
An official website of the United States government
The .gov means it’s official. Federal government websites often end in .gov or .mil. Before sharing sensitive information, make sure you’re on a federal government site.
The site is secure. The https:// ensures that you are connecting to the official website and that any information you provide is encrypted and transmitted securely.
- Publications
- Account settings
Preview improvements coming to the PMC website in October 2024. Learn More or Try it out now .
- Advanced Search
- Journal List
- HHS Author Manuscripts


Brain Development and the Role of Experience in the Early Years
Adrienne l. tierney.
Harvard Graduate School of Education
Charles A. Nelson, III
Children’s Hospital Boston Harvard Medical School
Charles A. Nelson III, PhD, is a professor of pediatrics and neuroscience at Harvard Medical School and the Richard David Scott Chair in Pediatric Developmental Medicine Research at Children’s Hospital Boston. A developmental cognitive neuroscientist, Nelson’s interests lie broadly with how experience influences brain development, with a particular interest in the processing of faces and facial expressions. He has conducted research on typically developing infants and children and children who have experienced early biological and psychosocial adversity.
Research over the past several decades has provided insight into the processes that govern early brain development and how those processes contribute to behavior. In the following article, we provide an overview of early brain development beginning with a summary of the prenatal period. We then turn to postnatal development and examine how brain functions are built and how experience mediates this process. Specifically, we discuss findings from research on speech and on face processing. The results of this research highlight how the first few years of life are a particularly important period of development of the brain.
The past 30 years of research have provided a new and deeper understanding of the brain and its role in psychological functions. In particular, researchers now have a better sense of how brain development affects the development of behavior. Measurement techniques such as electroencephalogram (EEG) and event related potentials (ERP) can be used to study infants, children, and adults, and this flexibility has allowed researchers to investigate a variety of developmental processes.
Research using these measures on the developing brain has clarified several arguments about the nature of child development and informed debates such as those surrounding the state of the infant’s brain at birth (whether it is a “blank slate” or not), the identification of critical periods of development, and the relative importance of genes versus environment.
It is important to note that, although much of the research has been conducted on infants, it is a collaborative effort between infant and animal research that has uncovered the neurobiological principles that govern development in humans. Researchers have made use of the homology that exists among developing nervous systems of different species, and many of the cutting-edge ideas discussed in the developmental literature have their origins in animal research—but they have been tested and clarified in neurobehavioral experiments with infants and young children. In humans, researchers can investigate the neural correlates of behavior whereas in animals they can dig deeper into the mechanisms that drive the processes that these neural correlates reflect. To this end, much of human brain research in the past three decades has focused on the brain basis of behavior. A more recent a focus on experience has helped refine researchers’ understanding of how developmental processes are fueled.
In the following paragraphs, we will examine some of the essential ideas that have helped researchers understand the development of the human brain in the early years of life. We begin with an overview of the stages involved in the anatomical development of the brain. Subsequently, we examine three topics that research in brain development has uncovered, clarified, and elaborated: how development is hierarchically structured, such that later development depends on early development; how experience in the first year of life modulates the plasticity of the brain; and how early deprivation has strong and lasting effects on the brain.
Early Stages of Brain Development
An account of brain development in the early years of childhood is only complete if we first examine the origins of this process during the prenatal months. Brain development is a protracted process that begins about 2 weeks after conception and continues into young adulthood 20 years later. Brain development that occurs during the prenatal months is largely under genetic control, although clearly the environment can play a role; for example, it is well known that the lack of nutrition (e.g., folic acid) and the presence of toxins (e.g., alcohol) can both deleteriously influence the developing brain. In contrast, much of brain development that occurs postnatally is experience-dependent and defined by gene–environment interactions. Below we provide brief descriptions of the anatomical changes that characterize the early stages of brain development.
Neurulation
About 2 weeks after conception, the developing embryo has organized itself into a three-layered, spherical structure. In one area of this sphere, the cells thicken to form what is called the neural plate . This plate then folds over onto itself, forming a tube that gradually closes first at the bottom and then at the top, much like a zipper. This creates the neural tube, the inner cells of which will lead to the formation of the central nervous system (brain and spinal cord) while the outer cells will give rise to the autonomic nervous system (nerves outside the brain and spinal cord).
Once the neural tube is closed, it becomes a three-vesicle structure and shortly thereafter a five-vesicle structure. The different regions of tissue around the ventricles will become distinct brain structures. The anterior portion of the tube will become the forebrain , which includes the cerebral hemispheres; the diencephalon (the thalamus and the hypothalamus); and the basal ganglia. The cells around the middle vesicle will become the midbrain , a structure that connects the diencephalon to the hindbrain. The rear-most portion of the tube will give rise to the hindbrain , which will consist of the medulla oblongata, the pons, and the cerebellum. Finally, the cells that remain will give rise to the spinal cord.
Proliferation
Once the general structure of the neural tube has been laid out, the cells that line the innermost part of the tube, called the ventricular zone , proliferate at a logarithmic rate. As these cells multiply, they form a second zone, the marginal zone , which will contain axons and dendrites. This proliferative stage continues for some time, with the consequence that the newborn brain will have many more neurons than the adult brain. The overproduction of neurons is eventually balanced by a process of apoptosis , or programmed cell death. Apoptosis is responsible for a decrease in the cell numbers to adult levels and is completely under genetic control.
Cell migration
After the cells are born, they travel to their final destinations. The cerebral cortex is composed of multilayered tissue several millimeters thick. It is formed by the movement of cells in an inside-out direction, beginning in the ventricular zone and migrating through the intermediate zone, with the cells eventually reaching their final destination on the outside of the developing brain. The earliest migrating cells occupy the deepest cortical layer, whereas the subsequent migrations pass through previously formed layers to form the outer layers. About 25 weeks after conception, all six layers of the cortex will have formed.
The inside-out pattern of migration described here is that of radial migration , which applies to about 70%–80% of migrating neurons, most of which are pyramidal neurons and glia. Pyramidal neurons are the large neurons in the cortex that are responsible for sending signals to different layers of the cortex and other parts of the brain. Glia are nonneuronal brain cells that are involved in the support of neuronal processes (such as producing myelin or removing debris, such as dead brain cells). In contrast, interneurons —relatively smaller neurons that are involved in communication between pyramidal cells within a particular layer of the cortex—follow a pattern of tangential migration.
Differentiation
Once a neuron has migrated to its target destination, it generally proceeds along one of two roads: It can differentiate into a mature neuron, complete with axons and dendrites, or it can be retracted through apoptosis. Current estimates suggest that the number of neurons that are retracted is between 40% and 60% (see Oppenheim & Johnson, 2003 ). The development of axons is facilitated by growth cones , small structures that form at the edge of an axon. The cellular processes that occur at the growth cone promote growth toward certain targets and away from others. Such processes are driven by molecular guidance cues as well as by anatomic structures at the tip of the growth cone.
Dendrite formation occurs by a slightly different process, one that is thought to be driven by genes controlling calcium-regulated transcription factors ( Aizawa et al., 2004 ). Early dendrites appear as thick strands with few spines (small protuberances) that extend from the cell body. As dendrites mature, the number and density of spines increases, which in turn increases the chances that a dendrite will make contact with a neighboring axon. Connections between dendrites and axons are the basis for synaptic connections between neurons, which, as we will describe below, is essential for brain function.
Synaptogenesis
A synapse is a point of contact between two brain cells, often two neurons and frequently a dendrite and an axon. The first synapses are generally observed by about the 23rd week of gestation ( Molliver, Kostovic, & Van der Loos, 1973 ), although the peak of production does not occur until some time in the first year of life. As is the case with neurons, massive overproduction of synapses is followed by a gradual reduction. This process of synapse reduction, or pruning , is highly dependent on experience and serves as the basis of much of the learning that occurs during the early years of life. It is important to note that the various structures of the brain reach their peak of synapse production at different points. In the visual cortex, for example, the peak is reached somewhere between the 4th and 8th postnatal month, but areas of the prefrontal cortex do not reach their peak until the 15th postnatal month. The difference in timing in peak synapse production is important because it affects the timing of the plasticity of these regions; the later the peak synapse production, the longer the region remains plastic.
Synapse pruning
The overproduction of synapses is followed by a pruning back of the unused and overabundance of synapses. Until the stage of synaptogenesis, the stages of brain development are largely gene driven. However, once the brain reaches the point where synapses are eliminated, the balance shifts; the process of pruning is largely experience driven. As with synapse production, the timing of synapse pruning is dependent on the area of the brain in which it occurs. In the parts of the cortex involved in visual and auditory perception, for example, pruning is complete between the 4th and 6th year of life. In contrast, pruning in areas involved in higher cognitive functions (such as inhibitory control and emotion regulation) continues through adolescence ( Huttenlocher & Dabholkar, 1997 ). The processes of overproduction of synapses and subsequent synaptic reduction are essential for the flexibility required for the adaptive capabilities of the developing mind. It allows the individual to respond to the unique environment in which he or she is born. Those pathways that are activated by the environment are strengthened while the ones that go unused are eliminated. In this way, the networks of neurons involved in the development of behavior are fine-tuned and modified as needed.
Myelination
The final process involved in the development of the brain is called myelination . In this process the axons of neurons are wrapped in fatty cells, which ultimately facilitates neuronal activity and communication because this insulation allows myelinated axons to transmit electrical signals faster than unmyelinated axons. The timing of myelination is dependent on the region of the brain in which it occurs. Regions of the brain in certain sensory and motor areas are myelinated earlier in a process that is complete around the preschool period. In contrast, regions involved in higher cognitive abilities, such as the prefrontal cortex, the process is not complete until adolescence or early adulthood (for recent reviews see Nelson, de Haan, & Thomas, 2006 ; Nelson & Jeste, 2008 ).
In general, brain development begins a few weeks after conception and is thought to be complete by early adulthood. The basic structure of the brain is laid down primarily during the prenatal period and early childhood, and the formation and refinement of neural networks continues over the long term. The brains’ many functions do not develop at the same time nor do their developmental patterns follow the same time frame. Although basic sensation and perception systems are fully developed by the time children reach kindergarten age, other systems such as those involved in memory, decision making, and emotion continue to develop well into childhood. The foundations of many of these abilities, however, are constructed during the early years.
The principles of anatomical change described above are essential to the maturation and development of the brain. These processes are in turn responsible for the development of a vast repertoire of behaviors that characterizes the early years of life. In terms of motor development, both synaptic pruning and myelination are responsible for the improved precision and speed of coordinated movement. In addition, they are important in the development of cognitive skills. Improved perception of speech sounds and face recognition, for example, are likely the result of synaptic reorganization, a process that is dependent on experience.
Although development continues into early adult years, early childhood represents a period particularly important to development of a healthy brain. The foundations of sensory and perceptual systems that are critical to language, social behavior, and emotion are formed in the early years and are strongly influenced by experiences during this time. This is not to say that later development cannot affect these behaviors—on the contrary, experiences later in life are also very important to the function of the brain. However, experiences in the early years of childhood affect the development of brain architecture in a way that later experiences do not. In the following pages we will elaborate on how experience affects development between birth and 3 years of age.
Brain Beginnings: Constructing a Foundation for the Future
The development of the brain is a life-long process. Indeed, recent research suggests that the brain is capable of changing throughout the lifespan ( Crawford , Pesch, & von Noorden, 1996 ; Jones, 2000 ; Keuroghlian & Knudsen, 2007 ), although perhaps not in all ways (e.g., humans do not “learn” to see or hear better as they age). However, the changes that take place during the early years are particularly important because they are the bedrock of what comes after. Higher level functions are dependent on lower level functions, the evidence for which is primarily in the basic cognitive processes and sensory perceptual systems. When infants are born, their brains are prepared for certain types of experience. For example, as discussed below, infants’ brains are tuned to the sounds of virtually all languages, but with experience, their brains become most tuned to their native language (see Kuhl, 2004 , for discussion). This perceptual bias is the basis for learning language; the brain is partially tuned to be sensitive to language sounds but not so broadly tuned as to be sensitive to all possible sounds.
Subsequent language development builds on this initial sensitivity. Within the first year of life, infants learn to discriminate among sounds that are specific to the language they are exposed to in their particular environment. Before the time they are 6 months old, infants can discriminate among sounds of almost any language. Between 6 and 12 months, the brain begins to specialize in discriminating sounds of the native language and loses the ability to discriminate sounds in nonnative languages ( Kuhl, Tsao, & Liu, 2003 ). This narrowing of perceptual sensitivity is important because it is related to later language ability in that better discrimination of native language sounds predicts better language skills later in life ( Kuhl, 2004 ).
Sensitive Period: Plasticity Is Affected by Experience
The brain is much more sensitive to experience in the first few years of life than in later years. The plasticity of the brain underlies much of the learning that occurs during this period. In the language example in the previous section, we noted that infants are sensitive to most language sounds in the first half-year of life but during the second half they begin to specialize in their native tongue at the expense of the broad sensitivity to nonnative language sounds. The period of heightened sensitivity to language exposure is not, however, a critical period in the sense that infants can no longer learn the sounds of another language once it is over. In fact, 12-month-old infants given additional experience with speech sounds from a nonnative language continue to be able to discriminate among sounds ( Kuhl, Tsao, & Liu, 2003 ).
Similarly, in the domain of face processing , an index of development of visual perception important to social behavior, 6-month-olds, 9-month-olds, and adults are all equally capable of discriminating between two human faces, whereas 6-month-olds alone can discriminate between two monkey faces ( Pascalis, de Haan, & Nelson, 2002 ). However, 6-month-olds given 3 months of experience viewing a range of monkey faces retain the recognition ability at 9 months ( Pascalis et al., 2005 ). Thus, the plasticity that characterizes brain processes during this time suggests that although the brain is particularly sensitive to experiences that occur, experience-dependent change is not limited to this short window. The sensitive period is effectively extended by specific experience.
A similar phenomenon exists in visual acuity, which is demonstrated by the natural occurrence of cataracts, rather than the laboratory manipulations discussed above. Maurer, Lewis, Brent, and Levin (1999) reported that for infants who are born with cataracts, a few moments of visual experience after the cataracts have been removed and replaced with new lenses leads to substantial improvements in visual acuity. This effect is stronger the sooner after birth this corrective procedure takes place. The longer the cataracts are left untreated, however, the lower the effect of experience on the outcome.
As demonstrated above, both speech and faces are initially processed by a broadly tuned window that then narrows with experience, yet the window can remain broader if experience includes a wide range of inputs. These studies suggest that the early period of life is characterized by sensitive periods that are dependent on the pattern of input from the environment. In response to certain input, the networks become biased, and future modifications become more difficult.
Deprivation: Environmental Effects on Brain Structure and Function
The effects of experience go beyond the simple modulation of plasticity. In fact, experience shapes the structure of the brain, a finding that has been demonstrated by the Bucharest Early Intervention Project (BEIP). This ongoing longitudinal study has found that institutionalization at a young age leads to severe consequences in the development of both brain and behavior. The study is following three groups of children: an Institutionalized group, children who have lived virtually all their lives in an institutional setting in Bucharest, Romania; a Foster Care group, which includes children who were institutionalized at birth and then placed in foster care ( at a mean age of placement of 22 months); and a Never Institutionalized group, which includes children living with their biological families in the Bucharest region (for details see Zeanah et al., 2003 ). As discussed above, for healthy development of brain circuits, the individual needs to have healthy experiences; the lack of these may lead to the underspecification and miswiring of brain circuits. Children raised in institutional settings in Romania lack experiences that stimulate healthy growth and thus we would expect to see consequent “errors” in brain development giving rise to a range of problems. Indeed this is the case; the institutionalized children show patterns of physical and cognitive growth that are stunted and delayed, and they have very different patterns of brain activity when compared to children who have never been institutionalized ( Marshall, Fox, & the BEIP Core Group, 2004 ). In addition, the effect of timing of experience is also important in preventing and amelioriating the effects of deprivation: children who were placed in foster care before they were 2 years old show patterns of brain activity that are more similar to never-institutionalized children than do those placed in foster care after they turn 2 ( Marshall et al., 2008 ). The same general trends are also observed for IQ ( Nelson et al., 2007 ) and language ( Windsor et al., 2007 ). These results support the idea that the lack of good quality experience has detrimental effects on brain function and that once the child is older than 2 years these effects tend to be worse.
An important distinction that must be made here is between deprivation and enrichment . The studies described earlier took place in the context of deprivation, in comparison to a baseline norm in which certain needs of the child are met, not enrichment beyond the norm, and they clearly showed that a child deprived of a certain quality of experience will have abnormal brain development. These findings do not indicate, however, whether environments that provide more than the baseline norm will produce brain development that is in some way superior. So although the BEIP studies do suggest that a lack of good quality experience is detrimental, they do not provide evidence for the effects of enriched experience.
Conclusions
In this article we have attempted to illustrate how the developmental neurosciences can shed light on early childhood development. Prenatal development is largely driven by genetic processes, many of which are sensitive to the biochemical makeup of the mother’s body but are under genetic regulation. In postnatal development, however, the environment plays a crucial role in fostering development, and the interactions between genetics and experiences account for most developmental outcomes. Brain research suggests that development is a hierarchical process of wiring the brain, in that higher level processes build on a foundation of lower level processes. For example, language development depends critically on sensory and perceptual development (e.g., discrimination of speech sounds). The types of stimuli infants and children are exposed to help shape the brain and behavior. Although the brain may come equipped with biases for certain perceptual information, such as for speech, language, or faces, it is the specific speech, language, and range of faces they are exposed to that drives subsequent development. Depriving young children of the kinds of experiences that are essential to later development—that is, the building blocks that create the scaffolding upon which development depends—leads to severe consequences in both brain structure and function. Studies of institutionalized children suggest that quality psychosocial experiences are necessary for the development of a healthy brain.
It is important to emphasize that the individual does not play a passive role in this process. By experience we do not mean events and circumstances that simply happen in an individual’s life; rather, we define experience as the interaction between the individual and his or her environment. The individual is an agent that can shape his or her experience ( Scarr & McCartney, 1983 ). For example, a child who appears happy in response to a caregiver singing a song may elicit more singing. This child consequently may have more experience with songs, which could affect his or her language development and the brain processes that underlie it.
Much of brain research is descriptive and simply tells us how the brain contributes to the development of behavior that is typical of young children (e.g., language and face processing). However, some of this research has implications on the decisions we make for young children. Research on deprivation can be used to make the case that environments that adversely affect infants and young children need to be remedied before they have long lasting consequences on both brain and behavior. Intervening in adverse circumstances is more successful if it occurs before brain processes become entrenched and in turn harder to rewire.
Contributor Information
Adrienne L. Tierney, Harvard Graduate School of Education.
Charles A. Nelson, III, Children’s Hospital Boston Harvard Medical School.
- Aizawa H, Hu S-C, Bobb K, Balakrishnan K, Ince G, Gurevich I, Cowan M, Ghosh A. Dendrite development regulated by CREST, a Calcium-Regulated Transcriptional Activator. Science. 2004; 303 :197–202. [ PubMed ] [ Google Scholar ]
- Crawford ML, Pesch TW, von Noorden GK. Excitatory binocular neurons are lost following prismatic binocular dissociation in infant monkeys. Behavioural Brain Research. 1996; 79 :227–232. [ PubMed ] [ Google Scholar ]
- Huttenlocher PR, Dabholkar AS. Regional differences in synaptogenesis in human cerebral cortex. The Journal of Comparative Neurology. 1997; 387 :167–178. [ PubMed ] [ Google Scholar ]
- Jones EG. Cortical and subcortical contributions to activity-dependent plasticity in primate somatosensory cortex. Annual Review of Neuroscience. 2000; 23 :1–37. [ PubMed ] [ Google Scholar ]
- Keuroghlian AS, Knudsen EI. Adaptive auditory plasticity in developing and adult animals. Progress in Neurobiology. 2007; 82 :109–121. [ PubMed ] [ Google Scholar ]
- Kuhl PK. Early language acquisition: Cracking the speech code. Nature Reviews Neuroscience. 2004; 5 :831–843. [ PubMed ] [ Google Scholar ]
- Kuhl PK, Tsao FM, Liu HM. Foreign-language experience in infancy: Effects of short-term exposure and social interaction on phonetic learning. Proceedings of the National Academy of Sciences. 2003; 100 :9096–9101. [ PMC free article ] [ PubMed ] [ Google Scholar ]
- Marshall P, Fox NA, the BEIP Core Group A comparison of the electroencephalogram between institutionalized and community children in Romania. Journal of Cognitive Neuroscience. 2004; 16 (8):1327–1338. [ PubMed ] [ Google Scholar ]
- Marshall P, Reeb BC, Fox NA, the BEIP Core Group Effects of early intervention on EEG power and coherence in previously institutionalized children in Romania. Development and Psychopathology. 2008; 20 :861–880. [ PMC free article ] [ PubMed ] [ Google Scholar ]
- Maurer D, Lewis TL, Brent HP, Levin AV. Rapid improvement in the acuity of infants after visual input. Science. 1999; 286 :108–110. [ PubMed ] [ Google Scholar ]
- Molliver M, Kostovic I, Van der Loos H. The development of synapses in the human fetus. Brain Research. 1973; 50 :403–407. [ PubMed ] [ Google Scholar ]
- Nelson CA, de Haan M, Thomas KM. Neural bases of cognitive development. In: Damon W, Lerner R, Kuhn D, Siegler R, editors. Handbook of Child Psychology. Vol. 2. John Wiley & Sons; New Jersey: 2006. pp. 3–57. [ Google Scholar ]
- Nelson CA, Jeste S. Neurobiological perspectives on developmental psychopathology. In: Rutter M, Bishop D, Pine D, Scott S, Stevenson J, Taylor E, et al., editors. Textbook on Child and Adolescent Psychiatry. 5th ed Blackwell Publishing; London: 2008. pp. x–y. [ Google Scholar ]
- Nelson CA, Zeanah CH, Fox NA, Marshall PJ, Smyke A, Guthrie D. Cognitive recovery in socially deprived young children: The Bucharest Early Intervention Project. Science. 2007; 318 :1937–1940. [ PubMed ] [ Google Scholar ]
- Oppenheim RW, Johnson JE. Programmed cell death and neurotrophic factors. In: Squire LR, Bloom FE, McConnell SK, Roberts JL, Spitzer NC, Zigmond MJ, editors. Fundamental Neuroscience. 2nd ed Academic Press; New York: 2003. pp. 499–532. [ Google Scholar ]
- Pascalis O, de Haan M, Nelson CA. Is face processing species-specific during the first year of life? Science. 2002; 296 :1321–1323. [ PubMed ] [ Google Scholar ]
- Pascalis O, Scott LS, Kelly DJ, Dufour RW, Shannon RW, Nicholson E, Coleman M, Nelson CA. Plasticity of face processing in infancy. Proceedings of the National Academy of Sciences. 2005; 102 :5297–5300. [ PMC free article ] [ PubMed ] [ Google Scholar ]
- Scarr S, McCartney K. How people make their own environments: A theory of genotype→environment effects. Child Development. 1983; 54 (2):424–435. [ PubMed ] [ Google Scholar ]
- Windsor J, Glaze LE, Koga SF, the BEIP Core Group Language acquisition with limited input: Romanian institution and foster care. Journal of Speech, Language, and Hearing Research. 2007; 50 :1365–1381. [ PubMed ] [ Google Scholar ]
- Zeanah CH, Nelson CA, Fox NA, Smyke AT, Marshall P, Parker SW, Koga S. Designing research to study the effects of institutionalization on brain and behavioral development: The Bucharest Early Intervention Project. Development and Psychopathology. 2003; 15 :885–907. [ PubMed ] [ Google Scholar ]
InBrief: The Science of Early Childhood Development
This brief is part of a series that summarizes essential scientific findings from Center publications.
Content in This Guide
Step 1: why is early childhood important.
- : Brain Hero
- : The Science of ECD (Video)
- You Are Here: The Science of ECD (Text)
Step 2: How Does Early Child Development Happen?
- : 3 Core Concepts in Early Development
- : 8 Things to Remember about Child Development
- : InBrief: The Science of Resilience
Step 3: What Can We Do to Support Child Development?
- : From Best Practices to Breakthrough Impacts
- : 3 Principles to Improve Outcomes
The science of early brain development can inform investments in early childhood. These basic concepts, established over decades of neuroscience and behavioral research, help illustrate why child development—particularly from birth to five years—is a foundation for a prosperous and sustainable society.
Brains are built over time, from the bottom up.
The basic architecture of the brain is constructed through an ongoing process that begins before birth and continues into adulthood. Early experiences affect the quality of that architecture by establishing either a sturdy or a fragile foundation for all of the learning, health and behavior that follow. In the first few years of life, more than 1 million new neural connections are formed every second . After this period of rapid proliferation, connections are reduced through a process called pruning, so that brain circuits become more efficient. Sensory pathways like those for basic vision and hearing are the first to develop, followed by early language skills and higher cognitive functions. Connections proliferate and prune in a prescribed order, with later, more complex brain circuits built upon earlier, simpler circuits.

