- Corpus ID: 109984749

Physiology of Mungbean and Development of the Mungbean Crop Model
- Y. Chauhan , Col Douglas , +3 authors A. Skerman
- Published 2010
- Agricultural and Food Sciences
Figures from this paper
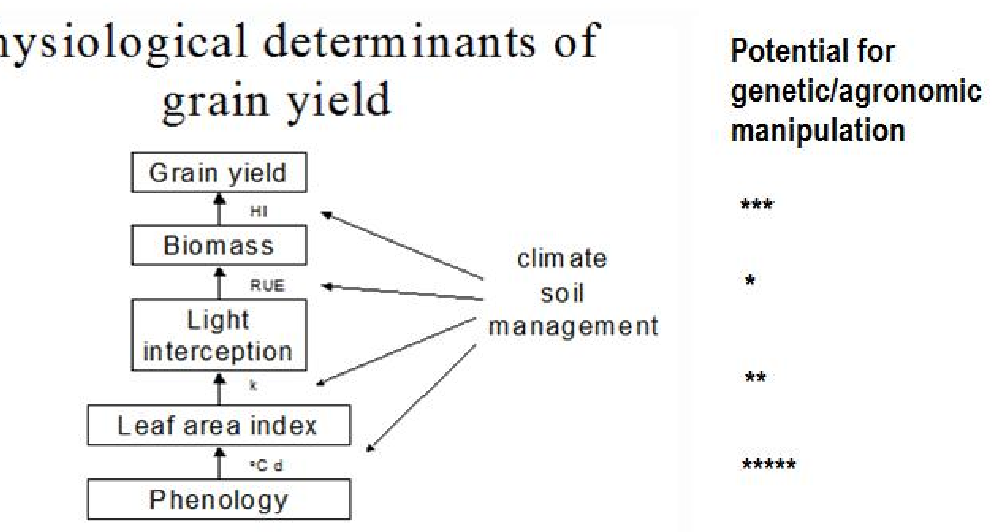
20 Citations
Breeding progress and future challenges: abiotic stresses, biotic and abiotic constraints in mungbean production—progress in genetic improvement, salinity and high temperature tolerance in mungbean [vigna radiata (l.) wilczek] from a physiological perspective.
- Highly Influenced
Towards Development of Climate Smart Mungbean: Challenges and Opportunities
The effect of growing season on growth rate, pod partitioning, phenology and yield variations of mungbean varieties, efficacy of integrated management of web blight of mungbean in kymore plateu and satpura hills agroclimatic zone of madhya pradesh, india, defining agro-ecological regions for field crops in variable target production environments: a case study on mungbean in the northern grains region of australia, green synthesis and application of go nanoparticles to augment growth parameters and yield in mungbean (vigna radiata l.), effect of peg-induced drought stress on mungbean plants revealed resistant varieties based on leaf wilting index and biochemical molecules, dynamical climatic model for time to flowering in vigna radiata, 30 references, physiological aspects of peanut yield improvement 1, agronomic and physiological constraints to the productivity of tropical grain legumes and prospects for improvement, simulation of growth and development of diverse legume species in apsim, accumulation and partitioning of biomass and nitrogen by soybean, mungbean and cowpea under contrasting environmental conditions, harvest index: a review of its use in plant breeding and crop physiology, towards the reliable prediction of time to flowering in six annual crops. iv. cultivated and wild mung bean, the effect of timing and severity of water deficit on growth, development, yield accumulation and nitrogen fixation of mungbean, analysis of genotypic and environmental variation in international mungbean trials, adaptive responses of wild mungbean (vigna radiata ssp. sublobata) to photo-thermal environment. ii. ∗ growth, biomass, and seed yield, gene action for surrogate traits of water-use efficiency and harvest index in peanut (arachis hypogaea), related papers.
Showing 1 through 3 of 0 Related Papers
Global Status and Economic Importance of Mungbean
- First Online: 22 February 2020
Cite this chapter
- Ramakrishnan Nair 5 &
- Pepijn Schreinemachers 6
Part of the book series: Compendium of Plant Genomes ((CPG))
1021 Accesses
46 Citations
7 Altmetric
This chapter provides an overview of the economic importance of mungbean globally and the status of mungbean improvement research. The global mungbean area is about 7.3 million ha, and the average yield is 721 kg/ha. India and Myanmar each account for 30% of global output of 5.3 million t. Other large producers are China, Indonesia, Thailand, Kenya, and Tanzania. The mungbean market is divided in four main segments by usage: dry grains (important in South Asia and Kenya), sprouts (important in East and Southeast Asia), transparent noodles/starch (important in East and Southeast Asia), and paste (important in East Asia). Mungbean research is under-resourced in most countries as it is considered a minor crop. There is a history of strong international collaboration in mungbean improvement research in Asia, which is particularly important for a minor crop like mungbean as no single country has the capacity to cover all aspects requiring research. The International Mungbean Improvement Network was established in 2016 to further this collaboration and is coordinated by the World Vegetable Center.
This is a preview of subscription content, log in via an institution to check access.
Access this chapter
Subscribe and save.
