implicated in vascular remodeling and the cellular response to arterial injury [ 66 , 67 ]. Both are often elevated in not only AD, but also cancer and tumorigenesis. Overall, these results revealed how the LBD brain network can be a source of not only unique pathophysiological markers, but also those that overlap with other neurodegenerative conditions, distinguishing LBD cases with mixed pathology from cases with more pure LB deposition. It also highlighted potential divergent glial-mediated pathophysiology in LBD cases with low versus high amyloid plaque burdens.

Matrisome proteins distinguish UPenn LBD cases with low and high amyloid burden. A Volcano plot displaying the log 2 fold change (x-axis) against the -log 10 statistical p value (y-axis) for proteins differentially expressed between UPenn LBD cases with low (CERAD 0–1) versus high (CERAD 2–3) amyloid deposition. All p values were derived by t-test analysis. Proteins are shaded according to color of module membership. Proteins mapping to M10 matrisome in the UPenn LBD network were among those most differentially expressed. B Heat maps depicting the fold change magnitude of select matrisome and other proteins across UPenn LBD and AD cases. Increases in protein levels are indicated in red, while decreases are in blue. Asterisks in each heat map indicate the statistical significance of the fold change (*, p < 0.05; **, p < 0.01; ***, p < 0.001). (C) Plots of individual protein abundances across groups, including low- and high-amyloid LBD. ANOVA p values are provided for each abundance plot (*, p < 0.05; **, p < 0.01; ***, p < 0.001). Abbreviations: CTL, control; PD, Parkinson’s disease; LBD Low , Low-amyloid Lewy body dementia; LBD High , High-amyloid Lewy body dementia
The corticolimbic pathophysiology underlying the aggressive cognitive and neuropsychiatric deterioration in LBD is extremely complex, poorly understood, and features significant overlap with AD. In the current study, we employed co-expression network proteomics to define systems-based pathophysiologic alterations in the frontal cortex of a large UPenn autopsy cohort and compare these signatures to those observed in AD. We identified a diverse array of protein modules altered in the brains of those with PDD and DLB, encompassing synaptic, metabolic, and inflammatory pathophysiology. We then validated these network signatures across independent LBD cohorts and identified reproducible synaptic alterations that diverged from those in the AD brain. We also identified informative overlapping signatures between LBD and AD, including glial-associated matrisome markers that proved highly concordant with Aβ deposition and capable of stratifying LBD cases with low versus high burdens of amyloid plaque co-pathology. These results underscore how proteomic co-expression network analysis can yield insights into key divergent and overlapping pathophysiological signatures in the LBD and AD brain.
Synaptic protein loss is generally considered a universal feature of the neuropathological changes observed in dementia. Numerous studies in AD have shown that pathological measures of synaptic loss correlate more strongly with cognitive impairment compared to Aβ and tau pathology [ 68 , 69 , 70 , 71 ]. Accordingly, we and others have observed stark decreases in a variety of synaptic proteins in the AD brain across multiple independent cohorts and brain regions [ 15 , 16 , 17 , 18 , 19 , 20 , 21 , 72 ]. We have also identified these synaptic decreases in the brains of those with AsymAD, or individuals with significant neuritic plaque and NFT deposition but no evidence of clinical cognitive impairment at death [ 17 , 18 , 20 ]. These results suggest early synaptic losses in AD independent of clinical declines. Aligning with these observations, we found significant decreases among our LBD cases in two large modules linked to postsynaptic function (P-M6, P-M8). These modules included synaptic markers that already feature well-described decreases in neurodegeneration, such as VGF and NPTX2 [ 31 , 32 , 33 , 34 , 35 , 36 , 37 , 38 , 39 ], reinforcing their potential as reliable markers of degeneration across different neurologic diseases.
Yet, we also observed increased levels in presynaptic modules among our UPenn LBD cases, contrasting with our prior AD observations. These included P-M17, a neuron-enriched module linked to synaptic vesicular transport that featured various GTPases among its hub proteins (RAB1A, GNB2, GNAO1), as well as P-M19, another neuronal module that included SNCA and other proteins linked to vesicular signaling. The third neuronal module significantly increased in LBD was P-M26, which maintained ontological associations to both the synapse and cellular localization. While its hub proteins included largely cell surface proteins (ANK2, L1CAM), this module also featured proteins involved in protein targeting, folding, and processing (CEP68, NEDD8). All three of these modules were either unchanged or significantly decreased when examined in our comparison AD networks, indicating divergent pathophysiology between the two dementias. The reasons underlying these presynaptic increases in LBD are unclear and will require further investigation. The network-based links these modules shared with SNCA levels and LB deposition suggests that aberrant SNCA function is to some extent mediating these findings. Yet, it is still possible these increases represent a compensatory, rather than pathological, response in this brain region.
SNCA served as a strong bottleneck node among these presynaptic modules. While hub proteins, which demonstrate high connectivity within their respective modules, are often considered key molecular drivers in co-expression networks [ 73 ], there is also growing recognition that proteins with a high degree of connectivity between modules are also highly relevant to disease. These bottleneck proteins have been shown to play central roles in various disorders and serve as successful therapeutic targets [ 50 , 74 , 75 , 76 ]. As a strong presynaptic bottleneck, SNCA mediated communication between the three modules highlighted above (P-M17, P-M19, P-M26), as well as a fourth larger module (P-M7) also heavily associated with vesicular signaling and the presynaptic compartment. This aligns with the growing amount of research indicating SNCA localizes to the presynaptic terminal and participates in vesicular cycling, including regulation of vesicle pool size, mobilization, and endocytosis [ 45 ]. In addition, these presynaptic modules featured proteins known to interact closely with SNCA, such as synaptobrevin-2 (VAMP2) and synapsin 1 (SYN1) [ 45 , 55 , 77 ]. L1 cell adhesion molecule (L1CAM), an increasingly studied neuronal surface marker for isolating and measuring exosome associated SNCA in biofluids [ 56 , 57 ], also mapped to these presynaptic modules (P-M26), and its increasing abundance in LBD correlated strongly with those of SNCA. Thus, our LBD network underscored well-established links between SNCA and the presynaptic compartment and supported its central role in the unique pathophysiological changes of LBD.
These presynaptic LBD signatures also revealed unique alterations in cholinergic pathways. P-M26 featured muscarinic cholinergic receptors CHRM1 and CHRM4, which have both demonstrated promise as synaptic targets for cognitive and behavioral symptoms in dementia [ 78 , 79 ]. Drugs that enhance synaptic CHRM1 and CHRM4 activity are currently being explored in the management of both AD and DLB. Compared to controls, both receptors demonstrated significant increases among LBD cases but stable, largely unchanged levels in AD. While this reveals potential differences in AD and LBD cholinergic function, it also suggests both diseases are able to preserve these receptors to some extent and may be responsive to CHRM1 and CHRM4 agonists. The stark increases LBD demonstrated in these cholinergic receptors, as well as others (CHRM2, CHRM3), could be in response to significant losses in other components of the cholinergic pathways. For instance, all three LB disorders in our UPenn network demonstrated dramatic, several-fold decreases in SLC5A7, a protein necessary for presynaptic choline uptake and ACh synthesis [ 42 ]. Of note, though much of its activity is localized to the presynaptic terminal, SLC5A7 expression most closely aligned with postsynaptic P-M8. Thus, despite their predominant ontologies, it is important to note that all our neuronal modules likely harbor some mixture of proteins that function in both the presynaptic and postsynaptic spaces. Nevertheless, these cholinergic trends further showcased the diversity of alterations within LBD synaptic pathways, highlighting those proteins with preserved to increased levels in disease that may respond well to therapeutic agonists.