The interactive influences of genes and experience shape the developing brain.
Scientists now know a major ingredient in this developmental process is the “ serve and return ” relationship between children and their parents and other caregivers in the family or community. Young children naturally reach out for interaction through babbling, facial expressions, and gestures, and adults respond with the same kind of vocalizing and gesturing back at them. In the absence of such responses—or if the responses are unreliable or inappropriate—the brain’s architecture does not form as expected, which can lead to disparities in learning and behavior.
The brain’s capacity for change decreases with age.
The brain is most flexible, or “plastic,” early in life to accommodate a wide range of environments and interactions, but as the maturing brain becomes more specialized to assume more complex functions, it is less capable of reorganizing and adapting to new or unexpected challenges. For example, by the first year, the parts of the brain that differentiate sound are becoming specialized to the language the baby has been exposed to; at the same time, the brain is already starting to lose the ability to recognize different sounds found in other languages. Although the “windows” for language learning and other skills remain open, these brain circuits become increasingly difficult to alter over time. Early plasticity means it’s easier and more effective to influence a baby’s developing brain architecture than to rewire parts of its circuitry in the adult years.
Cognitive, emotional, and social capacities are inextricably intertwined throughout the life course.
The brain is a highly interrelated organ, and its multiple functions operate in a richly coordinated fashion. Emotional well-being and social competence provide a strong foundation for emerging cognitive abilities, and together they are the bricks and mortar that comprise the foundation of human development. The emotional and physical health, social skills, and cognitive-linguistic capacities that emerge in the early years are all important prerequisites for success in school and later in the workplace and community.
Toxic stress damages developing brain architecture, which can lead to lifelong problems in learning, behavior, and physical and mental health.
Scientists now know that chronic, unrelenting stress in early childhood, caused by extreme poverty, repeated abuse, or severe maternal depression, for example, can be toxic to the developing brain. While positive stress (moderate, short-lived physiological responses to uncomfortable experiences) is an important and necessary aspect of healthy development, toxic stress is the strong, unrelieved activation of the body’s stress management system. In the absence of the buffering protection of adult support, toxic stress becomes built into the body by processes that shape the architecture of the developing brain.

Policy Implications
- The basic principles of neuroscience indicate that early preventive intervention will be more efficient and produce more favorable outcomes than remediation later in life.
- A balanced approach to emotional, social, cognitive, and language development will best prepare all children for success in school and later in the workplace and community.
- Supportive relationships and positive learning experiences begin at home but can also be provided through a range of services with proven effectiveness factors. Babies’ brains require stable, caring, interactive relationships with adults — any way or any place they can be provided will benefit healthy brain development.
- Science clearly demonstrates that, in situations where toxic stress is likely, intervening as early as possible is critical to achieving the best outcomes. For children experiencing toxic stress, specialized early interventions are needed to target the cause of the stress and protect the child from its consequences.
Suggested citation: Center on the Developing Child (2007). The Science of Early Childhood Development (InBrief). Retrieved from www.developingchild.harvard.edu .
Related Topics: toxic stress , brain architecture , serve and return
Explore related resources.
- Reports & Working Papers
- Tools & Guides
- Presentations
- Infographics

Videos : Serve & Return Interaction Shapes Brain Circuitry

Reports & Working Papers : From Best Practices to Breakthrough Impacts

Briefs : InBrief: The Science of Neglect

Videos : InBrief: The Science of Neglect

Reports & Working Papers : The Science of Neglect: The Persistent Absence of Responsive Care Disrupts the Developing Brain

Reports & Working Papers : Young Children Develop in an Environment of Relationships

Tools & Guides , Briefs : 5 Steps for Brain-Building Serve and Return

Briefs : 8 Things to Remember about Child Development

Partner Resources : Building Babies’ Brains Through Play: Mini Parenting Master Class

Podcasts : About The Brain Architects Podcast

Videos : FIND: Using Science to Coach Caregivers

Videos : How-to: 5 Steps for Brain-Building Serve and Return

Briefs : How to Support Children (and Yourself) During the COVID-19 Outbreak

Videos : InBrief: The Science of Early Childhood Development

Partner Resources , Tools & Guides : MOOC: The Best Start in Life: Early Childhood Development for Sustainable Development

Presentations : Parenting for Brain Development and Prosperity

Videos : Play in Early Childhood: The Role of Play in Any Setting

Videos : Child Development Core Story

Videos : Science X Design: Three Principles to Improve Outcomes for Children

Podcasts : The Brain Architects Podcast: COVID-19 Special Edition: Self-Care Isn’t Selfish

Podcasts : The Brain Architects Podcast: Serve and Return: Supporting the Foundation
Videos : Three Core Concepts in Early Development

Reports & Working Papers : Three Principles to Improve Outcomes for Children and Families

Partner Resources , Tools & Guides : Training Module: “Talk With Me Baby”

Infographics : What Is COVID-19? And How Does It Relate to Child Development?