- Get 10 units per month
- Download Article/Chapter or eBook
- 1 Unit = 1 Article or 1 Chapter
- Cancel anytime
- Available as PDF
- Read on any device
- Instant download
- Own it forever
- Available as EPUB and PDF
- Compact, lightweight edition
- Dispatched in 3 to 5 business days
- Free shipping worldwide - see info
- Durable hardcover edition
Tax calculation will be finalised at checkout
Purchases are for personal use only
Institutional subscriptions
Similar content being viewed by others
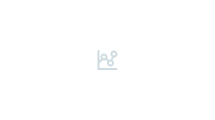
Mungbean in Southeast Asia and East Africa: varieties, practices and constraints
Biofortification of Mungbean
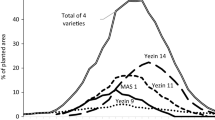
Impact and returns on investment of mungbean research and development in Myanmar
Abd El-Salam MS, El-Metwally IM, Abd El-Ghany HM, Hozayn M (2013) Potentiality of using mung bean as a summer legume forage crop under Egyptian condition. J Appl Sci Res 9(2):1238–1243
Google Scholar
Ali M, Malik IA, Sabir HM, Ahmad B (1997) The mungbean green revolution in Pakistan. Technical Bulletin No. 24, AVRDC. Shanhua, Taiwan, p 66
AMA (2014) Australian mungbean industry strategic plan 2015–2019. Queensland, Australian Mungbean Association
Cao D, Li H, Yi J, Zhang J, Che H, Cao J et al (2011) Antioxidant properties of the mungbean flavonoids on alleviating heat stress. PLOS One 6:e21071
Article CAS Google Scholar
Chen WL (2003) Republic of China. In: Shanmugasundaram S (ed) Processing and utilization of legumes. Report of the APO seminar on processing and utilization of legumes Japan, Chapter 2. Asian Productivity Organization, Tokyo, Japan, 9–14 Oct 2000
Dahiya PK, Linnemann AR, Van Boekel MAJS, Khetarpaul N, Grewal RB, Nout MJR (2015) Mung bean: technological and nutritional potential. Crit Rev Food Sci Nutr 55:670–688
Haque M, Miah MM, Ali A, Luna A (2014) Adoption of mungbean technologies and technical efficiency of mungbean ( Vigna radiata ) farmers in selected areas of Bangladesh. Bangladesh J Agric Res 39:113–125
Article Google Scholar
Kim DK, Jeong SC, Gorinstein S, Chon SU (2012) Total polyphenols, antioxidant and antiproliferative activities of different extracts in mungbean seeds and sprouts. Plant Foods Hum Nutr 67:71
Lee SH (2003) Republic of Korea. In: Shanmugasundaram S (ed) Processing and utilization of legumes. Report of the APO seminar on processing and utilization of legumes Japan, Chapter 7. Asian Productivity Organization, Tokyo, Japan, 9–14 Oct 2000
Ministry of Agriculture, Livestock and Irrigation (2016) Myanmar agriculture at a glance 2016. Department of Planning, Ministry of Agriculture, Livestock and Irrigation, Nay Pyi Taw
Nair RM, Giri RR, Boddepalli VN, Prasad KVSV, Devulapalli R, Blümmel M (2019) Variation in grain yield, fodder quality and animal intake in two dual purpose legume crops: mungbean and vegetable soybean grown in semi-arid tropical India. Legume Res. https://doi.org/10.18805/LR-4078
Nair RM, Schafleitner R, Kenyon L, Srinivasan R, Easdown W, Ebert AW (2012) Genetic improvement of mungbean. SABRAO J Breed Genet 44:177–190
Pataczek L, Zahir Z, Ahmad M, Rani S, Nair R, Schafleitner R, Cadisch G, Hilger T (2018) Beans with benefits—the role of mungbean ( Vigna radiata ) in a changing environment. Am J Plant Sci 9:1577–1600. https://doi.org/10.4236/ajps.2018.97115
Rani S, Schreinemachers P, Kuziyev B (2018) Mungbean as a catch crop for dryland systems in Pakistan and Uzbekistan: a situational analysis. Cogent Food Agric 4:1499241
Schafleitner R, Nair RM, Rathore A, Wang YW, Lin CY, Chu SH et al (2015) The AVRDC—the world vegetable center mungbean ( Vigna radiata ) core and mini core collections. BMC Genom 16:344
Schreinemachers P, Ebert A, Wu MH (2014) Costing the ex situ conservation of plant genetic resources at AVRDC—the world vegetable center. Genetic Res Crop Evol 61:757–773
Schreinemachers P, Sequeros T, Rani S, Rashid MA, Gowdru NV, Rahman MS, Ahmed MR, Nair RM (2019) Counting the beans: quantifying the adoption of improved mungbean varieties in South Asia and Myanmar. Food Secur 11(3):623–634
Shanmugasundaram S (2003) Present situation and economic importance of legume in Asia and Pacific region. In: Processing and utilization of legumes. Report of the APO seminar on processing and utilization of legumes Japan. Asian Productivity Organization, Tokyo, Japan, pp 17–52, 9–14 Oct 2000
Shanmugasundaram S, Keatinge JDH, d’Arros Hughes J (2009) The mungbean transformation. Diversifying crops, defeating malnutrition. In: Spielman DJ, Pandya-Lorch R (eds) Millions fed: proven successes in agricultural development. International Food Policy Research Institute, Washington, DC, pp 103–108
USDA (2009) China—peoples republic of, edible bean annual. Global Agricultural Information Network (GAIN), GAIN Report Number: CH9088. USDA Foreign Agricultural Service, Washington, DC
USDA (2014) Thailand—grain and feed annual. Global Agricultural Information Network (GAIN), GAIN Report Number: TH4021. USDA Foreign Agricultural Service, Washington, DC
Download references
Author information
Authors and affiliations.
World Vegetable Center, South Asia, ICRISAT Campus, Patancheru, Hyderabad, Telangana, India
Ramakrishnan Nair
World Vegetable Center, East and Southeast Asia, Bangkok, Thailand
Pepijn Schreinemachers
You can also search for this author in PubMed Google Scholar
Corresponding author
Correspondence to Ramakrishnan Nair .
Editor information
Editors and affiliations.
World Vegetable Center, ICRISAT Campus, Hyderabad, Telangana, India
Ramakrishnan M. Nair
World Vegetable Center, Shanhua, Tainan, Taiwan
Roland Schafleitner
College of Agriculture and Life Sciences, Seoul National University, Seoul, Korea (Republic of)
Rights and permissions
Reprints and permissions
Copyright information
© 2020 Springer Nature Switzerland AG
About this chapter
Nair, R., Schreinemachers, P. (2020). Global Status and Economic Importance of Mungbean. In: Nair, R., Schafleitner, R., Lee, SH. (eds) The Mungbean Genome. Compendium of Plant Genomes. Springer, Cham. https://doi.org/10.1007/978-3-030-20008-4_1
Download citation
DOI : https://doi.org/10.1007/978-3-030-20008-4_1
Published : 22 February 2020
Publisher Name : Springer, Cham
Print ISBN : 978-3-030-20007-7
Online ISBN : 978-3-030-20008-4
eBook Packages : Biomedical and Life Sciences Biomedical and Life Sciences (R0)
Share this chapter
Anyone you share the following link with will be able to read this content:
Sorry, a shareable link is not currently available for this article.