Synaptic pathways were not the only ones distinctly altered in LBD. In both the UPenn and Emory LBD networks, proteins involved in protein targeting, folding, and ER function were also co-expressed with elevated presynaptic LBD markers. P-M26 best reflected this co-expression of synaptic and protein processing molecules in the UPenn network, while E-M24 of the Emory network mapped strongly to both synaptic and ER ontologies. These network associations likely reflect the well-established functional relationships between ER and synaptic regulation [ 58 , 59 , 60 ]. Many have also linked SNCA itself to ER stress and aberrant protein processing. For instance, Colla et al. found oligomeric species of SNCA in the ER of both animal and human brains with synucleinopathy [ 80 ], suggesting an ER-mediated stress response may play an integral role in disease pathophysiology. Others have also reported colocalization of ER stress markers with synuclein inclusions in diseased brain tissue [ 81 , 82 ]. Furthermore, LB disease has been linked to various disruptions in protein processing, such as neddylation [ 83 ]. NEDD8, a ubiquitin-like protein involved prominently in neddylation, was among the synapse-associated modules in our UPenn and Emory networks that demonstrated strong increases in LBD and marked decreases in AD. Dysfunction of this protein has been previously linked to multiple neurodegenerative diseases with ubiquitinated inclusions [ 83 , 84 , 85 ]. However, our results suggest the pathophysiological mechanism underlying this dysfunction may differ between LBD and AD.
These divergent network changes could eventually yield much needed diagnostic and therapeutic markers of LBD. The observation in our ROSMAP cases that these presynaptic proteins are elevated in asymptomatic disease further supports their potential clinical utility as early targets. In addition, tools that help identify overlapping LBD and AD co-pathology are also useful. Studies have shown that the burden and distribution of AD pathology can have a significant impact on LBD presentation and progression [ 86 , 87 ]. Our network analysis revealed that proteins associated with the extracellular matrix (ECM) were best at distinguishing LBD cases with low versus high levels of amyloid co-pathology. This supports growing evidence that these proteins associate strongly with amyloid plaques [ 72 ]. Furthermore, these results underscored our prior AD brain network analyses, which have established many of these matrisome proteins (SMOC1, NTN1, MDK) as hubs of a highly preserved glia-associated module consistently elevated in both AsymAD and AD [ 16 , 17 , 18 ]. Our current LBD data provides an additional role for these emerging biomarkers as early indicators of AD co-pathology in LB populations, which could help guide clinical management throughout the course of disease. In addition, their elevation in such a sizeable portion of LBD patients suggests these markers could provide another avenue independent of SNCA for therapeutic targeting.
Prior integrative proteomic studies from our group and others have demonstrated the translation potential of brain network analysis into promising biofluid markers of disease [ 16 , 18 , 23 , 72 , 88 ]. We have previously demonstrated up to 70% overlap between the brain and CSF proteomes using TMT-MS. This has allowed us to identify panels of CSF biomarkers in AD reflecting a diverse range of pathophysiology and further validate these markers in cross-sectional and longitudinal AD cohorts [ 16 , 18 , 23 , 24 , 88 ]. In a recent manuscript, we showed that a panel of 48 CSF AD markers, originally identified in brain network analyses, improved early diagnostic and predictive assessments of sporadic AD [ 23 ]. A separate study demonstrated that CSF levels of matrisome markers SMOC1 and SPON1 were elevated nearly 30 years prior to the onset of symptoms in an autosomal dominant AD population [ 24 ]. Thus, future directions include integrating the LBD brain network proteome with the CSF and plasma proteomes of diseased patients to similarly identify molecularly diverse biofluid panels that could advance the diagnostic and predictive accuracy of LBD.
Our focus on the DLPFC could be viewed as a limitation in this study. This region was chosen because it is commonly affected in diffuse neocortical LBD and routinely scored in its neuropathological diagnosis. In addition, frontal executive deficits are commonly among the first symptoms observed in LBD [ 26 ], indicating this region could provide a valuable map of early pathophysiological changes in the evolution of LB-mediated cognitive changes. The robust differential expression we observed even in non-demented PD cases further suggests this region is affected early in the brainstem-to-corticolimbic disease evolution thought to eventually provoke PDD. Yet, it is possible other heavily affected regions in LBD, including the inferior temporal and parietal lobes, may yield different network findings and additional insights. Among other limitations, we also regressed our proteomic data for alterations related to age and sex. While this prioritized alterations most associated with disease, it also resulted in an incomplete examination of these demographic effects on protein levels. In our prior network studies of the AD brain proteome, we have found that both age and sex have a very limited impact on disease-associated module trends [ 18 ]. Yet, it will be important in future studies, particularly when examining these markers in biofluids, to utilize large, more diverse cohorts to define the impact of age, sex, race, and other demographic variables on LBD protein signatures.
In summary, our study offers a network-level map of the LBD brain proteome, which revealed disease-associated alterations in a diverse range of protein systems. Using this network, we were able to identify key overlapping and divergent protein signatures in LBD and AD tissues and correlate these disease-associated alterations to core neuropathologies. These results can serve as a strong systems-based framework for future integrative studies focused on identifying protein biofluid markers relevant to corticolimbic pathophysiology in the LBD brain.
UPenn and Emory brain tissues
Human postmortem DLPFC (BA 9) brain tissues were acquired by the UPenn and Emory ADRCs in accordance with their respective Institutional Review Board (IRB) protocols. Disease classification reflected neuropathological diagnoses provided by expert pathologists at each institution. These diagnoses were made in accordance with established criteria and guidelines [ 26 , 89 ]. In both cohorts, neuritic plaque distribution was scored according to the Consortium to Establish a Registry for Alzheimer’s Disease (CERAD) criteria [ 29 ], and the extent of neurofibrillary tangle pathology was assessed with the Braak staging system [ 28 ]. The frequency of LB deposition in the frontal cortex was scored in the UPenn and Emory cases using similar semi-quantitative scales. The UPenn scale included scores of 0 (absent), 1 (sparse), 2 (moderate), and 3 (frequent), while the Emory scale comprised scores of 0 (absent), 1 (sparse to moderate), and 2 (frequent). Mini-Mental Status Examination (MMSE) scores were available for a subset of UPenn tissues. All sample metadata are provided in https://www.synapse.org/#!Synapse:syn53177242 . The subsequent Methods sections outline the processing, TMT labeling, and MS analysis of these tissue cohorts, which were newly analyzed for this study. All procedures were performed within the Emory University Center for Neurodegenerative Disease and remained largely consistent across cohorts with minor differences where indicated. A total of 354 UPenn samples with a variety of neurological diagnoses were processed and MS quantified, only diagnoses of interest (i.e., control, PD, PDD, DLB, AD) were included in the current analyses. For the Emory replication analyses, only control, PDD, and DLB cases were available.
ROSMAP brain tissues
The ROSMAP analyses in this manuscript were performed using a previously published dataset comprising a total of 610 DLPFC (BA 9) samples [ 64 ]. All ROSMAP participants signed informed and repository consents and an Anatomic Gift Act. The available traits for these cases have been previously described [ 64 ]. LB deposition was scored using a global regional scale that indicated whether these inclusions were absent, nigral-predominant, limbic-type, or neocortical-type. Clinical consensus cognitive diagnoses were also provided for ROSMAP cases based on detailed neuropsychological testing, which indicated whether an individual had no cognitive impairment (NCI), mild cognitive impairment (MCI), or dementia at death. For the current manuscript, disease classification of control, AsymLB, and LBD were determined using a combination of available clinical and pathological traits. Controls included those with NCI, absent corticolimbic LBs, and minimal neuritic plaque and NFT deposition (CERAD 0–1, Braak NFT 0-II). AsymLB cases were those with NCI, present corticolimbic LBs, and mild to moderate NFT deposition (Braak NFT 0-IV), while those with LBD featured dementia at death, present corticolimbic LBs, and mild to moderate NFT deposition (Braak NFT 0-IV). These criteria surrounding tau levels helped ensure a high likelihood that LB deposition, as opposed to AD pathology, was the primary contributor to cognitive decline in our LBD cases [ 26 ]. These diagnostic classifications encompassed 103 of the 610 cases in the original cohort. All sample metadata are provided in https://www.synapse.org/#!Synapse:syn53177242 . Detailed methods have been published for the processing and MS analysis of the ROSMAP cohort [ 64 ], which we refer to in subsequent sections when appropriate.