Partner Resources , Tools & Guides : Vroom
Brain Development and the Role of Experience in the Early Years
Affiliation.
- 1 Harvard Graduate School of Education.
- PMID: 23894221
- PMCID: PMC3722610
Research over the past several decades has provided insight into the processes that govern early brain development and how those processes contribute to behavior. In the following article, we provide an overview of early brain development beginning with a summary of the prenatal period. We then turn to postnatal development and examine how brain functions are built and how experience mediates this process. Specifically, we discuss findings from research on speech and on face processing. The results of this research highlight how the first few years of life are a particularly important period of development of the brain.
Grants and funding
- R01 MH078829/MH/NIMH NIH HHS/United States
- R01 MH078829-14/MH/NIMH NIH HHS/United States
Thank you for visiting nature.com. You are using a browser version with limited support for CSS. To obtain the best experience, we recommend you use a more up to date browser (or turn off compatibility mode in Internet Explorer). In the meantime, to ensure continued support, we are displaying the site without styles and JavaScript.
- View all journals
- My Account Login
- Explore content
- About the journal
- Publish with us
- Sign up for alerts
- Review Article
- Open access
- Published: 02 June 2022
A methodological perspective on learning in the developing brain
- Anna C. K. van Duijvenvoorde ORCID: orcid.org/0000-0001-9213-8522 1 , 2 ,
- Lucy B. Whitmore ORCID: orcid.org/0000-0002-5672-5081 3 ,
- Bianca Westhoff ORCID: orcid.org/0000-0003-2481-5661 1 , 2 &
- Kathryn L. Mills ORCID: orcid.org/0000-0002-6463-186X 3 , 4
npj Science of Learning volume 7 , Article number: 12 ( 2022 ) Cite this article
4826 Accesses
2 Citations
4 Altmetric
Metrics details
- Cognitive neuroscience
- Human behaviour
The brain undergoes profound development across childhood and adolescence, including continuous changes in brain morphology, connectivity, and functioning that are, in part, dependent on one’s experiences. These neurobiological changes are accompanied by significant changes in children’s and adolescents’ cognitive learning. By drawing from studies in the domains of reading, reinforcement learning, and learning difficulties, we present a brief overview of methodological approaches and research designs that bridge brain- and behavioral research on learning. We argue that ultimately these methods and designs may help to unravel questions such as why learning interventions work, what learning computations change across development, and how learning difficulties are distinct between individuals.
Similar content being viewed by others
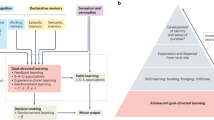
Goal-directed learning in adolescence: neurocognitive development and contextual influences
Linda Wilbrecht & Juliet Y. Davidow
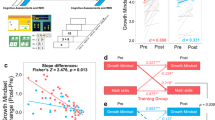
Cognitive training enhances growth mindset in children through plasticity of cortico-striatal circuits
Lang Chen, Hyesang Chang, … Vinod Menon
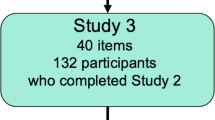
Pathfinder: a gamified measure to integrate general cognitive ability into the biological, medical, and behavioural sciences
Margherita Malanchini, Kaili Rimfeld, … Robert Plomin
Introduction
Childhood and adolescence are considered natural times of learning and adjustment. In the first decades of life, changes in physical appearance, as well as cognitive and social-emotional development, are readily apparent 1 , 2 , 3 , 4 . What is, however, less readily observed are the profound changes in the structure, function, and connectivity of the brain. These changes underlie the development of skills and cognitive processes, for which input from the environment (i.e., experience) is required to fully develop.
Learning, defined as the gain of knowledge or skills through experience, can take on many different forms and can be studied at multiple levels. For instance, cognitive domains of learning—such as learning to read, or the ability to learn through feedback—can be studied together with measures of brain development to eventually inform our understanding of how children and adolescents learn in environments such as school and society. In this review, we discuss three methodological approaches with clear potential for advancing the study of learning in relation to the developing brain.
We will start this review with a concise overview of how the brain is changing over time in structure and function, and the role of plasticity in learning. We will first highlight the opportunities afforded by longitudinal intervention designs, which can inform approaches to understand learning processes during periods of substantial brain development. We then discuss a computational modeling approach that examines how people adjust to their environment based on the processing of positive and negative outcomes. In computational models, learning processes can be broken down into several steps—cognitive computations—that may help to formalize the process of learning and can be linked to underlying brain development. Finally, we highlight the importance of recognizing that developmental patterns differ across individuals when examining learning. As development is not the same for everyone, subgroups of individuals may share learning strategies or even struggles in learning. We will discuss methodological approaches that allow us to study individual differences in learning, and the degree to which these differences in learning are reflected in the developing brain.
Taken together, we present a developmental cognitive neuroscience perspective on child and adolescent learning by summarizing different methodological approaches and their potential combination with brain imaging techniques, such as magnetic resonance imaging (MRI).
Structural and functional brain development
Methods such as MRI have greatly advanced the study of the development of the human brain. The first years of life are characterized by a vast increase in cortical grey matter 5 , which is composed of neural and glial cell bodies, dendritic processes and synapses, as well as blood vessels. This initial overgrowth is followed by a reduction in cortical grey matter volume between roughly ages 8–25 years 6 , 7 . While the resolution of MRI does not allow for us to identify specific cellular changes occurring, the observed reduction in cortical grey matter during childhood and adolescence is thought to, in part, reflect synaptic pruning , in which the brain is cutting back and reorganizing synaptic connections potentially on the basis of how frequently they are used 6 , 7 . This reduction in cortical grey matter is a normative developmental pattern that can vary across individuals and also varies across regions of the cortex 8 , 9 . Another structural brain measure, white matter volume, is composed of the myelinated axons connecting distal regions of the brain and increases until mid-adolescence or early adulthood before it begins to stabilize 6 . This increase in white matter volume reflects, in part, the strengthening of synaptic connections between brain regions 1 , 10 . In short, these changes in the brain result in a more efficient and specialized brain system, with stronger connections between brain regions.
These developmental changes in brain structure are paralleled by changes in brain function and behavior in the affective, cognitive, and social domains 1 , 11 , 12 . Brain function is typically studied in task-based paradigms, examining individuals’ brain responses to a specific event, such as receiving negative feedback or a rewarding outcome. In addition to task-based paradigms, the intrinsic functional connectivity of the brain has been studied during scans called ‘resting-state’. In these scans, participants’ spontaneous brain activation is examined while they are lying in the scanner (but without falling asleep). Resting-state fMRI analyses are designed to probe functional brain connectivity . Functional brain connectivity refers to the correlation of activation signals between different brain regions or networks and is thought to signal to what extent these regions/networks are functionally related. Developmental studies have observed changes in functional connectivity strength, and changes in network interactions 13 . For instance, functional connectivity strength between subcortical and cortical brain regions increased with age 12 , and are tied to changes in reward-sensitivity and learning. Resting-state studies have shown that the brain becomes increasingly ‘modular’ (i.e., functionally segregated) across childhood and adolescence 14 , 15 . An increase in modularity is also seen in structural brain development 16 , and both these functional and structural network-level changes are shown to support the development of, for instance, executive functioning 14 , 16 . Resting-state and task-based studies can thus both provide unique insights into the development of a specific cognitive function or experimental manipulation, as well as the broad network-organization of the brain.
Although not the main focus of this article, when discussing the developing brain and learning, the importance of plasticity (i.e., the brain’s ability to change and adapt as a result of experience) is evident 17 . A typical distinction is made between brain plasticity that is experience-independent , in which brain changes unfolds, relatively independent of experience; brain plasticity that is experience-expectant , in which neural sensitivity is attuned to particular environmental stimuli during specific developmental windows (i.e., sensitive periods); and brain plasticity that is experience-dependent , which reflects experiences and environmental inputs that can vary between individuals and supports learning throughout life 18 . Neuroplasticity, particularly in sensitive periods, is related to molecular processes that can inhibit or stimulate brain plasticity through neurotrophic factors such as brain-derived neurotrophic factor (BDNF) that may result in changes in synaptic and neural pruning 19 .
An example of experience-expectant learning in the brain comes from the development of basic sensory processing regions. Seminal research on visual processing has demonstrated that the development of the visual cortex is dependent on relevant stimulation from the environment. Specifically, depriving visual input to one eye resulted in ocular dominance in the visual cortex of the eye that received input, and an absence of developing binocular vision 20 . Moreover, this work highlighted that the impact of experience on visual cortex development depended on the environmental input in a specific developmental window, referred to as a sensitive period . Sensitive periods are periods of heightened neuroplasticity to specific environmental input. They have been historically studied for the development of the visual neural system, yet are thought to occur across multiple cognitive and social-emotional domains such as language and face processing 17 , 21 . Experience-dependent learning, on the other hand, can be thought of as learning due to practice, exposure, or experience. This type of learning therefore depends on individual’s experiences and can occur at all points of ontogeny. Experience-dependent learning may contribute to brain development by gradually modulating brain connectivity, activation, or structure. The developmental cognitive neuroscience work we will discuss here focuses on experience-dependent learning in the brain across childhood and adolescence.
Learning shapes the developing brain: language
Although brain development may affect the efficiency with which we can learn about the world, or acquire a new skill, learning can also have an impact on brain structure and function at a level that can be measured through MRI, through observed changes in cortical thickness and changes in functional activation during task or at rest. Given that learning in cognitive domains often occurs at the same time as substantial development in both brain structure and function, it is a challenge to differentiate whether the observed changes in the brain reflect experience-independent maturational processes, or experience-dependent learning. In order to specify the areas of the brain that support learning in cognitive domains, we must be able to disentangle if an observed effect is related to the experience and not just reflective of maturational change that might occur in absence of the experience of learning a specific skill.
By taking an example of one skill that has to be explicitly taught in order to be learned, reading, we can begin to unpack these processes. The left arcuate fasciculus and inferior longitudinal fasciculus are white matter tracts that are considered crucial for skilled reading 22 , 23 . The left arcuate fasciculus connects anterior and posterior brain regions (i.e., frontal, parietal, and temporal lobes). The inferior longitudinal fasciculus connects the occipital lobe, important for vision, with the temporal lobe, which is—among others—important for semantics. In general, these two white matter tracts continue to mature during the same time as children develop reading skills across childhood and into adolescence. Longitudinal MRI studies have demonstrated substantial increases in fractional anisotropy (FA) in these two tracts from early childhood and into early adolescence 24 , 25 . This increase in FA is thought to reflect increased integrity of the white matter fibers. This may increase the potential for communication, and thus signaling between the brain regions connected by these tracts. Increases in FA within these tracts have been associated with improvements in reading skill in children 26 , and the rate to which the arcuate fasciculus and inferior longitudinal fasciculus increases in FA across childhood varies by the reading skill of the child 23 . However, in a given MRI study, how can we tell if it is the experience-independent maturational process or the experience of learning that underlies a change in brain measurement between two time points?
Longitudinal intervention designs are one possible method to disentangle maturational and experience-dependent processes in the brain. Although these designs might incur higher costs and burden on participants, repeatedly measuring the same individual over time brings unique opportunities to study ‘true’ development and the influence of experience. Further, the concurrent acquisition of behavioral and neuroimaging measures in these designs allows us to disentangle if change in a brain measure is coupled or uncoupled with change in the behavioral measure of interest. A recent study examined white matter integrity four times in children across the course of an intensive 8-week reading intervention and compared these children to a group of children who did not complete the reading intervention 27 . Participants of the intervention group were recruited based on parent reports of reading difficulties and/or a clinical diagnosis of dyslexia, and the control group was matched for age but not reading level 27 . By taking this longitudinal intervention approach, this study could compare the magnitude of experience-dependent learning (in this case, through a reading intervention) on white matter integrity to the magnitude of developmental change. This study identified the left arcuate fasciculus and inferior longitudinal fasciculus as being responsive to the experience-dependent learning in the cognitive domain of reading, as the observed change in integrity of these white matter paths was coupled to improved reading skill level in the children who received the intervention 27 . In contrast, this study also identified white matter paths that predicted a child’s reading skill level, but did not change in white matter integrity throughout the intervention, such as posterior callosal white-matter connections 27 . This finding suggests that some parts of the brain are already related to readiness to learn certain skills even during a developmental period marked by changes in brain structure.
When looking at evidence of learning on brain measures from another cognitive learning domain—second language acquisition—the age period when one learns a second language can result in differential effects on observed brain measures. For example, cortical thickness measures did not differ between monolinguals and bilingual individuals who acquired two languages simultaneously in early life 28 . However, bilingual individuals who acquired their second language later in childhood show differences in cortical thickness of the inferior frontal gyrus (thicker cortex in the left IFG, thinner cortex in the right IFG), and the magnitude of observed differences correlated with the age of second language acquisition 28 . Thus, multilingual individuals showed no difference in overall cortical thickness as long as they acquired their languages simultaneously, and only individuals who acquired another language later in life showed a difference in cortical thickness. Given that cortical thickness seems sensitive to experiences, such as later language learning, we must consider what differences in overall measures of cortical thickness could actually represent. Perhaps these group-level differences in cortical thickness changes are more likely to reflect the experience-dependent process of learning, which would be more in line with later second language acquisition, than learning that is expected to occur largely early in life. However, to answer this question, one would need longitudinal designs to compare the magnitude of change in cortical thickness observed in individuals who acquired a second language to magnitude of change observed in individuals of the same age who did not.
Using a computational modeling approach in the study of learning
One way in which we learn is by processing and integrating the good and bad outcomes we experience. For instance, through positive and negative feedback, we may learn to play a new videogame, learn to play the guitar, or learn the correct spelling of a difficult word. A computational approach can help us to understand these behavioral changes and formalize the process of outcome-based learning in the developing brain.
Computational learning models have been used to investigate questions such as how children, adolescents, and adults learn from positive and negative outcomes and integrate information into subsequent decision-making. An important element of learning in computational reinforcement learning models focuses on the difference between an expected outcome and a received outcome 29 , 30 . This difference, a so-called prediction error , forms the basis of a specific learning signal that indicates how much one should update expectations of the world, and thereby one’s subsequent actions. These prediction error computations have been linked to brain activation, as this learning signal was found to correlate with dopamine release 31 , 32 that would instigate neural activation. In the context of understanding learning, reinforcement learning models thus provide a computational link (e.g., a prediction-error signal) between brain-level processes and the observed behavior.
Research examining reinforcement learning in children, adolescents, and adults in combination with the developing brain, has shown that learning signals such as prediction errors are found in brain regions, including the striatum, medial prefrontal cortex, and hippocampus. These brain regions are linked to the processing of reward, value, and memory 33 . A number of studies have investigated differences in prediction-error learning in children, adolescents, and adults to understand sensitivities in learning across development. Some findings highlight that adolescents are particularly sensitive to positive prediction errors (feedback that is better than expected), resulting in higher neural activation in adolescents compared to children and adults in the striatum 34 . Moreover, adolescents outperformed adults in their learning performance, and the strength of functional connectivity between the striatum and hippocampus after positive (compared to negative) outcomes related to subsequent memory performance for positive events. These findings indicate that the striatum, and its closely connected regions (see also 35 ), may contribute to heightened reward-learning in some ages, and a bias towards learning from positive outcomes 36 . Note that other studies suggest that the valence-dependency in children’s and adolescent’s learning is context-specific. For instance, it has been observed that adolescents may be more prone to learn from unexpected negative outcomes than adults in other contexts, such as when reward-structures change quickly 37 , 38 .
Computational models may have several advantages for the study of learning 4 , 39 , 40 . A general advantage is that computational models allow to simulate behavior. Generating behavioral data with specific learning parameter settings, allows for better predictions of (expected) behavioral patterns and helps hypothesis testing as well as theory formation. A specific advantage of computational models for brain-behavioral studies is that they allow us to compute latent variables, i.e., variables (such as prediction errors) that cannot be directly observed in behavioral data, but that theory assumes is happening in the brain. These latent variables can be directly linked to brain activation and compared across groups or ages.
Taken together, combining a computational approach with measures of brain functioning allows us to examine learning at different levels of explanation. For instance, on a latent variable level, we can study whether different age groups weigh positive and negative outcomes differently or use different goal-directed strategies in learning 41 , 42 , 43 . On the other hand, developmental change may also occur at the brain (i.e., implementation) level. For instance, learning from experience can involve different brain regions or networks at different ages. Including these levels of explanation in the study of learning can help us to identify mechanisms of learning that may not be apparent, or cannot be disentangled, from observed behavior only.
Examining heterogeneity in the neurocognition of learning
Another challenge in learning research is to characterize individual differences in learning. Most of the studies on learning, or domains of learning, have focused on comparing brain and behavioral differences between ages or condition. This approach is useful for detecting mean-level differences. However, there may be striking heterogeneity in brain development and learning within groups. How could we target those in the study of learning?