Provided by the Springer Nature SharedIt content-sharing initiative
- Publish with us
Policies and ethics
- Find a journal
- Track your research
- Open access
- Published: 09 October 2021
Nutritional, phytochemical and antioxidant properties of 24 mung bean ( Vigna radiate L.) genotypes
- Fuhao Wang 1 , 2 na1 ,
- Lu Huang 1 na1 ,
- Xingxing Yuan 1 ,
- Xiaoyan Zhang 1 ,
- Luping Guo 3 ,
- Chenchen Xue 1 &
- Xin Chen ORCID: orcid.org/0000-0002-8292-7699 1 , 2 , 3
Food Production, Processing and Nutrition volume 3 , Article number: 28 ( 2021 ) Cite this article
6469 Accesses
8 Citations
Metrics details
This study aimed to investigate the proximate and phytochemicals present in seeds of 24 mung bean ( Vigna radiate L.) genotypes from four provinces of China for estimating their nutritional and antioxidant properties. Proximate analysis of mung bean genotypes revealed that starch, protein, fat, ash and water-soluble polysaccharide ranged from 39.54–60.66, 17.36–24.89, 4.24–12.18, 2.78–3.53 and 1.99–2.96 g/100 g respectively. The five principal fatty acids detected in mung beans were stearic acid, palmitic acid, linoleic acid, oleic acid, and linolenic acid. The contents of insoluble-bound phenolic compounds, soluble phenolic compounds, and flavonoids ranged from 0.78 to 1.5 mg GAE g − 1 , 1.78 to 4.10 mg GAE g − 1 , and 1.25 to 3.52 mg RE g − 1 , respectively. The black seed coat mung bean genotype M13 (Suheilv 1) exhibited highest flavonoid and phenolic contents which showed strong antioxidant activity. Two flavonoids (vitexin and isovitexin) and four phenolic acids (caffeic, syringic acid, p -coumaric, and ferulic acids) were identified by HPLC. Vitexin and isovitexin were the major phenolic compounds in all mung bean genotypes. The content of soluble phenolic compounds had positive correlation with DPPH ( r 2 = 0.713) and ABTS ( r 2 = 0.665) radical scavenging activities. Principal component analysis indicated that the first two principal components could reflect most details on mung bean with a cumulative contribution rate of 66.1%. Twenty-four mung bean genotypes were classified into four groups based on their phenolic compounds contents and antioxidant activities. The present study highlights the importance of these mung bean genotypes as a source of nature antioxidant ingredient for the development of functional foods or a source of health promoting food.
Graphical Abstract

Introduction
Mung bean ( Vigna radiate L.) is a species of Fabaceae plant which is well-known as green gram (Ganesan & Xu 2018 ). It has been widely grown in the Southeast Asia and is very common in consumer products around the world. Mung beans are rich in protein, starch, cellulose, minerals, and vitamins (Khaket et al. 2015 ). Studies have shown that mung bean has physiological functions such as anti-obesity, anti-oxidation, and anti-bacterial (Yao et al., 2013 ). Mung bean provides a high-quality natural plant protein source which has been used as a substitute for meat and milk protein in many underdeveloped countries (Connolly et al. 2015 ; Du et al. 2018 ). Studies have shown that mung bean polysaccharide has antioxidant and immunomodulatory activities (Lai et al. 2010 ). Moreover, the essential fatty acids (FAs) contained in mung beans can promote the growth and development of the body. In addition to its nutritional importance, mung beans are a rich source of phytochemicals including phenols and flavonoids that show health promoting effects such as antioxidants, anti-tumor and anti-radiation (Randhir & Shetty 2007 ; Soucek et al. 2005 ). Phenolic phytochemicals are the largest category of phytochemicals and the most widely distributed in plant (King & Young 1999 ). They mainly exist in free, soluble bound and insoluble bound forms (Alshikh et al. 2015 ; Jung et al. 2002 ).
Mung beans are commonly consumed food legume in Asian countries. Seeds of mung beans have been used to prepare a variety of fresh, fermented and dried foods. They are very popular foods in China and are known as “green pearls.” Mung beans are often used in food processing, such as mung bean porridge, mung bean soup, bean paste, mung bean cake, and raw bean sprouts. They can also be used as jelly, noodles, and vermicelli due to their high starch content. In addition, they are good raw materials for famous wines. Mung bean protein has excellent functional properties such as solubility, water retention, emulsification, gelation, foaming, and foam stability. It has application prospects in flour products, meat products, dairy products, and beverages in the food processing industry. Protein beverages (protein milk, coffee bean milk) made from protein isolates have high nutritional value and high-quality.
Following a country-wide collection during the 1980′s, more than 5000 accessions of mung bean germplasm have been deposited in the National Crop Genebank of China (Liu et al. 2006 ; Wang et al. 2018 ). In despite of the abundant germplasm resources of mung bean, the diversity of nutritional composition is still unknown. Due to importance of mung bean and its products, it is necessary to investigate nutritional and phytochemical compounds across various genotypes. In this study, 24 mung beans genotypes from four provinces in China were collected for the following purposes: (1) compare their nutritional compositions, (2) analyze their phytochemicals contents, and (3) evaluate correlations between phytochemical compounds and antioxidant activities. Results of this study will provide a good basis for the assessment and application of different mung bean genotypes.
Materials and methods
Twenty-four mung bean genotypes (Fig. 1 ) were collected from four provinces: Shanxi (north of China), Shaanxi (northwest of China), Jiangsu (east of China), and Hebei (north of China). We selected six mung bean genotypes per province. Among them, M12-M18 genotypes come from our lab. Name and geographical origin of these genotypes are presented in Table 1 . Seeds of these genotypes were grown in the Liuhe Base of Jiangsu Academy of Agricultural Sciences.