Brain tissue homogenization and protein digestion
Tissue homogenization of all cases was performed essentially as described [ 20 , 90 ]. Approximately 100 mg (wet weight) of each tissue sample was homogenized in 500 μL of 8 M urea lysis buffer (8 M urea, 10 mM Tris, 100 mM NaH2PO4, pH 8.5) with HALT protease and phosphatase inhibitor cocktail (ThermoFisher). Tissues were added to the lysis buffer immediately after excision in Rino sample tubes (NextAdvance) supplemented with ~ 100 μL of stainless-steel beads (0.9 to 2.0 mm blend, NextAdvance). Using a Bullet Blender (NextAdvance), tissues were then homogenized at 4 °C with 2 full 5 min cycles. The lysates were transferred to new Eppendorf Lobind tubes and sonicated for 3 cycles, each lasting 5 s at 30% amplitude. Sample lysates were then centrifuged for 5 min at 15,000 × g and the supernatant transferred to new tubes. Protein concentration was determined by bicinchoninic acid (BCA) assay (Pierce). For protein digestion, 100 μg of each sample was aliquoted and volumes normalized with additional lysis buffer. Samples were reduced with 1 mM dithiothreitol (DTT) at room temperature for 30 min followed by 5 mM iodoacetamide (IAA) alkylation in the dark for another 30 min. Lysyl endopeptidase (Wako) at 1:100 (w/w) was added, and digestion allowed to proceed overnight. Samples were then sevenfold diluted with 50 mM ammonium bicarbonate. Trypsin (Promega) was added at 1:50 (w/w) and digestion was carried out for another 16 h. The peptide solutions were acidified to a final concentration of 1% (vol/vol) formic acid (FA) and 0.1% (vol/vol) trifluoroacetic acid (TFA) before desalting with a 30 mg HLB column (Oasis). Prior to sample loading, each HLB column was rinsed with 1 mL of methanol, washed with 1 mL 50% (vol/vol) acetonitrile (ACN), and equilibrated with 2 × 1 mL 0.1% (vol/vol) TFA. Samples were then loaded onto the column and washed with 2 × 1 mL 0.1% (vol/vol) TFA. Elution was performed with 2 volumes of 0.5 mL 50% (vol/vol) ACN. An equal amount of peptide from each sample was aliquoted and pooled as the global internal standard (GIS), a fraction of which was TMT labeled and included in each batch as described below.
Isobaric Tandem Mass Tag (TMT) peptide labeling
TMT peptide labeling was performed as previously described [ 20 , 90 ]. Prior to labeling, the 354 UPenn cases were randomized into 24 batches by age, sex, and diagnosis. Labeling was performed using TMTpro 16-plex kits (ThermoFisher 44,520). Each batch included one TMT channel with a labeled GIS standard. Labeling of sample peptides was performed as previously described [ 19 , 90 , 91 ]. Briefly, each sample (100 μg of peptides) was re-suspended in 100 mM triethylammonium bicarbonate (TEAB) buffer (100 μL). TMT labeling reagents (5 mg) were equilibrated to room temperature. Anhydrous ACN (256 μL) was added to each reagent channel. Each channel was then gently vortexed for 5 min. A volume of 41 μL from each TMT channel was transferred to each peptide solution and allowed to incubate for 1 h at room temperature. The reaction was quenched with 5% (vol/vol) hydroxylamine (8 μL) (Pierce). All channels were then dried by SpeedVac (LabConco) to approximately 150 μL, diluted with 1 mL of 0.1% (vol/vol) TFA, and acidified to a final concentration of 1% (vol/vol) FA and 0.1% (vol/vol) TFA. Labeled peptides were desalted with a 200 mg C18 Sep-Pak column (Waters). Prior to sample loading, each Sep-Pak column was activated with 3 mL of methanol, washed with 3 mL of 50% (vol/vol) ACN, and equilibrated with 2 × 3 mL of 0.1% TFA. After sample loading, each column was washed with 2 × 3 mL 0.1% (vol/vol) TFA followed by 2 mL of 1% (vol/vol) FA. Elution was performed with 2 volumes of 1.5 mL 50% (vol/vol) ACN. The eluates were then dried to completeness by SpeedVac. The 44 Emory samples were randomized by age and diagnosis into 3 batches and labeled using TMTpro 16-plex kits (ThermoFisher 44520). Each batch included one TMT channel with a labeled GIS standard. Labeling of these sample peptides then proceeded according to the protocols above. Randomization and multiplex labeling of ROSMAP cases were performed according to very similar protocols, as previously described in detail [ 64 ].
High-pH off-line fractionation
High pH fractionation of all cases was performed essentially as described [ 90 , 92 ] with slight modifications. Dried samples were resuspended in high pH loading buffer comprising 0.07% (vol/vol) NH4OH, 0.045% (vol/vol) FA, and 2% (vol/vol) ACN. Resuspended samples were then loaded onto a Water’s Ethylene Bridged Hybrid (BEH) column (1.7 um, 2.1 mm × 150 mm). A Thermo Vanquish high-performance liquid chromatography (HPLC) system was used to carry out the fractionation. Solvent A consisted of 0.0175% (vol/vol) NH4OH, 0.01125% (vol/vol) FA, and 2% (vol/vol) CAN. Solvent B comprised 0.0175% (vol/vol) NH4OH, 0.01125% (vol/vol) FA, and 90% (vol/vol) ACN. The sample elution was performed over a 25 min gradient with a flow rate of 0.6 mL/min. A total of 192 individual equal volume fractions were collected across the gradient and subsequently pooled by concatenation into 96 fractions [ 92 ]. The fractions were then dried to completeness using a SpeedVac.
Mass spectrometry analysis of UPenn samples
MS analysis was performed on the fractionated UPenn samples as previously described with modifications [ 17 , 18 , 20 , 90 ]. Briefly, fractions were resuspended in an equal volume of loading buffer (0.1% FA, 0.03% TFA, 1% ACN) and analyzed by liquid chromatography coupled to tandem mass spectrometry (LC–MS/MS). Peptide eluents were separated on a custom in-house packed Charged Surface Hybrid (CSH) column (1.7 um, 15 cm × 150 μM internal diameter) by a Dionex RSLCnano ultra-performance liquid chromatography (UPLC) system (ThermoFisher Scientific). Buffer A comprised water with 0.1% (vol/vol) FA, and buffer B comprised 80% (vol/vol) ACN in water with 0.1% (vol/vol) FA. Elution was performed over a 30 min gradient with a flow rate of 1500 nL/min. The gradient ranged from 1 to 99% solvent B. Peptides were monitored on a Orbitrap Eclipse mass spectrometer with high-field asymmetric waveform ion mobility spectrometry (FAIMS) (FAIMS Pro Interface, ThermoFisher Scientific). Two compensation voltages were chosen for FAIMS. For each voltage (-45 and -65) top speed cycle of 1.5 s, the full scan (MS1) was performed with an m/z range of 410–1600 and 60,000 resolution at standard settings. The higher energy collision-induced dissociation (HCD) tandem scans were collected at 35% collision energy with an isolation of 0.7 m/z, resolution of 30,000 with TurboTMT, AGC setting of 250% normalized AGC target, and a maximum injection time of 54 ms. For all batches, dynamic exclusion was set to exclude previously sequenced peaks for 20 s within a 10-ppm isolation window.
Mass spectrometry analysis of Emory samples
MS analysis on the fractionated Emory samples was performed similarly to the UPenn samples with modifications. Fractions were resuspended in an equal volume of loading buffer (0.1% FA, 0.03% TFA, 1% ACN) prior to LC–MS/MS analysis. Peptide eluents were separated on a custom in-house packed Charged Surface Hybrid (CSH) column (1.7 um, 15 cm × 150 μM internal diameter) by a Dionex RSLCnano running capillary flow UPLC (ThermoFisher Scientific). Buffer A comprised water with 0.1% (vol/vol) FA, and buffer B comprised 80% (vol/vol) ACN in water with 0.1% (vol/vol) FA. Elution was performed over a 40 min gradient with flow rate of 7 uL/min. The gradient ranged from 1 to 99% solvent B. Peptides were monitored on a Orbitrap Exploris 240 mass spectrometer at 2 s top speed cycle. Each cycle comprised of a full scan (MS1) with an m/z range of 410–1600 and 120,000 resolution at standard settings. The HCD tandem scans were collected at 36% collision energy with an isolation of 0.7 m/z, resolution of 45,000, AGC setting of 250% normalized AGC target, and 100 ms maximum injection time. For all batches, dynamic exclusion was set to exclude previously sequenced peaks for 20 s within a 10-ppm isolation window.
Mass spectrometry of ROSMAP samples
MS analysis of fractionated ROSMAP samples was performed in two separate sets as previously described [ 64 ].
