Smart Water releases new software updates for both the WiFi LCD Keypad and iOS/Android apps!
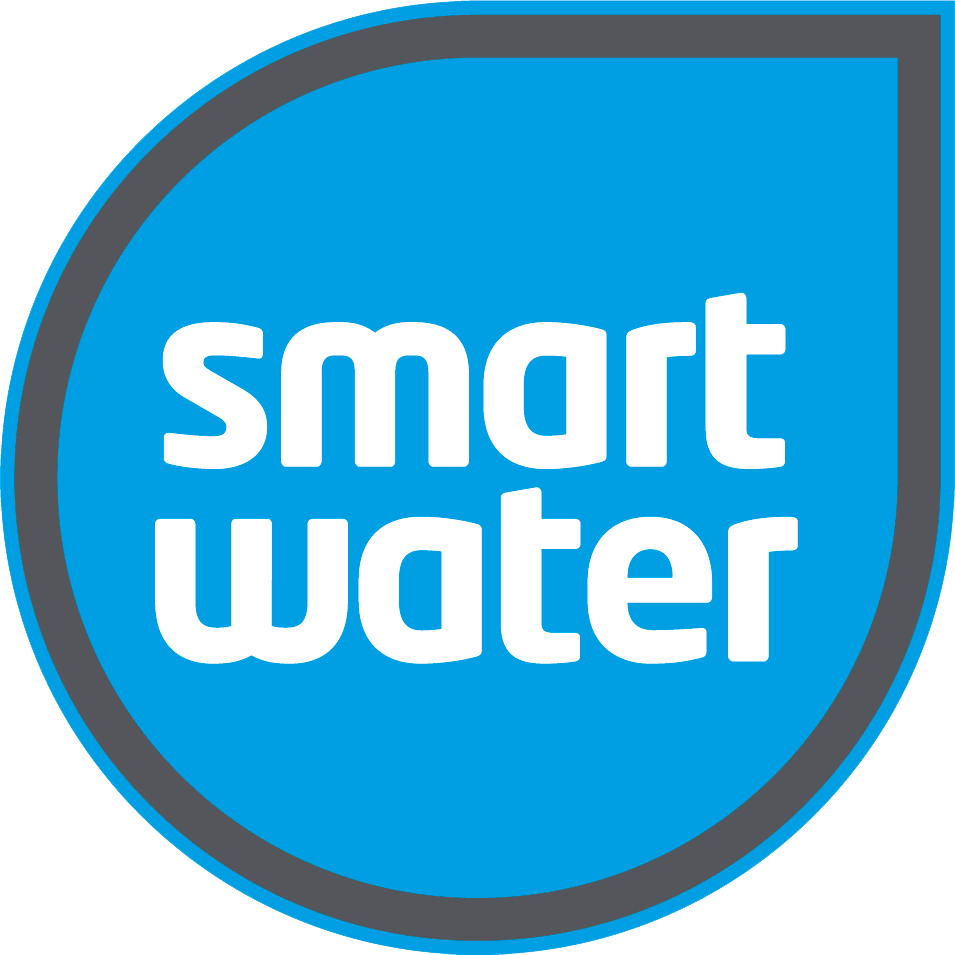

Rainwater as a solution to water scarcity: case studies from around the world
08 September 2024
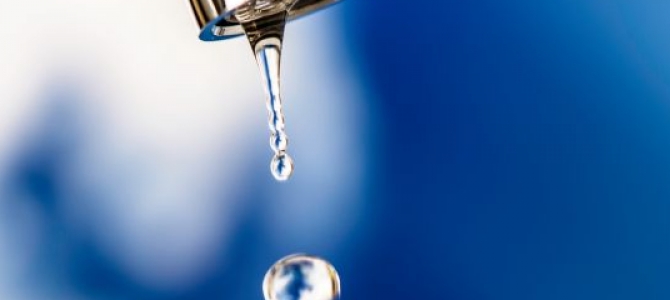
Water scarcity has emerged as a global concern, with the United Nations projecting that by 2030, nearly half of the global population could be grappling with water shortages.
One potential solution to this pressing issue is the use of rainwater.
This blog will explore various case studies from around the world, illustrating how rainwater harvesting has been successfully implemented as a sustainable countermeasure to water scarcity.
Overview of Water Scarcity Around the World
Water scarcity is a pressing issue that affects every continent on the globe. According to the World Wildlife Fund (WWF) , approximately 1.1 billion people worldwide lack access to water, and a total of 2.7 billion find water scarce for at least one month of the year.
In Africa alone, more than a quarter of the population faces water shortages, as detailed in a report by the United Nations Environment Program (UNEP) . Similarly, Asia is home to almost half of the world's poorest people who are living without access to clean water, as indicated by Water.org .
These alarming figures underscore the urgency of finding sustainable solutions to address water scarcity, rainwater harvesting being one of the most promising among them.
Here in New Zealand, water scarcity has become an alarming issue, largely due to climate change and population growth. Particularly in the North Island, water restrictions are frequently imposed during summer. The New Zealand Government has recognised this issue and is currently working on an extensive freshwater reform to address it.
Australia has long grappled with water shortages, primarily as a result of its arid climate, irregular rainfall, and high evaporation rates. The Murray-Darling Basin, Australia's primary agricultural region, is especially vulnerable to water scarcity. The Australian Government's Bureau of Meteorology provides extensive data on water availability, usage, and restrictions.
In the USA , water scarcity is also a significant issue, particularly in the Western states such as California and Arizona. California has experienced severe droughts over the last decade, leading to strict water conservation measures. The U.S. Geological Survey provides comprehensive information on water use and the challenges of water scarcity across the country.
Benefits of Rainwater Harvesting
Rainwater harvesting offers substantial benefits, both domestically and commercially, especially in regions experiencing water scarcity . At a domestic level, rainwater harvesting allows households to become less reliant on municipal water supplies and can provide a sustainable secondary water source for gardening, washing cars, flushing toilets, and even laundry with proper treatment. Moreover, it reduces water bills and the strain on shared water resources.
Commercially, rainwater harvesting can be a game-changer. Industries such as agriculture , which require significant amounts of water, can substantially reduce their operational costs and reliance on external water supplies. Moreover, properties like hotels and office buildings can use harvested rainwater for landscaping and sanitation purposes, promoting sustainability while reducing overheads.
By augmenting water supply, rainwater harvesting can substantially alleviate the impact of water scarcity in hard-hit areas. For instance, in drought-stricken regions, harvested rainwater can supplement the water supply, thereby easing the demand pressure on shared resources.
Additionally, rainwater harvesting aids in groundwater recharge, reduces soil erosion, and mitigates the impact of flash floods by slowing run-off rates. Consequently, rainwater harvesting isn't just a temporary solution, but a long-term strategy for managing water scarcity.
Case Studies from India, South Africa, Mexico, Australia, New Zealand, and the USA
There are many examples from around the world of the success of rainwater harvesting systems . Below, you can find a snapshot of some of the most successful case studies from various countries worldwide.
Case study: India - The success of the ancient art of rainwater harvesting
In India, rainwater harvesting has been an age-old practice, especially in the arid regions of Rajasthan. A shining example of rainwater conservation is the city of Jaipur , where traditional water structures have been revived to combat water scarcity.
Case study: South Africa - Greywater recycling and rainwater harvesting combination
South Africa, particularly Cape Town, faced severe water scarcity in 2018, leading to the promotion of rainwater harvesting. A successful initiative is the implementation of Greywater Recycling Systems alongside rainwater harvesting in homes, reducing dependence on the municipal water supply.
Case study: Mexico - Rainwater harvesting in Mexico City
In Mexico City, one of the world's largest and most densely populated cities, rainwater harvesting has been implemented to address water scarcity. A notable initiative is the community-led Isla Urbana project , which has been installing rainwater harvesting systems in households across the city.
Case study: Australia - Mandating rainwater harvesting in new homes
In Australia, rainwater harvesting is encouraged on a large scale . The state of Queensland mandates the installation of rainwater tanks in all new homes, resulting in significant water savings. The success story of Toowoomba , a city that adopted rainwater harvesting to overcome a prolonged drought, is a testament to the effectiveness of this approach.
Case study: New Zealand - Waiheke Island Rainwater Collection
In New Zealand, Waiheke Island demonstrates an inspiring example of rainwater harvesting. Due to its reliance on tank water, the majority of properties on the island have rainwater collection systems. The Waiheke Resources Trust promotes this practice through educational initiatives explaining the benefits of rainwater collection. The trust also provides guidance on the installation and maintenance of these systems, ensuring sustainable water management on the island.
Case study: USA - Rainwater Harvesting in Tucson, Arizona
The city of Tucson in Arizona, USA, known for its arid climate, has made notable strides in the implementation of rainwater harvesting. The Tucson Rainwater Harvesting Rebates Program offers financial incentives for residents and businesses to install rainwater harvesting systems. This initiative has led to the widespread adoption of rainwater harvesting methodologies, providing a solution to water scarcity and contributing to the conservation of the region's water resources.
Climate Change, Weather Patterns, and Expected El Niño in New Zealand
Climate change is causing dramatic alterations to weather patterns worldwide. The warming of the planet leads to more extreme weather events, such as intense storms, heat waves, and prolonged periods of drought.
In the context of New Zealand, these changes are anticipated to culminate in an El Niño climate shift this year. El Niño is a climate pattern characterised by the warming of ocean surface temperatures in the central and eastern tropical Pacific, which can significantly influence weather systems.
For New Zealand, this could mean the emergence of drier conditions on the east coast and more rainfall on the west coast.
This climate shift further emphasises the importance of sustainable water management practices, such as rainwater harvesting, to ensure the resilience of water resources in the face of changing weather patterns.
The Need for Regulations to Support Rainwater Collection
While rainwater harvesting is a practical and sustainable solution to water scarcity, it is still not widely regulated or supported in many regions. According to the Water Research Foundation , different countries and states have disparate laws regarding the collection and usage of rainwater, which can inhibit the widespread adoption of this method.
In the UK, for instance, the Rainwater Harvesting Association works towards creating a framework that fosters the implementation of rainwater harvesting systems. However, these efforts may still be hindered by the lack of a cohesive, nationwide policy.
In the United States, the situation is even more varied. Some states, like Colorado, have historically had laws that restrict rainwater collection , whereas states like Arizona (as mentioned earlier in this post) actively encourage it with rebate programs. The National Conference of State Legislatures provides an overview of these diverse regulations.
This lack of uniformity in regulations could lead to confusion and hesitance about committing to rainwater harvesting. Hence, the establishment of clear and supportive laws on a global scale is crucial for promoting the adoption of this water-saving practice and ensuring the sustainable use of our planet's resources.
How to Implement a Rainwater Harvesting System at Home
Implementing a rainwater harvesting system at home could be a pivotal step towards addressing water scarcity issues. Utilising rainwater tanks, homeowners can collect, store, and use rainwater for various non-potable tasks such as watering plants, washing cars, or even flushing toilets. This not only reduces the demand on our increasingly strained water supplies but also cuts down on household water bills.
Installing a rainwater tank may seem daunting, but the process is rather straightforward. Tanks can range in size from small barrels to larger, industrial-sized tanks and can fit into a variety of spaces - from backyards to rooftops. Once installed, a system of gutters and pipes directs rainwater into the tank. The water can then be treated, if necessary, and used as needed.
While individual home rainwater tanks might seem like a drop in the ocean, it is important to remember that every small step contributes towards a larger solution. If more households adopt such practices, the cumulative effect could significantly alleviate the pressure on municipal water supplies.
Remember, every sustainable action counts. By introducing a rainwater harvesting system into your home, you are making a positive impact on our planet's water resources.
The Future of Rainwater Harvesting as a Solution to Global Water Scarcity
As we look forward, rainwater harvesting has the potential to play a crucial role in combating global water scarcity. Already, we see countries like Australia and Germany leading the way with extensive rainwater harvesting infrastructures. In these nations, rainwater management is not just an individual responsibility but is embedded in urban planning and building codes, making it a collective effort.
In the future, with the advent of advanced technologies and increased awareness about water conservation, we can expect to see more sustainable and efficient rainwater harvesting systems. Developments like smart rainwater tanks that monitor water levels and usage could become commonplace, enabling households to manage their water supply more effectively.
Additionally, larger-scale rainwater harvesting projects could become a significant component of urban and rural water management. These could range from community-based rainwater harvesting systems to city-wide infrastructure projects, all aimed at making the most of every drop of rainfall.
However, for these potential futures to become a reality, it is crucial for policymakers, city planners, and individuals to understand and embrace the importance of rainwater harvesting. Education, policy changes, and incentives are key drivers that can encourage this adoption and ensure that rainwater harvesting becomes part of our solution to global water scarcity.
How Smart Water is Helping Homes and Businesses to Manage Harvested Rainwater
Smart Water, with our innovative technology and systems, is leading the way in effective rainwater harvesting management. This allows homes and businesses to effortlessly monitor and manage their harvested rainwater usage, resulting in significant water savings and improved sustainability.
Smart Water's standout product is our IoT-based water level sensor , which automatically tracks and records the water level in rainwater tanks. The data is then relayed to users through a mobile app or web platform, allowing them to monitor their water usage in real time and make necessary adjustments. This level of control and visibility not only helps to prevent water wastage but also ensures a constant, reliable supply.
But it's not just about individual tanks. Smart Water's technology can be integrated across multiple tanks and sites, making it an ideal solution for businesses or larger residential complexes. This feature allows for widespread water monitoring, facilitating efficient water management on a larger scale.
The benefits of using services like Smart Water are manifold, including reduced water bills, decreased reliance on mains water, and contributing to a more sustainable future. By making the process of rainwater harvesting seamless and user-friendly, Smart Water is playing a pivotal role in encouraging wider adoption of these practices.
Shipping Calculated at Checkout
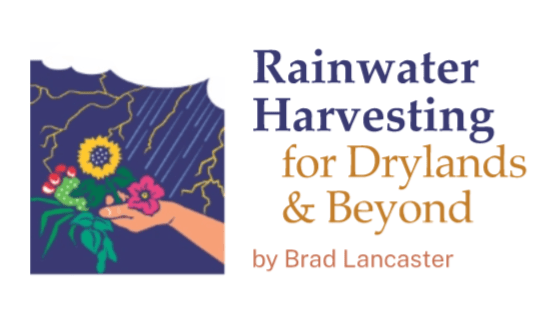
Active water harvesting case study
Underwood Family Sonoran Landscape Laboratory . College of Architecture, Planning, and Landscape Architecture (CAPLA). University of Arizona. Tucson, Arizona, USA. Latitude 32.2˚. Elevation 2,555 feet (779 meters). Average annual precipitation 11 inches (280 mm). Average annual humidity 29%…
The following is excerpted and adapted from Rainwater Harvesting for Drylands and Beyond, Volume 2, 2 nd Edition …
Many different free on-site waters such as rainwater, stormwater, air conditioning condensate, and greywater can be harvested in an integrated manner. Though only the highest quality waters (roof runoff and condensate) are typically harvested in an active tank system. While the lower quality waters (stormwater and greywater) are typically harvested within passive water-harvesting earthworks, whose life can better filter out the waters’ impurities. These passive systems also utilize the overflow from the active cistern system(s). All these waters are harvested at one of my favorite oases—the Underwood Family Sonoran Landscape Laboratory designed by Ten Eyck Landscape Architects—in an otherwise over-paved built environment. Leaving the exposed solar oven-like parking lot, you walk into a cool sanctuary of living classrooms and hang out spots beneath a canopy of abundant shade trees and diverse life. And all this is supported almost entirely by “wastewaters” harvested from adjoining buildings.