An approach that behavioral studies have taken is to use clustering techniques that detect subgroups in the data. For instance, a recent study used such a data-driven approach and has grouped children based on behavioral measures across a range of learning domains, including reading, phonological processing, and executive functioning 44 . This study showed that within a group of 442 struggling learners, three distinct subgroups were found using this range of behavioral indicators. The first group showed symptoms of elevated inattention and hyperactivity/impulsivity. The second group was characterized by learning problems, and the third by aggressive behavior and disturbed peer relations. Moreover, these groups were distinguished by their structural connectivity of the lateral prefrontal cortex, cingulate cortex, and the striatum. In particular, aggression and peer problems loaded on the integration between the prefrontal cortex and the striatum. These findings support the idea that data-driven profiling can distinguish common learning problems in children and provide insight into the neurobiological mechanisms underlying these problems.
Another study from the same group used a clustering technique on white-matter microstructure in a sample of 313 children and adolescents 45 . This analysis showed that the group with higher white matter integrity in the cingulum had profoundly different cognitive abilities. Applying the cingulate-based grouping to independent groups of typically-developing children and struggling learners showed that children with lower cingulum FA showed lower performance across a variety of cognitive performance measures (e.g., fluid intelligence, working memory, and vocabulary) 45 . The value in this approach may particularly relate to children and adolescents with learning difficulties or psychopathologies that show complex behavioral phenotypes that may be better qualified with brain-based than behavioral subtyping.
A recent study compared sub-grouping profiles generated on behavioral measures (e.g., literacy, numeracy, working memory) and structural brain measures (i.e., regional cortical thickness, gyrification, and sulci depth) 46 . This approach was used on a sample of 479 children and adolescents consisting partly of struggling learners. The results based on behavioral measures indicated six cognitive profiles ranging from high- to poor performers on executive function tasks. A similar profile mapping based on structural brain measures indicated that neural and cognitive mappings for individuals were not one-to-one. That is, the same neural profile could be associated with different cognitive impairments in different children 46 . In a subsequent analysis, the authors observed that an individual’s whole-brain network (i.e., the connectome) of white-matter tracts was more strongly related to the cognitive profiles of struggling learners. Particularly, the hub-like structure of individuals’ brain network related to children’s cognitive abilities. Hubs are well-connected nodes in a network and are therefore assumed play an important role in the communication across a network.
Together, these results aid our understanding how the relationship between brain and cognition may be moderated by the organizational properties of developing brain networks. Consequently, it challenges the idea that a neurodevelopmental disorder (such as learning difficulty) is only linked to one specific neuro-anatomical substrate, and instead suggests that learning difficulties are likely to depend on the interactions and organizational properties between different brain systems 47 . These findings also add to the discussion on the transdiagnostic nature of cognitive developmental problems, in which developmental difficulties in learning reflect complex patterns of associations that are not easily matched to singular diagnostic categories.
Note that these reviewed studies identified subgroups using brain and behavioral measures. Although this approach allows to quantify heterogeneity in learning, brain-behavioral relationships can also be studied at the individual level. For instance, finding robust individual-differences markers may help to identify children at risk for developing learning problems. Moreover, questions of heterogeneity in samples have often been tested on cross-sectional datasets with wide age-ranges thereby missing a longitudinal developmental perspective. Finally, these reviewed studies focus on brain structure and not on brain function. Functional brain measures may provide a new mapping for learning profiles, which remains to be explored 47 .
Where do we go from here?
By drawing from research methods and designs in the domains of reading, reinforcement learning, and learning difficulties, we have presented a brief overview of methodological approaches and key findings in developmental cognitive neuroscience research on learning. We started with the central question of how maturational processes can be distinguished from experience-dependent learning. Longitudinal intervention designs are one possible method to examine learning potential and to disentangle maturational and learning-related processes in the brain. Then, we discussed the use of computational modeling for understanding and disentangling the processes that underlie age-related changes in learning from positive and negative outcomes. Computational modeling approaches are rising in developmental studies, and such studies can move this field forward by quantifying the changes in learning processes over age, and their relation to changes in the developing brain. Finally, we discussed handling individual differences using clustering techniques to find data-driven subgroups that may share a commonality in behavioral learning difficulty, or neural patterns of connectivity and/or brain structure. These findings highlight that not all learning brains are the same, and that methods for detecting individual differences are applicable using brain- and behavioral measurements.
Developmental cognitive neuroscience studies have the potential to advance our understanding of learning by combining innovative research methods with longitudinal datasets capturing development from micro (genes, brain) to macro (behavior, environment) levels 48 . That is, (more) rich longitudinal studies are needed to understand learning and learning challenges within individuals, and to address outstanding questions on how interactions between individual characteristics, experience, and environmental influences shape learning across development 26 , 48 , 49 , 50 , 51 . In the methods discussed in this review, the longitudinal element is sometimes central (such as in intervention studies), whereas in other methodological approaches they have yet to be integrated (such as computational modeling approaches). Longitudinal brain research may also help towards better characterization of normative developmental trajectories, and the consequences for functional and structural brain development (see for instance an overview of normative structural development papers 52 ). Eventually, these insights may help in understanding how for instance psychopathology may be explained as a deviation from normative development 53 . Given that longitudinal studies within the field of developmental cognitive neuroscience are time-consuming, valuable, and dependent on long-lasting research funding, the large longitudinal datasets that are becoming increasingly accessible (e.g., 54 , 55 , 56 , 57 ) will be important for advancing the field and examine the neurodevelopmental changes of learning.
In this review, we focused predominantly on individual learning. However, learning obviously does not happen in a vacuum, and humans learn the vast majority of their knowledge from other humans or are influenced by the social context of learning. Learning in a social context is hugely complex and encompasses interactions between learning and the regulatory demands of a social context (e.g., distraction by others), motivational processes (e.g., the desire to interact and engage with others), and our experience with others (e.g., learned trustworthiness of others). As such, an individual’s social context can impact the rate of learning, as well as what is learned in a given situation depending on the developmental period. For instance, reinforcement learning models have been used to study how we update our expectations about others across development and how this is distinct from non-social learning (e.g., 58 , 59 , 60 , 61 , 62 , 63 ). Although the social context of learning warrants a review article of itself, we do want to highlight that the methods displayed in the current review are also valuable to apply to research that studies learning in a social context.
Taken together, the study of behavioral learning can benefit from both structural and functional MRI research. We discussed methodological approaches that aim to unravel why learning interventions work, what learning computations change across development, and how learning difficulties are distinct between subgroups of individuals. These corresponding findings indicate that these approaches have the potential to have a lasting impact on promoting children’s and adolescents’ positive development.
Reporting summary
Further information on research design is available in the Nature Research Reporting Summary linked to this article.
Data availability
No datasets were generated or analyzed during the current study.
Crone, E. A. & Dahl, R. E. Understanding adolescence as a period of social–affective engagement and goal flexibility. Nat. Rev. Neurosci. 13 , 636–650 (2012).
Article CAS PubMed Google Scholar
Dahl, R. E., Allen, N. B., Wilbrecht, L. & Suleiman, A. B. Importance of investing in adolescence from a developmental science perspective. Nature 554 , 441–450 (2018).
Crone, E. A. & Richard Ridderinkhof, K. The developing brain: From theory to neuroimaging and back. Dev. Cogn. Neurosci. 1 , 101–109 (2011).
Article PubMed Google Scholar
van Duijvenvoorde, A. C. K., Peters, S., Braams, B. R. & Crone, E. A. What motivates adolescents? Neural responses to rewards and their influence on adolescents’ risk taking, learning, and cognitive control. Neurosci. Biobehav. Rev. 70 , 135–147 (2016).
Gilmore, J. H., Knickmeyer, R. C. & Gao, W. Imaging structural and functional brain development in early childhood. Nat. Rev. Neurosci. 19 , 123–137 (2018).
Article CAS PubMed PubMed Central Google Scholar
Mills, K. L. et al. Structural brain development between childhood and adulthood: Convergence across four longitudinal samples. NeuroImage 141 , 273–281 (2016).
Tamnes, C. K. et al. Development of the cerebral cortex across adolescence: a multisample study of inter-related longitudinal changes in cortical volume, surface area, and thickness. J. Neurosci. 37 , 3402–3412 (2017).
Vijayakumar, N. et al. Brain development during adolescence: A mixed-longitudinal investigation of cortical thickness, surface area, and volume. Hum. Brain Mapp. 37 , 2027–2038 (2016).
Article PubMed PubMed Central Google Scholar
Mills, K. L. et al. Inter-individual variability in structural brain development from late childhood to young adulthood. NeuroImage 242 , 118450 (2021).
Vijayakumar, N., Op de Macks, Z., Shirtcliff, E. A. & Pfeifer, J. H. Puberty and the human brain: Insights into adolescent development. Neurosci. Biobehav. Rev. 92 , 417–436 (2018).
van Duijvenvoorde, A. C. K., Achterberg, M., Braams, B. R., Peters, S. & Crone, E. A. Testing a dual-systems model of adolescent brain development using resting-state connectivity analyses. NeuroImage 124 , 409–420 (2016).
van Duijvenvoorde, A. C. K., Westhoff, B., Vos, F., Wierenga, L. M. & Crone, E. A. A three‐wave longitudinal study of subcortical–cortical resting‐state connectivity in adolescence: Testing age‐ and puberty‐related changes. Hum. Brain Mapp . hbm.24630, https://doi.org/10.1002/hbm.24630 (2019).
Ernst, M., Torrisi, S., Balderston, N., Grillon, C. & Hale, E. A. fMRI functional connectivity applied to adolescent neurodevelopment. Annu. Rev. Clin. Psychol. 11 , 361–377 (2015).
Gu, S. et al. Emergence of system roles in normative neurodevelopment. Proc. Natl Acad. Sci. 112 , 13681–13686 (2015).
Gu, S. et al. Unifying the notions of modularity and core–periphery structure in functional brain networks during youth. Cereb. Cortex 30 , 1087–1102 (2020).
Baum, G. L. et al. Modular segregation of structural brain networks supports the development of executive function in youth. Curr. Biol. 27 , 1561–1572.e8 (2017).
Hartley, C. A. & Frankenhuis, W. E. Editorial overview: Sensitive and critical periods. Curr. Opin. Behav. Sci. 36 , iii–v (2020).
Article Google Scholar
Greenough, W. T., Black, J. E. & Wallace, C. S. Experience and brain development. Child Dev. 58 , 539–559 (1987).
Takesian, A. E. & Hensch, T. K. Balancing plasticity/stability across brain development. in Prog. Brain Res. 207 3–34 (Elsevier, 2013).
Hubel, D. H. & Wiesel, T. N. Receptive fields, binocular interaction and functional architecture in the cat’s visual cortex. J. Physiol. 160 , 106–154.2 (1962).
Kievit, R. A. Sensitive periods in cognitive development: a mutualistic perspective. Curr. Opin. Behav. Sci. 36 , 144–149 (2020).
Grotheer, M., Zhen, Z., Lerma-Usabiaga, G. & Grill-Spector, K. Separate lanes for adding and reading in the white matter highways of the human brain. Nat. Commun. 10 , 3675 (2019).
Yeatman, J. D., Dougherty, R. F., Ben-Shachar, M. & Wandell, B. A. Development of white matter and reading skills. Proc. Natl Acad. Sci. 109 , E3045–E3053 (2012).
Krogsrud, S. K. et al. Changes in white matter microstructure in the developing brain—A longitudinal diffusion tensor imaging study of children from 4 to 11years of age. NeuroImage 124 , 473–486 (2016).
Reynolds, J. E., Grohs, M. N., Dewey, D. & Lebel, C. Global and regional white matter development in early childhood. NeuroImage 196 , 49–58 (2019).
Chyl, K., Fraga-González, G., Brem, S. & Jednoróg, K. Brain dynamics of (a)typical reading development—a review of longitudinal studies. Npj Sci. Learn 6 , 4 (2021).
Huber, E., Donnelly, P. M., Rokem, A. & Yeatman, J. D. Rapid and widespread white matter plasticity during an intensive reading intervention. Nat. Commun. 9 , 2260 (2018).
Klein, D., Mok, K., Chen, J.-K. & Watkins, K. E. Age of language learning shapes brain structure: a cortical thickness study of bilingual and monolingual individuals. Brain Lang. 131 , 20–24 (2014).
Sutton, R. S. & Barto, A. G. Toward a modern theory of adaptive networks: Expectation and prediction. Psychol. Rev. 88 , 135–170 (1981).
Sutton, R. S. & Barto, A. G. Reinforcement Learning: An Introduction. vol. 2 (MIT Press, 1998).
Montague, P. R., Dayan, P. & Sejnowski, T. J. A framework for mesencephalic dopamine systems based on predictive Hebbian learning. J. Neurosci. 16 , 1936–1947 (1996).
Schultz, W., Dayan, P. & Montague, P. R. A neural substrate of prediction and reward. Science 275 , 1593–1599 (1997).
DePasque, S. & Galván, A. Frontostriatal development and probabilistic reinforcement learning during adolescence. Neurobiol. Learn. Mem. 143 , 1–7 (2017).
Cohen, J. R. et al. A unique adolescent response to reward prediction errors. Nat. Neurosci. 13 , 669–671 (2010).
van den Bos, W., Cohen, M. X., Kahnt, T. & Crone, E. A. Striatum–medial prefrontal cortex connectivity predicts developmental changes in reinforcement learning. Cereb. Cortex 22 , 1247–1255 (2012).
van Duijvenvoorde, A. C. K., Zanolie, K., Rombouts, S. A. R. B., Raijmakers, M. E. J. & Crone, E. A. Evaluating the negative or valuing the positive? Neural mechanisms supporting feedback-based learning across development. J. Neurosci. 28 , 9495–9503 (2008).
Hauser, T. U., Iannaccone, R., Walitza, S., Brandeis, D. & Brem, S. Cognitive flexibility in adolescence: Neural and behavioral mechanisms of reward prediction error processing in adaptive decision making during development. NeuroImage 104 , 347–354 (2015).
van der Schaaf, M. E., Warmerdam, E., Crone, E. A. & Cools, R. Distinct linear and non-linear trajectories of reward and punishment reversal learning during development: Relevance for dopamine’s role in adolescent decision making. Dev. Cogn. Neurosci. 1 , 578–590 (2011).
van den Bos, W., Bruckner, R., Nassar, M. R., Mata, R. & Eppinger, B. Computational neuroscience across the lifespan: Promises and pitfalls. Dev. Cogn. Neurosci. 33 , 42–53 (2018).
Wilson, R. C. & Collins, A. G. Ten simple rules for the computational modeling of behavioral data. eLife 8 , e49547 (2019).
Eppinger, B., Walter, M., Heekeren, H. & Li, S.-C. Of goals and habits: age-related and individual differences in goal-directed decision-making. Front. Neurosci. 7 , 253 (2013).
Nussenbaum, K., Scheuplein, M., Phaneuf, C. V., Evans, M. D. & Hartley, C. A. Moving developmental research online: comparing in-lab and web-based studies of model-based reinforcement learning. Collabra Psychol. 6 , 17213 (2020).
Decker, J. H., Otto, A. R., Daw, N. D. & Hartley, C. A. From creatures of habit to goal-directed learners. Psychol. Sci. 27 , 848–858 (2016).
Bathelt, J., Holmes, J., The CALM Team & Astle, D. E. Data-driven subtyping of executive function–related behavioral problems in children. J. Am. Acad. Child Adolesc. Psychiatry 57 , 252–262.e4 (2018).
Bathelt, J., Johnson, A., Zhang, M. & Astle, D. E. The cingulum as a marker of individual differences in neurocognitive development. Sci. Rep. 9 , 2281 (2019).
Siugzdaite, R., Bathelt, J., Holmes, J. & Astle, D. E. Transdiagnostic brain mapping in developmental disorders. Curr. Biol. 30 , 1245–1257.e4 (2020).
Thomas, M. S. C. Developmental disorders: few specific disorders and no specific brain regions. Curr. Biol. 30 , R304–R306 (2020).
van Atteveldt, N., Vandermosten, M., Weeda, W. & Bonte, M. How to capture developmental brain dynamics: gaps and solutions. Npj Sci. Learn 6 , 10 (2021).
Crone, E. A. & Elzinga, B. M. Changing brains: how longitudinal functional magnetic resonance imaging studies can inform us about cognitive and social-affective growth trajectories: Changing brains: how longitudinal fMRI studies. Wiley Interdiscip. Rev. Cogn. Sci. 6 , 53–63 (2015).
Telzer, E. H. et al. Methodological considerations for developmental longitudinal fMRI research. Dev. Cogn. Neurosci. 33 , 149–160 (2018).
Mills, K. L. & Tamnes, C. K. Methods and considerations for longitudinal structural brain imaging analysis across development. Dev. Cogn. Neurosci. 9 , 172–190 (2014).
Vijayakumar, N., Mills, K. L., Alexander-Bloch, A., Tamnes, C. K. & Whittle, S. Structural brain development: A review of methodological approaches and best practices. Dev. Cogn. Neurosci. 33 , 129–148 (2018).
Marquand, A. F. et al. Conceptualizing mental disorders as deviations from normative functioning. Mol. Psychiatry 24 , 1415–1424 (2019).
Casey, B. J. et al. The Adolescent Brain Cognitive Development (ABCD) study: Imaging acquisition across 21 sites. Dev. Cogn. Neurosci. 32 , 43–54 (2018).
Kemner, C. et al. Teaming up to understand individual development. Dev. Cogn. Neurosci . 100910 (2021) https://doi.org/10.1016/j.dcn.2021.100910 .
Kooijman, M. N. et al. The Generation R Study: design and cohort update 2017. Eur. J. Epidemiol. 31 , 1243–1264 (2016).
Kievit, R. A., McCormick, E. M., Fuhrmann, D., Deserno, M. K. & Orben, A. Using large, publicly available data sets to study adolescent development: opportunities and challenges. Curr. Opin. Psychol. 44 , 303–308 (2022).
Bolenz, F., Reiter, A. M. F. & Eppinger, B. Developmental changes in learning: computational mechanisms and social influences. Front. Psychol. 8 , 2048 (2017).
Ciranka, S. & van den Bos, W. Social influence in adolescent decision-making: a formal framework. Front. Psychol . 10 , (2019).
Ma, I., Westhoff, B. & van Duijvenvoorde, A. C. K. Uncertainty about other’s trustworthiness increases during adolescence and guides social information sampling. Sci. Rep. 12 , 7634 (2022).
Westhoff, B., Molleman, L., Viding, E., van den Bos, W. & van Duijvenvoorde, A. C. K. Developmental asymmetries in learning to adjust to cooperative and uncooperative environments. Sci. Rep. 10 , 21761 (2020).
Lockwood, P. L., Apps, M. A. J. & Chang, S. W. C. Is There a ‘Social’ Brain? Implementations and Algorithms. Trends Cogn. Sci. 24 , 802–813 (2020).
Westhoff, B., Blankenstein, N. E., Schreuders, E., Crone, E. A. & van Duijvenvoorde, A. C. K. Increased ventromedial prefrontal cortex activity in adolescence benefits prosocial reinforcement learning. Dev. Cogn. Neurosci. 52 , 101018 (2021).
Download references
Acknowledgements
K.L.M. was supported by the Research Council of Norway, grant number 288083. A.C.K.D. and B.W. were supported by an Open Research Area (ORA) grant, grant number 464-15-176, financed by the Netherlands Organization for Scientific Research (NWO). A.C.K.D. was supported by the Gratama Foundation and the Leiden University Fund. We thank Marieke G.N. Bos and Kiki Zanolie for carefully reviewing the manuscript.
Author information
Authors and affiliations.
Institute of Psychology, Leiden University, Leiden, The Netherlands
Anna C. K. van Duijvenvoorde & Bianca Westhoff
Leiden Institute for Brain & Cognition, Leiden University, Leiden, The Netherlands
Department of Psychology, University of Oregon, Eugene, OR, USA
Lucy B. Whitmore & Kathryn L. Mills
PROMENTA Research Center, Department of Psychology, University of Oslo, Oslo, Norway
Kathryn L. Mills
You can also search for this author in PubMed Google Scholar
Contributions
K.M and A.C.K.D. wrote the manuscript. L.W. and B.W. provided critical input, revisions, and contributed to the interpretation of the reviewed findings. All authors contributed extensively to the work presented in this paper.
Corresponding author
Correspondence to Anna C. K. van Duijvenvoorde .
Ethics declarations
Competing interests.
All authors declare no competing interests.
Additional information
Publisher’s note Springer Nature remains neutral with regard to jurisdictional claims in published maps and institutional affiliations.
Supplementary information
Reporting summary checklist, rights and permissions.
Open Access This article is licensed under a Creative Commons Attribution 4.0 International License, which permits use, sharing, adaptation, distribution and reproduction in any medium or format, as long as you give appropriate credit to the original author(s) and the source, provide a link to the Creative Commons license, and indicate if changes were made. The images or other third party material in this article are included in the article’s Creative Commons license, unless indicated otherwise in a credit line to the material. If material is not included in the article’s Creative Commons license and your intended use is not permitted by statutory regulation or exceeds the permitted use, you will need to obtain permission directly from the copyright holder. To view a copy of this license, visit http://creativecommons.org/licenses/by/4.0/ .
Reprints and permissions
About this article
Cite this article.
van Duijvenvoorde, A.C.K., Whitmore, L.B., Westhoff, B. et al. A methodological perspective on learning in the developing brain. npj Sci. Learn. 7 , 12 (2022). https://doi.org/10.1038/s41539-022-00127-w
Download citation
Received : 31 July 2020
Accepted : 05 May 2022
Published : 02 June 2022
DOI : https://doi.org/10.1038/s41539-022-00127-w
Share this article
Anyone you share the following link with will be able to read this content:
Sorry, a shareable link is not currently available for this article.
Provided by the Springer Nature SharedIt content-sharing initiative
This article is cited by
Editorial: capturing developmental brain dynamics.
- Milene Bonte
- Nienke van Atteveldt
npj Science of Learning (2022)
Quick links
- Explore articles by subject
- Guide to authors
- Editorial policies
Sign up for the Nature Briefing newsletter — what matters in science, free to your inbox daily.