Database searches and protein quantification
All RAW files acquired from TMT-MS of all cases were searched against a human reference protein database using the Proteome Discoverer suite (version 2.4, ThermoFisher Scientific). MS2 spectra were searched against the UniProtKB human proteome database containing Swiss-Prot human reference protein sequences (20,338 target proteins downloaded in 2019). Searches were performed using previously published protocols [ 17 , 18 , 90 ]. Percolator was used to filter peptide spectral matches (PSMs) and peptides to a false discovery rate (FDR) of less than 1%. Following spectral assignment, peptides were assembled into proteins and were further filtered based on the combined probabilities of their constituent peptides to a final FDR of 1%. A multi-consensus in Proteome Discoverer was then performed to achieve parsimonious protein grouping across both sets of samples. In cases of redundancy, shared peptides were assigned to the protein sequence in adherence with the principles of parsimony. As default, the top matching protein or “master protein” was the protein with the largest number of unique peptides and smallest value in the percent peptide coverage (i.e., the longest protein). Reporter ions were quantified using an integration tolerance of 20 ppm with the most confident centroid setting. Only parsimonious peptides were considered for quantification.
Controlling for batch-specific variance
A tunable median polish approach (TAMPOR) [ 27 ] was used to remove technical batch variance in the proteomic data from all three cohorts, as previously described [ 17 ]. TAMPOR is utilized to remove inter-batch variance while preserving meaningful biological variance in protein abundance values, normalizing to the median of selected intra-batch samples and the median samplewise abundance, alternately and iteratively in a median polish [ 27 ]. We have previously applied this batch-correction approach to multiple large proteomic datasets [ 17 , 18 , 64 ]. This approach is robust to outliers and up to 50% of measurements missing. If a protein had more than 50% of samples with missing values, it was removed from the protein abundance matrix. No imputation of missing values was performed for any cohort. For the current data, TAMPOR leveraged the median protein abundance from the pooled GIS TMT channels as the denominators in both factors to normalize sample-specific protein abundances across batches.
Regression of covariates
Following TAMPOR batch correction, the protein abundance matrices from all three cohorts were subjected to non-parametric bootstrap regression by subtracting the covariate of interest multiplied by the median estimated coefficient from 1000 iterations of fitting for each protein in the log 2 (abundance) matrix, as previously described [ 17 ]. The UPenn and Emory datasets were regressed for age, sex, and PMI. The ROSMAP cases were regressed for these three covariates, as well as any residual variation related to batch. Ages at death used for regression were uncensored. Case diagnosis was also explicitly modeled and protected in each iteration.
Weighted Gene Co-expression Network Analysis (WGCNA)
The WGCNA algorithm [ 52 ], implemented into the R library of the same name, was used to perform co-expression network analysis on batch-corrected and regressed TMT-MS data. A total of four co-expression networks were built for this study. For UPenn cases, two separate networks were built on the data matrices from 1) an LB subset of cases including those with neuropathological diagnoses of control, PD, PDD, and DLB and 2) an AD subset of cases comprising controls and AD. These subset data matrices also included GIS data. Likely due to the overwhelming loss of synaptic signatures in AD dementia, we have previously observed that this disease tends to drive the module organization and alterations observed in multi-disease brain networks and mask changes present in non-tauopathies, such as LBD. Thus, building the UPenn networks separately by disease allowed us to better capture the module structure and changes associated more specifically with LB pathology. Two additional validation networks were also built on the 44 Emory and 103 ROSMAP cases. For each build, network connectivity outlier removal was performed as described [ 17 , 18 , 20 ], with any samples having a network connectivity Z-score of 3 in either direction being removed. The WGCNA::blockwiseModules() function was then used to generate each network. The UPenn and Emory LBD networks were built using the following WGCNA settings: soft threshold power = 11.0, deepSplit = 2, minimum module size = 25, merge cut height = 0.07, mean topological overlap matrix (TOM) denominator, a signed network with partitioning about medioids (PAM) respecting the dendrogram, and a reassignment threshold of p < 0.05 with clustering completed within a single block. Soft threshold power was adjusted in the UPenn AD and ROSMAP LBD networks to 12.0 and 7.5 respectively, keeping the other arguments to the blockwiseModules function consistent across the networks. This function generates a correlation matrix across all proteins within each network and subsequently clusters proteins hierarchically into modules based on protein expression pattern similarity across samples. Module eigenproteins are also generated, each representing the first principal component of the module’s hub proteins. The signedkME function of WGCNA then allowed us to determine the bicor correlation between each individual protein and each module eigenprotein. This measure of module membership is defined as kME and was ultimately utilized to determine hub status [ 52 ]. To enforce a kME table with minimal ambiguity in module assignments, a post-hoc clean-up procedure with iterative protein reassignments were performed as previously described [ 17 , 20 ].
Gene ontology and cell type marker enrichment analyses
Gene ontology (GO) annotations were retrieved from the Bader Lab’s monthly updated. GMT formatted ontology lists as previously described [ 93 ]. A Fisher’s exact test for enrichment was performed into each module’s protein membership using an in-house script. Molecular function, biological process, and cellular compartment assignments for each module were determined using the highest ranked GO terms associated with each module. Cell type enrichment was also investigated as previously described [ 17 , 20 ] by analyzing module overlap with RNA sequencing (RNA-seq) and proteomic reference lists of cell type-specific markers [ 94 , 95 ]. Fisher’s exact tests (FET) were performed to measure the extent of cell type enrichment in each module and were corrected by the Benjamini–Hochberg procedure.
GWAS module association
The enrichment of UPenn LBD modules with GWAS targets was performed using MAGMA (version 1.08b) [ 46 ] and disease-specific single nucleotide polymorphism (SNP) summary statistics, as previously described [ 17 , 20 ]. PD summary statistics were derived from http://www.pdgene.org , while AD summary statistics were obtained from Kunkle et al. [ 96 ]. These lists were filtered for those genes with disease association values of p < 0.05 prior to enrichment analyses.
Betweenness centrality calculations
To determine the bottleneck status of SNCA and other proteins in its assigned module (M19) in the UPenn LBD network, we calculated the betweenness centrality ( g ) of each of these proteins relative to related presynaptic modules, including M7, M17, and M26. Essentially, these betweenness values represent the number of shortest paths passing through a certain protein or node, and those nodes with high betweenness are responsible for the flow of information in that portion of the network [ 50 , 51 , 53 ]. These betweenness measures were calculated in NetworkX (v3.1) in Python (v3.11.5) using the topological overlap matrix (TOM) plot generated by WGCNA upon building the UPenn LBD network. A subgraph was constructed using the protein members of M19, M7, M17, and M26. Edges with weights less than the mean edge weight were removed to focus on proteins with the most similar expression patterns. The betweenness centrality was calculated for the resultant graph with protein members of M19 as source nodes and the remaining proteins in M7, M17, and M26 as target nodes. After betweenness centrality was calculated, proteins in M19 were ranked.
Network module preservation
The WGCNA::modulePreservation() function was used to assess network module preservation across networks, as previously described [ 17 , 18 , 20 ]. This function generated a Z summary composite score for each module, using one designated network as the template for each pairwise network comparison. We also assessed module preservation using synthetic eigenproteins as previously published [ 17 , 18 , 20 ]. Briefly, using one network as a template, synthetic modules were assembled in the comparison network comprising the top 20th percentile of proteins by kME. The WGCNA::moduleEigengenes() function was then used to calculate the weighted eigenproteins of these synthetic modules, representing the variance of all synthetic module members across disease cohorts.
Other statistics
Statistical analyses were performed in R (version 3.5.2). Correlations were performed using the biweight midcorrelation function as implemented in the WGCNA R package, using only pairwise complete observations for the calculations. Comparisons between two groups were performed by t test after confirmation that the data was normally distributed. Comparisons among three or more groups were performed with one-way ANOVA with Tukey or Bonferroni post-hoc correction methods for specific pairwise comparisons. P values were adjusted for multiple comparisons by false discovery rate (FDR) correction where indicated. The hinges of the box plots represent the median and the interquartile range between the 25th and 75th percentiles, while data points up to 1.5 times the interquartile range from each box hinge define the extent of error bar whiskers. Data points outside this range were identified as outliers.