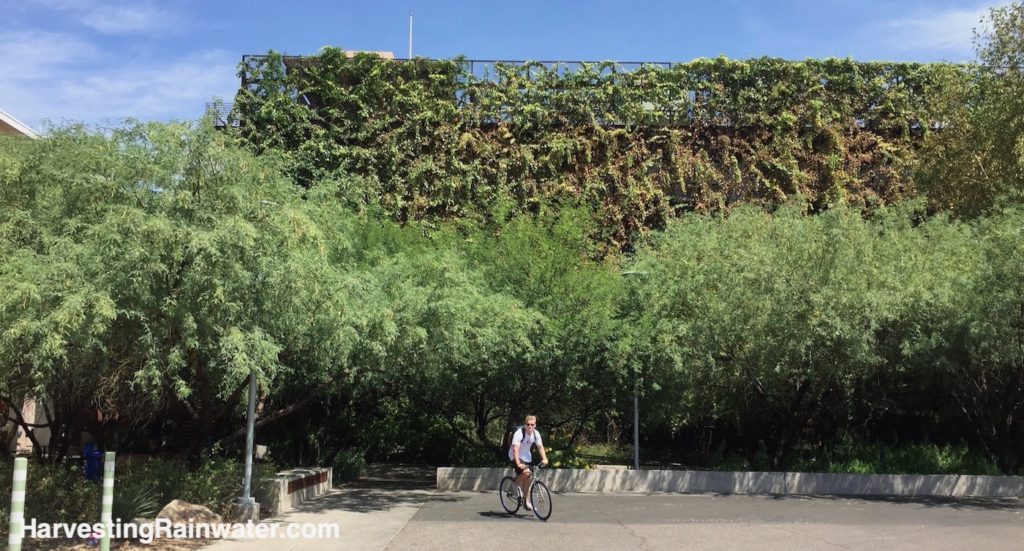
On average, 85,000 gallons (322,000 liters) of rainwater a year enters the landscape from the roofs of two adjoining buildings. More surprisingly, 95,000 gallons (360,000 liters) of air conditioning condensate a year comes to the landscape from the HVAC (heating, ventilation and air conditioning) units on these roofs, despite Tucson’s dry climate. The perspiring, respiring bodies of many students and professors inhabiting the buildings increase the moisture in the recirculating air, which the HVAC systems capture. Additionally, the landscape harvests backwash water from the daily maintenance of an adjoining sand filter well (about 200 gallons [760 liters] per day) and greywater from the building’s drinking fountains. Sink greywater is not captured because students doing studio design projects might unconsciously pour toxic solvents, paints, or glues down sink drains.
See here for the ENERGY costs of condensate and other waters , and information on how the refrigerant in air conditioners, refrigeration units, freezers, and ice machines is a major contributor to climate change.
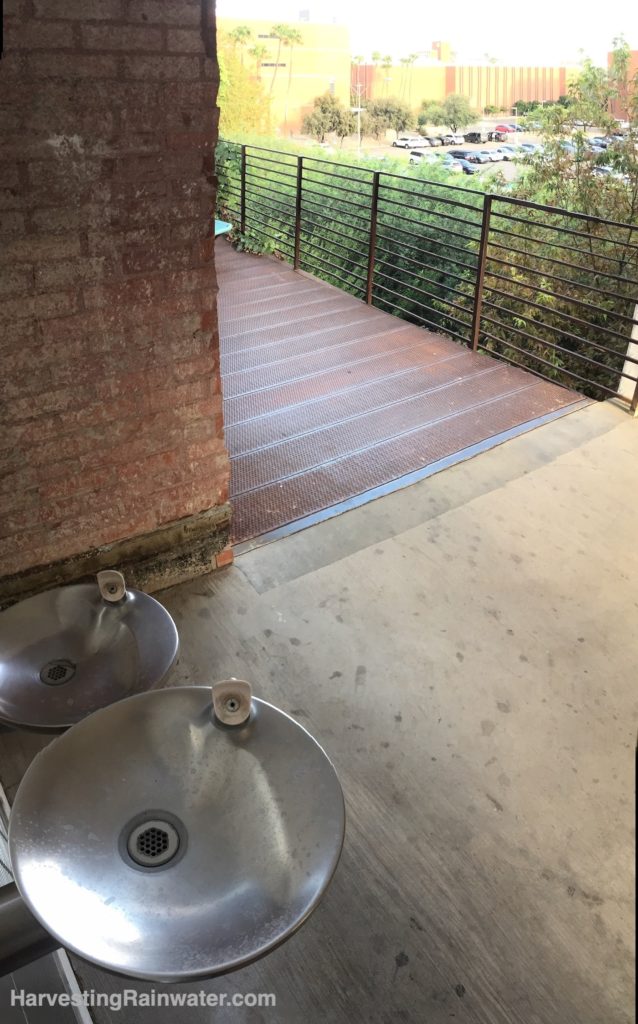
An 11,600-gallon (43,900-liter) cistern actively captures some of these waters (namely the roof runoff and condensate), while the rest—along with overflow from the cistern—is passively harvested and captured by a small pond and arroyo-like swale and its infiltration basins meandering through much of the landscape. In times of no rain, the cistern distributes its water to the landscape via a drip irrigation system.
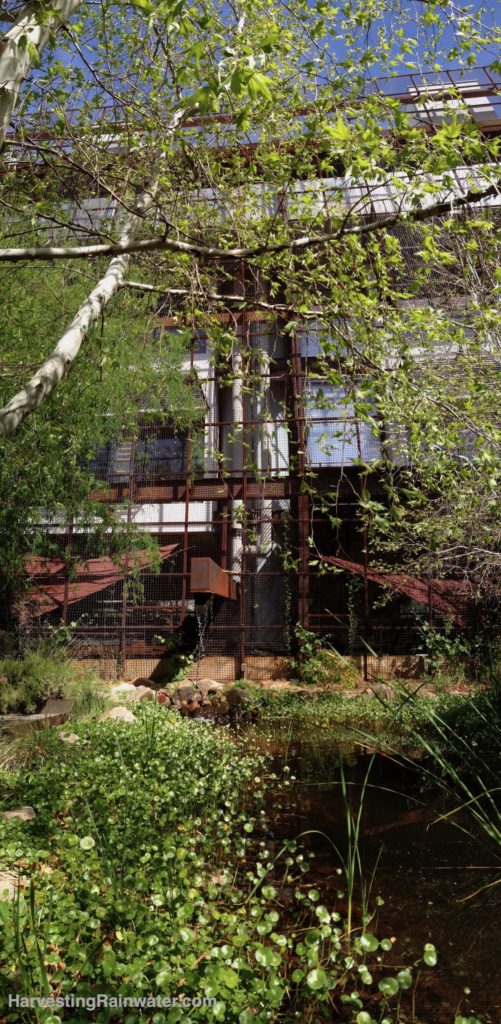
Five biomes of the Sonoran Desert—wetland, canyon, riparian, mesquite bosque, and upland Sonoran—are represented by microclimate groupings of native plant species. Endangered Gila topminnows and lowland leopard frogs are raised in the wetland’s pond. Great blue herons, roadrunners, and hawks visit to partake of the bounty. Students can eat wild grapes, chuparosa flowers, and mesquite pods.
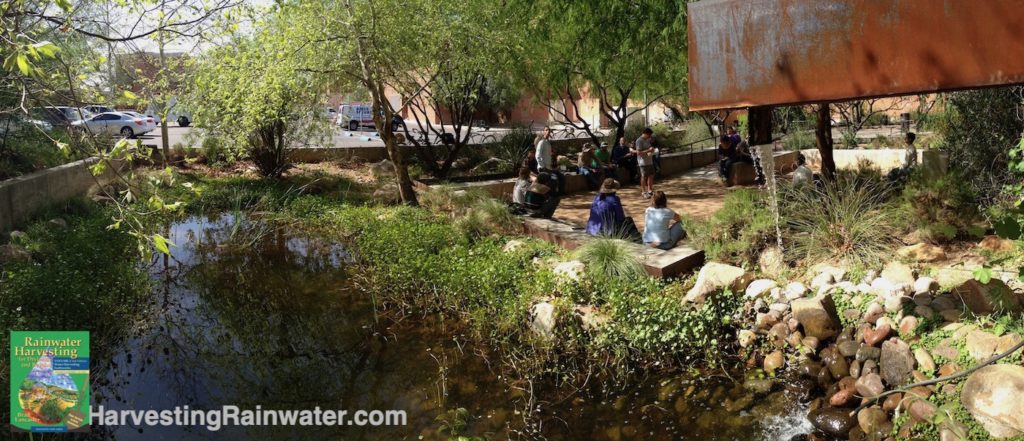
Most of the landscape’s water needs (83 to 87%) are met by these on-site “wastewaters” turned “resource waters,” though 100%+ (to give back more than one takes) could easily be met with a few small changes:
• Reduce water needs—replace a few high-water-use plants, such as an Arizona sycamore tree, with a lower-water-use tree.
• Increase water supply—direct more runoff surfaces, such as a parking lot or another roof, to the landscape.
• More efficiently slow, spread, and sink the water within the landscape—direct cistern overflow to the highest point of the landscape (currently it is directed to the midpoint or mid elevation of the landscape), which can then overflow to—and help irrigate— all points below.
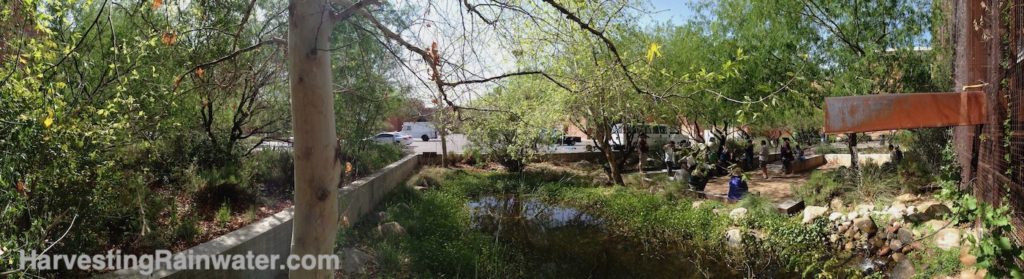
• Create higher-capacity basins and/or vertical mulching within the swale and upland Sonoran biome to increase the amount of moisture captured in the soil before overflow water escapes.
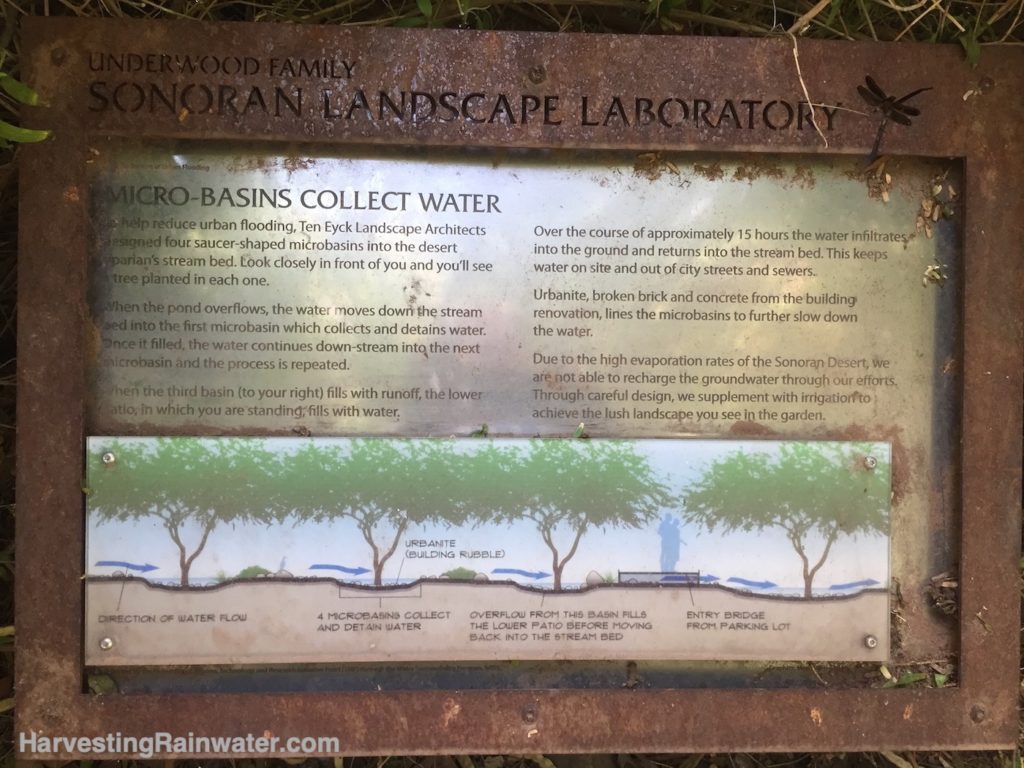
Part of the vision of this biosponge-like landscape was to illustrate a new way of landscaping, creating a model for how to transform the campus and regional landscaping. This got off to a great start with a number of local landscaping companies volunteering their time to implement the project and become familiar with these new ways of water management. The forward-thinking landscape has also lured more students into the college that want to be part of such positive change. Former associate dean Dr. Ron Stoltz, who oversaw the project, now wants to see the parking lot south of the CAPLA landscape transformed into the second-largest green space on campus, irrigated solely by discarded waters from adjoining rooftops, hardscapes, air conditioners, and greywater drains. You needn’t look far to see that this works.
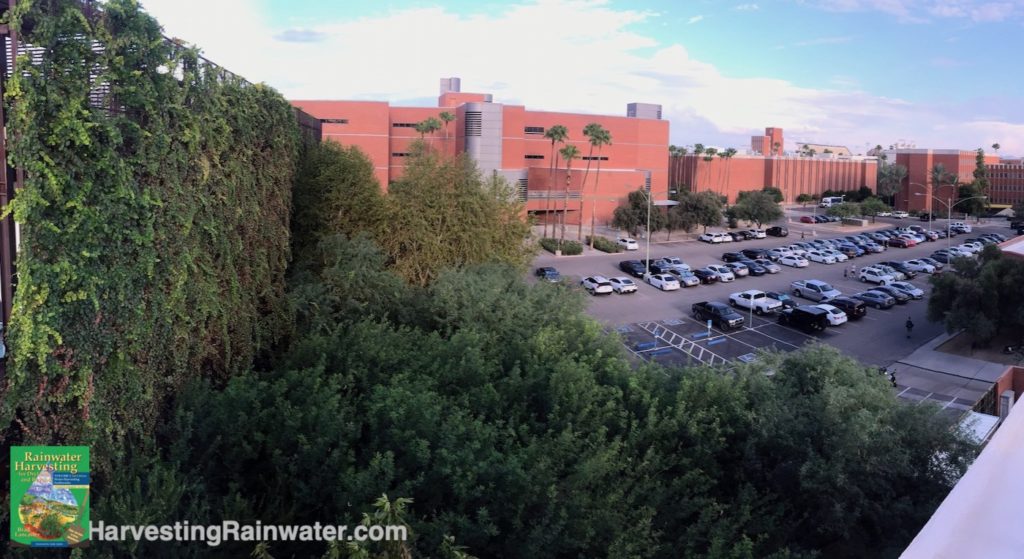
To see how this project transformed a dehydrating concrete-lined drainage -way into a hydrating cobble infiltration- way see the end of chapter 7 in Rainwater Harvesting for Drylands and Beyond, Volume 2, 2 nd Edition .