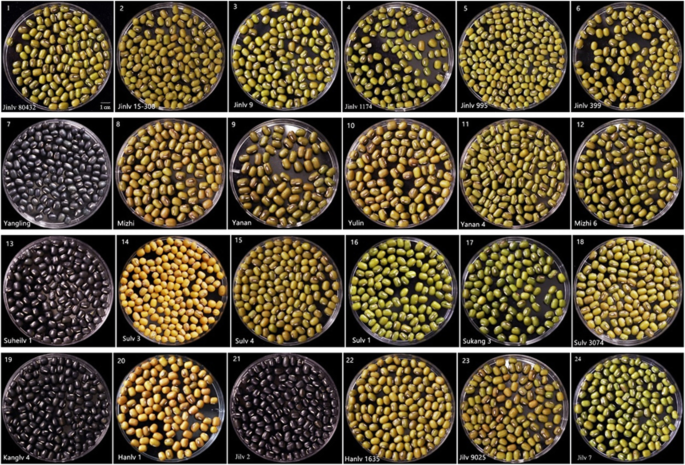
Photos of 24 mung bean genotypes (M1–24)
2, 2-diphenyl-1-picrylhydrazyl (DPPH), 2, 2-azinobis (3-ethylbenzothiazoline-6-sulfonic acid) diammonium salt (ABTS), ascorbic acid (vitamin C, Vc), Folin–Ciocalteu’s phenol reagent were purchased from Sigma-Aldrich Chemical Co. (St. Louis, MO, USA). All other reagents used were of analytical grade and purchased from Sodebio Reagent Co., Ltd. (Nanjing, Jiangsu, China).
Analysis of nutrient components
Protein content was determined by using Kjeldahl method with Kjeltec TM2300 Auto Sampler System (Foss Analytical, Hillerød, Denmark) (Thiex et al. 2002 ). Soxhlet extraction (Extraction System B-811, Buchi, Flawil, Sankt Gallen, Switzerland) with petroleum ether was used to determine crude fat content (AOAC 1990 ). Ash content was determined by using the combustion method with an electric muffle furnace (SX2–4-13, Leiyun Instruments, Shanghai, China) (AOAC 1990 ). Starch content was determined using a starch kit (BC0700, Solarbio Science & Technology Co., Ltd., Beijing, China).
Fatty acid composition
Fatty acid (FA) composition was determined according to the method of Zhang et al. ( 2013 ) with slight modifications. Briefly, 0.5 g mung bean powder was added in a 2 mL centrifuge tube with 1.5 mL n-hexane. The mixture was left overnight and then centrifuged at 8200 g for 5 min (Eppendorf Centrifuge 5804R, Hamburg, Germany). Then, 350 μL of sodium methoxide solution was added and vortexed for 1 h. After centrifugation, the supernatant was as used for high-performance gas chromatography analysis (Agilent 7890B, Agilent Technologies Inc., Wilmington, DE, USA).
Determination of water-soluble polysaccharide (WSP)
The WSP was determined according to Yao et al. ( 2016 ) with several modifications. The mung bean powder was extracted with 80% ethanol for 1 h and then centrifuged at 2000 g for 5 min. The supernatant was removed and extracted twice with distilled water at 90 °C for 3 h. After centrifugation, the supernatant was collected with Sevag reagent (chloroform:n-butanol = 4:1,v/v) and shaken for 10 min. The mixture was centrifuged at 2000 g for 5 min. The lower organic solvent and protein at the interface were then removed. The gelatin was denatured, and the upper polysaccharide solution was retained.
Preparation of standard curve: 10 mg of glucose standard solution was accurately weighed and dissolved in 10 mL to obtain 1 mg/mL glucose standard solution. The solution was diluted to 10, 20, 40, 60, 80, and 100 μg/mL. Then, 1.0 mL of the diluted solution was drawn, and 1 mL of distilled water was added. Immediately thereafter, 1.0 mL of a 6% phenol solution was added, and 5.0 mL of concentrated sulfuric acid was slowly added and shaken while adding. After mixing well, the solution was left to stand at room temperature for 20 min. The absorbance of the solution was measured at 490 nm by a spectrophotometer (Alpha-1101 m, Puyuan Instruments, Shanghai, China). Next, 1.0 mL of polysaccharide sample solution with certain concentration was drawn, and distilled water was added to make it 2.0 mL. The subsequent operation was the same as glucose labeling. The purity of the corresponding polysaccharide was calculated according to its absorbance value and standard curve.
Extract analysis
Preparation of ethanol extracts.
Mung bean powder (1 g) was mixed with 25 mL of 80% ethanol solution and extracted in a water bath for 4 h. The solution was cooled to room temperature and centrifuged at 15000 g for 15 min. The operation was repeated 2–3 times, and the obtained supernatant was mixed and concentrated. The volume was made to reach 20 mL with methanol and stored at − 20 °C for testing. The collected supernatants were examined for soluble phenolic compounds.
Determination of total flavonoid content
Total flavonoid content (TFC) was determined according to the method reported by Xie et al. ( 2015 ). The sample extract (250 μL) was mixed with 1.25 mL of distilled water, and 25 μL of 5% NaNO 2 was added to react for 6 min. Then, 150 μL of 10% AlCl 3 was added to react for 5 min. Finally, 0.5 mL of 1 M NaOH and 275 μL of distilled water were added to the mixture, and the absorbance was measured at 510 nm after 10 min. Rutin with different concentrations was plotted as the standard curve, and the results were expressed as rutin equivalents (RE).
The content of soluble phenolic compounds
The content of soluble phenolic compounds in the samples was determined by using the Folin-Ciocalteu method. The sample solution (400 μL) and 4.6 mL of deionized water were placed in a test tube, and 1 mL of Folin-Ciocalteu and 24 mL of 7.5% (w/v) of sodium carbonate solution were injected into the test tube. After reacting for 2 h at room temperature, the absorbance of the sample was measured at a wavelength of 760 nm. The standard curve was configured with different concentrations of gallic acid, and the results were expressed as gallic acid equivalents (GAE).
The content of insoluble-bound phenolic compounds
Extraction of insoluble-bound phenolic compounds was performed according to the method reported by Zhang et al. ( 2013 ). Briefly, 20 mL of NaOH (2 mol/L) was mixed with the residue left after extracting soluble phenolic compounds and incubated for 1 h at room temperature. The mixture was centrifuged at 4500 g for 5 min, and the supernatant was extracted and adjusted to pH 2 with HCl (6 mol/L). Then, an equal volume of ethyl acetate was added for extraction, and the extraction was repeated 3 times. The extract was mixed, evaporated to dryness by a rotary evaporator at 45 °C, reconstituted to 5 mL of methanol, and determined by using the Folin-Ciocalteu method.