Availability of data and materials
Raw mass spectrometry data from the ROSMAP dorsolateral prefrontal cortex tissues can be found at https://www.synapse.org/#!Synapse:syn53177242 . Pre- and post-processed protein expression data and case traits related to this manuscript are available at https://www.synapse.org/#!Synapse:syn53177242 . The algorithm used for batch correction is fully documented and available as an R function, which can be downloaded from https://github.com/edammer/TAMPOR . Other algorithms implemented in R for cell type marker enrichment ( https://github.com/edammer/CellTypeFET ), GO annotation ( https://github.com/edammer/GOparallel ), MAGMA gene level risk enrichment ( https://github.com/edammer/MAGMA.SPA ), and bicor or standard volcano statistic calculation and plotting ( https://github.com/edammer/parANOVA ) are also available online. The results published here are in whole or in part based on data obtained from the AMP-AD Knowledge Portal ( https://adknowledgeportal.synapse.org ). The AMP-AD Knowledge Portal is a platform for accessing data, analyses, and tools generated by the AMP-AD Target Discovery Program and other programs supported by the National Institute on Aging to enable open-science practices and accelerate translational learning. The data, analyses, and tools are shared early in the research cycle without a publication embargo on secondary use. Data are available for general research use according to the following requirements for data access and data attribution ( https://adknowledgeportal.synapse.org/#/DataAccess/Instructions ).
Jellinger KA. Dementia with Lewy bodies and Parkinson’s disease-dementia: current concepts and controversies. J Neural Transm (Vienna). 2018;125(4):615–50.
Article CAS PubMed Google Scholar
Braak H, et al. Staging of brain pathology related to sporadic Parkinson’s disease. Neurobiol Aging. 2003;24(2):197–211.
Article PubMed Google Scholar
Harding AJ, Broe GA, Halliday GM. Visual hallucinations in Lewy body disease relate to Lewy bodies in the temporal lobe. Brain. 2002;125(Pt 2):391–403.
Pezzoli S, et al. Frontal and subcortical contribution to visual hallucinations in dementia with Lewy bodies and Parkinson’s disease. Postgrad Med. 2019;131(7):509–22.
Rietdijk CD, et al. Exploring Braak’s Hypothesis of Parkinson’s Disease. Front Neurol. 2017;8:37.
Article PubMed PubMed Central Google Scholar
Schneider JA, et al. Cognitive impairment, decline and fluctuations in older community-dwelling subjects with Lewy bodies. Brain. 2012;135(Pt 10):3005–14.
Article CAS PubMed PubMed Central Google Scholar
Chia R, et al. Genome sequencing analysis identifies new loci associated with Lewy body dementia and provides insights into its genetic architecture. Nat Genet. 2021;53(3):294–303.
Guerreiro R, et al. Investigating the genetic architecture of dementia with Lewy bodies: a two-stage genome-wide association study. Lancet Neurol. 2018;17(1):64–74.
Aarsland D, et al. Cognitive decline in Parkinson disease. Nat Rev Neurol. 2017;13(4):217–31.
Jellinger KA, Attems J. Challenges of multimorbidity of the aging brain: a critical update. J Neural Transm (Vienna). 2015;122(4):505–21.
Uemura MT, et al. Distinct characteristics of limbic-predominant age-related TDP-43 encephalopathy in Lewy body disease. Acta Neuropathol. 2022;143(1):15–31.
Lemstra AW, et al. Concomitant AD pathology affects clinical manifestation and survival in dementia with Lewy bodies. J Neurol Neurosurg Psychiatry. 2017;88(2):113–8.
Scott GD, et al. Fluid and tissue biomarkers of Lewy body dementia: report of an LBDA symposium. Front Neurol. 2021;12: 805135.
Rayaprolu S, et al. Systems-based proteomics to resolve the biology of Alzheimer’s disease beyond amyloid and tau. Neuropsychopharmacology. 2021;46(1):98–115.
Higginbotham L, et al. Network analysis of a membrane-enriched brain proteome across stages of Alzheimer’s disease. Proteomes. 2019;7(3):30.
Higginbotham L, et al. Integrated proteomics reveals brain-based cerebrospinal fluid biomarkers in asymptomatic and symptomatic Alzheimer’s disease. Sci Adv. 2020;6(43):eaaz9360.
Johnson ECB, et al. Large-scale deep multi-layer analysis of Alzheimer’s disease brain reveals strong proteomic disease-related changes not observed at the RNA level. Nat Neurosci. 2022;25(2):213–25.
Johnson ECB, et al. Large-scale proteomic analysis of Alzheimer’s disease brain and cerebrospinal fluid reveals early changes in energy metabolism associated with microglia and astrocyte activation. Nat Med. 2020;26:769–80.
Johnson ECB, et al. Deep proteomic network analysis of Alzheimer’s disease brain reveals alterations in RNA binding proteins and RNA splicing associated with disease. Mol Neurodegener. 2018;13(1):52.
Seyfried NT, et al. A multi-network approach identifies protein-specific co-expression in asymptomatic and symptomatic Alzheimer’s disease. Cell Syst. 2017;4(1):60–72 e4.
Swarup V, et al. Identification of conserved proteomic networks in neurodegenerative dementia. Cell Rep. 2020;31(12): 107807.
Wingo AP, et al. Shared proteomic effects of cerebral atherosclerosis and Alzheimer’s disease on the human brain. Nat Neurosci. 2020;23(6):696–700.
Haque R, et al. A protein panel in cerebrospinal fluid for diagnostic and predictive assessment of Alzheimer’s disease. Sci Transl Med. 2023;15(712):eadg4122.
Johnson ECB, et al. Cerebrospinal fluid proteomics define the natural history of autosomal dominant Alzheimer’s disease. Nat Med. 2023;29(8):1979–88.
McKeith IG, et al. Diagnosis and management of dementia with Lewy bodies: third report of the DLB Consortium. Neurology. 2005;65(12):1863–72.
McKeith IG, et al. Diagnosis and management of dementia with Lewy bodies: Fourth consensus report of the DLB Consortium. Neurology. 2017;89(1):88–100.
Dammer EB, Seyfried NT, Johnson ECB. Batch correction and harmonization of -omics datasets with a tunable median polish of ratio. Front Syst Biol. 2023;3:1092341.
Braak H, Braak E. Neuropathological stageing of Alzheimer-related changes. Acta Neuropathol. 1991;82(4):239–59.
Mirra SS, et al. The Consortium to Establish a Registry for Alzheimer’s Disease (CERAD). Part II. Standardization of the neuropathologic assessment of Alzheimer’s disease. Neurology. 1991;41(4):479–86.
Sturm RM, Lietz CB, Li L. Improved isobaric tandem mass tag quantification by ion mobility mass spectrometry. Rapid Commun Mass Spectrom. 2014;28(9):1051–60.
Tubi MA, et al. Regional relationships between CSF VEGF levels and Alzheimer’s disease brain biomarkers and cognition. Neurobiol Aging. 2021;105:241–51.
Cao L, et al. VEGF links hippocampal activity with neurogenesis, learning and memory. Nat Genet. 2004;36(8):827–35.
Fournier NM, et al. Vascular endothelial growth factor regulates adult hippocampal cell proliferation through MEK/ERK- and PI3K/Akt-dependent signaling. Neuropharmacology. 2012;63(4):642–52.
Licht T, et al. Reversible modulations of neuronal plasticity by VEGF. Proc Natl Acad Sci U S A. 2011;108(12):5081–6.
Zacchigna S, Lambrechts D, Carmeliet P. Neurovascular signalling defects in neurodegeneration. Nat Rev Neurosci. 2008;9(3):169–81.
Gora-Kupilas K, Josko J. The neuroprotective function of vascular endothelial growth factor (VEGF). Folia Neuropathol. 2005;43(1):31–9.
CAS PubMed Google Scholar
Chang MC, et al. Narp regulates homeostatic scaling of excitatory synapses on parvalbumin-expressing interneurons. Nat Neurosci. 2010;13(9):1090–7.
Lee SJ, et al. Presynaptic Neuronal Pentraxin Receptor Organizes Excitatory and Inhibitory Synapses. J Neurosci. 2017;37(5):1062–80.
Article CAS PubMed Central Google Scholar
Xiao MF, et al. NPTX2 and cognitive dysfunction in Alzheimer’s Disease. Elife. 2017;6:e23798.