• See this website for CAPLA’s Underwood Family Sonoran Landscape Laboratory
• See this self-guided Tucson water-harvesting tour
See the new, full-color, revised editions of Brad’s award-winning books – available a deep discount, direct from Brad:
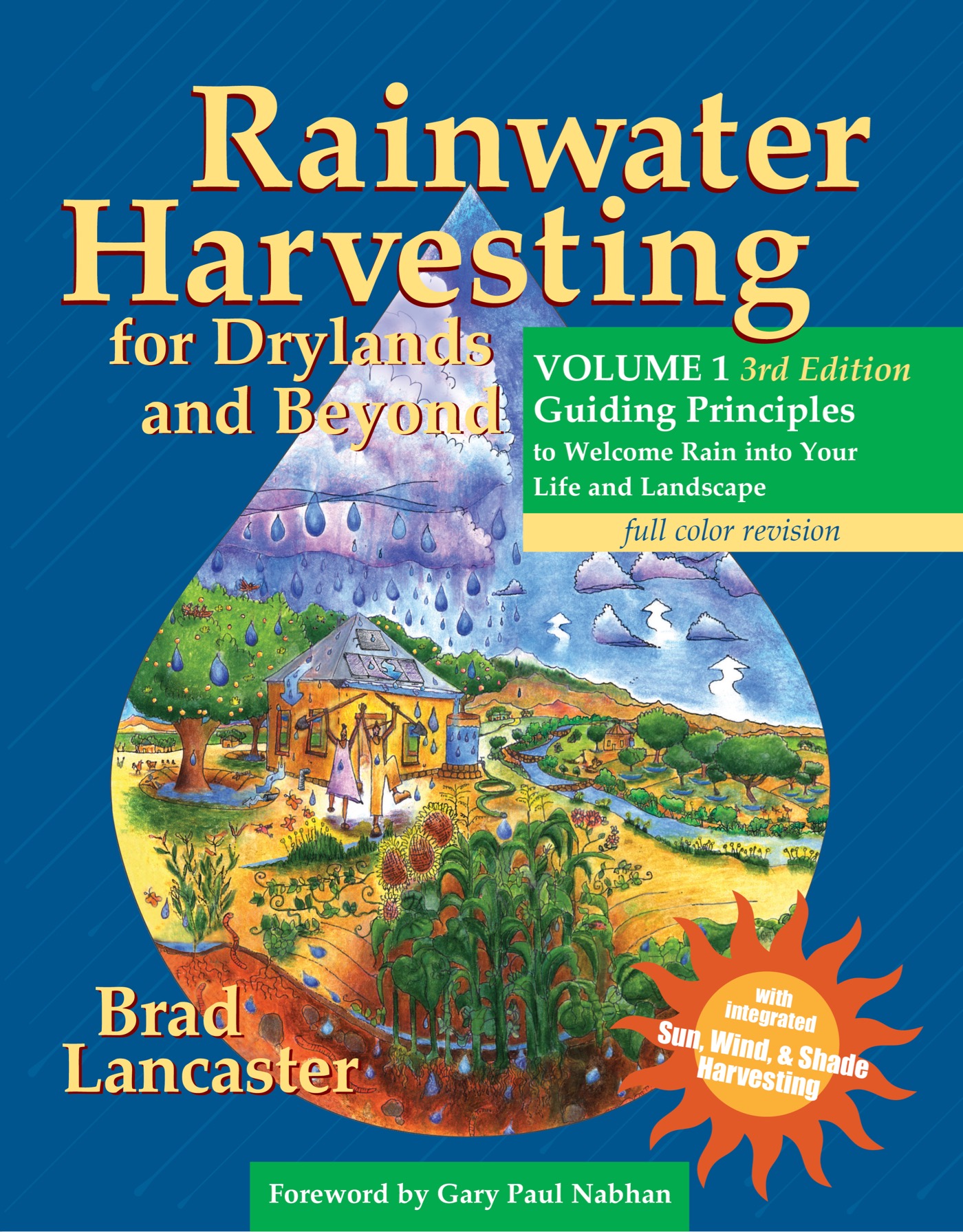
Volume 1 contains more information on active water-harvesting systems including a set of guiding principles for cistern systems, and how to size your tank based on how much water is running off your collection surface (roof) and your water needs. The book also contains information on passive water harvesting systems to which you can direct your tank overflow.
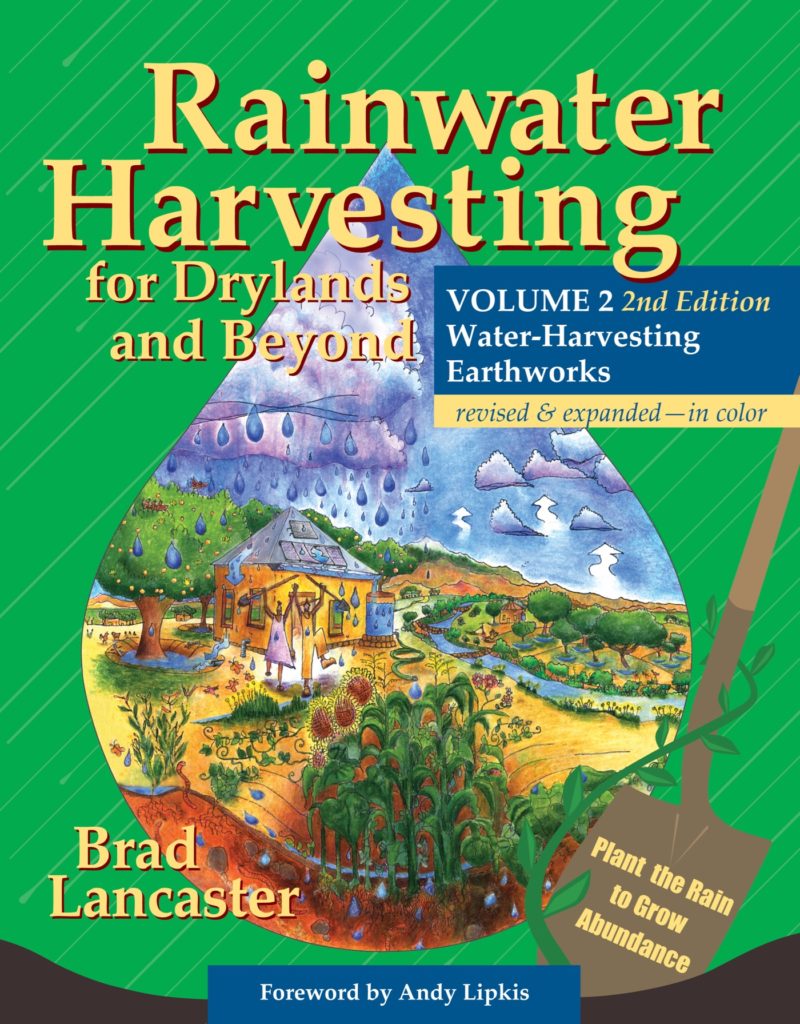
Volume 2 does not cover active water-harvesting systems, but does contain detailed step-by-step instructions on how to design, build, and grow dozens of different passive systems to which you can direct your tank overflow, or dole out your stored water.
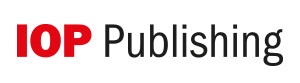
We apologize for the inconvenience...
To ensure we keep this website safe, please can you confirm you are a human by ticking the box below.
If you are unable to complete the above request please contact us using the below link, providing a screenshot of your experience.
https://ioppublishing.org/contacts/
Information
- Author Services
Initiatives
You are accessing a machine-readable page. In order to be human-readable, please install an RSS reader.
All articles published by MDPI are made immediately available worldwide under an open access license. No special permission is required to reuse all or part of the article published by MDPI, including figures and tables. For articles published under an open access Creative Common CC BY license, any part of the article may be reused without permission provided that the original article is clearly cited. For more information, please refer to https://www.mdpi.com/openaccess .
Feature papers represent the most advanced research with significant potential for high impact in the field. A Feature Paper should be a substantial original Article that involves several techniques or approaches, provides an outlook for future research directions and describes possible research applications.
Feature papers are submitted upon individual invitation or recommendation by the scientific editors and must receive positive feedback from the reviewers.
Editor’s Choice articles are based on recommendations by the scientific editors of MDPI journals from around the world. Editors select a small number of articles recently published in the journal that they believe will be particularly interesting to readers, or important in the respective research area. The aim is to provide a snapshot of some of the most exciting work published in the various research areas of the journal.
Original Submission Date Received: .
- Active Journals
- Find a Journal
- Proceedings Series
- For Authors
- For Reviewers
- For Editors
- For Librarians
- For Publishers
- For Societies
- For Conference Organizers
- Open Access Policy
- Institutional Open Access Program
- Special Issues Guidelines
- Editorial Process
- Research and Publication Ethics
- Article Processing Charges
- Testimonials
- Preprints.org
- SciProfiles
- Encyclopedia
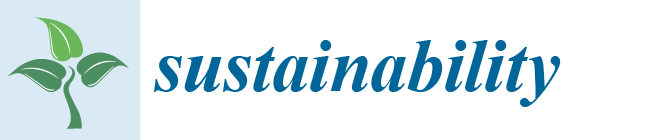
Article Menu
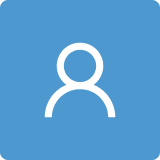
- Subscribe SciFeed
- Recommended Articles
- Google Scholar
- on Google Scholar
- Table of Contents
Find support for a specific problem in the support section of our website.
Please let us know what you think of our products and services.
Visit our dedicated information section to learn more about MDPI.
JSmol Viewer
Impact of climate change on agricultural production and food security: a case study in the mekong river delta of vietnam.
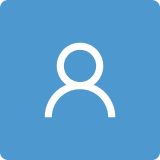
1. Introduction
2. study area and methodology, 2.1. study area, 2.2. methodology, 2.2.1. vietnam’s method of building climate change scenarios, 2.2.2. empirical research method, 2.2.3. expert method, 3.1. current status of climate change in the mekong delta region, 3.2. climate change forecast in the mekong river delta region, 4. discussion, 4.1. impacts of climate change on agricultural production, 4.1.1. impact on water resources, 4.1.2. impact on land resources, 4.1.3. impact on crop yield, 4.2. impacts of climate change on food security, 4.3. response solutions of the vietnamese government, 5. conclusions, author contributions, institutional review board statement, informed consent statement, data availability statement, conflicts of interest.
- Bibi, F.; Rahman, A. An Overview of Climate Change Impacts on Agriculture and Their Mitigation Strategies. Agriculture 2023 , 13 , 1508. [ Google Scholar ] [ CrossRef ]
- Vien, T.D. Climate change and its impact on agriculture in Vietnam. J. Int. Soc. Southeast Asian Agric. Sci. 2011 , 17 , 17–21. [ Google Scholar ]
- Hatfield, J.L.; Antle, J.; Garrett, K.A.; Izaurralde, R.C.; Mader, T.; Marshall, E.; Nearing, M.; Philip Robertson, G.; Ziska, L. Indicators of climate change in agricultural systems. Clim. Change 2020 , 163 , 1719–1732. [ Google Scholar ] [ CrossRef ]
- Qiu, J.; Shen, Z.; Xie, H. Drought impacts on hydrology and water quality under climate change. Sci. Total Environ. 2023 , 858 , 159854. [ Google Scholar ] [ CrossRef ] [ PubMed ]
- Neupane, D.; Adhikari, P.; Bhattarai, D.; Rana, B.; Ahmed, Z.; Sharma, U.; Adhikari, D. Does Climate Change Affect the Yield of the Top Three Cereals and Food Security in the World? Earth 2022 , 3 , 45–71. [ Google Scholar ] [ CrossRef ]
- Yang, W.; Feng, G.; Miles, D.; Gao, L.; Jia, Y.; Li, C.; Qu, Z. Impact of biochar on greenhouse gas emissions and soil carbon sequestration in corn grown under drip irrigation with mulching. Sci. Total Environ. 2020 , 729 , 138752. [ Google Scholar ] [ CrossRef ] [ PubMed ]
- Vietnam Institute of Meteorology, Hydrology and Environment. Guidance Document: Assessing Climate Change Impacts and Identifying Adaptation Options ; Vietnam Publishing House of Environmental Resources and Maps: Ha Noi City, Vietnam, 2011. Available online: http://csdl.dcc.gov.vn/upload/csdl/1827816293_2.-Tai-lieu-huong-dan-_-Danh-gia-tac-dong-cua-BDKH-va-xac-dinh-cac-giai-phap-thich-ung.pdf (accessed on 16 August 2024).
- Fu, J.; Jian, Y.; Wang, X.; Li, L.; Ciais, P.; Zscheischler, J.; Wang, Y.; Tang, Y.; Müller, C.; Webber, H.; et al. Extreme rainfall has reduced one-twelfth of China’s rice yield over the last two decades. Nat. Food 2023 , 4 , 416–426. [ Google Scholar ] [ CrossRef ] [ PubMed ]
- Kerr, R.B.; Hasegawa, T.; Lasco, R.; Bhatt, I.; Deryng, D.; Farrell, A.; Gurney-Smith, H.; Ju, H.; Lluch-Cota, S.; Meza, F.; et al. Chapter 5: Food, Fibre, and Other Ecosystem Products. In Climate Change 2022: Impacts, Adaptation and Vulnerability. Contribution of Working Group II to the Sixth Assessment Report of the Intergovernmental Panel on Climate Change ; Pörtner, H.-O., Roberts, D.C., Tignor, M., Poloczanska, E.S., Mintenbeck, K., Alegría, A., Craig, M., Langsdorf, S., Löschke, S., Möller, V., et al., Eds.; Cambridge University Press: Cambridge, UK; New York, NY, USA, 2022. [ Google Scholar ] [ CrossRef ]
- Shukla, P.R.; Skea, J.; Buendia, E.C.; Masson-Delmotte, V.; Pörtner, H.-O.; Roberts, D.C.; Zhai, P.; Slade, R.; Connors, S.; van Diemen, R.; et al. (Eds.) Summary for Policymakers. In Climate Change and Land: An IPCC Special Report on Climate Change, desertification, Land Degradation, Sustainable Land Management, Food Security, and Greenhouse Gas Fluxes in Terrestrial Ecosystems ; IPCC: Geneva, Switzerland, 2019; in press . [ Google Scholar ]
- FAO; IFAD; UNICEF; WFP; WHO. The State of Food Security and Nutrition in the World 2021. Transforming Food Systems for Food Security, Improved Nutrition, and Affordable Healthy Diets for All, In Brief (Report) ; FAO: Rome, Italy, 2021; ISBN 978-92-5-134634-1. [ Google Scholar ] [ CrossRef ]
- Hasegawa, T.; Sakurai, G.; Fujimori, S.; Takahashi, K.; Hijioka, Y.; Masui, T. Extreme climate events increase risk of global food insecurity and adaptation needs. Nat. Food 2021 , 2 , 587–595. [ Google Scholar ] [ CrossRef ] [ PubMed ]
- Kummu, M.; Heino, M.; Taka, M.; Varis, O.; Viviroli, D. Climate change risks pushing one-third of global food production outside the safe climatic space. One Earth 2021 , 4 , 720–729. [ Google Scholar ] [ CrossRef ] [ PubMed ]
- van Dijk, M.; Morley, T.; Rau, M.L.; Saghai, Y. A meta-analysis of projected global food demand and population at risk of hunger for the period 2010–2050. Nat. Food 2021 , 4 , 416–426. [ Google Scholar ] [ CrossRef ] [ PubMed ]
- Janssens, C.; Havlík, P.; Krisztin, T.; Baker, J.; Frank, S.; Hasegawa, T.; Leclère, D.; Ohrel, S.; Ragnauth, S.; Schmid, E.; et al. Global hunger and climate change adaptation through international trade. Nat. Clim. Change 2020 , 10 , 829–835. [ Google Scholar ] [ CrossRef ] [ PubMed ]
- Ministry of Agriculture and Rural Development of Vietnam—MARD of Vietnam. Vietnam Agriculture Sector Summary Report 2023 ; Ministry of Agriculture and Rural Development of Vietnam—MARD of Vietnam: Hanoi, Vietnam, 2023.