High-performance liquid chromatography (HPLC) analysis of phenolic compounds
Agilent 1260 high performance liquid chromatography (Agilent Technologies Inc. Santa Clara, CA, USA) equipped with the Agilent Poroshell 120 EC-C18 Column was used to detect the main phenolic compounds in mung bean seeds. The mobile phase consisted of acetonitrile (A) and ultrapure water containing 0.1% trifluoroacetic acid (B). Gradient elution was performed as follows: 0–7 min, 5–30% A; 7–15 min, 30–40% A; 15–25 min, 40–50% A; 25–30 min, 50–95% A; 30–35 min, 95–5% A. The flow rate was set at 1.0 mL min − 1 and the injection volume was 10 μL. The column was operated at 30 °C. The wavelength of the detector was set at 280 nm. Quantification of phenolic compounds was carried out by an external standard method using calibration curves.
Evaluation of antioxidant capacity
Assay of dpph radical scavenging activity.
DPPH analysis was executed in line with the report of Chai et al. ( 2018 ). Briefly, DPPH was dissolved in methanol. Then, 0.5 mL of DPPH (0.4 mmol/L) and 0.5 mL of the extract were mixed to react in the dark at room temperature for 30 min. The absorbance was measured at 517 nm with a spectrophotometer. The results were expressed as μmol vitamin C per gram samples.
Assay of ABTS radical cation scavenging activity
ABTS radical cation scavenging assay was performed according to the method reported by Lee et al. ( 2011 ) with some modifications. ABTS radical ions were produced by mixing 7 mM ABTS aqueous solution with 2.45 mM K 2 S 2 O 8 aqueous solution, storing in the dark for 16 h, diluting 20 times with absolute ethanol before use, and storing at 30 °C. Next, 1.2 mL of ABTS ethanol solution was mixed with 300 μL of extract to react at 30 °C for 6 min. The absorbance was measured at 734 nm with a spectrophotometer. The results were expressed as μmol vitamin C per gram.
Hydroxyl radical scavenging ability
The hydroxyl radical scavenging ability was determined according to Xiao et al. ( 2015 ) with simple modifications. Briefly, 300 μL of FeSO 4 (9 mmol/L), 300 μL of H 2 O 2 and 300 μL of the extract were mixed. After shaking, the mixture was added with 300 μL of salicylic acid-ethanol (9 mmol/L) and incubated at 37 °C for 30 min. The absorbance was measured at 510 nm. The results were expressed as μmol vitamin C per gram samples.
Statistical analysis
All experiments were conducted in triplicate and results were expressed as mean ± standard deviation. The statistical significance of the results was obtained by one-way analysis of variance and PCA using SPSS version 21.0 software. The Pearson correlation coefficient was used to estimate the correlation between phytochemical compounds and antioxidant activities. PCA was used to assess the contribution of chemical components to mung beans.
Results and discussion
Nutritional compositions.
Protein and starch are the two most abundant nutritional components in mung bean seed. In this study, the protein and starch contents of 24 mung bean genotypes ranged from 17.36 to 24.89 g/100 g and 39.54 g/100 g to 60.66 g/100 g (Table 2 ), respectively. M6 (Jinlv 399) showed the highest contents of protein (24.89 g/100 g) and starch (60.66 g/100 g) which could be used for specific food processing, such as noodles and vermicelli. Compared with other legumes, mung beans have a higher carbohydrate content, predominantly starch (Tang et al. 2014 ). The contributions of ash were in the ranges of 2.78 to 3.53 g/100 g. The content of water-soluble polysaccharide (WSP) ranged from 1.99 to 2.96 g/100 g. M14 (Sulv 3) exhibited the highest content of WSP, while M2 showed the lowest value. The biological activity of polysaccharides has received attention, such as antioxidant and immunological activities.
Table 2 shows the crude fat content and FA composition of 24 kinds of mung beans. The content of crude fat ranged from 4.24 to 12.18 mg/g. High-performance gas chromatography was used for the analysis of FA in mung bean. Five principal FAs were observed and identified according to standards (Fig. 2 ). Peaks 1, 2, 3, 4 and 5 with the retention time of 2.402, 3.187, 3.318, 3.602 and 3.953 min were referred to as palmitic acid, stearic acid, oleic acid, linoleic acid and linolenic acid, respectively. Linoleic acid was the most abundant in mung bean and accounted for 38.95–44.74 percentage of the total fatty acids. A similar observation was reported by Anwar et al. ( 2007 ) who investigated fatty acid composition of different mung bean cultivars grown in Pakistan. Studies have shown that polyunsaturated fatty acids are important structural substances in the retina and neurons which can protect the vision. Polyunsaturated fatty acids have important physiological functions in human metabolism, such as esterification of cholesterol, lowering blood cholesterol and triglycerides (Yates et al. 2014 ).
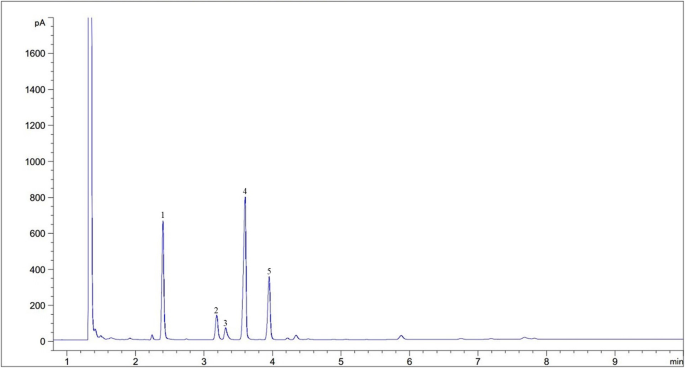
High performance gas chromatogram of fatty acids in mung bean. Peaks 1, 2, 3, 4 and 5 were identified as palmitic acid, stearic acid, oleic acid, linoleic acid and linolenic acid
Contents of phytochemicals (total flavonoid content, insoluble-bound phenolic content and soluble phenolic content)
As shown in Table 3 , the total flavonoid content of 24 mung bean genotypes ranged from 1.25 to 3.52 mg RE g − 1 . M13 (Suheilv 1) presented the highest flavonoid content (3.52 mg RE g − 1 ) and M5 (Jinlv 995) presented the lowest flavonoid content. Flavonoids are general name of a group of chemicals including catechins, anthocyanidins, proanthocyanidins, flavonols, isoflavonoids and flavones (Luo et al. 2016 ). Flavonoids have important physiological functions such as anti-oxidation and anti-inflammatory (Zuk et al. 2019 ). Zhang et al. ( 2013 ) used acetone and water as extraction solvents, and the highest flavonoid content obtained was 6.0 mg g − 1 . This may be different from the extraction solvent. The nature of the raw materials itself also has a relationship, such as the difference in the color of the skin and the difference in maturity.