Cookies on this website
We use cookies to ensure that we give you the best experience on our website. If you click 'Accept all cookies' we'll assume that you are happy to receive all cookies and you won't see this message again. If you click 'Reject all non-essential cookies' only necessary cookies providing core functionality such as security, network management, and accessibility will be enabled. Click 'Find out more' for information on how to change your cookie settings.

- Accessibility
- Knowledge Base
New Research Highlights Importance of Early Years Development on Future Wellbeing
Child and Adolescent Psychiatry Early intervention Mental Health
25 November 2021
Oxford researchers involved nearly 4,000 children across the UK in three specially developed science lessons to educate pupils about brain development during early childhood. The SEEN (Secondary Education around Early Neurodevelopment) project was commissioned and funded by KindredSquared and is part of a wider drive to increase public understanding of how early experiences can shape the adults we become.
Dr Elizabeth Rapa , Co-investigator of SEEN and Senior Scientist, University of Oxford, said:
'This ground-breaking project could improve the lives of children for generations. In the same way that we teach children about the risks of smoking or poor diet, children also need to know about why experiences in our early years are so important for later health. We hope that the key principles of early child development into KS3/4 (aged 11-14) will now be taught in more schools.'
Just one in four (24%) adults recognise the specific importance of the first five years of life for providing lifelong health and happiness. This programme of work from the University of Oxford aims to increase public understanding of how the early experiences of babies and children can influence long-term mental and physical health. The ways in which we talk to babies, encourage learning through play and how to strengthen resilience are all important in early development.
The programme engaged 11-14-year-olds in three science lessons, which taught the neuroscience of brain development, and what that means in terms of how a child grows and develops. Evaluation of the project showed that after the lessons:
- 86% of children could give a practical example of what they could do to maximise a child's development through everyday activities or play
- Over 90% of pupils knew how a caregiver should speak to a baby to promote their language development
- 80% understood that a child's environment affects their development
- 80% correctly reported that a child's brain develops fastest in the first 5 years of life
Her Royal Highness, The Duchess of Cambridge, visited Nower Hill High School in Harrow, North London, and spoke to pupils about their experiences of taking part in the lessons and how this has impacted on their understanding of early years development.

©Kensington Palace
Pupils said:
'I told my mum what we learnt about in the lesson like what stages a baby's brain develops the most and how playing games or talking with exaggerated facial expressions helps with serve and return interactions, memory and good brain development.'
'Baby talk is more than just playing, it is an important thing to baby's brain development.'
Crucially more than 40 teachers from across the 21 schools which took part in the project were unanimous in their view that brain development during early childhood should be taught in schools.
One teacher whose class took part in the SEEN lessons, said:
'This is a scientific topic that can have multiple applications to real life, students are a lot more engaged when they can see how something relates to them in their life, it has meaning.'
Dr Louise Dalton , Co-investigator of SEEN and Consultant Clinical Psychologist, University of Oxford, said:
'The change we have seen in children's understanding of how to promote children's development is astonishing, particularly in such a short period of time. The positive impact of the teaching programme is exciting to see, and the potential to easily scale up the programme to reach all children across the country, instilling this core knowledge in carers and parents of the future is so important.'
Felicity Gillespie, Director of Kindred 2 , said:
'The most significant levelling up that's needed in the country is to rethink our perception of early years. A child's development at 22 months serves as a strong predictor of education outcomes at age 26. Most of the human brain is developed before we can even talk and in the first year of life, the brain literally doubles in size. The evidence of the massive impact our earliest relationships, environments and experiences has on our future development is incontrovertible. This includes helping pupils understand that how a parent interacts with their baby impacts on their future potential. Working with 11-14-year-olds to educate them and to transform their own understanding early enough in their lives, means that we are not leaving these lessons too late. The hope is that the striking results of this programme will now be replicated in more secondary schools across the country.'
The school visit event was followed by a meeting of Chief Executives of Academy Trusts at Buckingham Palace, where the implications of the research for schools and young people were discussed. It was chaired by Felicity Gillespie, Kindred 2 , the commissioner and funder of the SEEN project. Louise Aukland, SEEN project lead, presented results of the research and the experiences of teachers and students in another of the participating schools, James Hornsby School, Basildon.
For more information about SEEN: Secondary Education around Early Neurodevelopment contact Dr Elizabeth Rapa and Dr Louise Dalton .
This research is funded by Kindred 2 , the early years charitable foundation.
NIHR OXFORD HEALTH BIOMEDICAL RESEARCH CENTRE NEWS
Please follow the link below to read the news on the NIHR BRC website.
Similar stories
Alcohol and drug overdose a leading cause of death in people recently released from prison, the importance of talking to children about serious illness, new mental health toolkit available for oxford students on university mental health day, professor rachel upthegrove appointed director designate of the oxford health brc, new online tool for child anxiety could expand access to child mental health services, next step in plans for warneford park.
One of the largest-ever early-brain development studies has just launched
- Feinberg School of Medicine
- Mental Health
- School of Communication
Pregnancy and the first few years of life are a period of exponential brain growth and development. The unprecedented convergence of the opioid epidemic and COVID-19 pandemic provides a unique opportunity to shed light on how these early exposures during pregnancy may shape young children’s development and functioning at the population level.
In the largest, most diverse and comprehensive study of its kind, Northwestern University, along with 24 other research sites across the United States and in collaboration with the Ann & Robert H. Lurie Children’s Hospital of Chicago, has embarked on a longitudinal study to assess how exposures to substances and environments before and after birth may alter early brain development.
Funded by 10 institutes and offices at the National Institutes of Health, and the Helping to End Addiction Long-term Initiative, or NIH HEAL Initiative , and led by the National Institute on Drug Abuse (NIDA), the HEALthy Brain and Child Development (HBCD) Study will establish a large, diverse cohort of more than 7,500 pregnant people in their second trimester across a variety of regions and socioeconomic contexts and follow them and their children for up to a decade. The scientists will collect a large, well-characterized dataset that captures biologic, brain, behavior and social information about the children and their families, that will enable comparisons of brain development and behavioral outcomes of young children from a variety of environments.


Capturing ‘cinematic journey’ of brain development vs. ‘snapshots’
“We will know so much more about how brain development unfolds,” Norton said. “Right now, we have snapshots, but 10 years from now, we hope to have a greater picture of the whole cinematic journey, and a better understanding of how the structure of the brain relates to how it works.”
Findings from this study will be made accessible to scientists across the country in order to better determine urgent health needs, such as the current impact of the COVID-19 pandemic on development, or future health and environmental crises.
“One thing that is truly exciting about this study is its joint focus on risk and resilience, with the ultimate goal of creating strategies for promoting healthy outcomes despite early adversities for children from a broad range of backgrounds,” said Wakschlag, a professor and vice chair of medical social sciences at Northwestern. “Because development from pregnancy through the first years of life sets the critical foundation for lifelong health, learning and engagement with the world, capturing the many inflection points across this period will guide future personalized early life prevention in this period when children are most primed to benefit.”
Tracing early markers of variability in brain development

Redevelopment of Norris University Center’s East Lawn to begin this summer
‘the night watchman’ named next one book selection, six northwestern faculty named 2024 guggenheim fellows, related stories.
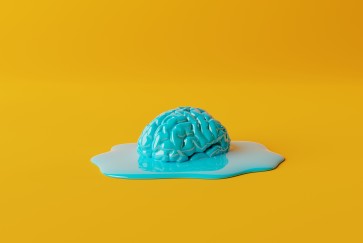
Brain power lessens over time. Why everyone needs a cognitive test at a certain age
New cause of neuron death in alzheimer's discovered, immersive vr goggles for mice unlock new potential for brain science.
Early Brain Development and Health
The early years of a child’s life are very important for later health and development . One of the main reasons is how fast the brain grows starting before birth and continuing into early childhood. Although the brain continues to develop and change into adulthood, the first 8 years can build a foundation for future learning, health and life success .
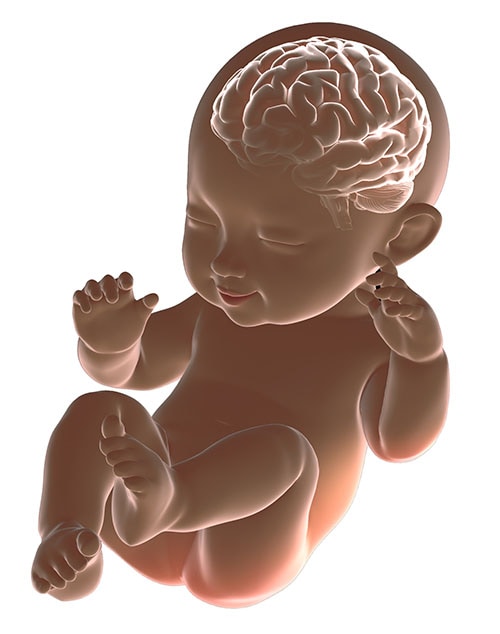
How well a brain develops depends on many factors in addition to genes, such as:
- Proper nutrition starting in pregnancy
- Exposure to toxins or infections
- The child’s experiences with other people and the world
Nurturing and responsive care for the child’s body and mind is the key to supporting healthy brain development. Positive or negative experiences can add up to shape a child’s development and can have lifelong effects . To nurture their child’s body and mind, parents and caregivers need support and the right resources. The right care for children, starting before birth and continuing through childhood, ensures that the child’s brain grows well and reaches its full potential. CDC is working to protect children so that their brains have a healthy start.
The importance of early childhood experiences for brain development
Children are born ready to learn, and have many skills to learn over many years. They depend on parents, family members, and other caregivers as their first teachers to develop the right skills to become independent and lead healthy and successful lives. How the brain grows is strongly affected by the child’s experiences with other people and the world. Nurturing care for the mind is critical for brain growth. Children grow and learn best in a safe environment where they are protected from neglect and from extreme or chronic stress with plenty of opportunities to play and explore.
Parents and other caregivers can support healthy brain growth by speaking to, playing with, and caring for their child. Children learn best when parents take turns when talking and playing, and build on their child’s skills and interests. Nurturing a child by understanding their needs and responding sensitively helps to protect children’s brains from stress. Speaking with children and exposing them to books, stories, and songs helps strengthen children’s language and communication, which puts them on a path towards learning and succeeding in school.
Exposure to stress and trauma can have long-term negative consequences for the child’s brain, whereas talking, reading, and playing can stimulate brain growth. Ensuring that parents, caregivers, and early childhood care providers have the resources and skills to provide safe, stable, nurturing, and stimulating care is an important public health goal.
When children are at risk, tracking children’s development and making sure they reach developmental milestones can help ensure that any problems are detected early and children can receive the intervention they may need.
Learn more about supporting early childhood experiences:
- Tracking developmental milestones
- Preventing abuse and neglect
- Positive parenting tips
- Healthy childcare
A healthy start for the brain
To learn and grow appropriately, a baby’s brain has to be healthy and protected from diseases and other risks. Promoting the development of a healthy brain can start even before pregnancy. For example, a healthy diet and the right nutrients like sufficient folic acid will promote a healthy pregnancy and a healthy nervous system in the growing baby. Vaccinations can protect pregnant women from infections that can harm the brain of the unborn baby.
During pregnancy, the brain can be affected by many types of risks, such as by infectious diseases like Cytomegalovirus or Zika virus, by exposure to toxins , including from smoking or alcohol , or when pregnant mothers experience stress, trauma, or mental health conditions like depression . Regular health care during pregnancy can help prevent complications, including premature birth, which can affect the baby’s brain. Newborn screening can detect conditions that are potentially dangerous to the child’s brain, like phenylketonuria (PKU).
Healthy brain growth in infancy continues to depend on the right care and nutrition. Because children’s brains are still growing, they are especially vulnerable to traumatic head injuries , infections, or toxins, such as lead . Childhood vaccines, such as the measles vaccine, can protect children from dangerous complications like swelling of the brain . Ensuring that parents and caregivers have access to healthy foods and places to live and play that are healthy and safe for their child can help them provide more nurturing care.
Learn more about the recommended care:
- Before pregnancy
- During pregnancy
- Around birth
- During infancy
- During early childhood
What does CDC do to support early brain health?
CDC is committed to supporting early brain health through evidence-based programs and partnerships within communities. Below are just a few examples of CDC programs that support early brain health:
- Learn the Signs. Act Early
- Legacy for Children TM
- Early Hearing Detection and Intervention
- Essentials for Childhood
- Concussion Prevention
- Lead Prevention
- Fetal Alcohol Spectrum Disorder Prevention
- Childhood Immunization
- Treating for Two
- Preconception Care
- Infant and Toddler Nutrition
- Addressing Health Disparities in Early Childhood
To receive email updates about this topic, enter your email address:
- Policy Makers
Exit Notification / Disclaimer Policy
- The Centers for Disease Control and Prevention (CDC) cannot attest to the accuracy of a non-federal website.
- Linking to a non-federal website does not constitute an endorsement by CDC or any of its employees of the sponsors or the information and products presented on the website.
- You will be subject to the destination website's privacy policy when you follow the link.
- CDC is not responsible for Section 508 compliance (accessibility) on other federal or private website.

EYFS Best Practice: All about ... neuroscience and the infant brain
David Whitebread and Lysandra Sinclaire-Harding Monday, October 20, 2014
What can we learn from research on early brain development? David Whitebread and Lysandra Sinclaire-Harding debunk some of the 'neuromyths' and misdiagnoses doing the rounds and look at how neuroscience findings might inform practice in early childhood education.