- Vien, T.D. Developing Vietnam’s Agriculture: Problems and Some Solutions. Vietnam Communist Magazine . 2023. Available online: https://www.tapchicongsan.org.vn/web/guest/kinh-te/-/2018/828917/phat-trien-nong-nghiep-viet-nam--van-de-dat-ra-va-mot-so-giai-phap.aspx#:~:text=TCCS%20%2D%20N%C3%B4ng%20nghi%E1%BB%87p%20l%C3%A0%20ng%C3%A0nh,Nam%20tr%C3%AAn%20tr%C6%B0%E1%BB%9Dng%20qu%E1%BB%91c%20t%E1%BA%BF (accessed on 16 August 2024).
- GSO of Vietnam. Agricultural Sector Statistics 2023 ; GSO of Vietnam: Hanoi, Vietnam, 2023.
- Germanwatch. Global Climate Risk Index 2020. Germanwatch e.V. 2020. Available online: www.germanwatch.org/en/cri (accessed on 16 August 2024).
- World Bank. Sea Level Rise from Global Warming: Potential Impact on Developing Countries ; DECRG Research Paper; World Bank: Washington, DC, USA, 2007. [ Google Scholar ]
- FAO; UNDP. Integrating Agriculture in National Adaptation Plans (NAP-Ag) Programme: Viet Nam Case Study. 2020; pp. 6,16. Available online: https://sdghelpdesk.unescap.org/sites/default/files/2020-10/nap-ag_viet-nam_case_study_publication.pdf (accessed on 16 August 2024).
- Ministry of Natural Resources and Environment in Vietnam—MONRE. Climate Change Scenario Report ; Vietnam Natural Resources—Environment and Map Publishing House: Ha Noi City, Vietnam, 2021.
- Tran, P.T.; Vu, B.T.; Ngo, S.T.; Tran, V.D.; Ho, T.D. Climate change and livelihood vulnerability of the rice farmers in the North Central Region of Vietnam: A case study in Nghe An province, Vietnam. Environ. Chall. 2022 , 7 , 100460. [ Google Scholar ] [ CrossRef ]
- Woillez, M.-N.; Espagne, E.; Ngo, D.T.; Nguyen, M.H.; Pannier, E.; Drogoul, A.; Huynh TP, L.; Le, T.T.; Nguyen TT, H.; Nguyen, T.T.; et al. Climate Change in Vietnam, Impacts and Adaptation (p. 612) [A COP26 Assessment Report of the GEMMES Vietnam Project]. AFD Group. 2021, pp. 199–200. Available online: https://www.afd.fr/en/ressources/gemmes-vietnam-climate-change-impacts-and-adaptation (accessed on 16 August 2024).
- General Statistics Office (GSO) of Vietnam. Statistics of the Mekong Delta Region ; General Statistics Office (GSO) of Vietnam: Hanoi, Vietnam, 2023.
- Le, S. Salinity intrusion in the Mekong Delta ; Agriculture Publisher: Ho Chi Minh City, Vietnam, 2016. [ Google Scholar ]
- Mekong River Commission (MRC). Study on Hydro-Meteorological Monitoring for Water Quality Rules in the Mekong River Basin: Final Report, II (Supporting Report 2/2) ; WUP-JICA: Phnom Penh, Cambodia, 2024. [ Google Scholar ]
- MONRE—Ministry of Natural Resources and Environment in Vietnam. National State of the Environment Report 2018: Special Topic—Water Environment of River Basins ; MONRE—Ministry of Natural Resources and Environment in Vietnam: Hanoi, Vietnam, 2018.
- Son, C.T.; Giang, N.T.H.; Thao, T.P.; Nui, N.H.; Lam, N.T.; Cong, V.H. Assessment of Cau River water quality assessment using a combination of water quality and pollution indices. J. Water Qual. Supply: Res. Technol.-Aqua 2020 , 69 , 160–172. [ Google Scholar ] [ CrossRef ]
- MARD—Ministry of Agriculture and Rural Development in Vietnam. Report on Drought and Salinity Intrusion in the Mekong Delta Provinces in 2016 ; MARD—Ministry of Agriculture and Rural Development in Vietnam: Hanoi, Vietnam, 2016.
- MONRE. Decision No. 1432 of the Ministry of Natural Resources and Environment in Vietnam to Announce the Results of Land Investigation and Assessment, Nationwide, Socio-Economic Regions ; MONRE: Hanoi, Vietnam, 2021. [ Google Scholar ]
- United Nations Development Program—UNDP. Vietnam’s Special Report on Disaster Risk Management and Extreme Phenomena to Promote Adaptation to Climate Change ; Vietnam Natural Resources—Environment and Map Publishing House: Ha Noi City, Vietnam, 2015. [ Google Scholar ]
- UN; CRS; Save the Children. Drought and Saltwater Intrusion in the Mekong Delta in Vietnam ; Combined Assessment Report; UN, CRS and Save the Children: London, UK, 2020. [ Google Scholar ]
- Trong, P.T.; Duc, V.T.; Truong, S.C.; Thanh, T.D.; Huu, D.N.; Scholz, W. Analysing Factors Influencing Land Use Planning for Sustainable Land Resource Management in Vietnam: A Case Study of Dan Phuong District in Hanoi City. Sustainability 2023 , 15 , 16557. [ Google Scholar ] [ CrossRef ]
- Asian Development Bank—ADB. Viet Nam: Environment and Climate Change Assessment ; Asian Development Bank: Mandaluyong City, Philippines, 2013. [ Google Scholar ]
- World Bank. Transforming Vietnamese Agriculture: Gaining More from Less ; Vietnam Development Report; World Bank Group: Washington, DC, USA, 2016; p. 148. Available online: https://openknowledge.worldbank.org/bitstream/handle/10986/24375/108510.pdf?sequence=5&isAllowed=y (accessed on 16 August 2024).
- Vietnam Government. Decision 1490/QD-TTg Dated November 27, 2023, Approving the Project “Sustainable Development of one Million Hectares of High-Quality and Low-Emission Rice Cultivation Associated with Green Growth in the Mekong Delta by 2030” ; Vietnam Government: Hanoi, Vietnam, 2023.
- MOSAT—Ministry of Science and Technology in Vietnam. Decision 3289/QĐ-BKHCN Dated December 29, 2023, Approved the “National Science and Technology Program for the Period Up to 2030—Science and Technology in Response to Climate Change for Sustainable Development of the Mekong Delta, Code: KC.15/21-30” ; MOSAT—Ministry of Science and Technology in Vietnam: Hanoi, Vietnam, 2021.
- Vietnam Government. Decision 1658/QD-TTg Dated October 1, 2021, Approved the “National Strategy on Green Growth for the 2021–2030 Period, with a Vision to 2050” ; Vietnam Government: Hanoi, Vietnam, 2021.
Click here to enlarge figure
No | Province | RCP4.5 | RCP8.5 | ||
---|---|---|---|---|---|
2046–2065 | 2080–2099 | 2046–2065 | 2080–2099 | ||
1 | Long An | 1.3 (0.9–2.0) | 1.8 (1.2–2.6) | 1.9 (1.4–2.6) | 3.4 (2.6–4.6) |
2 | Tien Giang | 1.3 (0.9–2.0) | 1.8 (1.2–2.6) | 1.9 (1.4–2.7) | 3.4 (2.7–4.6) |
3 | Dong Thap | 1.3 (0.9–2.0) | 1.7 (1.1–2.5) | 1.9 (1.3–2.6) | 3.3 (2.6–4.5) |
4 | Vinh Long | 1.3 (0.9–1.9) | 1.7 (1.2–2.6) | 1.8 (1.3–2.6) | 3.4 (2.6–6.5) |
5 | Tra Vinh | 1.3 (0.9–2.0) | 1.7 (1.2–2.5) | 1.8 (1.3–2.6) | 3.3 (2.6–4.5) |
6 | Can Tho | 1.3 (0.9–1.9) | 1.7 (1.2–2.6) | 1.8 (1.3–2.6) | 3.4 (2.6–4.5) |
7 | Hau Giang | 1.3 (0.9–2.0) | 1.7 (1.2–2.5) | 1.8 (1.3–2.5) | 3.2 (2.6–4.2) |
8 | Soc Trang | 1.3 (0.9–1.9) | 1.7 (1.1–2.5) | 1.8 (1.3–2.5) | 3.3 (2.5–4.3) |
9 | Ben Tre | 1.3 (0.9–1.9) | 1.7 (1.1–2.4) | 1.8 (1.3–2.5) | 3.2 (2.6–4.2) |
10 | An Giang | 1.3 (0.9–2.0) | 1.8 (1.1–2.6) | 1.9 (1.3–2.6) | 3.4 (2.5–4.6) |
11 | Kien Giang | 1.3 (0.9–2.0) | 1.7 (1.2–2.5) | 1.8 (1.3–2.5) | 3.2 (2.6–4.2) |
12 | Bac Liêu | 1.3 (0.9–1.9) | 1.7 (1.1–2.4) | 1.7 (1.3–2.4) | 3.2 (2.5–4.2) |
13 | Ca Mau | 1.3 (0.9–1.9) | 1.7 (1.1–2.4) | 1.8 (1.3–2.5) | 3.2 (2.5–4.3) |
14 | Mekong Delta | 1.3 (0.9–1.95) | 1.7 (1.15–2.5) | 1.8 (1.3–2.55) | 3.3 (2.5–6.5) |
No | Province | RCP4.5 | RCP8.5 | ||
---|---|---|---|---|---|
2046–2065 | 2080–2099 | 2046–2065 | 2080–2099 | ||
1 | Long An | 8.3 (0.7–32.0) | 14.7 (3.2–26.6) | 19.2 (7.8–30.2) | 26.3 (15.5–42.1) |
2 | Tien Giang | 16.8 (−1.8–37.1) | 14 (−0.9–28.6) | 18.9 (6.7–31.1) | 23.7 (8.9–40.7) |
3 | Dong Thap | 17.0 (−2.0–31.0) | 14.9 (2.3–26.9) | 18.3 (9.1–28.7) | 24.6 (15.7–39.4) |
4 | Vinh Long | 16.3 (0.8–28.5) | 12.5 (1.4–22.0) | 20.3 (12.4–31.2) | 21.2 (13.0–35.3) |
5 | Tra Vinh | 16.7 (−3.3–30.3) | 13.2 (4.4–20.2) | 20.6 (11.4–32.3) | 24.3 (14.4–37.5) |
6 | Can Tho | 16.3 (0.8–28.5) | 12.5 (1.4–22.0) | 20.3 (12.4–31.2) | 21.2 (13.0–35.5) |
7 | Hau Giang | 14.5 (4.2–25.5) | 19.2 (3.8–33.8) | 19.0 (7.8–30.1) | 24.9 (12.1–42.8) |
8 | Soc Trang | 15 (1.2–26.0) | 14.1(4.0–23.1) | 19.0 (11.4–26.9) | 23.4 (12.3–39.8) |
9 | Ben Tre | 17.9 (−2.8–33.3) | 19.2 (4.9–33.4) | 21.6 (10.4–33.8) | 29.2 (12.6–47.9) |
10 | An Giang | 16.9 (2.3–31.2) | 15.0 (2.1–27.9) | 18.3 (5.7–31.5) | 20.4 (8.2–37.4) |
11 | Kien Giang | 14.5 (4.2–25.5) | 19.2 (3.8–33.8) | 19.0 (7.8–30.1) | 24.9 (12.1–42.8) |
12 | Bac Liêu | 12.5 (1.3–21.8) | 13.1 (5.0–19.8) | 18.0 (11.3–24.7) | 20.1 (11.4–33.0) |
13 | Ca Mau | 13.9 (1.8–23.8) | 13.9 (6.0–20.8) | 15.4 (6.9–22.7) | 19.9 (11.4–30.3) |
14 | Mekong Delta | 8.3–17.9 (−3.3–37.1) | 12.5–19.2 (−0.9–33.8) | 18.3–21.6 (5.7–33.8) | 19.9–26.3 (8.2–47.9) |
Province | Areas (ha) | % Flooded Area Corresponding to Rising Sea Levels | |||||||||
---|---|---|---|---|---|---|---|---|---|---|---|
10 cm | 20 cm | 30 cm | 40 cm | 50 cm | 60 cm | 70 cm | 80 cm | 90 cm | 100 cm | ||
Long An | 449,100 | 0 | 0 | 0.31 | 0.49 | 0.61 | 1.36 | 2.85 | 7.12 | 12.89 | 27.21 |
Tien Giang | 251,061 | 0.13 | 0.71 | 1.43 | 2.57 | 3.79 | 6.71 | 12.58 | 24.06 | 37.69 | 47.8 |
Dong Thap | 337,860 | 0 | 0 | 0.17 | 0.21 | 0.36 | 0.69 | 0.96 | 1.28 | 1.94 | 4.64 |
Vinh Long | 152,573 | 0 | 0.34 | 0.61 | 0.91 | 1.31 | 2.02 | 3.66 | 8.28 | 18.34 | 32.03 |
Tra Vinh | 235,826 | 0.5 | 0.61 | 0.89 | 1.28 | 2.29 | 4.95 | 11.51 | 22.22 | 32.79 | 43.88 |
Can Tho | 143,896 | 0 | 0 | 0.03 | 0.05 | 0.99 | 2.88 | 9.97 | 26.69 | 44.89 | 55.82 |
Hau Giang | 162,170 | 0 | 0.75 | 3.42 | 10.31 | 18.83 | 29.37 | 38.5 | 45.88 | 53.21 | 60.85 |
Soc Trang | 331,188 | 1.78 | 2.91 | 5.13 | 8.32 | 11.32 | 14.97 | 20.25 | 26.91 | 33.13 | 55.41 |
Ben Tre | 239,481 | 0.55 | 1.43 | 2.52 | 4.08 | 6.74 | 10.19 | 15.11 | 21.46 | 27.83 | 35.11 |
An Giang | 342,400 | 0 | 0 | 0 | 0.03 | 0.08 | 0.16 | 0.29 | 0.49 | 0.9 | 1.82 |
Kien Giang | 634,878 | 0.66 | 3.38 | 12.63 | 23.67 | 36.82 | 48.85 | 58.47 | 66.16 | 71.69 | 75.68 |
Bac Liêu | 266,901 | 0.71 | 2.87 | 6.66 | 12.14 | 20.08 | 27.78 | 36.84 | 46.31 | 54.38 | 61.87 |
Ca Mau | 522,119 | 7.21 | 14.06 | 20.17 | 28.73 | 40.31 | 48.05 | 56.81 | 64.42 | 73.58 | 79.62 |
Mekong Delta | 4,069,453 | 1.29 | 2.97 | 5.92 | 9.86 | 14.86 | 19.69 | 27.94 | 31.94 | 38.8 | 47.29 |
No | Impact | Unit | Value |
---|---|---|---|
1 | Area of agricultural land affected by salinity | Ha | 119,913 |
2 | Lack of fresh water for daily life | Household | 155,000 |
Person | 800,000 | ||
3 | Affected rice area by salinity (Not cultivated in the right season) | Ha | 400,000 |
4 | Area of rice lost by salinity | Ha | 160,000 |
Types of Soil Degradation | Vietnam (10 ha) | MRD | |
---|---|---|---|
Area (10 ha) | Compared to the Whole Country (%) | ||
Fertility loss | 13,417 | 833 | 6.21 |
Soil erosion | 13,358 | 0 | 0.00 |
Drought, desertification, and desertification | 16,823 | 29 | 0.17 |
Concretion, laterite | 1156 | 0 | 0.00 |
Salinization | 197 | 23 | 11.68 |
Acid sulfate soil | 125 | 67 | 53.60 |
The statements, opinions and data contained in all publications are solely those of the individual author(s) and contributor(s) and not of MDPI and/or the editor(s). MDPI and/or the editor(s) disclaim responsibility for any injury to people or property resulting from any ideas, methods, instructions or products referred to in the content. |
Share and Cite
Phuong, T.T.; Vien, T.D.; Son, C.T.; Thuy, D.T.; Greiving, S. Impact of Climate Change on Agricultural Production and Food Security: A Case Study in the Mekong River Delta of Vietnam. Sustainability 2024 , 16 , 7776. https://doi.org/10.3390/su16177776
Phuong TT, Vien TD, Son CT, Thuy DT, Greiving S. Impact of Climate Change on Agricultural Production and Food Security: A Case Study in the Mekong River Delta of Vietnam. Sustainability . 2024; 16(17):7776. https://doi.org/10.3390/su16177776
Phuong, Tran Trong, Tran Duc Vien, Cao Truong Son, Doan Thanh Thuy, and Stefan Greiving. 2024. "Impact of Climate Change on Agricultural Production and Food Security: A Case Study in the Mekong River Delta of Vietnam" Sustainability 16, no. 17: 7776. https://doi.org/10.3390/su16177776
Article Metrics
Article access statistics, further information, mdpi initiatives, follow mdpi.