The contents of soluble and insoluble-bound phenolic compounds in 24 mung bean genotypes investigated are shown in Table 3 . The phenolic content of the soluble fraction ranged from 1.78 to 4.10 mg GAE g − 1 . Insoluble-bound phenolic content ranged from 0.78 to 1.5 mg GAE g − 1 . M13 genotype exhibited the highest content of both soluble and insoluble-bound phenolic compounds. Meanwhile, M22 had the lowest soluble phenolic content of 1.78 mg GAE g − 1 and M8 exhibited the lowest insoluble-bound phenolic content of 0.78 mg GAE g − 1 . The difference between soluble and insoluble-bound phenolic contents is significant, which is similar to Wang et al. ( 2016 ). A similar result was reported by de Camargo et al. ( 2015 ) who observed that the content of soluble phenolic compounds (free and esterified) was significantly higher than insoluble-bound fraction in peanut skin. The amount of phenolic compound is affected by genotype, agronomic habits (irrigation, fertilization, and pest management), harvest maturity, post-harvest storage, and climatic conditions (Mattila et al. 2005 ).
Identification of major phenolic compounds in mung bean seeds
Soluble and insoluble-bound phenolic compounds in mung bean seeds were identified by HPLC as shown in Fig. 3 and Table 4 . Peaks 1, 2, 3, 4, 5 and 6 with the retention time of 17.785, 18.534, 24.160, 29.017 and 29.731 min were referred to as caffeic acid, syringic acid, p -coumaric acid, ferulic acid, vitexin and isovitexin, respectively (Fig. 3 ). In the present study, six phenolic compounds were identified, including four phenolic acids (syringic, caffeic, p -coumaric, and ferulic acids) and two flavonoids (vitexin and isovitexin). Results showed that vitexin and isovitexin were the dominant phenolic compounds in all mung bean genotypes. This is consistent with a previous study (Yang et al. 2020 ). The vitexin content of soluble and insoluble-bound fractions ranged from 481.02 to 910.26 μg g − 1 and from 123.77 to 463.25 μg g − 1 , respectively. The isovitexin content of soluble and insoluble-bound fractions ranged from 568.57 to 1572.74 μg g − 1 and from 104.42 to 421.77 μg g − 1 , respectively. The isovitexin content in the soluble fractions of mung bean seeds were higher than their corresponding vitexin content. M13 showed the highest vitexin (910.26 μg g − 1 ) and isovitexin (1572.74 μg g − 1 ) contents in the soluble fractions of all studied mung beans. Large differences were found among all mung beans in the contents of both soluble and insoluble-bound flavonoids. The vitexin and isovitexin contents in the soluble fractions of mung beans are higher than that in the insoluble-bound fractions. The contents of individual phenolic acids in different bean varieties are also shown in Table 4 . Contrary to flavonoids, the contents of phenolic acids in the soluble fractions are lower than that in the insoluble-bound fractions. Shi et al. ( 2016 ) also reported that the average content of bound phenolic acids in the mung bean samples accounted for 89.8% of the total amount of phenolic acids. Insoluble-bound phenolic compounds can survive upper gastrointestinal digestion and are released from the colon by the effect of microorganism. Results suggested that caffeic acid was the major phenolic acid in mung bean cultivars, ranged from 2.45 to 15.12 μg g − 1 in the soluble fractions and from 2.38 to 16.46 μg g − 1 in the insoluble-bound fractions, respectively. Caffeic acid is an effective scavenger of the ABTS + and DPPH radicals, which has strong antioxidant activity (Gülin 2006 ). Syringic acid was not detected in all mung bean varieties except M18. Flavonoids and phenolic acids are regarded as the major compounds contributing to the total antioxidant activities of mung bean seeds (Shi et al. 2016 ).
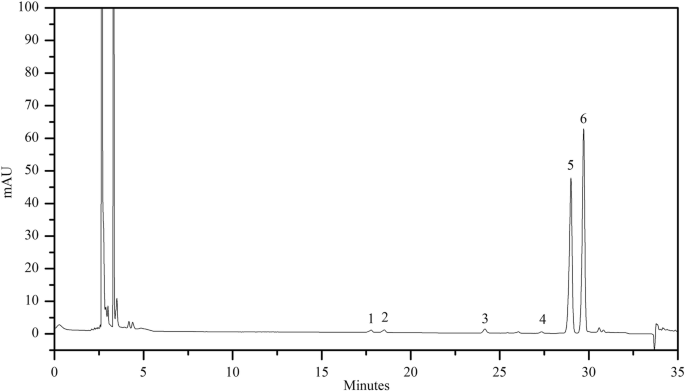
High-performance liquid chromatogram of phenolic compounds in mung bean. Peaks 1, 2, 3, 4, 5 and 6 were identified as caffeic acid, syringic acid, p -coumaric acid, ferulic acid, vitexin and isovitexin

Antioxidant activity
The results of antioxidant activities are presented in Fig. 4 . Soluble phenolic compounds showed stronger antioxidant activity than insoluble-bound phenolic compounds. M23 (Jilv 9025) exhibited the strongest DPPH free radical scavenging ability (Fig. 4 a) for soluble phenolic compounds (4.44 μmol/g). At the same time, M13 had the strongest DPPH free radical scavenging ability for the insoluble-bound fraction (3.73 μmol/g). The antioxidant activity can be influenced by many factors and cannot be fully described with one single method. These commonly used methods have their advantages and disadvantages for measuring antioxidant activity. Therefore, in this study, the radical scavenging performance was also evaluated by using the ABTS radical cation and hydroxyl radical assays. Regardless of the soluble or insoluble-bound phenolic compounds, M13 (Suheilv 1) genotype showed the highest ABTS radical scavenging ability (Fig. 4 b). The result showed that the ability of soluble phenolic compounds to scavenge hydroxyl radicals ranged from 10.38 to 15.54 μmol/g (Fig. 4 c). However, the insoluble-bound phenolic compounds exhibited significantly lower hydroxyl radical scavenging activity. Free radicals will seize the electrons of biomolecules, causing the biomolecules to be altered and cause various diseases, such as inflammation, aging, and cardiovascular diseases. Phenolic compounds can act as hydrogen or electron donors when reacting with oxidative substances (Luo et al. 2016 ). Therefore, they present strong free radical scavenging activity and antioxidant activity. In general, results indicated that the black seed coat mung bean genotype M13 from our lab presented the strongest antioxidant activity.