Hurst C, et al. Integrated Proteomics Identifies Neuritin (NRN1) as a mediator of cognitive resilience to Alzheimer’s disease. bioRxiv. 2022:2022.06.15.496285.
Yu L, et al. Cortical proteins associated with cognitive resilience in community-dwelling older persons. JAMA Psychiat. 2020;77(11):1172–80.
Article Google Scholar
Black SA, Rylett RJ. Choline transporter CHT regulation and function in cholinergic neurons. Cent Nerv Syst Agents Med Chem. 2012;12(2):114–21.
Payette DJ, Xie J, Guo Q. Reduction in CHT1-mediated choline uptake in primary neurons from presenilin-1 M146V mutant knock-in mice. Brain Res. 2007;1135(1):12–21.
Petrasek T, et al. A rat model of Alzheimer’s disease based on Abeta42 and pro-oxidative substances exhibits cognitive deficit and alterations in glutamatergic and cholinergic neurotransmitter systems. Front Aging Neurosci. 2016;8:83.
Villar-Pique A, Lopes da Fonseca T, Outeiro TF. Structure, function and toxicity of alpha-synuclein: the Bermuda triangle in synucleinopathies. J Neurochem. 2016;139 Suppl 1:240–55.
de Leeuw CA, et al. MAGMA: generalized gene-set analysis of GWAS data. PLoS Comput Biol. 2015;11(4): e1004219.
Geiger JT, et al. Next-generation sequencing reveals substantial genetic contribution to dementia with Lewy bodies. Neurobiol Dis. 2016;94:55–62.
Tsuang D, et al. APOE epsilon4 increases risk for dementia in pure synucleinopathies. JAMA Neurol. 2013;70(2):223–8.
Wingo TS, et al. Shared mechanisms across the major psychiatric and neurodegenerative diseases. Nat Commun. 2022;13(1):4314.
Nithya C, Kiran M, Nagarajaram HA. Dissection of hubs and bottlenecks in a protein-protein interaction network. Comput Biol Chem. 2023;102: 107802.
Joy MP, et al. High-betweenness proteins in the yeast protein interaction network. J Biomed Biotechnol. 2005;2005(2):96–103.
PubMed PubMed Central Google Scholar
Langfelder P, Horvath S. WGCNA: an R package for weighted correlation network analysis. BMC Bioinformatics. 2008;9:559.
Brandes U. On variants of shortest-path betweenness centrality and their generic computation. Social Networks. 2008;30(2):136–45.
Hayashi J, Carver JA. beta-Synuclein: An Enigmatic Protein with Diverse Functionality. Biomolecules. 2022;12(1):142.
Betzer C, et al. Identification of synaptosomal proteins binding to monomeric and oligomeric alpha-synuclein. PLoS ONE. 2015;10(2): e0116473.
Jiang C, et al. Serum neuronal exosomes predict and differentiate Parkinson’s disease from atypical parkinsonism. J Neurol Neurosurg Psychiatry. 2020;91(7):720–9.
Nila IS, et al. Identification of exosomal biomarkers and its optimal isolation and detection method for the diagnosis of Parkinson’s disease: A systematic review and meta-analysis. Ageing Res Rev. 2022;82: 101764.
Cohen S, Valm AM, Lippincott-Schwartz J. Interacting organelles. Curr Opin Cell Biol. 2018;53:84–91.
Deng C, et al. Dynamic remodeling of ribosomes and endoplasmic reticulum in axon terminals of motoneurons. J Cell Sci. 2021;134(22):jcs258785.
Wu Y, et al. Contacts between the endoplasmic reticulum and other membranes in neurons. Proc Natl Acad Sci U S A. 2017;114(24):E4859–67.
Bennett DA, et al. The Rush Memory and Aging Project: study design and baseline characteristics of the study cohort. Neuroepidemiology. 2005;25(4):163–75.
Bennett DA, Yu L, De Jager PL. Building a pipeline to discover and validate novel therapeutic targets and lead compounds for Alzheimer’s disease. Biochem Pharmacol. 2014;88(4):617–30.
Bennett DA, et al. Religious orders study and rush memory and aging project. J Alzheimers Dis. 2018;64(s1):S161–89.
Higginbotham L, et al. Unbiased classification of the elderly human brain proteome resolves distinct clinical and pathophysiological subtypes of cognitive impairment. Neurobiol Dis. 2023;186: 106286.
Jack CR Jr, et al. NIA-AA Research Framework: Toward a biological definition of Alzheimer’s disease. Alzheimers Dement. 2018;14(4):535–62.
Aynacioglu AS, Bilir A, Tuna MY. Involvement of midkine in autoimmune and autoinflammatory diseases. Mod Rheumatol. 2019;29(4):567–71.
Qin S, et al. CTHRC1 promotes wound repair by increasing M2 macrophages via regulating the TGF-beta and notch pathways. Biomed Pharmacother. 2019;113: 108594.
de Wilde MC, et al. Meta-analysis of synaptic pathology in Alzheimer’s disease reveals selective molecular vesicular machinery vulnerability. Alzheimers Dement. 2016;12(6):633–44.
DeKosky ST, Scheff SW, Styren SD. Structural correlates of cognition in dementia: quantification and assessment of synapse change. Neurodegeneration. 1996;5(4):417–21.
Scheff SW, Price DA. Alzheimer’s disease-related alterations in synaptic density: neocortex and hippocampus. J Alzheimers Dis. 2006;9(3 Suppl):101–15.
Terry RD, et al. Physical basis of cognitive alterations in Alzheimer’s disease: synapse loss is the major correlate of cognitive impairment. Ann Neurol. 1991;30(4):572–80.
Bai B, et al. Deep multilayer brain proteomics identifies molecular networks in Alzheimer’s disease progression. Neuron. 2020;105(6):975–991 e7.
Parikshak NN, Gandal MJ, Geschwind DH. Systems biology and gene networks in neurodevelopmental and neurodegenerative disorders. Nat Rev Genet. 2015;16(8):441–58.
Ozgur A, et al. Identifying gene-disease associations using centrality on a literature mined gene-interaction network. Bioinformatics. 2008;24(13):i277–85.
Yao L, Rzhetsky A. Quantitative systems-level determinants of human genes targeted by successful drugs. Genome Res. 2008;18(2):206–13.
Fu Y, et al. Exploring the relationship between hub proteins and drug targets based on GO and intrinsic disorder. Comput Biol Chem. 2015;56:41–8.
Atias M, et al. Synapsins regulate alpha-synuclein functions. Proc Natl Acad Sci U S A. 2019;116(23):11116–8.
Pahwa M, et al. New Antipsychotic Medications in the Last Decade. Curr Psychiatry Rep. 2021;23(12):87.
Thorn CA, et al. Effects of M1 and M4 activation on excitatory synaptic transmission in CA1. Hippocampus. 2017;27(7):794–810.
Colla E, et al. Accumulation of toxic alpha-synuclein oligomer within endoplasmic reticulum occurs in alpha-synucleinopathy in vivo. J Neurosci. 2012;32(10):3301–5.
Hoozemans JJ, et al. Activation of the unfolded protein response in Parkinson’s disease. Biochem Biophys Res Commun. 2007;354(3):707–11.
Makioka K, et al. Involvement of endoplasmic reticulum stress defined by activated unfolded protein response in multiple system atrophy. J Neurol Sci. 2010;297(1–2):60–5.
Choo YS, et al. Regulation of parkin and PINK1 by neddylation. Hum Mol Genet. 2012;21(11):2514–23.
Mori F, et al. Accumulation of NEDD8 in neuronal and glial inclusions of neurodegenerative disorders. Neuropathol Appl Neurobiol. 2005;31(1):53–61.
Dil Kuazi A, et al. NEDD8 protein is involved in ubiquitinated inclusion bodies. J Pathol. 2003;199(2):259–66.
Ferman TJ, et al. Subtypes of dementia with Lewy bodies are associated with alpha-synuclein and tau distribution. Neurology. 2020;95(2):e155–65.
Chatterjee A, et al. Clinico-pathological comparison of patients with autopsy-confirmed Alzheimer’s disease, dementia with Lewy bodies, and mixed pathology. Alzheimers Dement (Amst). 2021;13(1): e12189.
Watson CM, et al. Quantitative Mass Spectrometry Analysis of Cerebrospinal Fluid Protein Biomarkers in Alzheimer’s Disease. Sci Data. 2023;10(1):261.