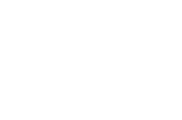
Subscribe to receive issue release notifications and newsletters from MDPI journals
- Open access
- Published: 01 September 2024
Downstream impacts of dam breach using HEC-RAS: a case of Budhigandaki concrete arch dam in central Nepal
- Anu Awal 1 ,
- Utsav Bhattarai 2 ,
- Vishnu Prasad Pandey 3 &
- Pawan Kumar Bhattarai 4
Environmental Systems Research volume 13 , Article number: 37 ( 2024 ) Cite this article
Metrics details
Studies on concrete dam breach are limited compared to earthen and other types of dams. With an increase in the construction of concrete dams, particularly in the developing world, it is imperative to have a better understanding of the dam breach phenomena and the identification of the most influential breach parameters. This study aims to contribute to this gap by taking the case of the concrete arch dam proposed for the 1200 MW Budhigandaki Hydropower Project located in central Nepal. This study carries special significance for Nepal, primarily because of the increasing number of under construction and proposed large dams for water resources development in the country. We carry out dam breach analysis of the Budhigandaki dam using HEC-RAS 2D model to calculate the flood discharge peaks, time to peak, water surface elevation and the extent of inundation for two scenarios (with and without probable maximum flood) to estimate the damage on four downstream settlements. We carry out sensitivity analysis of the breach parameters on the flood magnitudes and severity. Results show that all the study locations lie in the high flood hazard zone. Flood peaks can reach as high as 286,000 m 3 s − 1 to 511,000 m 3 s − 1 in the considered settlements. The time to peak ranges from 11.3 to 17 h after the breach at these locations. We estimate that if a breach should happen, it would most likely inundate around 150,000 buildings, impact nearly 672,000 lives and flood 3,500 km of road downstream. Furthermore, dam breach elevation is found to be the most sensitive parameter to downstream floods. Hence, rather than structural measures, it is recommended that non-structural measures are implemented for minimizing the impacts of flood disasters at the study locations. The findings could be a useful reference for future dam projects in Nepal and other areas with similar hydrological and topographical conditions.
Introduction
Dams are storage structures providing beneficial functions such as flood control and water supply for different types of users (for example, domestic water supply, hydropower, irrigation, recreation and water transport). The construction of large dams along with generation of electricity started during the industrial revolution in Europe and America. The early 1900s ushered in an era of “big dam” building in America mostly for hydropower generation as demands for electricity increased, the Hoover Dam being regarded as an engineering marvel. The Asian region includes some of the largest dams in the world today such as Tarbela Dam and Mangla Dam in Pakistan, Nurek Dam in Tajikistan, San Rogue Dam in Phillipines and Three Gorges Dam in China, mostly for hydropower generation.
Despite the benefits, failure of dams can cause tremendous losses by generation of unforeseen flood magnitudes in downstream areas. Unfortunately, the history of dams has been studded with disasters of various types, sometimes of great magnitude, with loss of human lives and destruction of property and infrastructure (Aureli et al. 2021 ). USACE ( 2018 ) lists causes of dam breach as earthquakes, landslides, extreme storms, piping, equipment malfunction, structural damage, foundation failure, and sabotage. Regardless of the reason, almost all failures begin with a breach formation.
Basically, breach is defined as the opening formed in the dam body that leads the dam to fail and this phenomenon causes the stored water behind the dam to propagate rapidly downstream (Dincergok 2007 ). Despite piping or overtopping being the main modes of dam failure, the actual mechanics are still not completely understood for either earthen or concrete dams (USACE 2018 ). Past dam-failure disasters have shown that the majority of dams that have failed are earthen (74 dam breaks out of 7812 earthen dams) and the highest percentage of failure of rockfill dams (17 dam breaks out of 200 rockfill dams) (Fang et al. 2017 ). The world’s worst dam disaster happened in China in 1975 when the Banqiao and Shimantan dams failed killing about 171,000 people while 11 million lost their homes (Vincent et al. 2020 ). In 1979, the 25 m high Machu Dam in India, which stored 100 million m 3 , failed after several hours of over-topping causing about 10,000 deaths, 150,000 people were displaced, and 10,000 habitations were destroyed (Lempérière 2017 ). A recent case of the failure of the Rishiganga dam in Uttarakhand (India) in 2021 due to glacier avalanche caused more than 200 deaths and severely damaged infrastructure (Shugar et al. 2021 ). Similarly, failure of the Edenville dam followed by the Sanford dam downstream on the same day in 2020 due to heavy rain in Michigan USA ( Independent Forensic Team 2022 ), and failure of the Spencer Dam in Nebraska USA in 2019 due to ice run (Ettema et al. 2021 ), demonstrate the devastation that dam breaches can lead to. Thus, identification of the vulnerable areas and being aware of the likely damages are key for minimization of the adverse impacts of dam breach.
Dam breach analysis involves three key sequential steps: predicting the reservoir outflow hydrograph, determining dam breach parameters, and routing the hydrograph downstream. Essentially, the breach flood hydrograph depends on the prediction of breach geometry and breach formation time (Basheer et al. 2017 ). There have been many studies on dam breach analysis around the world from the 1980’s (Leng et al. 2023 ; Singh and Snorrason 1984 ; USACE 2024 ). Dam breach analysis is generally carried out by either numerical/computer models or scaled-down physical models. The United States Department of Interior ( 1988 ), recommends estimating a reasonable maximum breach discharge using four principal methods:
Physically Based Methods: Using erosion models based on principles of hydraulics, sediment transport and soil mechanics, development of breach and resulting breach outflow are estimated;
Parametric Models: Time to failure and ultimate breach geometry are assessed utilizing case studies; breach growth is simulated as a time-dependent linear process and breach outflows are computed using principles of hydraulics;
Predictor Equations: Using data of case studies, peak discharge is estimated from empirical equations and a reasonable shape of outflow hydrograph is assumed; and.
Comparative Analysis: Breach parameters are determined by comparison of dam under consideration and a dam that failed.
There are far fewer studies on the failures of concrete dams compared to earthen dams, especially due to breaches which leads to difficulty in determining the concrete dam breach parameters (Fang et al. 2017 ). Moreover, a study of well documented dam-failure cases showed that empirical formulas provide results closer to reality (Fang et al. 2017 ). For instance, Froehlich( 1995 ) developed a prediction equation for the average breach width based on 63 cases of embankment-dam failures and an equation for the breach-formation time based on 21 cases. Focusing on earthen dams has been driven by their historical prevalence, cost-effectiveness, and adaptability. However, studying concrete arch dams is crucial for advancing engineering practices, improving safety and efficiency in dam construction, supporting hydroelectric power generation, addressing environmental impacts, and preserving significant cultural landmarks. Many federal agencies such as FERC ( 1993 ),Office of the State Engineer( 2020 ) and USACE ( 2014 ) have published guidelines recommending possible ranges of values for breach width, side slopes, and development time for different types of dams. This study aims to investigate the breach characteristics of concrete arch dams, an area with limited existing literature. Several dam breach analysis studies have been carried out in Nepal such as in Kulekhani dam using HEC-RAS (Pandey et al. 2023 ), Kaligandaki landslide dam using BREACH (Bricker et al. 2017 ), Koshi high dam using HEC-RAS (Gyawali, D.R. and Devkota, 2015 ), among others. However, no sensitivity analysis of dam breach parameters has been carried out for the afore-mentioned studies.
The proposed Budhigandaki dam located in the transboundary Budhigandaki Basin, spread over southern China and central Nepal, is taken as a case. The Government of Nepal (GoN) has prioritized hydropower generation as the backbone of economic development to attain the goals to raise the country’s status to middle income country level by 2030 (Government of Nepal 2020 ). As a result, there are currently more than 9 planned and proposed large hydropower dam projects by the state (Nepal Electricity Authority 2022 ). The Budhigandaki Hydropower Project (BGHPP) could be the largest storage project of Nepal, if constructed, which could lead to catastrophic damages downstream in the event of a breach.
Hence, the overarching objective of this study is to assess the flood impacts of the Budhigandaki Dam on the downstream settlements due to possible dam breach scenarios. Specifically, this study intends to quantify the peak discharge, time to peak, and the water surface elevation at the downstream locations due to a dam-breach flood. Further, sensitivity analysis of five different dam breach parameters is conducted to acquire information about extent of influence of each parameter on the dam breach. The analysis is carried out in the widely-used hydraulic model Hydrologic Engineering Center’s - River Analysis System (HEC-RAS) developed by the United States Army Corps of Engineers (USACE). Furthermore, zoning of the downstream settlement areas in Geographic Information System (GIS) based on flood severity provides meaningful information to the project developers as well as planners in the impacted areas.
Materials and methods
The Budhigandaki Hydropower Project (BGHPP) is a 1200 MW storage type proposed project of Nepal located approximately 2 km upstream of the confluence of Budhigandaki River with Trishuli River as shown in Fig. 1 . The Budhigandaki Dam is a 263 m high double curvature concrete arch dam with a reservoir volume of 4.5 billion cubic meters (BCM), out of which the active storage is 2.2 BCM. The dam crest length is 737.4 m and the reservoir Full Supply Level (FSL) is at 540 m above sea level (masl) (Budhigandaki Development Committee, 14a). There are some major settlement areas nearly 110 km downstream which are susceptible to danger in case of dam breach. For this study, four major towns namely, Narayangarh, Baraghare, Divyanagar and Meghauli, have been assessed. Moreover, future risk of impact from the dam failure can be expected to increase as increased in population growth due to improved job opportunities and other economic activities in the area because of the construction of the dam. Therefore, the Budhigandaki Dam has been taken as a case in this study to assess the flooding impacts of the dam on the downstream areas through simulation of a hypothetical dam failure.
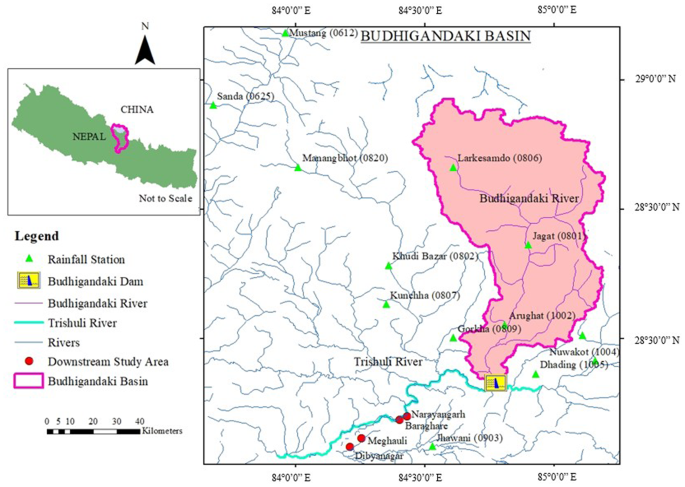
Location of Budhigandaki dam and downstream settlement areas
Methodology
Dam breach analysis of the Budhigandaki dam has been carried out in HEC-RAS using unsteady flow simulation with terrain and land cover as the geometric input data. The upstream boundary condition is the probable maximum flood (PMF) hydrograph which has been generated using an empirical method while the downstream boundary condition is normal depth. Two dam failure scenarios, namely, dam breach at reservoir full condition with PMF (Scenario I: base case) and dam breach at reservoir full condition without PMF (Scenario II), have been modelled in the study. Outputs of the simulation are used for creating flood inundation maps, flood hazard vulnerability maps and flood arrival time maps corresponding to the different scenarios. Sensitivity analysis of the dam breach parameters is also carried out to assess their impacts on the flood conditions downstream of the dam. Figure 2 summarizes the overall research methodology.
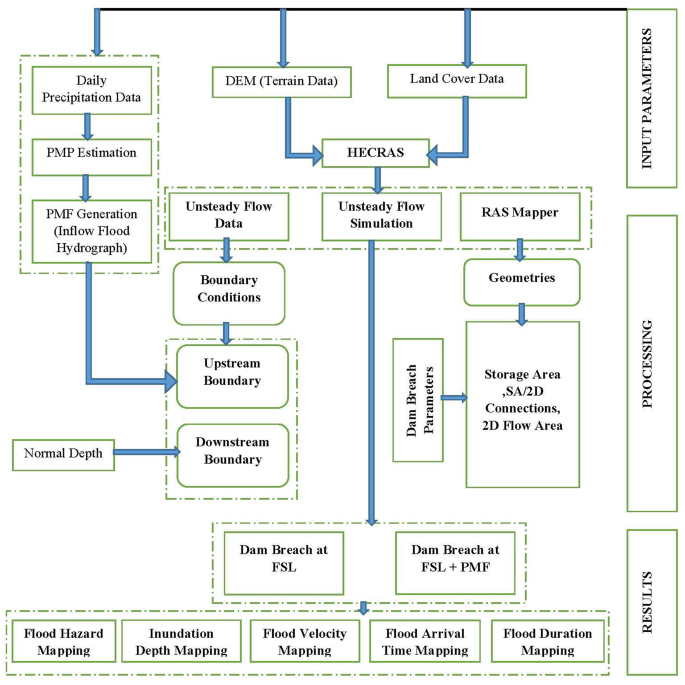
Overall research methodology of this study. DEM: Digital Elevation Model, PMP: Probable Maximum Precipitation, PMF: Probable Maximum Flood, SA: Storage Area, 2D: Two Dimensional, FSL: Full Supply Level
The spatial inputs required to model the dam breach are digital elevation model (DEM), land cover and Manning’s roughness coefficient. Rainfall and discharge are needed for generation of inflow hydrograph as upstream boundary condition to the model. In addition, infrastructure data of the downstream area is required for estimating the impacts of floods. Details of the required data and their sources are presented in Table 1 .