There is currently a great deal of interest in neuroscience and what it might tell us about education, particularly in relation to the early years. Governments around the world are investing huge sums of money in understanding differences, disorders and diseases of the brain, and it is clear that there is huge potential for this new science to inform us about early brain development.
At the same time, however, the appetite for 'brain-based' curricula has led to the promulgation of a number of 'neuromyths' (critical stages, left and right brain learning) and the publication of a number of pseudo-scientific programmes (for example, Brain Gym), which arise from overgeneralisations or misinterpretations of the actual scientific findings so far established.
The now infamous and highly misleading use of brain scans of three-year-old children, labelled 'normal' and 'extreme neglect', on the cover of Graham Allen's Early Intervention report ( www.gov.uk/government/uploads/system/uploads/attachment_data/file/ 284086/early-intervention-next-steps2.pdf ) is another worrying example of the misuse of brain research to push particular political agendas - in this case, falsely suggesting that the brains of young children who suffer socio-economic deprivation or 'poor parenting' will be severely and permanently withered.
THE HISTORY AND NATURE OF NEUROSCIENCE
To begin with, it is important to recognise the limitations of current neuroscientific research. This is a relatively new science, which has exciting potential, but the human brain is enormously complex and we have a great deal still to learn. Recent developments in investigative techniques have enabled huge advances, but they are still only in their infancy and need to be refined much further.
Much of the early knowledge about the brain depended on the examination of patients with brain injuries arising from unfortunate accidents. For example, the first anatomical proof of the localisation of brain function came in the 1860s from a French physician named Paul Broca.
He studied the brains of patients who had lost the ability to speak after injury (lesion) to a part of the cortex known as the posterior inferior frontal gyrus (Broca, 1861). However, brain lesions are not always specific and analysing damaged brains has limited use for understanding healthy brains.
New tools and methods of non-invasive neuro-imagery techniques have proved invaluable in increasing our knowledge about the brain. There are two main techniques used in neuroscience research related to early childhood development and education. These are:
- electroencephalography (EEG)
- functional magnetic resonance imagery (fMRI).
EEG involves measuring the electrical activity in different regions of the brain. It provides very precise measurements of when a particular event occurs in the brain (within milliseconds), but is rather vague about where such events are located.
Conversely, fMRI provides very accurate measurement of the location of activity, but is imprecise regarding timings. It uses magnetism and radio frequency waves to identify where the haemoglobin in the blood flow is carrying oxygen (when it has a different resonance) to identify where the brain is currently most active.
Using these techniques, it has been established that early brain development involves brain functions being achieved at greater speeds and becoming more focused on specific brain regions. Learning consists of the establishment of increasing numbers of synaptic connections between neurons and the increasing efficiency or strength of these connections. Developing expertise in any particular skill or area of knowledge will result in increased capacity in the related brain region.
However, both these techniques can only tell us when an area of the brain is particularly active in relation to a particular experience or activity, and the activities or experiences we can investigate are only those we can manage while lying in an fMRI scanner or sitting very still with an EEG 'net' stuck to our scalp.
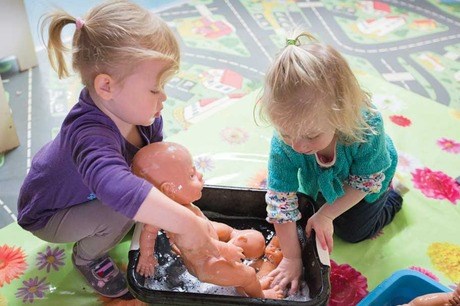
MYTHS DEBUNKED AND MISDIAGNOSES EXPOSED
The limitations of current neuroscientific research have, however, not prevented the promulgation of a range of neuromyths and misdiagnoses resulting from oversimplifications and overgeneralisations of early research and often tentative findings.
The left brain/right brain idea is one example, which originates from a study on patients with epilepsy. Sperry (1961) noticed that the surgical separation of brain hemispheres affected a person's ability to perform certain tasks, and this led to speculation that processing language is handled by a specific hemisphere.
Subsequent research appeared to support the idea of hemispheric dominance (for example, Fink and Marshall, 1996) and this idea pervaded Western education for many years. A person who is 'left-brained' was said to be more logical, analytical and objective, while a person who is 'right-brained' more intuitive, thoughtful and subjective.
A year after their initial study, Fink and Marshall (1997) repeated their work and obtained opposite results. It has been subsequently established that both hemispheres work together in collaborative, complex ways.
In addition to premature generalisations of this kind from a small number of initial and tentative findings, there has also been a tendency, unfortunately, to over-diagnose conditions that have been shown to have some neurological basis (see Elliott and Grigorenko, 2014).
Many theories exist for the causes of dyslexia, some of which have established neurological causes. However, reading and writing difficulties arise from a whole range of genetic, neurological or social reasons.
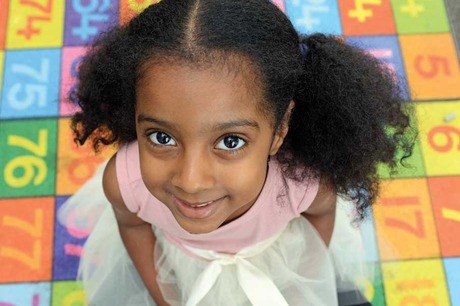
SUPPORTING 'GOOD PRACTICE'
Despite these reservations and what might be regarded as 'teething problems' of a young and fascinating science, it is clear that neuroscience has the potential to make important contributions to our understanding of early child development and early childhood education.
Current neuroscience supports much of developmental psychology and behavioural research, as well as confirming a number of insights that education professionals will recognise as the basis of existing 'good practice'. For example, neuroscientific research has supported the importance of children developing early secure emotional attachments, of children learning through play and the dangers of children being required to learn formal literacy skills too young.
Secure relationships
Secure family relationships that provide stimulation, interaction and nurture promote adaptation and growth in neural structures that respond to the needs and demands of everyday experience.
Where conditions are less than optimal, perhaps in situations of insecure maternal attachments, chronic stress or malnourishment, the development of the child may suffer. For example, chronic maternal stress during pregnancy and in infancy has been shown to threaten healthy brain development due to the toxic effect of stress hormones (Talge, Neal and Glover, 2007).
Playful environments
The beneficial effects of a stimulating, playful environment for young children's development as learners has also been supported. While there is little neuroscientific research on play in humans, the relationship between play and brain size has been compared in a number of different animal species (for example, Iwaniuk, Nelson and Pellis, 2001). Here, the research has found that animals with larger brains are more likely to engage in play than smaller brained animals, because more complex behaviour requires more neural tissue (Jerison, 1973).
Comparative studies have also shown that particular parts of the brain are enlarged in response to specific types of play. For example, the amygdala and hypothalamus of mice have been shown to increase in anatomical volume with social play, but not with object play (Pellis and Pellis, 2009).
Teaching literacy
There has also been support for the view that there are dangers in the formal teaching of literacy when children are too young, or too cognitively immature. One example is a study of five-year-olds in the USA who received formal literacy teaching in their first year of school.
In this instance, Yamada et al (2011) compared the effects of this teaching on typically developing children with those who were less mature and had more limited attention and self-regulatory skills, and who were assessed as being 'at risk' of reading difficulties.
After one semester of schooling, both groups of children were given letter recognition tasks while in an fMRI scanner. The on-track, typically developing children demonstrated activation in a region of the left angular gyrus, closely resembling neural networks activated in mature literate adults.
By contrast, the less mature children were seen to recruit compensatory neural tissue in frontal regions, even after they had received supplementary reading intervention.
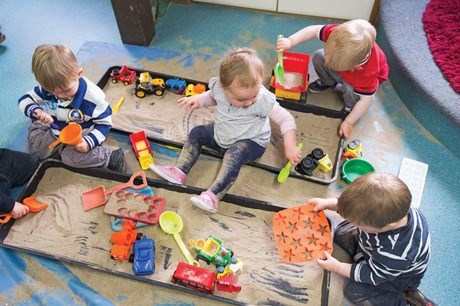
EARLY DIAGNOSIS AND TREATMENT
Perhaps the clearest contribution currently and potentially of neuroscience is in the diagnosis and treatment of developmental disorders. For example, neuroscientists have begun to reveal biological explanations for two such disorders increasingly identified in the early years: autistic spectrum disorder (ASD) and attention deficit and hyperactivity disorder (ADHD).
Early in their development, children begin to understand that the beliefs and intentions of others may be different from their own and are able to show empathy towards others. Baron-Cohen et al (1985) demonstrated that children with autism had particular difficulties with understanding the beliefs of others and suggested they lack a 'theory of mind'.
ASD is also associated with repetitive behaviours, obsessive desire for sameness, delayed language development and difficulties with planning. Genetic studies suggest that environmental triggers may collude with genetic vulnerability to increase the risk of ASD (Gerschwind and Levitt, 2007). Children with ASD are found to have anatomical differences in several areas of the brain (Branson, 2013).
All young children are naturally active, impulsive and easily excitable. Even excessive behaviours of this nature are usually outgrown. For some children, however, attention span is so short, activity level so high and impulse control so limited that learning and social development is severely impaired (Barkley, 2013).
In the UK, inattention and hyperactivity are now the most common problems for children in the early years classroom.
A number of genes have been associated with ADHD and as research continues it is likely that more will follow. For example, the dopamine receptor (DRD4) is found in 50-60 per cent of the ADHD population (Polanczyk et al, 2007).
Other contributory factors identified include foetal exposure to alcohol and nicotine, and complicated pregnancies or birthing. Research does not support popularly held views that ADHD arises from excessive sugar intake, food additives, excessive television viewing or poor parenting (Sciutto, Terjeson and Frank, 2000; Barkley, 2013), although such factors can contribute unhelpfully.
This type of research into the neurology of common disorders is likely to be helpful in aiding early diagnosis, and may also help in the development of effective treatments. For example, a recent brain imaging study in the USA showed that pre-schoolers who received eight weeks of attention-training exercises significantly improved their performance in tests of cognition, language and behaviour (Neville et al, 2013).
Children and parents were selected from low socio-economic status families and trained in activities of self-regulation that included vigilance, selective attention and task switching. Tasks became increasingly more challenging and similar to classroom activities. For example, one selective attention activity required children to focus their attention (colouring within the lines of detailed figures) while simultaneously suppressing distractions (ignoring other children balancing balloons in the air around them).
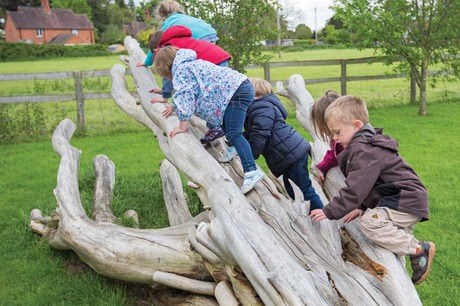
It is clear that early childhood educators need to be critically informed about neuroscience, both to avoid being seduced by apparently plausible but fallacious neuromyths and to be able to take advantage of the important evidence about early development that it is providing.
What is abundantly clear is that the human brain is distinctively attuned to respond to its environment. Neuroscience provides every reason for early years educators to believe that the environment they provide in the early years classroom can make a significant contribution to the healthy mental development of the children in their care.
David Whitebread is a senior lecturer in psychology and education at the Faculty of Education, University of Cambridge, and Lysandra Sinclaire-Harding is a PhD student of cognitive and behavioural neuroscience, Cambridge Neuroscience, University of Cambridge
Barkley RA (2013). Taking Charge of ADHD: the complete, authoritative guide for parents. Guilford Publications.
Baron-Cohen S, Leslie AM and Frith U (1985). 'Does the autistic child have a "theory of mind"?' in Cognition.
Branson D (2013). 'Brain differences in children with autism spectrum disorders and subsequent impact on learning' in Early Childhood and Neuroscience - Links to Development and Learning (pp121-139). Springer, Netherlands.
Broca P (1861). 'Remarks on the Seat of the Faculty of Articulated Language, Following an Observation of Aphemia (Loss of Speech)' in Bulletin de la Societe Anatomique.
Elliott J and Grigorenko EL (2014). The Dyslexia Debate. Cambridge University Press.
Fink GR, Halligan PW, Marshall JC, Frith CD, Frackowiak RS and Dolan RJ (1996). 'Where in the brain does visual attention select the forest and the trees?' in Nature.
Fink GR, Marshall JC, Halligan PW, Frith CD, Frackowiak RS and Dolan RJ (1997). 'Hemispheric specialization for global and local processing: the effect of stimulus category' in Proceedings B.
Gerschwind DH and Levitt P (2007). 'Autism spectrum disorders: developmental disconnection syndromes' in Current Opinion in Neurobiology.
Iwaniuk AN, Nelson JE and Pellis SM (2001). 'Do big-brained animals play more? Comparative analyses of play and relative brain size in mammals' in Journal of Comparative Psychology.
Jerison H (1973). Evolution of the Brain and Intelligence. Elsevier.
Neville H J, Stevens C, Pakulak E, Bell TA, Fanning J, Klein S and Isbell E (2013). 'Family-based training program improves brain function, cognition, and behavior in lower socio-economic status preschoolers' in Proceedings of the National Academy of Sciences.
Pellis S and Pellis V (2009). The Playful Brain: venturing to the limits of neuroscience. Oneworld.
Polanczyk G, de Lima MS, Horta BL, et al (2007). 'The worldwide prevalence of ADHD: a systematic review and metaregression analysis' in American Journal of Psychiatry.
Sciutto MJ, Terjesen MD and Frank ASB (2000). 'Teachers' knowledge and misperceptions of attention deficit/hyperactivity disorder' in Psychology in the Schools.
Talge NM, Neal C and Glover V (2007). 'Antenatal maternal stress and long-term effects on child neurodevelopment: how and why?' in Journal of Child Psychology and Psychiatry.
Thomas R, Mitchell GK and Batstra L (2013). 'Attention-deficit/hyperactivity disorder: are we helping or harming?' in British Medical Journal.
Yamada Y, Stevens C, Dow M, Harn BA, Chard DJ and Neville HJ (2011). 'Emergence of the neural network for reading in five-year-old beginning readers of different levels of pre-literacy abilities: an fMRI study' in NeuroImage.
Download the PDF
Related Articles
Child development: your guide to the first five years: part 2 - the beautiful brain.
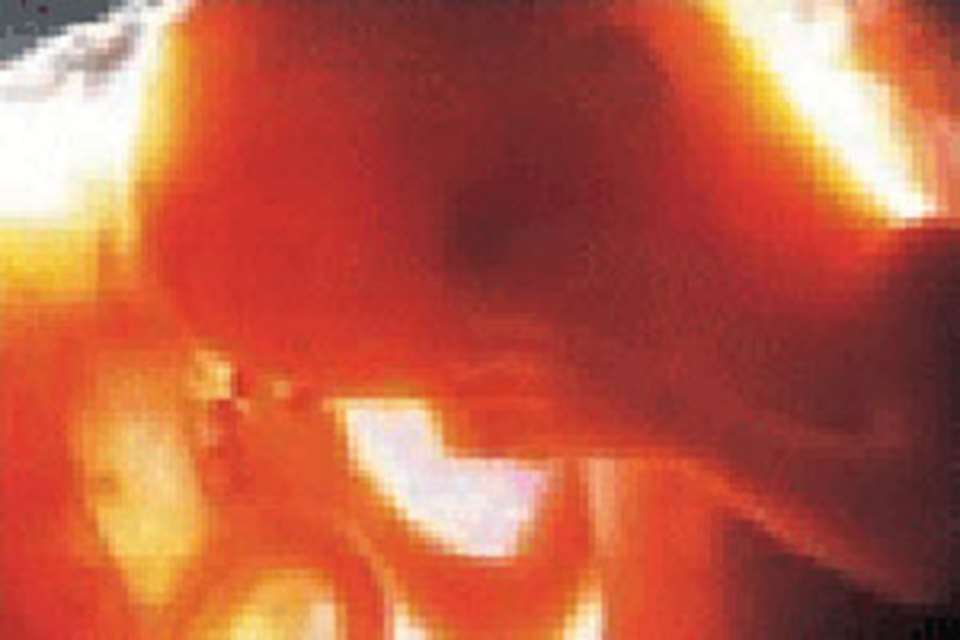
A Unique Child: The Developing Brain, part 1 - Life before birth
A unique child: the developing brain, part 2 - piaget revisited, a unique child: the developing brain, part 3 - face to face, a unique child: the developing brain: part 4 - numbers game.
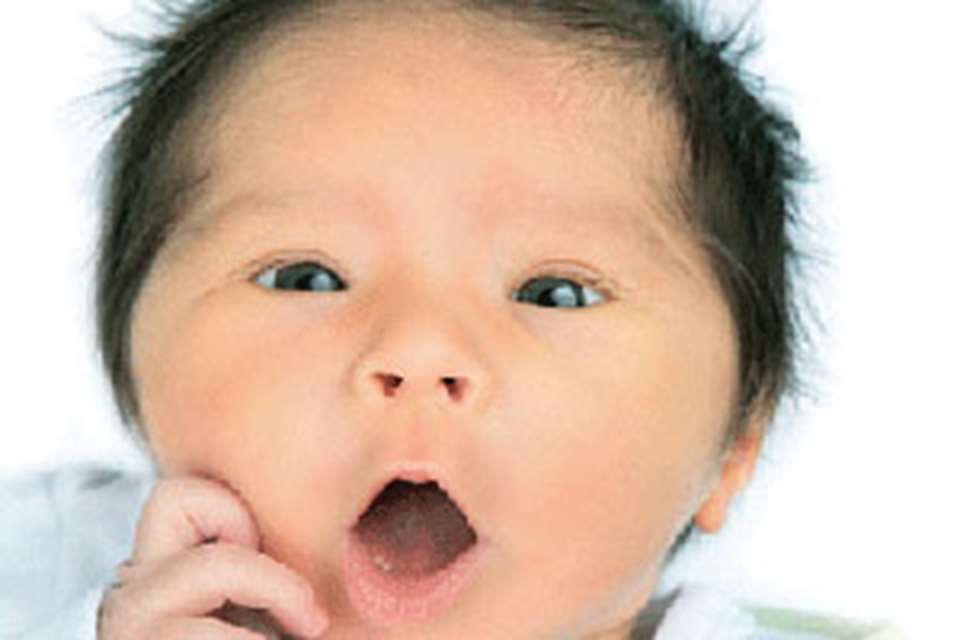
A Unique Child: the Developing Brain, part 5 - Speech patterns
A unique child: the developing brain: part 6 - sleep tight.
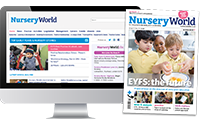
Nursery World Print & Website
- Latest print issues
- Latest online articles
- Archive of more than 35,000 articles
- Free monthly activity poster
- Themed supplements
From £11 / month