Bonanni L, Thomas A, Onofrj M. Diagnosis and management of dementia with Lewy bodies: third report of the DLB Consortium. Neurology. 2006;66(9):1455; author reply 1455.
Ping L, et al. Global quantitative analysis of the human brain proteome in Alzheimer’s and Parkinson’s Disease. Sci Data. 2018;5: 180036.
Ping L, et al. Global quantitative analysis of the human brain proteome and phosphoproteome in Alzheimer’s disease. Sci Data. 2020;7(1):315.
Mertins P, et al. Reproducible workflow for multiplexed deep-scale proteome and phosphoproteome analysis of tumor tissues by liquid chromatography-mass spectrometry. Nat Protoc. 2018;13(7):1632–61.
Modeste ES, et al. Quantitative proteomics of cerebrospinal fluid from African Americans and Caucasians reveals shared and divergent changes in Alzheimer’s disease. Mol Neurodegener. 2023;18(1):48.
Sharma K, et al. Cell type- and brain region-resolved mouse brain proteome. Nat Neurosci. 2015;18(12):1819–31.
Zhang Y, et al. An RNA-sequencing transcriptome and splicing database of glia, neurons, and vascular cells of the cerebral cortex. J Neurosci. 2014;34(36):11929–47.
Kunkle BW, et al. Genetic meta-analysis of diagnosed Alzheimer’s disease identifies new risk loci and implicates Abeta, tau, immunity and lipid processing. Nat Genet. 2019;51(3):414–30.
Download references
Acknowledgements
We are grateful to those who agreed to donate their brains for research and who participated in the described observational studies.
This study was supported by the following National Institutes of Health funding mechanisms: K23NS119964-01 (L.H.), R21NS123882-01 (L.H.), U01AG061357 (N.T.S.) and 1U01NS128433-01 (N.T.S. and A.I.L.). Procurement of UPenn tissues was supported by the following grants: P30AG072979, P01AG066597, U19AG062418 (E.B.L.).
Author information
Authors and affiliations.
Center for Neurodegenerative Disease, Emory University School of Medicine, Atlanta, GA, USA
Anantharaman Shantaraman, Eric B. Dammer, Obiadada Ugochukwu, Duc M. Duong, E. Kathleen Carter, Marla Gearing, James J. Lah, Allan I. Levey, Nicholas T. Seyfried & Lenora Higginbotham
Department of Biochemistry, Emory University School of Medicine, Atlanta, GA, USA
Anantharaman Shantaraman, Eric B. Dammer, Duc M. Duong, Luming Yin, E. Kathleen Carter & Nicholas T. Seyfried
Department of Neurology, Emory University School of Medicine, Atlanta, GA, USA
E. Kathleen Carter, Marla Gearing, James J. Lah, Allan I. Levey, Nicholas T. Seyfried & Lenora Higginbotham
Department of Pathology and Laboratory Medicine, Emory University School of Medicine, Atlanta, GA, USA
Marla Gearing
Department of Neurology, Perelman School of Medicine at the University of Pennsylvania, Philadelphia, PA, USA
Alice Chen-Plotkin
Department of Pathology and Laboratory Medicine, Perelman School of Medicine at the University of Pennsylvania, Philadelphia, PA, USA
Edward B. Lee & John Q. Trojanowski
Rush Alzheimer’s Disease Center, Rush University Medical Center, Chicago, IL, USA
David A. Bennett
You can also search for this author in PubMed Google Scholar
Contributions
Conceptualization, A.S., A.I.L., N.T.S., and L.H.; Methodology, A.S., E.B.D., D.M.D., N.T.S., and L.H.; Investigation, D.M.D., L.Y., and L.H.; Formal Analysis, A.S., E.B.D., O.U., E.K.C., and L.H.; Writing – Original Draft, A.S. and L.H..; Writing – Review & Editing, A.S., E.B.D., E.K.C., D.M.D., J.J.L., A.I.L., N.T.S., and L.H.; Funding Acquisition, A.I.L., N.T.S., and L.H.; Resources, M.G., A.C.P., E.B.L., D.A.B.; Supervision, A.I.L., N.T.S., and L.H.
Corresponding authors
Correspondence to Nicholas T. Seyfried or Lenora Higginbotham .
Ethics declarations
Ethics approval and consent to participate.
All human postmortem brain tissues analyzed in this study were acquired under Institutional Review Board protocols at each representative institution. All ROSMAP participants signed informed and repository consents and an Anatomic Gift Act.
Consent for publication
Not applicable.
Competing interests
A.I.L, N.T.S., and D.M.D. are co-founders of Emtherapro Inc. The authors declare no conflicts of interest.
Additional information
Publisher’s note.
Springer Nature remains neutral with regard to jurisdictional claims in published maps and institutional affiliations.
Supplementary Information
13024_2024_749_moesm1_esm.pdf.
Supplementary Material 1: Figure S1. TAMPOR and covariate regression of UPenn TMT-MS dataset.Multidimensional scaling plot displaying case distribution based on inter-sample variance pre- and post-TAMPOR normalization between batches in the UPenn TMT-MS dataset. This batch correction was performed across all 354 cases and 24 batches in the original UPenn cohort prior to LB subset analyses.Variance partition plots for the UPenn TMT-MS dataset pre- and post-TAMPOR and covariate regression, demonstrating that protein abundance variance due to age, sex, and post-mortem interval was effectively minimized in favor of highlighting alterations driven by group, i.e. disease diagnosis. Abbreviations: TAMPOR, tunable median polish of ratio; PMI, post-mortem interval.
13024_2024_749_MOESM2_ESM.pdf
Supplementary Material 2: Figure S2. Module overlap between UPenn and Emory co-expression networks. A hypergeometric Fisher’s exact test was used to determine which modules shared significant overlap of protein members between the UPenn and Emory networks. The 33 modules in the UPenn network (y-axis) were aligned to the 39 modules in the Emory network (x-axis). Numbers indicate the -log10 p -value with red shading indicating the degree of significance of overlap (*, p < 0.05; **, p < 0.01; ***, p < 0.001). The green box indicates those UPenn modules that overlapped most strongly with E-24, which stood out in the Emory network given its association with synaptic ontologies, robust elevations in LBD, and selectively positive correlation to LB deposition relative to amyloid and tau. Its overlapping UPenn modules included P-M17 and P-M26, which were also SNCA-associated synaptic modules with strong increases in LBD.
13024_2024_749_MOESM3_ESM.pdf
Supplementary Material 3: Figure S3. LBD-associated network alterations are replicated in a ROSMAP tissue cohort. (A) Module preservation analysis of UPenn network into the ROSMAP network. Modules with a Z summary score of greater than or equal to 1.96 ( q = 0.05, blue dotted line) were considered preserved, while modules with Z summary scores of greater than or equal to 10 ( q = 1.0E-23, red dotted line) were considered highly preserved. (B) Select UPenn LBD network module eigenproteins associated with their corresponding synthetic eigenproteins in the ROSMAP network. The ROSMAP synthetic eigenproteins reflected the weighted module abundance of the top 20% of proteins by kME comprising each LBD module. ANOVA p values are provided for each eigenprotein plot. Box plots represent the median and 25th and 75th percentiles, while data points up to 1.5 times the interquartile range from the box hinge define the extent of error bar whiskers. Abbreviations: CTL, control; PD, Parkinson’s disease; PDD, Parkinson’s disease dementia; DLB, Dementia with Lewy bodies; AsymLB, Asymptomatic Lewy body pathology; LBD, Lewy body dementia.
13024_2024_749_MOESM4_ESM.xlsx
Supplementary Material 4: Table S1. UPenn cohort demographics and clinicopathological traits. Table S2. Summary of UPenn cohort demographics and clinicopathological traits. Table S3. Differential protein abundance across UPenn LBD cases. Table S4. UPenn LBD network module assignments. Table S5. UPenn LBD module correlations to traits. Table S6. Gene ontology z-scores for UPenn LBD network modules. Table S7. PD GWAS MAGMA in UPenn LBD network. Table S8. AD GWAS MAGMA in UPenn LBD network. Table S9. Bottleneck rankings for M19 proteins among UPenn presynaptic modules. Table S10. UPenn Protein correlations to synuclein (SNCA) abundance. Table S11. Emory cohort demographics and clinicopathological traits. Table S12. Summary of Emory cohort demographics and clinicopathological traits. Table S13. Emory LBD network module assignments. Table S14. Emory LBD module correlations to traits. Table S15. Gene ontology z-scores for Emory LBD network modules. Table S16. ROSMAP cohort demographics and clinicopathological traits. Table S17. Summary of ROSMAP cohort demographics and clinicopathological traits. Table S18. Differential protein abundance across high- and low-amyloid UPenn LBD cases.