PMP and PMF
The probable maximum precipitation (PMP) is the theoretical maximum precipitation for a given duration under current meteorological conditions (World Meteorological Organization 2009 ). Daily maximum rainfall data of 13 surrounding stations from 1972 to 2014 has been used for the calculation of PMP. The 1-day PMP for all the stations was calculated using Hershfield formula (Hershfield 1965 ) given in Eq. ( 1 ) :
Where, PMP = Probable maximum precipitation.
M = mean of maximum daily rainfall sample S = Standard deviation.
K = Frequency factor = 15 (Hershfield 1965 ).
The calculated 1-day PMP of the point stations was further interpolated using Thiessen Polygon, Kriging, Spline and Inverse Distance Weighing (IDW) methods in GIS to compute the 1-day PMP for the Budhigandaki Basin. In order to model a worst-case scenario, the maximum value of the PMP among these methods was chosen for generating the PMF hydrograph.
Probable Maximum Flood (PMF) is theoretically the flood resulting from a combination of the most severe meteorological and hydrologic conditions that could conceivably occur in a given area (FERC 2001 ). HEC-RAS requires a flood hydrograph to be provided as input for the unsteady flow analysis in the dam breach model. Therefore, a synthetic unit hydrograph was developed using Snyder’s Method (American Geophysical Union 1938 ) using the following equations (Eq. ( 2 ) to Eq. ( 7 ) which was then transposed to generate a direct runoff hydrograph of PMF.
Mathematically,
Dam breach analysis
Dam breach analysis of the Budhigandaki dam has been carried out in HEC-RAS model under two-dimensional dynamic (unsteady-flow) mode. Hypothetical breach of the dam and its propagation downstream has been modelled using 2D Diffusion wave equations (Eq. ( 8 ) to Eq. ( 10 )).
Where, h is the water depth (m), p and q are the specific flow in the x and y directions (m 2 s − 1 ), ζ is the surface elevation (m), g is the acceleration due to gravity (9.8 m s − 2 ), n is the Manning’s coefficient, ρ is the water density (1000 kg m − 3 ), τ xx , τ yy , and τ xy are the components of the effective shear stress along x and y directions (N m − 2 ), and f is the Coriolis (s − 1 ).
Two-dimensional (2D) mesh of size 100 m x 100 m was chosen to represent the downstream land. Comparison of different mesh sizes (100 m and 200 m) indicated no significant difference in model performance. The storage areas and downstream areas are connected using an inline structure (Budhigandaki dam) as shown in Fig. 3 . “Storage Area” refers to upstream reservoir of the dam axis while “Downstream Study Area” represents the four towns (Narayangarh, Baraghare, Divyanagar, and Meghauli) located downstream which are likely to be inundated in case of dam breach (BGHP, 2015). Boundary conditions are required at the upstream and downstream ends of the model for flood routing. The upstream boundary was fixed at the reservoir extent (storage area) and the boundary condition was provided in the form of flood hydrograph generated from PMF. Outlet is the downstream boundary past the settlement areas as shown in Fig. 3 while the boundary condition of normal depth is maintained by providing the river bed-slope obtained from the DEM.
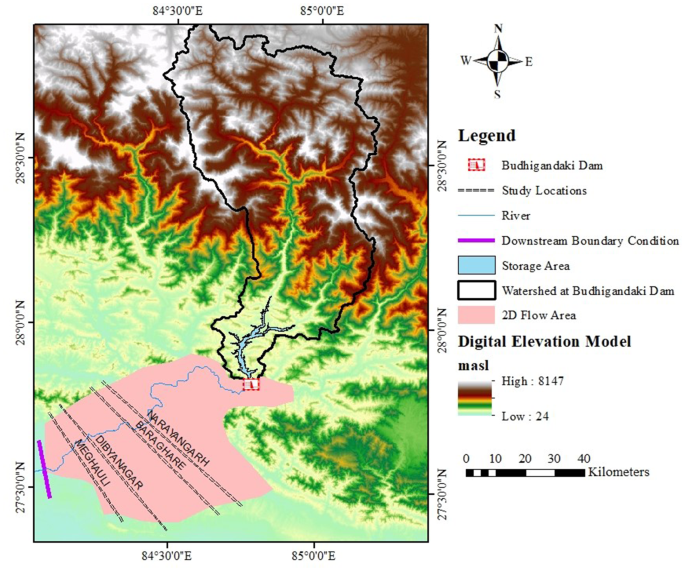
HEC-RAS 2D flow area and model schematic for the flood simulation of Budhigandaki dam breach
Scenarios and sensitivity analysis
In order to quantify the downstream effects of the Budhigandaki dam breach, the following two scenarios have been simulated:
Scenario 1: Dam breach when reservoir is at FSL with PMF. Scenario 2: Dam breach when reservoir is at FSL.
Only overtopping breach mode was analyzed as the dam is made up of concrete and there are less chances of other failure modes (Zhang et al. 2016 ). Moreover, for better understanding the Budhigandaki dam breach mechanism and impacts, sensitivity analysis of the following five important breach parameters as breach bottom elevation, breach bottom width, breach weir coefficient, breach formation time and breach side slope was carried out by varying their values over a reasonable range obtained from literature.
Scenario I have been considered as the base case. Sensitivity of the above-mentioned breach parameters on flood peak discharge, water surface elevation and flood arrival time at the four downstream locations along with inundation area are analyzed considering the base case.
The inputs for the dam break analysis adopted for the base case i.e., Scenario I is listed in the Table 2 . The values of breach parameters have been derived from FERC ( 1993 ), Office of the State Engineer ( 2020 ) and USACE ( 2014 ) specific for concrete dams.
Flood characteristics from 2D simulations
Using RAS Mapper, a series of flood maps were generated based on the outputs of the 2D simulation of the Scenario I dam breach. These maps were helpful in identifying the potentially risky and safe areas. The outputs of the HEC-RAS model were exported to GIS for further analysis and mapping.
Maximum Flood depth map
Using the simulation results, flood inundation maps were prepared illustrating the maximum flood depths across the study area for the different scenarios.
Flood Hazard Vulnerability Map : A flood hazard vulnerability map based on the product of depth and velocity was prepared using the Australian Rainfall-Runoff Guidelines (Australian Rainfall and Runoff 2019 ) which categorize the flood in six zones as: H1 ( D*V ≤ 0.3, D max = 0.3 m, V max = 2.0 m/s, safe for people, vehicles and buildings); H2 ( D*V ≤ 0.6, D max = 0.5 m, V max = 2.0 m/s, unsafe for small vehicles); H3 ( D*V ≤ 0.6, D max = 1.2 m, V max = 2.0 m/s, unsafe for vehicles, children and elderly); H4 ( D*V ≤ 1.0, D max = 2.0 m, V max = 2.0 m/s, unsafe for people and vehicles); H5 ( D*V ≤ 4.0, D max = 4.0 m, V max = 4.0 m/s, unsafe for people and vehicles, buildings vulnerable to structural damage) ; H6 ( D*V > 4.0, unsafe for people and vehicles, all buildings vulnerable to failure) where D and V refer to the flood depth and velocity, respectively while D max and V max refers to the maximum depth and maximum velocity, respectively.
Flood arrival Time Map
Flood arrival time maps represent the computed time (in hours or days) from a specified time in the simulation when the water depth reaches a specified inundation depth. For the case of Budhigandaki dam breach, flood arrival times at the four settlement areas were calculated and mapped.
Estimated values of PMP and PMF
The 1-day PMP value using the 13 precipitation stations was calculated to be 518 mm, 530 mm, 556 mm and 485 mm using Thiessen polygon, Kriging, inverse distance weighted (IDW), and Spline interpolation methods, respectively. As a worst-case scenario, we chose the IDW method, which gave the maximum value of PMP among the four methods, for generating the PMF hydrograph. Using the input data listed in the Appendix 1, ordinates of the synthetic unit hydrograph was computed using Snyder’s method as shown in Fig. 4 .

Synthetic Unit Hydrograph and Probable Maximum Flood Hydrograph for the Budhigandaki dam
From the synthetic unit hydrograph and rainfall intensity duration curve, Direct Runoff Hydrograph was generated. The flood values are generated for a 60-minute interval by linear interpolation between the ordinates of the unit hydrograph. August is the month with the highest flows at the Budhigandaki dam site. Therefore, base flow of 441 m 3 s − 1 which is the mean August flow (during 1964–2012) was added to obtain the final hydrographs (BGHPP Development Committee 2014b ). The final results are plotted in Fig. 4 . I t can be seen that the peak discharge of 11,669 m 3 s − 1 occurs at 33.9 h after the start of rainfall for PMF + base flow.

Flood depth and flood hazard vulnerability
The river valley of 110 km length from Budhigandaki dam to Meghauli was considered for the analysis. The maximum flood depth Fig. 5 shows that the flood depth is as high as 212 m in the upstream area as the river channel is narrow whereas the depth becomes lesser in the downstream river sections where the area is relatively wide and plain. The maximum water depths at Narayangarh is estimated to be 90 m followed by 50.3 m at Baraghare.
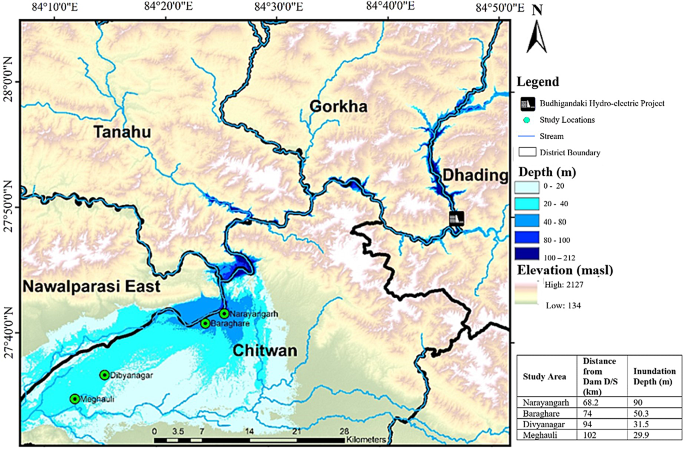
Flood Inundation Map Based on Maximum Depths
Similarly, Flood Hazard Vulnerability Map based on the depth and velocity was prepared as shown in Fig. 6 . It can be identified from the map that all the downstream area lies in H6 zone i.e., unsafe for people and vehicles and all buildings are vulnerable to failure.

Flood Hazard Vulnerability Mapping Based on Depth and Velocity
Flood arrival time
Simulated flood peak arrival times calculated at the four downstream settlement areas are shown in Fig. 7 . It is useful in designing of early warning systems at these locations. It can be seen that the travel times range from 11.3 h (Narayangarh) to 17 h (Meghauli) immediately after the dam breach depending on the proximity from the dam.
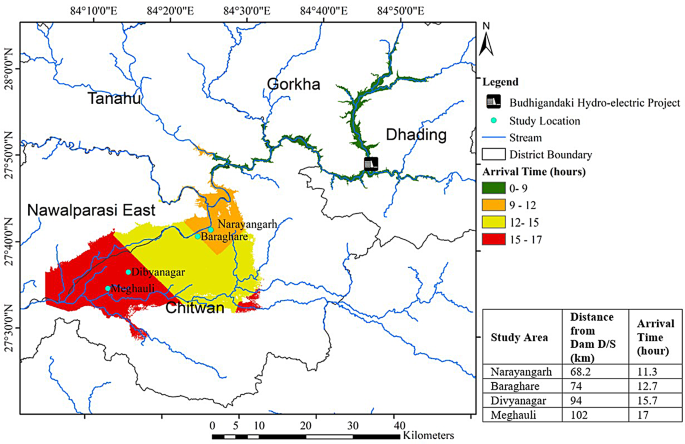
Flood arrival time for the major downstream settlement locations; D/S is downstream
Flood inundation across different land covers
As an impact of dam breach on land cover, it is seen that the inundated type to be most likely inundated is agricultural area (538 km 2 ). Similarly, 239 km 2 of forest is likely to be inundated second in rank. Grassland, water body, barren area, built-up area and shrub land are expected to be inundated with areas of 43 km 2 , 38 km 2 , 25 km 2 , 22 km 2 and 1.5 km 2 respectively as shown in Fig. 8 .
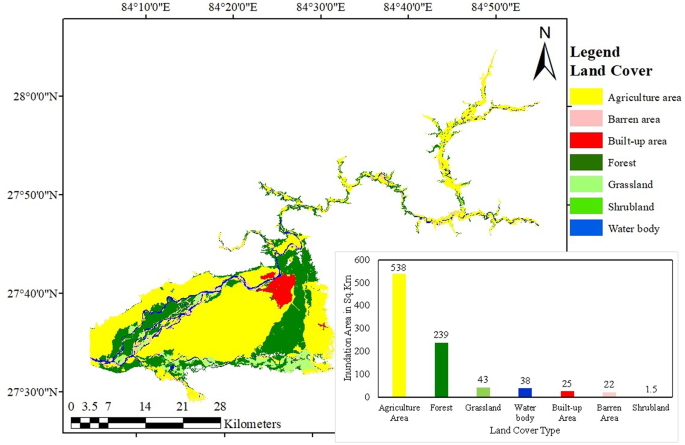
Inundation extent due to dam breach by land cover
Flood Impact on Water Surface Elevation (WSE) and peak discharge
Water surface elevations along the modelled river reach corresponding to the two scenarios are shown in Fig. 9 . It is seen that the water surface is nearly 110 m above the bed level at immediate downstream of the dam site while it is as low as 30 m in the downstream study areas. There is an enormous volume of water flowing down in a very short time because of the breach resulting in such high values of water depths along the river reach. There is very less change in the water surface elevation between Scenario-1 and 2. Also, at the settlement areas, the flow width is large i.e., flat plain area and hence lesser change is seen on the water surface elevation at downstream areas.
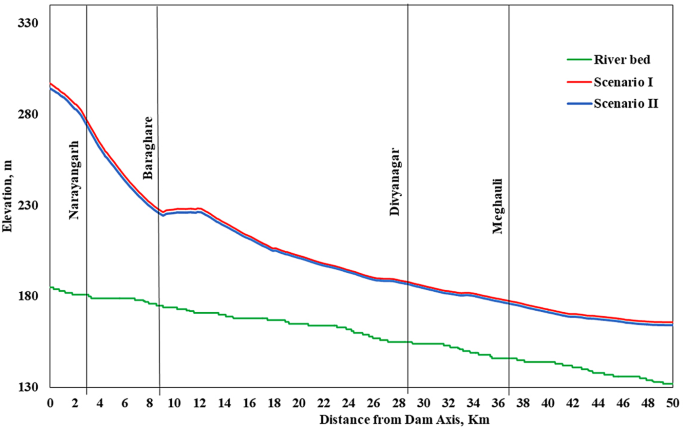
Profile of water surface elevation and river bed for Scenario I and Scenario II. Scenario I: Dam Breach at FSL with PMF and Scenario II: Dam Breach at FSL without PMF
For the two scenarios (Scenario-1 and Scenario-2), the flow hydrographs have been compared at immediate downstream of the dam and at the four major settlement locations as shown in Fig. 10 . It is to be noted that the peak discharge occurs nearly at the same time for both scenarios at all locations. At Narayangarh, peak discharges for Scenarios-1 and 2 are 511,587 m 3 s − 1 and 501,479 m 3 s − 1 respectively i.e., around 2% of difference in the value. Similarly, at Baraghare, the peak discharge for Scenario-1 is 454,267 m 3 s − 1 whereas 441,862 m 3 s − 1 for Scenario-2 and for Divyanagar, the peak discharge for Scenario-1 is 364,697 m 3 s − 1 whereas 357,294 m 3 s − 1 for Scenario II respectively. Lastly for Meghauli, the peak discharge for Scenario-1 is 294,928 m 3 s − 1 whereas 286,813 m 3 s − 1 for Scenario-2. It is obvious that the peak discharge for Scenario-1 is greater than that of Scenario-2, however, the differences in the peak values between the two scenarios are quite small (in the range of 2–3%). This implies that the storage volume of the dam is the major contributor to the flood discharge rather than the PMF.