Nursery World Full Membership
- Latest digital issues
- Exclusive offers
From £13 / month

Nursery World Digital Membership
© MA Education 2024. Published by MA Education Limited, St Jude's Church, Dulwich Road, Herne Hill, London SE24 0PB, a company registered in England and Wales no. 04002826. MA Education is part of the Mark Allen Group. – All Rights Reserved
- International edition
- Australia edition
- Europe edition
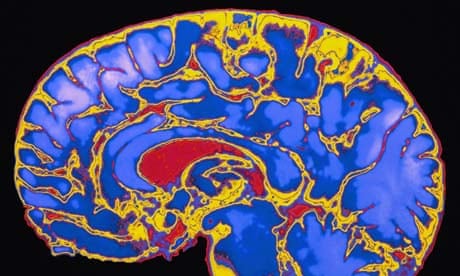
Childhood stimulation key to brain development, study finds
An early childhood surrounded by books and educational toys will leave positive fingerprints on a person's brain well into their late teens, a two-decade-long research study has shown.
Scientists found that the more mental stimulation a child gets around the age of four, the more developed the parts of their brains dedicated to language and cognition will be in the decades ahead.
It is known that childhood experience influences brain development but the only evidence scientists have had for this has usually come from extreme cases such as children who had been abused or suffered trauma. Martha Farah , director of the centre for neuroscience and society at the University of Pennsylvania, who led the latest study, wanted to find out how a normal range of experiences in childhood might influence the development of the brain.
Farah took data from surveys of home life and brain scans of 64 participants carried out over the course of 20 years. Her results, presented on Sunday at the annual meeting of the Society for Neuroscience in New Orleans , showed that cognitive stimulation from parents at the age of four was the key factor in predicting the development of several parts of the cortex – the layer of grey matter on the outside of the brain – 15 years later.
The participants had been tracked since they were four years old. Researchers had visited their homes and recorded a series of details about their lives to measure cognitive stimulation, details such as the number of children's books they had, whether they had toys that taught them about colours, numbers or letters, or whether they played with real or toy musical instruments.
The researchers also scored the participants on "parental nurturance" – how much warmth, support or care the child got from the parent. The researchers carried out the same surveys when the children were eight years old. When the participants were between 17 and 19, they had their brains scanned.
Farah's results showed that the development of the cortex in late teens was closely correlated with a child's cognitive stimulation at the age of four. All other factors including parental nurturance at all ages and cognitive stimulation at age eight – had no effect. Farah said her results were evidence for the existence of a sensitive period, early in a person's life, that determined the optimal development of the cortex. "It really does support the idea that those early years are especially influential."
As the brain matures during childhood and adolescence, brain cells in the cortex are pruned back and, as unnecessary cells are eliminated, the cortex gets thinner. Farah found that the more cognitive stimulation a participant had had at the age of four, the thinner, and therefore more developed, their cortex. "It almost looks like whatever the normal developmental process is, has either accelerated or gone further in the kids with the better cognitive stimulation," she said.
The most strongly affected region was the lateral left temporal cortex, which is on the surface of the brain, behind the ear. This region is involved in semantic memory, processing word meanings and general knowledge about the world.
Around the time the participants had their brains scanned in their late teens, they were also given language tests and, Farah said, the thinner their cortex, the better their language comprehension.
Andrea Danese , a clinical lecturer in child and adolescent psychiatry at the Institute of Psychiatry , King's College London, said the study suggested that the experience of a nurturing home environment could have an effect on brain development regardless of familial, perhaps genetic, predispositions to better brains. Danese added that this kind of research highlighted the "tremendous role" that parents and carers had to play in enabling children to develop their cognitive, social, and emotional skills by providing safe, predictable, stimulating, and responsive personal interactions with children.
"Parents may not be around when their teenage children are faced with important choices about choosing peers, experimenting with drugs, engaging in sexual relationships, or staying in education," said Danese. "Yet, parents can lay the foundations for their teenage children to take good decisions, for example by promoting their ability to retain and elaborate information, or to balance the desire for immediate reward with the one for greater, long-term goals since a young age."
Bruce Hood , an experimental psychologist who specialises in developmental cognitive neuroscience at the University of Bristol, said his advice to parents was just to "be kind to your children. Unless you raise them in a cardboard box without any stimulation or interaction, then they will probably be just fine."
- Neuroscience
- Medical research
- Society for Neuroscience annual meeting
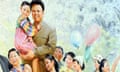
Young blood can reverse some effects of ageing, study finds
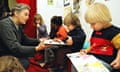
Policymakers seduced by neuroscience to justify early intervention agenda
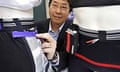
Electric pants may prevent bed sores
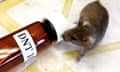
GM mouse created to detect landmines
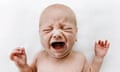
Why crying babies are so hard to ignore
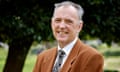
John Bruer: 'Growing up in poverty doesn't damage your brain irretrievably
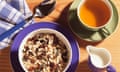
Skipping breakfast primes brain to seek high-calorie food, study finds
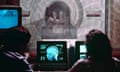
Is misused neuroscience defining early years and child protection policy?
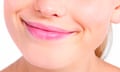
Status affects how readily people return smiles, research reveals
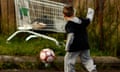
Childhood adversity affects adult brain and body functions, researchers find
Comments (…), most viewed.
- News stories
- Blog articles
- NSPCC Learning podcast
- Why language matters
- Sign up to newsletters
- Safeguarding in Education Update
- CASPAR email alert
- Key topics home
- Safeguarding and child protection
Child abuse and neglect
- Child health and development
- Safer recruitment
- Case reviews
- Online safety
- Research and resources home
- NSPCC research
- Safeguarding resources
- How Safe conference
- Self-assessment tool
- Schools and colleges
- Training home
- Basic safeguarding courses
- Advanced training
- Elearning courses
- Designated person training
- Schools and education courses
- Services home
- Direct work: children and families
- Talk Relationships
- Consultancy
- Library and Information Service
- Support for local communities
- NSPCC Helpline
- Speak out Stay safe schools service
- My learning
- Self-assessment
- /g,'').replace(/ /g,'')" v-html="suggestion">
How childhood trauma affects child brain development
How do childhood experiences affect brain development.
Our brains develop from before birth and into adulthood (Siegel and Bryson, 2012). But there are key ‘sensitive periods’ during early childhood and adolescence where children and young people’s brains are more affected by positive or negative experiences (Shonkoff et al, 2008).
What happens in a child or young person’s life during these periods can have a significant effect on their brain development.
Positive experiences throughout childhood help to build healthy brains, while experiencing childhood trauma and abuse can harm a child’s brain development (Shonkoff et al, 2015).
But our brains always have the potential to change and grow. It’s never too late to give a child or young person positive brain building experiences.
Having caring relationships and access to support services can reduce the harmful effects of negative experiences and help a child’s brain develop in a healthy way (Shonkoff et al, 2015).
> Find out why the bond between a child and their caregivers is important
> Find out more about the adolescent brain in our How safe are our children? report for 2020
How we use metaphors to explain brain development
We want to create a shared and simple language around child brain development that can be used by all professionals, parents, carers and children.
Sharing the Brain Story uses six key metaphors, developed by the Harvard Center on the Developing Child , the Alberta Family Wellness Initiative and the FrameWorks Institute .
These metaphors can be used to improve understanding of child development and give positive brain building experiences to children who have experienced trauma.
On this page you’ll find information about each of the six metaphors and tips on how to use them in your work with children and families. We also have a summary booklet you can download to learn more about each of the metaphors and access further reading.
Download the booklet (PDF)
Trauma and child brain development training
Sign up for our face-to-face training programme delivered by experts where we explore child brain development and the six metaphors through practical exercises, case studies, examples and more.
Register now
Brain architecture: how children’s brains develop
The first Sharing the Brain Story metaphor describes how the development of children’s brains is like the development and construction of a house.
Early experiences build the foundations of our brains. Like a house is built step-by-step – first laying the foundations, then creating the rooms and wiring the electrical system – our brains are built in a similar way.
As good quality materials help build a strong house, positive experiences during childhood help build healthy brains.
Although it’s never too late for the brain to benefit from positive experiences, early experiences build the foundations of brain architecture. A strong brain foundation increases the chances of healthy learning later in life (Shonkoff et al, 2007).
Negative experiences such as abuse and neglect can affect the brain’s architecture by increasing stress-related disorders including:
- mental health problems
- diabetes and cardiovascular disease
(Shonkoff et al, 2014).
Building brain architecture
Like improving or rebuilding the architecture of a house – refurbishing rooms, strengthening foundations – the brain can also be improved and rebuilt following childhood trauma through an active process of maintenance and care from supportive, trusted adults.
Brain architecture can be built throughout childhood, adolescence and even into adulthood (Shonkoff et al, 2008).
The remaining five metaphors - serve and return, air traffic control, toxic stress, overloaded, tipping the scales - will help you give parents and carers the tools to build strong brain architecture in children and young people.
> Register for our face-to-face training to better understand how to foster healthy brain development in children
> Read more about how you can use the brain architecture metaphor in our Sharing the Brain Story booklet (PDF)
Serve and return: the importance of interaction
The serve and return metaphor from Sharing the Brain Story looks at adult-child relationships by comparing interactions between a child and adult to rallies in a tennis match.
From the ages of 0 to 5, children naturally start interacting with adults through babbling, gesturing and facial expressions. This develops vital language, cognitive and social skills.
Interactions between a child and an adult is like playing a game of tennis. When adults ‘return’ the child’s ‘serve’ with a positive response, a ‘rally’ is created.
Just as good rallies between tennis players develop their athletic abilities, good rallies between children and adults strengthen children’s developing brain architecture, improve their cognitive skills and develop resilience (Shonkoff et al, 2004; Shonkoff et al, 2015).
When adults do not respond to a child’s serve or if children spend a long time on passive activity – such as watching TV – this can break the rally and interrupt development. For example, children learn language, more effectively face-to-face with a teacher or caregiver than by watching TV or a video (Shonkoff et al, 2018).
Improving interaction with children
Sensitive and responsive adult-child relationships help build children’s cognitive skills and brain architecture. Research shows a clear link between social and emotional development and intellectual growth (Shonkoff, Boyce and McEwen, 2009).
> Discover how sensitive, responsive parenting can help to build bonds between children and their caregivers
> Sign up for free brain-building tips with Look, Say, Sing, Play
> Download our free Look, Say, Sing, Play resources
To increase serve and return interactions, you can:
- focus on interactive, face-to-face activity, such as talking, singing and playing
- respond to and build on physical and verbal cues: if they babble, babble back; if they play with toys, play with them
- allow the child to ‘serve’ – follow their focus and return their serve with support and encouragement
- maintain eye contact when interacting with the child – this helps to build a nurturing, secure relationship
- positively reinforce children’s vocalisations: children repeat behaviours when they are praised, which helps shape their babbles into words (Crowley, 2017).
> Learn how to build strong brain architecture through serve and return interactions in our face-to-face training
> Get more information on how you can use the serve and return metaphor in our Sharing the Brain Story booklet (PDF)
Air traffic control: managing and ordering tasks
This Sharing the Brain Story metaphor compares how children manage mental processes to how an air traffic controller manages aeroplanes in an airport.
Every day, we manage various demands on our attention, from paying attention and controlling impulses, to managing emotions and prioritising tasks (Shonkoff et al, 2011).
With practice and the right support, children learn to organise and control the tasks in their mental headspace, like an air traffic controller organises and lands aeroplanes in a busy airport (Shonkoff et al, 2011).
Executive functioning skills
The air traffic control system in a child’s brain is also known as ‘executive function’: the system that manages social and cognitive skills. Children depend on these emerging executive function skills as they learn to read and write, develop arithmetic skills and interact with peers (Shonkoff et al, 2011).
Although executive function skills can be improved at any time during and after childhood, negative experiences such as trauma and abuse , can hinder the brain’s cognitive development (Diamond, 2013). This can weaken the child’s air traffic control system and may lead to:
- reduced impulse control
- weakened working memory and attention skills
- disruptive behaviour
(Shonkoff et al, 2011).
Just as an air traffic controller can learn to manage arriving and departing flights, children can learn to manage their mental processes through practice and support.
Building executive function skills
Executive function skills develop throughout childhood, adolescence and into adulthood (Shonkoff et al, 2011). It’s important to give children opportunities to develop these skills through experiences appropriate to their age and ability.
You can help children learn air traffic control skills in various ways.
- Set a good example through your own actions: children can see adults performing tasks and model themselves on the adult’s behaviour. This is called observational learning (Crowley, 2017).
- Engage in playful serve and return interactions: social play is important to the development of executive function skills (Shonkoff et al, 2011).
- Talk to children about the ‘planes’ flying around their mind. Discuss how a trusted adult could help them land or look after some of these planes if they are feeling stressed or overwhelmed.
- Help children foster decision making and cognitive skills within playful situations appropriate to their age and ability: encourage the child to make choices and ‘direct their own activities with decreasing adult supervision over time’ (Shonkoff et al, 2011, p.6).
> Take our training course to gain knowledge of building strong executive function skills and air traffic control systems
> Explore how you can use the air traffic control metaphor in our Sharing the Brain Story booklet (PDF)
Toxic stress: the effects of stress on child development
Everyone experiences stress, and learning how to cope with stresses is an important part of child brain development (Shonkoff et al, 2014).
Some stress can be ‘positive’, such as solving problems or preparing for an exam. With adult or peer support, these experiences can help children develop coping and concentration skills that will help in later life.
Other stresses can be ‘tolerable’. For example, children are usually able to cope with bereavement if they have the right ‘buffers’ or support from parents, carers, friends and family.
But if children are exposed to prolonged or repeated traumatic experiences, such as child abuse and neglect , this can cause ‘toxic’ or ‘harmful’ stress, where they start to feel more stressed more often and for longer periods. This can disrupt the building of healthy brain architecture (Shonkoff et al, 2014).
This can affect children’s physical and cognitive development, including:
- a weakened immune system
- problems with memory and learning
- a reduced ability to control one’s moods or emotions
- slower information processing
(Crowley, 2017; Shonkoff et al, 2014).
Reducing toxic or harmful stress
The effects of harmful stress on a child’s brain and body can be reduced with long-term, consistent help and support (Shonkoff et al, 2014).
The parts of the brain responsible for dealing with stress are particularly malleable during early childhood. Childhood outcomes can be improved through positive changes, such as:
- community-based interventions
- increased adult-child interaction
- supportive relationships.
(Franke, 2014; Shonkoff et al, 2014).
You can help reduce harmful stress in various ways.
- Soothe and care for children when they are distressed – this helps their brains develop healthier ways to manage stress (Crowley, 2017).
- Interact with young children through serve and return, for example by listening and responding to their concerns. This helps build brain architecture and stress management systems (Center on the Developing Child, 2013).
- Help ‘buffer’ the effects of tolerable stresses by offering safe, reliable and responsive support to children and young people.
- Help parents and carers reduce the level of stress their child is exposed to, by giving them support to manage any challenges their family is experiencing.
> Take our face-to-face training where you'll find out more about toxic stress and how it can be reduced
> Read more about how you can use the stress metaphor in our Sharing the Brain Story booklet (PDF)
Overloaded: the effects of parental stress on children
This Sharing the Brain Story metaphor compares the process of lorries carrying large loads to parents and carers being overloaded with challenges.
When a lorry carries too much weight, it can be overloaded to the point of breaking down. When parents are experiencing challenges like poverty or lack of support, the weight of these problems can overload their mental and emotional capacity to take care of their children’s needs.
Challenges are often out of a parent or carer’s control, such as:
- violence or feeling unsafe in the community
- financial insecurity
- lack of community support
- losing a job
- a relationship ending.
Just as we can lighten an overloaded lorry’s load by bringing in other lorries or moving some things by train, we can lighten parents’ and carers’ loads by providing them with appropriate support. This can help improve their capacity to care for their children (Kendall-Taylor and Stanley, 2018).
> Find out more about early help for families and children
Recognising when parents need help
Some overloaded parents may be reluctant to ask for help for a number of reasons. For example, they might be worried their child will be removed from their care (National Commission on Domestic and Sexual Violence and Multiple Disadvantage, 2019).
This means that it’s important for professionals to recognise the load that parents and carers might be carrying, provide appropriate support and work with families to reduce unnecessary weight and strain.
There are various ways that you can do this.
- Start talking to parents and carers about what support they need as soon as problems are identified.
- Take time to understand a parent or carer’s position. Sometimes services designed to help might be overwhelming for already overloaded parents.
- Encourage parents and carers to seek out support from their community and family members or guide them to support directly. Interventions which strengthen community resources can help manage children’s toxic stress response (Franke, 2014).
> Learn about the science behind the effects of stress and how you can tackle parental overloading by taking our training
> See a breakdown of how you can use the overloaded metaphor in our Sharing the Brain Story booklet (PDF)
Tipping the scales: outweighing adverse childhood experiences
The final Sharing the Brain Story metaphor uses the concept of scales to explain how to achieve long-term positive brain building environments.
Child brain development is like a set of scales. On one side of the scales there are positive experiences and on the other, negative experiences.
Over time, if the positive experiences outweigh negative ones, the scales tip towards more positive long-term outcomes for the child. We want to help all children’s scales tip towards the positive.
All parents, carers, family, friends or those who work with children can help tip the scales positively.
(Shonkoff et al, 2015).
Tipping the scales towards positive outcomes
By using each of the six metaphors, separately and together, you can help parents, carers and communities understand how to build a healthy environment in which children’s brains can develop.
This is particularly important between the ages of 0 to 5 and during adolescence. Due to the speed of growth, children’s brains are at their most adaptable during these ‘sensitive’ periods of development – so it’s beneficial to stack children and young people’s scales with as much support as possible to help build their resilience.
By sharing the brain story through these six metaphors, all the adults around a child can learn how to help create positive brain building environments and experiences. These include:
- building strong brain architecture through serve and return interactions
- helping children to develop their air traffic control skills
- helping parents and carers avoid becoming overloaded and support them in maintaining a stable, low-stress environment within a framework of supportive and committed adult-child relationships.
> For more information on all our metaphors and advice for helping healthy child brain development, take our face-to-face training
> Learn more about how you can use the tipping the scales metaphor in our Sharing the Brain Story booklet (PDF)
References
Center on the Developing Child (2013) Innovating in early head start: can reducing toxic stress improve outcomes for young children? [Accessed 16/03/2021].
Crowley, Kevin (2017) Child development: a practical introduction. London: SAGE Publications Ltd.
Diamond, A (2013) Executive functions Annual Review of Psychology, 64: 135-168.
Franke H.A (2014) Toxic stress: effects, prevention and treatment . Children, 1(3): 390–402.
Kendall-Taylor, N and Stanley, K (2018) Seeing context through metaphor: using communications research to bring a social determinants perspective to public thinking about child abuse and neglect . International Journal of Environmental Research and Public Health, 15(1): 8-14.
National Commission on Domestic and Sexual Violence and Multiple Disadvantage (2019) Breaking down the barriers (PDF) . [s.l.]: National Commission on Domestic and Sexual Violence and Multiple Disadvantage.
Siegel, D. J. and Bryson, T. P. (2012) The whole-brain child 12 revolutionary strategies to nurture your child's developing mind, survive everyday parenting struggles, and help your family thrive. London: Robinson.
Shonkoff, J.P. et al (2004) Young children develop in an environment of relationships (PDF) . Cambridge: Center on the Developing Child, Harvard University.
Shonkoff, J.P. et al (2008) The timing and quality of early experiences combine to shape brain architecture: working paper 5 (PDF) . Cambridge: Center on the Developing Child, Harvard University.
Shonkoff, J.P., Boyce, W.T. and McEwen, B.S. (2009) Neuroscience, molecular biology, and the childhood roots of health disparities: building a new framework for health promotion and disease prevention , Journal of the American Medical Association, 301 (21), 2252-2259.
Shonkoff, J.P. et al (2011) Building the brain’s “air traffic control” system: how early experiences shape the development of executive function: working paper 11 (PDF) . Cambridge: Center on the Developing Child, Harvard University.
Shonkoff, J.P. et al (2014), Excessive stress disrupts the architecture of the developing brain: working paper 3 (PDF) . Cambridge: Center on the Developing Child, Harvard University.
Shonkoff, J.P. et al (2015) Supportive relationships and active skill-building strengthen the foundations of resilience: working paper 13 (PDF) . Cambridge: Center on the Developing Child, Harvard University.
Shonkoff, J.P. et al (2018) Understanding motivation: building the brain architecture that supports learning, health, and community participation: working paper 14 (PDF) . Cambridge: Center on the Developing Child, Harvard University.
If a child or young person needs confidential help and advice, direct them to Childline. Calls to 0800 1111 are free and children can also contact Childline online or read about dealing with stress on the Childline website. You can also download or order Childline posters and wallet cards .
Further reading
For further reading about child brain development, search the NSPCC library catalogue using the keyword "brain" and "child development".
> Find out more about the Library and Information Service
Metaphors summary booklet
Download Sharing the Brain Story: using metaphors to explain child development (PDF)
Register for child brain development training
Attend our face-to-face training course to inform your practice around child brain development and trauma.
Children and families at risk
What research tells us about risk, impact and what works to help children and families who need additional support.
There are several different types of child abuse. Do you know what all of these are?