Rights and permissions
Open Access This article is licensed under a Creative Commons Attribution 4.0 International License, which permits use, sharing, adaptation, distribution and reproduction in any medium or format, as long as you give appropriate credit to the original author(s) and the source, provide a link to the Creative Commons licence, and indicate if changes were made. The images or other third party material in this article are included in the article's Creative Commons licence, unless indicated otherwise in a credit line to the material. If material is not included in the article's Creative Commons licence and your intended use is not permitted by statutory regulation or exceeds the permitted use, you will need to obtain permission directly from the copyright holder. To view a copy of this licence, visit http://creativecommons.org/licenses/by/4.0/ . The Creative Commons Public Domain Dedication waiver ( http://creativecommons.org/publicdomain/zero/1.0/ ) applies to the data made available in this article, unless otherwise stated in a credit line to the data.
Reprints and permissions
About this article
Cite this article.
Shantaraman, A., Dammer, E.B., Ugochukwu, O. et al. Network proteomics of the Lewy body dementia brain reveals presynaptic signatures distinct from Alzheimer’s disease. Mol Neurodegeneration 19 , 60 (2024). https://doi.org/10.1186/s13024-024-00749-1
Download citation
Received : 26 January 2024
Accepted : 24 July 2024
Published : 06 August 2024
DOI : https://doi.org/10.1186/s13024-024-00749-1
Share this article
Anyone you share the following link with will be able to read this content:
Sorry, a shareable link is not currently available for this article.
Provided by the Springer Nature SharedIt content-sharing initiative
- Lewy body dementia
- Mass spectrometry
Molecular Neurodegeneration
ISSN: 1750-1326
- Submission enquiries: Access here and click Contact Us
- General enquiries: [email protected]

IMAGES
COMMENTS
Vascular dementia is among the most common etiologies of major neurocognitive disorder (MND), affecting primarily older adults (>65), and it is the leading nondegenerative cause of dementia. The Diagnostic and Statistical Manual of Mental Disorders, Fifth Edition (DSM-V) subsumes all dementing diseases under the syndromic term MND. MND requires an acquired decline in one or more cognitive ...
Vascular dementia refers to any dementia that is primarily caused by cerebrovascular disease or impaired cerebral blood flow and falls within the spectrum of vascular cognitive impairment (VCI), a syndrome that includes all cognitive disorders in which cerebrovascular disease or impaired cerebral blood flow is a contributing causative factor.
Vascular dementia can also result from other conditions that damage blood vessels and reduce circulation, depriving your brain of vital oxygen and nutrients. Factors that increase your risk of heart disease and stroke — including diabetes, high blood pressure, high cholesterol and smoking — also raise your vascular dementia risk.
Patients with vascular dementia commonly have mood and behavioral changes. In some patients with lacunar state and Binswanger disease, such problems may be more prominent than intellectual deficits. Executive functioning deficits are seen prior to severe memory loss in the early stages of subcortical vascular cognitive impairment.
Cerebrovascular and cardiovascular diseases cause vascular brain injury that can lead to vascular cognitive impairment (VCI). VCI is the second most common neuropathology of dementia and mild cognitive impairment (MCI), accounting for up to one-third of the population risk. It is frequently present …
Vascular dementia - learn about symptoms, diagnosis, causes and treatments and how this disorder relates to Alzheimer's and other dementias.
Vascular Dementia: Causes, Symptoms, and Treatments Vascular dementia refers to changes to memory, thinking, and behavior resulting from conditions that affect the blood vessels in the brain. Cognition and brain function can be significantly affected by the size, location, and number of vascular changes.
Major depression is a widely observed mood disorder in vascular dementia. Patients with vascular dementia commonly have mood and behavioral changes. In some patients with lacunar state and Binswanger disease, such problems may be more prominent than intellectual deficits. Executive functioning deficits are seen prior to severe memory loss in ...
Vascular dementia is the second most common cause of dementia in older people. A large overlap exists with Alzheimer's dementia and many patients have a mixed form of dementia. Early aggressive treatment of vascular risk factors is suggested in order to prevent further cerebrovascular disease.
Vascular dementia is a disorder that damages brain tissue because of a lack of blood flow. Causes can include blood clots, ruptured blood vessels, or narrowing or hardening of blood vessels that supply the brain. Symptoms can include problems with memory and focus, confusion, changes in personality and behavior, loss of speech and language ...
Diagnosis Doctors can nearly always determine that you have dementia, but there's no specific test that confirms you have vascular dementia. Your doctor will make a judgment about whether vascular dementia is the most likely cause of your symptoms based on the information you provide, your medical history for stroke or disorders of the heart and blood vessels, and results of tests that may ...
Vascular dementia (VaD) is the most common cause of dementia in the elderly, second only to Alzheimer's disease (AD). Between 1% and 4% of people of 65 years of age suffer from VaD and the prevalence appears to double every 5-10 years ...
Vascular dementia is dementia caused by a series of strokes. [2] [4] Restricted blood flow due to strokes reduces oxygen and glucose delivery to the brain, causing cell injury and neurological deficits in the affected region. [6] Subtypes of vascular dementia include subcortical vascular dementia, multi-infarct dementia, stroke-related dementia ...
Vascular dementia, also known as vascular cognitive impairment, is the second most common cause of dementia after the far more common Alzheimer disease. It is primarily seen in patients with atherosclerosis and chronic hypertension and results fr...
If it's vascular dementia, certain lifestyle changes can help prevent further damage. WebMD takes a look at vascular dementia, its causes, symptoms, and prognosis.
Vascular dementia is one of the most common causes of dementia after Alzheimer's disease, causing around 15% of cases. However, unlike Alzheimer's disease, there are no licensed treatments for vascular dementia. Progress in the specialty has been difficult because of uncertainties over disease classification and diagnostic criteria, controversy over the exact nature of the relation between ...
Signs and symptoms Vascular dementia is a heterogeneous entity with a large clinicopathological spectrum that has been classically linked to cortical and subcortical ischemic changes resulting from systemic, cardiac, or local large- or small-vessel disease occlusion. Symptoms of vascular dementia include the following: Memory impairment Impa...
Vascular dementia is a common type of dementia caused by reduced blood flow to the brain. It's estimated to affect around 180,000 people in the UK. Dementia is the name for problems with mental abilities caused by gradual changes and damage in the brain. It's rare in people under 65.
Subcortical vascular dementia Subcortical vascular dementia is thought to be the most common type of vascular dementia. It is caused by diseases of the very small blood vessels that lie deep in the brain (known as 'small vessel disease'). Over time, these blood vessels can develop thick walls and become stiff and twisted, so blood cannot travel through them easily. The parts of the brain ...
Vascular dementia is caused by a range of conditions that disrupt blood flow to the brain and affect memory, thinking, and behavior. Learn about the causes of vascular dementia and modifiable risk factors.
Alzheimer's disease and vascular dementia (sometimes called vascular cognitive impairment or vascular neurocognitive disorder) are both types of dementia. They have several symptoms and characteristics that overlap, but there are also some clear differences between the two.
Vascular Dementia. Presented By Liz Graham Brightwater Care Group. Objectives. To increase knowledge and understanding of Vascular Dementia To demonstrate the progression of Vascular Dementia To discuss the importance of team management of the person with Vascular Dementia.
The trials excluded those who had evidence of co-neuropathologies (e.g., significant vascular pathology on MRI scan) ... and AD 85. 32 Only part of this age gap can be explained by delays in presentation or diagnosis, ... If a person's dementia is also determined by neuropathologies other than amyloid, ...
Lewy body dementia (LBD), a class of disorders comprising Parkinson's disease dementia (PDD) and dementia with Lewy bodies (DLB), features substantial clinical and pathological overlap with Alzheimer's disease (AD). The identification of biomarkers unique to LBD pathophysiology could meaningfully advance its diagnosis, monitoring, and treatment.