Comparison of flood hydrographs at major study locations for Scenario I and Scenario II. Scenario I: Dam Breach at FSL with PMF and Scenario II: Dam Breach at FSL without PMF
Flood impact on infrastructure
The possible impact of inundation due to dam breach on buildings and roads was assessed. The total road length includes several types of roads such as highways, feeder roads, district roads and local roads. The inundated highway road length has been computed separately and all other types of roads has been kept as other roads (Table 3 ). It can be seen that Chitwan is the most impacted district with 58.5% of buildings and 2,541 km of road likely to be inundated. Meanwhile, Gorkha is expected to be the least affected district with 2.6% buildings and 132.4 km road inundated. Also, 149,311 numbers of buildings are inundated in total. If the total number of persons on average per household is taken as 4.5 (Cental Bureau of Statisitics 2016 ), a total of about 0.7 million people are likely to be affected by inundation in the case of dam breach. This is about 2.3% of the total population of Nepal.
- Sensitivity analysis
Sensitivity analysis was performed in order to estimate the impact of the breach parameters on the simulated floods in the downstream impacted areas. The values of the input breach parameters were changed within a reasonable range, one at a time, in the dam breach model and the corresponding values of the peak discharge, water surface elevation, flood arrival time and land inundation area were recorded. Breach bottom elevation was varied from 450 masl to 525 masl. Similarly, breach width was varied from 55 m to 150 m and breach weir coefficient was varied from 0.9 to 1.7. Also, breach formation time was varied from 0.05 h to 0.3 h and breach side slope was varied from 0.7:1 to 2.5:1 ( H : V ). Results of the sensitivity analysis have been presented in Table 4 .
Breach bottom elevation
It is seen from Table 4 that as the breach bottom elevation is increased from 450 masl to 525 masl, the value of peak discharge and WSE are significantly decreased at the different downstream locations. It is observed that a 30% increase in breach bottom elevation (450 masl to 475 masl) led to 20–35% decrease in peak discharge, 20–25% decrease in WSE at different downstream locations and nearly 30% decrease in inundation area (893 km 2 to 735 km 2 ). However, the flood peak arrival time is not much altered due to change in breach bottom elevation.
Breach bottom Width
It is seen from Table 4 that an increase in breach width from 55 m to 150 m corresponds to an increase in discharge, WSE and inundation area but the change is not as significant as compared to that of change in breach bottom elevation. A 30% increase in breach width (80 m to 105 m) led to nearly 3% increase in peak discharge at all downstream locations. However, not much change is seen on the WSE, flood arrival time and inundation area due to change in breach bottom width.
Breach weir coefficient
An increase in the breach weir coefficient from 0.9 to 1.7 led to increase in discharge, WSE and inundation area but with a smaller magnitude compared to that of change in breach bottom elevation (Table 4 ). A 20% increase in breach weir coefficient (1.44 to 1.7) led to nearly 3% increase in peak discharge at all downstream locations. Also, no significant change is seen on the WSE, flood arrival time and inundation area due to change in breach weir coefficient.
Breach formation time
Interestingly, there is very insignificant change in peak discharge, WSE, flood arrival time and inundation area due to varying breach formation time (Table 4 ). The values of peak discharge, WSE, flood arrival time and inundation area remain almost unchanged despite the breach formation time is increased up to 200% (0.1 h to 0.3 h).
Breach side slope
A 50% increase in the side slope (1.3:1 to 2:1) led to nearly 2–3% increase in peak discharge as shown in Table 4 . Also, no significant change is seen on the WSE, flood arrival time and inundation area due to change in breach side slope.
Thus, results of the sensitivity analysis varying the values of the breach parameters, namely, dam breach bottom elevation, breach bottom width, breach weir coefficient, breach formation time and breach side slope on the peak discharge, WSE, flood arrival time and downstream inundation area has been summarized in Table 5 . It can be seen that dam breach bottom elevation is the most sensitive parameter with respect to output values such as peak discharge, WSE and downstream inundation area while breach formation time is the least sensitive parameter with respect to all the output parameters.
We have estimated the PMP followed by PMF which is the upstream boundary condition required for the dam breach model in HEC-RAS. The PMP value was chosen as 556 mm from the IDW method. Also, the PMP value as per the detail design report (BGHPP Development Committee 2014b ) is 594 mm. Both the values of PMP are generated using Hershfield formula. However, this slight variation in the PMP values is due to the difference in the values of frequency factor. The value of frequency factor in this study is taken as 15 (Hershfield 1965 ). Subsequently, the PMF value for this study is generated using Snyder’s Unit Hydrograph Method with peak discharge as 11,669 m 3 s − 1 . Besides, by using regional method the PMF was calculated to be 11,479 m 3 s − 1 and regional regression flood analysis method 11,957 m 3 s − 1 (Department of Electricity Development 2006 ). Hence, the PMF values considered in this study are assumed to be reliable.
Impacts of dam breach and sensitivity analysis of dam breach parameters
Simulation results of Scenario I and Scenario II showed that there is a huge peak discharge immediately downstream of the dam breach (Fig. 10 and the difference in discharge values for both scenarios is low. The reason for this is due to the large storage volume of the dam leading to minimum effect of PMF being observed. Also, the downstream tributaries are much smaller compared to the Budhigandaki mainstream river. Hence, their additional impacts on the dam breach flood magnitudes can be considered to be marginal. Additionally, the outputs such as peak discharge, WSE, flood arrival time and inundation area from the dam breach has been estimated as a standalone event. The impact of addition of inflows from the other tributaries (for example, due to localized cloudburst events) to the mainstream river in the downstream settlement area could be areas of further study.
Previous dam breach analysis on Budhigandaki dam has been carried out by Tractebel and jade consult as JV using TELEMAC software (BGHPP Development Committee 2014a ). The output results of the previous study appeared to be quite different from the study carried out using HEC-RAS. There could be various reasons for such discrepancies. The TELEMAC model has considered full dam breach whereas our study does not consider full dam breach. Also, the earlier model has considered high accuracy resolution LiDAR data and other input data (mesh size 30 m*50 m) whereas our study considers 30 m*30 m DEM data and 100 m*100 m mesh size due to model stability issues. However, the pattern of change in peak discharge and WSE at the different study locations are quite similar for both models.
Dam breach analysis has been carried out in different parts of the world using HEC-RAS adopting a methodology similar to ours. For example, simulations of the breach of Batutegi earthen Dam, Indonesia (Wahyudi 2004 ), Mosul earthen Dam, Iraq (Basheer et al. 2017 ) and the results of sensitivity analysis are found out to be quite similar to this study. All these studies showed that dam breach bottom elevation is the most sensitive parameter. Further, the trends in WSE and peak discharge with time and distance from the dam obtained in these studies are also comparable to those of our study. The WSE and peak discharge increased with the increase in the breach parameters as breach bottom elevation, breach bottom width, breach weir coefficient and breach side slope. The peak discharge decreased with increase in breach formation time and negligible change was seen on WSE. Hence, through sensitivity analysis, it is seen that dam breach bottom elevation is the most sensitive parameter while breach formation time is the least sensitive parameter with regards to the floods.
Challenges to flood management
This analysis of a hypothetical dam breach provides insight to the level of possible damage should such a breach occur. Also, it can be deduced from this study that construction of embankments along the river is not a practical mitigation measure because of the extremely high-water depths (nearly 90 m) that these structures need to retain within them. Hence, other non-structural preventive measures such as creating awareness regarding flood risks, community-based flood early warning system (CBFEWS), training and deployment of efficient disaster response teams, zoning of high-risk areas, avoiding construction/settlements in such areas, identification of evacuation centers etc. are recommended. The Yokohama Strategy and Plan of Action (World Conference on Natural Disaster Reduction 1994 ), Hyogo Framework for Action 2005–2015 (International Strategy for Disaster Reduction 2005 ), and the current Sendai Framework for Action 2015–2030 (United Nations 2015 ) highlight the importance of early warning in reducing disaster risk and enhancing the resilience of vulnerable communities. CBFEWS generates and disseminates meaningful and timely flood warnings to vulnerable communities threatened by flood, so they can prepare and act correctly in sufficient time to minimize the possibility of harm. Owing to non-structural measures, the response and adaptation to floods of the vulnerable communities vary widely and are impacted upon by various factors, such as community resilience and susceptibility to flood. Also, the effectiveness of the non-structural measures appears sensitive to the socio-economic changes and governance arrangements (Dawson et al. 2011 ). Nonetheless, non-structural measures provide flexible flood management options for adapting to the ever-changing river basins, socio-economic and climate scenarios, and are in line with the spirit of environment friendly and sustainable development (Shah et al. 2018 ). Also, research on identification of shelter areas and evacuation plan can be an extension of this study using network analysis, buffers and proximity analysis in GIS. Moreover, the sensitivity analysis depicts the most sensitive breach parameters which need to be considered with extreme importance during planning, design, construction and operation of the dam.
Conclusions
This paper simulated the dam breach scenarios of the proposed Budhigandaki dam in central Nepal using HEC-RAS and assessed the impacts on the downstream settlements. Flood peaks, water surface elevations and flood arrival times were calculated for the two scenarios with and without PMF. In addition, sensitivity analysis was carried out to examine the influence of the breach parameters on the flood characteristics.
Results show that the entire downstream area lies in high hazard zone with flood arrival times at Narayangarh, Baraghare, Divyanagar and Meghauli ranges from 11.3 h to 17 h. Moreover, a total of 1,49,311 number of buildings are prone to inundation in the case of dam breach along with 671,900 lives at risk and around 3,500 km stretch of road most likely to be severely damaged. The dam-break flood peak exceeds 650,000 m 3 s − 1 in the immediate downstream of the dam while it attenuates to 511,000 and 286,000 m 3 s − 1 at Narayangarh and Meghauli, respectively. The maximum depth of water ranges from 30 m (in the downstream flat areas) to 212 m (in the upstream steep gorges) clearly discarding the physical and economic feasibility of structural measures for flood management in this case. In addition, 538 km 2 of agricultural land and 25 km 2 of built-up land is at risk of flood inundation. Therefore, it is imperative to implement preventive and non-structural measures such as creating awareness regarding flood risks, developing community-based flood early warning system (CBFEWS), training and deployment of efficient disaster response teams, zoning of high-risk areas, avoiding construction/settlements in such areas, identification of evacuation centers, monitoring and constant auscultation of the structure and developing robust and efficient emergency and alert plans.
Furthermore, the differences in the peak discharges and water surface elevations between the two scenarios are very less at the study locations. This implies that the impact of the huge storage volume of the reservoir on the breach flood characteristics is considerably larger in comparison to the PMF. In addition, change in dam breach bottom elevation was found to be the most sensitive to floods compared to other dam breach parameters.
Additionally, the methodology applied in this study is conveniently replicable of other dams, large or small. However, the simulation run-times may vary depending upon the size of the dam, mesh size, simulation time step and other model complexities. It is to be noted that the case may change for snow fed rivers and glacier lakes. Also, while applying this method to other projects, one should always be careful about the boundary conditions and the initial values of dam breach parameters as they vary depending upon the dam under consideration.
Nepal has currently only one storage dam hydropower project (Kulekhani) in operation. With a greater number of storage projects being planned and under construction, this study could be a useful reference for such future projects. Moreover, this study provides interesting results particularly related to the sensitivity of the breach parameters of concrete arch dams, which could be applicable in study of similar dams in other regions of the world.
Data availability
No datasets were generated or analysed during the current study.