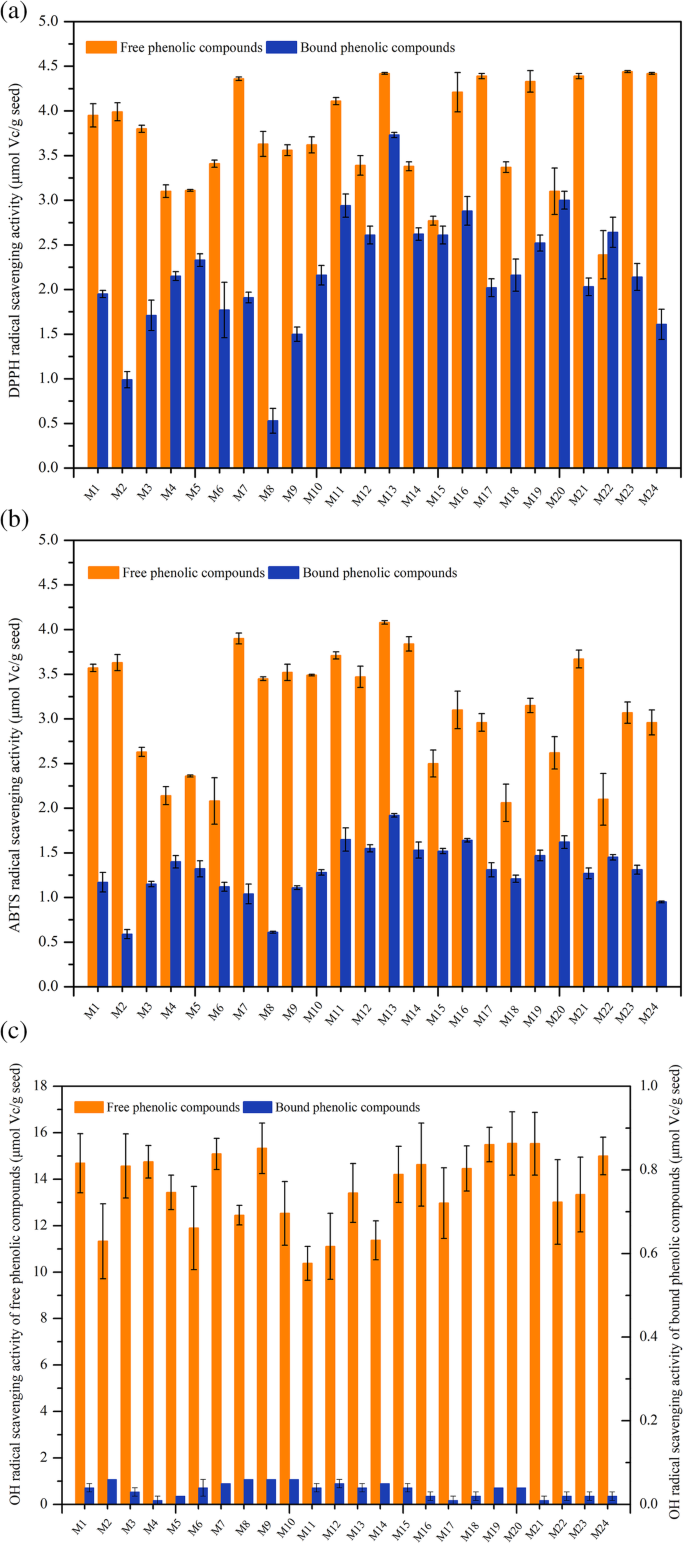
Antioxidant activities of different mung bean genotypes (mean ± SD, n = 3), a DPPH radical scavenging activity; b ABTS radical cation scavenging activity; c hydroxyl radical scavenging ability. Results were expressed as μmol VC/g
Correlation of antioxidant activity with the contents of soluble phenolic compounds and flavonoids
As shown in Table 5 , soluble phenolic content has significantly high correlation with DPPH ( r 2 = 0.713) and ABTS ( r 2 = 0.665) radical scavenging activities. Flavonoid content is significantly correlated with DPPH ( r 2 = 0.463) radical scavenging activity. No evident correlation is observed between hydroxyl radical scavenging ability and phytochemical contents. A high correlation between the content of phenolic compounds and antioxidant activity has also been previously demonstrated by Shi et al. ( 2016 ).
- Principal component analysis
Data of phenolic compounds content, flavonoid content and antioxidant activities of the 24 mung bean genotypes were subjected to principal component analysis (PCA). As shown in Fig. 5 , the first two principal components explained 66.1% of the total variation (R2X [1] =37.9% and R2X [2] =28.2%). The PCA scatter plot revealed the dispersion between the 24 mung bean genotypes. M13 genotype had the largest deviation from the other genotypes, presenting the highest antioxidant activity, phenolic compounds content and flavonoid contents. The longest distance between M13 and M8 showed significant differences in terms of phytochemical contents and antioxidant activities. Principal component analysis indicated that although different mung beans had similar growth environment, they could show significantly differences in phytochemical and antioxidant properties because of their different genotypes. The 24 mung beans were classified into four groups. Group 1 was characterized by high levels of TFC, phenolic contents, DPPH and ABTS free radical scavenging abilities, which contained only M13. Group 2 contained M4, M5, M15, M18 and M22. These genotypes mainly presented similar antioxidant activity. Results of PCA showed satisfactory separation of phenolic compounds and antioxidant activity of these genotypes, indicating that M13 is significantly different from other mung bean genotypes, and implying its potential nutritional and functional values in food processing. Results of this study could provide a good reference for the selection of mung bean genotypes in food production and processing.