Explain how current scientific research relating to neurological and brain development in Early Years influences practice in Early Years settings
Qualification : NCFE CACHE Level 3 Diploma for the Early Years Educator Unit : Unit 3.9: Facilitate the cognitive development of children Learning outcome : Understand about cognitive development in children Assessment criteria : Explain how current scientific research relating to neurological and brain development in Early Years influences practice in Early Years settings
In the context of early years childcare in the UK, current scientific research relating to neurological and brain development plays a significant role in shaping practices. Understanding how children’s brains develop in the early years is crucial for childcare supervisors, as it informs how they can best support the cognitive, emotional, and social development of young children. Here’s how this research influences practice in early years settings:
- Emphasis on Early Experiences : Research has shown that the first few years of a child’s life are critical for brain development. Positive experiences, including nurturing care, stimulation, and a safe environment, significantly impact the brain’s architecture. In early years settings, this leads to a focus on creating enriching, supportive, and safe environments for children.
- Importance of Play-Based Learning : Studies have demonstrated that play is not just a leisure activity; it’s a key part of learning and brain development. Play-based learning approaches are therefore central in early years settings, encouraging creativity, problem-solving, and social skills.
- Focus on Social and Emotional Development : Understanding that early brain development is not just about cognitive skills but also about emotional and social skills, childcare supervisors ensure that practices support the development of empathy, self-regulation, and interpersonal skills.
- Tailored Learning and Inclusion : Insights into brain development have highlighted the importance of recognizing individual differences in children’s development. This leads to more personalized approaches to learning and support for children with special educational needs or disabilities.
- Parental Engagement and Family Support : Research underscores the importance of the home environment and parental involvement in early brain development. This has led to increased efforts to involve families in early years settings, through communication, education, and support programs.
- Professional Development of Staff : With ongoing research and discoveries, it’s important for early years professionals to stay informed. This means continuous professional development and training in the latest research and best practices.
- Integration of Technology : Emerging research on the impact of technology on brain development influences how and when technology is used in early years settings. It’s used thoughtfully to support learning while being mindful of the risks of overexposure at a young age.
- Nutrition and Physical Health : Understanding the link between brain development and physical health, there’s an emphasis on nutritious meals and physical activities in early years settings.
- Early Identification and Intervention : Research has shown that early identification and intervention in cases of developmental delays can have significant impacts. Therefore, regular monitoring and assessment of children’s development is a key practice.
- Supporting Language Development : Given the critical period for language acquisition in early brain development, early years settings focus heavily on language-rich environments, including reading, storytelling, and conversation.
In summary, scientific research into brain and neurological development plays a pivotal role in shaping early years practices. It helps ensure that children’s experiences during these formative years provide a solid foundation for their future learning and development.

New origin of deep brain waves discovered
Knowledge of hippocampal activity could help improve sleep and cognition therapies.
University of California, Irvine biomedical engineering researchers have uncovered a previously unknown source of two key brain waves crucial for deep sleep: slow waves and sleep spindles. Traditionally believed to originate from one brain circuit linking the thalamus and cortex, the team's findings, published today in Scientific Reports , suggest that the axons in memory centers of the hippocampus play a role.
For decades, slow waves and sleep spindles have been identified as essential elements of deep sleep, measured through electroencephalography recordings on the scalp. However, the UC Irvine-led team revealed a novel source of these brain waves within the hippocampus and were able to measure them in single axons.
The study demonstrates that slow waves and sleep spindles can originate from axons within the hippocampus' cornu ammonis 3 region. These oscillations in voltage occur independently of neuronal spiking activity, challenging existing theories about the generation of these brain waves.
"Our research sheds light on a previously unrecognized aspect of deep sleep brain activity," said lead author Mengke Wang, former UC Irvine undergraduate student in biomedical engineering who is now a graduate student at Johns Hopkins University (Wang conducted the study while at UC Irvine). "We've discovered that the hippocampus, typically associated with memory formation, plays a crucial role in generating slow waves and sleep spindles, offering new insights into how these brain waves support memory processing during sleep."
The team utilized innovative techniques -- including in vitro reconstructions of hippocampal subregions and microfluidic tunnels for single axon communication -- to observe spontaneous spindle waves in isolated hippocampal neurons. These findings suggest that spindle oscillations originate from active ion channels within axons, rather than through volume conduction as previously thought.
"The discovery of spindle oscillations in single hippocampal axons opens new avenues for understanding the mechanisms underlying memory consolidation during sleep," said co-author Gregory Brewer, adjunct professor of biomedical engineering. "These findings have significant implications for sleep research, potentially paving the way for new approaches to treating sleep-related disorders."
Brewer's other research affiliations include the Institute for Memory Impairment and Neurological Disorders and the Center for Neurobiology of Learning and Memory.
By uncovering the hippocampus's role in generating slow waves and sleep spindles, this research expands our understanding of the brain's activity during deep sleep and its impact on memory processing. The findings offer a promising foundation for future studies exploring the therapeutic potential of targeting hippocampal activity to improve sleep quality and cognitive function.
Joining Brewer and Wang in this study, which received financial support from the UCI Foundation, were William Tang, professor emeritus of biomedical engineering; Bryce Mander, associate professor of psychiatry & human behavior; and Samuel Lassers, graduate student researcher in biomedical engineering.
- Sleep Disorders
- Obstructive Sleep Apnea
- Neuroscience
- Intelligence
- Disorders and Syndromes
- Brain-Computer Interfaces
- Memory-prediction framework
- Rapid eye movement
- Circadian rhythm sleep disorder
- Sleep deprivation
Story Source:
Materials provided by University of California - Irvine . Note: Content may be edited for style and length.
Journal Reference :
- Mengke Wang, Samuel B. Lassers, Yash S. Vakilna, Bryce A. Mander, William C. Tang, Gregory J. Brewer. Spindle oscillations in communicating axons within a reconstituted hippocampal formation are strongest in CA3 without thalamus . Scientific Reports , 2024; 14 (1) DOI: 10.1038/s41598-024-58002-0
Cite This Page :
Explore More
- Plastic Pollution Kills Ocean Embryos
- Most Massive Stellar Black Hole in Our Galaxy
- Coffee's Prehistoric Origin and It's Future
- Can Animals Count? New Rat Study
- A Single Atom Layer of Gold: Goldene
- Fool's Gold May Contain Valuable Lithium
- Exercise Cuts Stress-Related Brain Activity
- Microplastics Go from Gut to Other Organs
- Epilepsy Drug May Prevent Brain Tumors
- Evolution's Recipe Book
Trending Topics
Strange & offbeat.

IMAGES
VIDEO
COMMENTS
An account of brain development in the early years of childhood is only complete if we first examine the origins of this process during the prenatal months. Brain development is a protracted process that begins about 2 weeks after conception and continues into young adulthood 20 years later.
The science of early brain development can inform investments in early childhood. These basic concepts, established over decades of neuroscience and behavioral research, help illustrate why child development—particularly from birth to five years—is a foundation for a prosperous and sustainable society.
Our understanding of early development of these brain-related phenotypes has increased substantially in the past 20 years, driven by an expanding number of cross-sectional and longitudinal ...
Research on early development of the brain could also help to identify early markers for cognitive functioning. For example, visual-spatial working memory is a strong predictor for academic achievement and has been related to structural brain development in infants at 1 and 2 years of age [ 22 ] and children between 6 and 20 years of age [ 37 ].
Research on Brain Development F or decades researchers have been aware of the ex-traordinary development of a child's brain during the first five years of life. Recent advances in neuro-science have helped crystallize earlier findings, bringing new clarity and understanding to the field of early child-hood brain development. Children are born ...
Only a few studies have examined associations between SES and functional brain development using a network science ... FA in early childhood (4-7 years ... map to current developmental research.
The functional development of the human brain could, for example, be related to developmental theories in a testable way using the neural maturation accounts put forward by Johnson, 2001, Johnson, 2010 and Johnson et al. (2009). This theory was developed to provide a biological framework for the development of early attention systems.
Most recently, neurobiologic information on brain function and structure has been used to promote strategies for optimizing child development. This review will evaluate the current state of knowledge relating early child development to brain research and illustrate the potential misuse of this information. It will also suggest the following: (a ...
Cognitive development is marked by age-related improvements across a number of domains, as young children perform worse than their older counterparts on most tasks. ... Children's advantages appear to cluster around the preschool and early school years, though there are cases of benefits in infancy and later childhood. ... Current Directions ...
Abstract. Research over the past several decades has provided insight into the processes that govern early brain development and how those processes contribute to behavior. In the following article, we provide an overview of early brain development beginning with a summary of the prenatal period. We then turn to postnatal development and ...
npj Science of Learning (2022) The brain undergoes profound development across childhood and adolescence, including continuous changes in brain morphology, connectivity, and functioning that are ...
Oxford researchers involved nearly 4,000 children across the UK in three specially developed science lessons to educate pupils about brain development during early childhood. The SEEN (Secondary Education around Early Neurodevelopment) project was commissioned and funded by KindredSquared and is part of a wider drive to increase public understanding of how early experiences can shape the ...
Pregnancy and the first few years of life are a period of exponential brain growth and development. The unprecedented convergence of the opioid epidemic and COVID-19 pandemic provides a unique opportunity to shed light on how these early exposures during pregnancy may shape young children's development and functioning at the population level.
Brain development proceeds rapidly from gestation through early childhood. Environmental factors play an obligatory role in stress-sensitive early neurodevelopmental processes, with implications for health and disease throughout the lifespan. This foundational period thus represents a time of great vulnerability and opportunity. Over the last two decades, neuroimaging tools employed from the ...
Summary. In general, brain development begins a. few weeks after conception and is thought to. be complete by early adulthood. The basic. structure of the brain is laid down primarily. during the ...
Scientists say active early learning shapes the adult brain. Through the Abecedarian Project, an early education, randomized controlled trial that has followed children since 1971, researchers ...
The early years of a child's life are very important for later health and development. One of the main reasons is how fast the brain grows starting before birth and continuing into early childhood. Although the brain continues to develop and change into adulthood, the first 8 years can build a foundation for future learning, health and life ...
EYFS Best Practice: All about ... neuroscience and the infant brain. What can we learn from research on early brain development? David Whitebread and Lysandra Sinclaire-Harding debunk some of the 'neuromyths' and misdiagnoses doing the rounds and look at how neuroscience findings might inform practice in early childhood education.
Sun 14 Oct 2012 16.30 EDT. An early childhood surrounded by books and educational toys will leave positive fingerprints on a person's brain well into their late teens, a two-decade-long research ...
What happens in a child or young person's life during these periods can have a significant effect on their brain development. Positive experiences throughout childhood help to build healthy brains, while experiencing childhood trauma and abuse can harm a child's brain development (Shonkoff et al, 2015). But our brains always have the ...
The science is clear. In the first few years of life, a child's brain develops rapidly, driven by a mix of experience, environment and genes. Children will continue to develop throughout childhood and into adulthood, but in the early years their brains are particularly sensitive. By contrast, the science
In recent years, there has been a surge of interest in research concerning the neurological and brain development of children during their early years. Scientists have attempted to uncover how these cognitive and physical developments can be nurtured and encouraged in order to create the best possible environment for young children to thrive.
In the context of early years childcare in the UK, current scientific research relating to neurological and brain development plays a significant role in shaping practices. Understanding how children's brains develop in the early years is crucial for childcare supervisors, as it informs how they can best support the cognitive, emotional, and ...
April 10, 2024. Source: University of California - Irvine. Summary: Biomedical engineering researchers have uncovered a previously unknown source of two key brain waves crucial for deep sleep ...