Abbreviations
Catchment Area (km2)
Peak flow coefficient (-)
Lag Coefficient (-)
Coriolis (s − 1 )
acceleration due to gravity (m s − 2 )
water depth (m)
Frequency Factor (-)
main channel length from basin outlet to upstream watershed boundary (km)
main channel length from outlet to a point nearest to centroid of watershed (km)
Mean of Maximum daily rainfall (mm)
Manning’s Coefficient (-)
Specific flow in x-direction (m 2 s − 1 )
Probable maximum precipitation (mm)
Discharge (m 3 s − 1 )
Specific flow in y-direction (m 2 s − 1 )
Unit peak discharge (m 3 s − 1 )
Standard Deviation (mm)
Base time (hours)
Rainfall excess duration time (hours)
Basin Lag time (hours)
Width of unit hydrograph at discharge value exceeded 50% of the peak discharge (hours)
Width of unit hydrograph at discharge value exceeded 75% of the peak discharge (hours)
Surface Elevation (m)
Water Density (kg m − 3 )
Effective Shear Stress (N m − 2 )
Effective Shear Stress along x direction (N m − 2 )
Effective Shear Stress along x and y direction (N m − 2 )
Effective Shear Stress along y direction (N m − 2 )
American Geophysical Union (1938) Reports and Papers, Hydrology
Aureli F, Maranzoni A, Petaccia G (2021) Review of historical dam-break events and laboratory tests on real topography for the validation of numerical models. Water 13(14). https://doi.org/10.3390/w13141968
Australian Rainfall and Runoff (2019) A Guide to Flood Estimation, Book 6 - Flood Hydraulics
Basheer TA, Wayayok A, Yusuf B, Rowshon MK, Basheer TA, Kamal R (2017) Dam breach parameters and their influence on flood hydrographs for Mosul dam. Article J Eng Sci Technol 12(11):2896–2908. https://www.researchgate.net/publication/328475986
Google Scholar
BGHPP Development Committee (2014a) Feasibility Study And Detailed Design of Budhi Gandaki HPP, Vol. 2B: Assessment of Downstream Impacts
BGHPP Development Committee (2014b) Feasibility Study and Detailed Design of Budhigandaki HPP Vol. 2A: Hydrological and Meteorological Analysis
Bricker JD, Schwanghart W, Adhikari BR, Moriguchi S, Roeber V, Giri S (2017) Performance of models for Flash Flood warning and Hazard Assessment: the 2015 Kali Gandaki Landslide Dam Breach in Nepal. Mt Res Dev 37(1):5–15. https://doi.org/10.1659/MRD-JOURNAL-D-16-00043.1
Article Google Scholar
C Froehlich D (1995) Embankment dam breach parameters revisited. Int Water Resour Eng Conf - Proc 1(January 1995):887–891
Cental Bureau of Statisitics (2016) Statistical Pocket Book of Nepal . www.cbs.gov.np
Dawson RJ, Ball T, Werritty J, Werritty A, Hall JW, Roche N (2011) Assessing the effectiveness of non-structural flood management measures in the Thames Estuary under conditions of socio-economic and environmental change. Glob Environ Change 21(2):628–646. https://doi.org/10.1016/j.gloenvcha.2011.01.013
Department of Electricity Development (2006) Design Guidelines for Headworks of Hydropower Projects . https://www.doed.gov.np/pages/guidelines-and-manuals
Dincergok T (2007) The role of dam safety in dam-break induced flood management. International Conference on River Basin Management , 683–691
Ettema R, Baker ME, Teal M, Trojanowski J (2021) Ice-Run Destruction of Spencer Dam on Nebraska’s Niobrara River. CGU HS Committee on River Ice Processes and the Environment, 21st Workshop on the Hydraulics of Ice-Covered Rivers, Saskatoon, Saskatchewan, Canada
Fang CH, Chen J, Duan YH, Xiao K (2017) A new method to quantify breach sizes for the flood risk management of concrete arch dams. J Flood Risk Manag 10(4):511–521. https://doi.org/10.1111/jfr3.12240
Federal Energy Regulatory Commission (FERC) (1993) FERC Engineering Guidelines
FERC (2001) FERC Engineering Guidelines for the Evaluation of Hydropower Projects
Government of Nepal (2020) The Fifteenth Plan (Fiscal Year 2019/20–2023/24) . www.npc.gov.np
Gyawali DR, Devkota LP (2015) Dam Break Analysis using HEC-RAS: A Case Study of Proposed Koshi High Dam. Proceedings of the Seminar on Water and Sustainable Development
Hershfield DM (1965) Method for estimating probable Maximum Precipitation. J Am Waterworks Association 57:965–972
Independent Forensic Team (2022) Final Investigation of Failures of Edenville and Sanford Dams
International Strategy for Disaster Reduction (2005) Hyogo framework for action 2005–2015. World Conference on Disaster Reduction , January 2005 , 508–516. https://doi.org/10.1007/978-1-4020-4399-4_180
Lempérière F (2017) Dams and floods. Elsevier 3(1):144–149. https://doi.org/10.1016/J.ENG.2017.01.018
Leng Q, Zhang M, Zhao G, Mao S, Jiang A (2023) Simulation and Hazard Map of flooding caused by the Break of a concrete gravitational dam. Lecture Notes Civil Eng 264 LNCE:1248–1260. https://doi.org/10.1007/978-981-19-6138-0_109
Nepal Electricity Authority (2022) Nepal Electricity Authority, A Year in Review- Fiscal Year 2021/2022 . www.nea.org.np
Office of the State Engineer (2020) Guidelines for Dam Breach Analysis
Pandey BR, Knoblauch H, Zenz G (2023) Potential dam Breach Flood Hazard Assessment of Kulekhani Reservoir Rock fill dam using 2D diffusion and full dynamic shallow water equation defining Coriolis Effect. Preprints Org. https://doi.org/10.20944/preprints202311.0088.v1
Shah MAR, Rahman A, Chowdhury SH (2018) Challenges for achieving sustainable flood risk management. J Flood Risk Manag 11:S352–S358. https://doi.org/10.1111/jfr3.12211
Shugar DH, Jacquemart M, Shean D, Bhushan S, Upadhyay K, Sattar A, Schwanghart W, McBride S, van Wyk de Vries M, Mergili M, Emmer A, Deschamps-Berger C, McDonnell M, Bhambri R, Allen S, Berthier E, Carrivick JL, Clague JJ, Dokukin M, Westoby MJ (2021) A massive rock and ice avalanche caused the 2021 disaster at Chamoli. Indian Himalaya Sci 373(6552):300–306. https://doi.org/10.1126/science.abh4455
Article CAS Google Scholar
Singh KP, Snorrason A (1984) Sensitivity of outflow peaks and flood stages to the selection of dam breach parameters and simulation models. J Hydrol 68(1–4):295–310. https://doi.org/10.1016/0022-1694(84)90217-8
United Nations (2015) Sendai Framework for Disaster Risk Reduction. In UN World Conference
United States Department of Interior (1988) Downstream Hazard Classification Guidelines. In ACER Technical Memorandum No. 11, Assisstant Commissioner - Engineering and Research, Denver, Colorado
USACE (2014) Using HEC-RAS for Dam Break Studies . www.hec.usace.army.mil
USACE (2018) Hydrologic Engineering Requirements for Reservoirs Engineer Manual
USACE (2024) HEC-RAS River Analysis System HEC-RAS Hydraulic Reference Manual
Vincent E, Emeka OM, Dominic P (2020) Dam and its Failure: A Brief Review of some selected Dams around the World. Adamawa State University Journal of Scientific Research , 8 . http://www.adsujsr.com
Wahyudi E (2004) Dam Break Analysis; A Case Study
World Conference on Natural Disaster Reduction (1994) Yokohama Strategy and Plan of Action for a Safer World: Guidelines for Natural Disaster Prevention, Preparedness and Mitigation. World Conference on Natural Disaster Reduction Yokohama, Japan, 23–27 May 1994 , May 1994 , 23–27
World Meteorological Organization (2009) Manual on estimation of probable maximum precipitation (PMP)
Zhang L, Peng M, Chang D, Xu Y (2016) Statistical Analysis of Failures of Concrete Dams. In Dam Failure Mechanisms and Risk Assessment . https://doi.org/10.1002/9781118558522.ch4
Download references
Acknowledgements
We wish to express my very deepest thanks and gratitude to Mr. Shreeram Shrestha, Civil Engineer, Chilime Hydropower Company Limited, Nepal for his continuous guidance, inspiration and encouragement during the initial preparation of building HEC-RAS model to result interpretation and completion of this study.
This research received no specific grant from any funding agency in the public, commercial, or not-for-profit sectors.
Author information
Authors and affiliations.
Nepal Engineering College, Pokhara University, Bhaktapur, Nepal
Institute for Life Sciences and the Environment, University of Southern Queensland, Toowoomba, Australia
Utsav Bhattarai
Centre for Water Resources Studies, Institute of Engineering, Tribhuvan University, Lalitpur, Nepal
Vishnu Prasad Pandey
Department of Civil Engineering, Pulchowk Campus, Institute of Engineering, Tribhuvan University, Lalitpur, Nepal
Pawan Kumar Bhattarai
You can also search for this author in PubMed Google Scholar
Contributions
A.A. and P.K.B. devised the project, the main conceptual ideas, and the proof outline. A.A. worked out almost all of the technical details, prepared figures, and performed the model analysis for the suggested topics. A.A., P.K.B, and U.B. verified the numerical results. A.A. and V.P.P. interpreted the Results. A.A. with the help of U.B., P.K.B., and V.P.P. wrote the manuscript. U.B., P.K.B., and V.P.P. worked on the discussion of results and commented on the manuscript. A.A. finalizes the manuscript after all the edits.
Corresponding author
Correspondence to Pawan Kumar Bhattarai .
Ethics declarations
Competing interests.
The authors declare no competing interests.
Additional information
Publisher’s note.
Springer Nature remains neutral with regard to jurisdictional claims in published maps and institutional affiliations.
Rights and permissions
Open Access This article is licensed under a Creative Commons Attribution-NonCommercial-NoDerivatives 4.0 International License, which permits any non-commercial use, sharing, distribution and reproduction in any medium or format, as long as you give appropriate credit to the original author(s) and the source, provide a link to the Creative Commons licence, and indicate if you modified the licensed material. You do not have permission under this licence to share adapted material derived from this article or parts of it. The images or other third party material in this article are included in the article’s Creative Commons licence, unless indicated otherwise in a credit line to the material. If material is not included in the article’s Creative Commons licence and your intended use is not permitted by statutory regulation or exceeds the permitted use, you will need to obtain permission directly from the copyright holder. To view a copy of this licence, visit http://creativecommons.org/licenses/by-nc-nd/4.0/ .
Reprints and permissions
About this article
Cite this article.
Awal, A., Bhattarai, U., Pandey, V.P. et al. Downstream impacts of dam breach using HEC-RAS: a case of Budhigandaki concrete arch dam in central Nepal. Environ Syst Res 13 , 37 (2024). https://doi.org/10.1186/s40068-024-00358-3
Download citation
Received : 31 March 2024
Accepted : 16 July 2024
Published : 01 September 2024
DOI : https://doi.org/10.1186/s40068-024-00358-3
Share this article
Anyone you share the following link with will be able to read this content:
Sorry, a shareable link is not currently available for this article.
Provided by the Springer Nature SharedIt content-sharing initiative
- Downstream impacts
- Budhigandaki dam
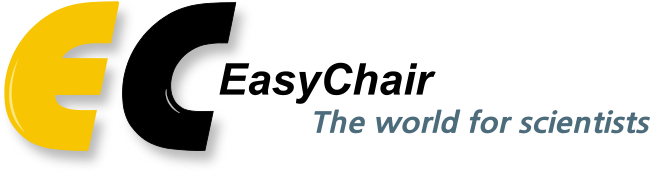
COMMENTS
South Africa, particularly Cape Town, faced severe water scarcity in 2018, leading to the promotion of rainwater harvesting. A successful initiative is the implementation of Greywater Recycling Systems alongside rainwater harvesting in homes, reducing dependence on the municipal water supply. Case study: Mexico - Rainwater harvesting in Mexico City
This article summarizes the practical, theoretical and social aspects of urban rainwater harvesting (RWH) systems, and identifies challenges and opportunities for future research. It covers the benefits, constraints, design, operation, maintenance and acceptance of RWH systems for water conservation and stormwater management.
Learn about the history, benefits, and challenges of rainwater harvesting systems in urban environments. This chapter from a book on sustainable water management covers active and passive systems, environmental and economic assessment, and future research needs.
Domestic Roof-water Harvesting (DRWH) and groundwater recharge provide innovative solution to the inadequate water supply. In this study, a complete RWH technology was designed and constructed for ...
The University of Arizona's College of. Architecture, Planning, and Landscape Architecture (CAPLA), has installed a rainwater. collection system on the 36,000 square-foot building - collecting about 269,000 gallons. of rainwater per year for use within the building (irrigation, water fountains, etc.). The.
Learn about the history, benefits, and design of rainwater harvesting systems for urban areas. This chapter covers rooftop characteristics, storage, treatment, and post-storage water quality, as well as selected case studies and impacts.
A review of practical, theoretical and social aspects of urban rainwater harvesting (RWH) systems, with a global focus. The article explores the benefits, challenges and opportunities of RWH for water conservation, stormwater management and energy consumption.
Community-Scale Rural Drinking Water Supply Systems Based on Harvested Rainwater: A Case Study of Australia and Vietnam. Water 2022, 14 (11) , 1763. ... Building climate resilience in degraded agricultural landscapes through water management: A case study of Bundelkhand region, Central India. Journal of Hydrology 2020, 591 , ...
The review of the literature and the case studies reveal that to realize the full benefits of these systems, one must: maximize and diversify water uses, optimize the design, integrate RWH into an overall sustainable water management plan, and ensure that rainwater harvesting is compared to viable site options as opposed to idealized alternatives.
PDF | On Mar 1, 2014, Nidhi Pasi published Urban Rain water harvesting- case studies from different agroclimatic regions | Find, read and cite all the research you need on ResearchGate
ii. S.N. Kalia (2013) " Rainwater Harvesting - A Case Study Catch Water where it Falls ", Sustainable Water Resources Development and Management, pp. 153-159 iii.
Active water harvesting case study. Underwood Family Sonoran Landscape Laboratory. College of Architecture, Planning, and Landscape Architecture (CAPLA). ... In times of no rain, the cistern distributes its water to the landscape via a drip irrigation system. 11,600-gallon (43,900-liter) cistern (vertical green pastel cylinder in center of ...
Rainwater harvesting has been used throughout history as a water conservation measure, particularly in regions where other water resources are scarce or difficult to access. Rainwater Harvesting: Conservation, Credit, Codes, and Cost Literature Review and Case Studies (pdf) (516.6 KB)
Figures included in the case studies illustrate different elements in the rainwater harvesting systems, from above ground cisterns to underground modular tank systems. The purpose of the case studies is to showcase how rainwater harvesting can be used to promote sustainability and meet project goals. 6. REFERENCES.
This report compiles case studies of rain water harvesting and artificial recharge techniques implemented by Central Ground Water Board (CGWB) and other agencies in different regions of India. It covers the ground water development scenario, the need for artificial recharge, the traditional practices, the planning, the design and the impact of the schemes.
This method is less expensive and very effective and if implemented properly helps in augmenting the ground water level of the area.The methods of rooftop rainwater harvesting are recharge pit, recharge trenches, storage tanks, abandoned dug wells, bore well. The present study tells us 2,87,536 litres ofwater harvested per year infour numbers ...
The rainwater collected from the roof is filtered by two WISY WFF300 vortex filters (see Figure 2), which remove particulates greater than 380 microns from the collected rainwater. The clean water then flows into one of two 29,700-gallon storage tanks, and the debris exiting the filter is carried to the storm drain. The clean water enters
A case study of rain water harvesting (RWH) in urban areas, focusing on the challenges and benefits of RWH in Bangalore, India. The study explores the perceptions, constraints and institutional interventions of RWH users and regulators.
[1] Marlappan M. S. Dr., B.E, Ph.D and Ramesh Mr. S. 2016 A partical Guide on Roof top Rain Water Harvesting 1 (New Delhi) Rain water harvesting guide book.pdf 1-15 M. Mr. Arumugham Kalimutu, B.E, M.Sc Google Scholar [2] Miguel Angel Lopez Zavala C. A. R. and Prieto Monica Jose Cruz 2018 Rainwater harvesting as an alternative for water supply in regions with high water stress Miguel Ángel ...
The objectives of this study are (1) To examine the present status of water requirements for major academic and residential units at South Indian University, (2) Evaluate the potential of storing roof top rainwater in major units of university campus, (3) Propose measures to improve the quality and quantity of rainwater collection.
A Case Study of Rain Water Harvesting. Initiative in Bangalore City. S. Manasi and K. S. Umamani. 1 Introduction. Urbanization and its consequences are challenging to manage as they increase the.
Maximum rainfall in a day is considered for design of harvesting structure. Sample Design: RWH1 for A and B blocks: Roof Area of A and B blocks =702.23m2. Volume of rain water available on roof top of A and B block = 0.02 m x702.23 m2. =14.03 cum RWH dimension = 3m x 2m x 2.5m Model RWH structure (not to scale):
Even though flushing cisterns can be replenished with non-potable rainwater, the water used in these devices has quality requirements . ... A Case Study. Water 2022, 14, 752. [Google Scholar] Alborzfard, N.; Berardi, U. (Un) Sustainable Water Practices in Dormitories. SB13 Dubai Paper-066. 8 December 2013.
The analogy case study method is the use of data from similar cases in another area to assess the impact of climate change on the subject under consideration. Finally, the expert method is the collection of opinions and assessments of research experts on the impact of climate change on the subject under consideration. ... heavy rain, floods ...
Studies on concrete dam breach are limited compared to earthen and other types of dams. With an increase in the construction of concrete dams, particularly in the developing world, it is imperative to have a better understanding of the dam breach phenomena and the identification of the most influential breach parameters. This study aims to contribute to this gap by taking the case of the ...
d Rizvi College of Engineering, Off Carter Road, Mumbai - 400050. [email protected] [email protected]. [email protected]. Abstract: Rain Water harvesting is one of the ...
BibTeX does not have the right entry for preprints. This is a hack for producing the correct reference: @booklet{EasyChair:14743, author = {Zulmira Ximenes da Costa and Keisuke Ikeda and Takumi Nagawaki and Yuichi Nishida and Tamura Satoshi and Floris Cornelis Boogaard}, title = {Classification of Water Quality Index Using Machine Learning Algorithm for Well Assessment: a Case Study in Dili ...
In recent years, realizing groundwater prospects using geospatial technologies gained attention due to their rapid and cost-effective nature for better management. The present study attempts to delineate the groundwater potential zones (GWPZs) of a tropical mountainous river, the Karamana, in southern India, using geographical information system (GIS) and analytical hierarchical process (AHP ...