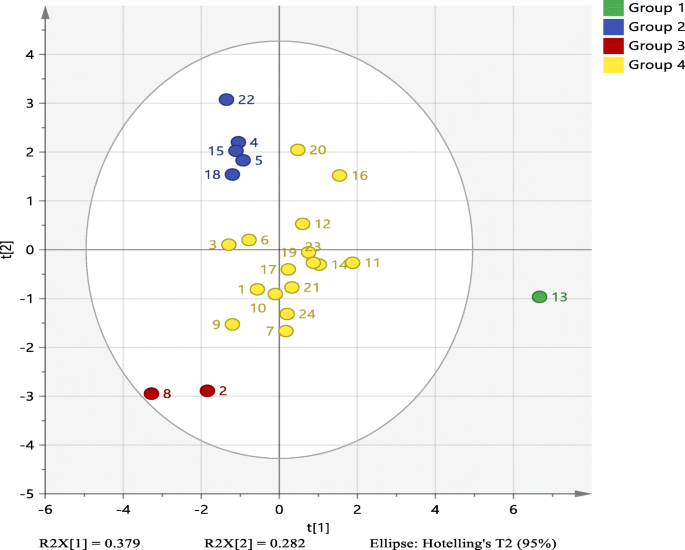
Principal component analysis based on the phenolic compounds content, flavonoid content and antioxidant activities of 24 mung bean genotypes
Conclusions
In this study, the nutritional composition, phytochemicals contents and correlations between phytochemical compounds and antioxidant activities of 24 mung bean genotypes from four provinces in China were investigated. The nutritional composition and phytochemical properties of 24 mung bean genotypes are different from each other. Starch, protein, fat, ash and water-soluble polysaccharide ranged from 39.54 to 60.66, 17.36 to 24.89, 4.24 to 12.18, 2.78 to 3.53 and 1.99 to 2.96 g/100 g respectively. M13 (Suheilv 1) showed the highest content of phytochemicals contents, such as flavonoids (3.52 mg RE g − 1 ), soluble phenolic compounds (4.10 mg GAE g − 1 ), and insoluble-bound phenolic compounds (1.50 mg GAE g − 1 ). Vitexin and isovitexin were identified by HPLC as the major phenolic compounds in all mung bean genotypes. M13 showed the highest vitexin (910.26 μg g − 1 ) and isovitexin (1572.74 μg g − 1 ) contents in the soluble fractions of all studied mung beans. Different assays were performed to judge the antioxidant activity of mung bean genotypes, and M13 exhibited strong DPPH and ABTS radical scavenging activities. According to principal component analysis, 24 mung bean samples were classified into four groups based on their phenolic compounds contents and antioxidant activity. The black seed coat mung bean genotype M13 could be used as a superior variety with high antioxidant capacity for functional food production and processing. Overall, this work provides useful information for the potential future application of different mung bean genotypes as source of functional and healthy food. More intensive studies are needed to identify the most effective chemical components in these investigated genotypes.
Availability of data and materials
The data presented in this study are available on request from the corresponding author.
Abbreviations
Gallic acid equivalents
Rutin equivalents
High-performance liquid chromatography
2, 2-diphenyl-1-picrylhydrazyl
2, 2-azinobis (3-ethylbenzothiazoline-6-sulfonic acid) diammonium salt
Water-soluble polysaccharide
Total flavonoid content
Alshikh, N., de Camargo, A. C., & Shahidi, F. (2015). Phenolics of selected lentil cultivars: Antioxidant activities and inhibition of low-density lipoprotein and DNA damage. Journal of Functional Foods , 18 , 1022–1038.
Article CAS Google Scholar
Anwar, F., Latif, S., Przybylski, R., Sultana, B., & Ashraf, M. (2007). Chemical composition and antioxidant activity of seeds of different cultivars of mung bean. Journal of Food Science , 72 , 503–510.
AOAC (1990). Official methods of analysis , (15th ed., ). Association of Official Analytical Chemists.
Google Scholar
Chai, W. M., Ou-Yang, C., Huang, Q., Lin, M. Z., Wang, Y. X., Xu, K. L., … Pang, D. D. (2018). Antityrosinase and antioxidant properties of mung bean seed proanthocyanidins: Novel insights into the inhibitory mechanism. Food Chemistry , 260 , 27–36.
Connolly, A., O'Keeffe, M. B., Piggott, C. O., Nongonierma, A. B., & FitzGerald, R. J. (2015). Generation and identification of angiotensin converting enzyme (ACE) inhibitory peptides from a brewers’ spent grain protein isolate. Food Chemistry , 176 , 64–71.
de Camargo, A. C., Regitano-d’Arce, M. A. B., Gallo, C. R., & Shahidi, F. (2015). Gamma-irradiation induced changes in microbiological status, phenolic profile and antioxidant activity of peanut skin. Journal of Functional Foods , 12 , 129–143.
Du, M. X., Xie, J. H., Gong, B., Xu, X., Tang, W., Li, X., … Xie, M. Y. (2018). Extraction, physicochemical characteristics and functional properties of mung bean protein. Food Hydrocolloids , 76 , 131–140.
Ganesan, K., & Xu, B. (2018). A critical review on phytochemical profile and health promoting effects of mung bean ( Vigna radiata ). Food Science and Human Wellness , 7 , 11–33.
Article Google Scholar
Gülin, I. (2006). Antioxidant activity of caffeic acid (3,4-dihydroxycinnamic acid). Toxicology , 217 , 213–220.
Jung, M. Y., Jeon, B. S., & Bock, J. Y. (2002). Free, esterified, and insoluble-bound phenolic acids in white and red Korean ginsengs ( Panax ginseng C.a. Meyer). Food Chemistry , 79 , 105–111.
Khaket, T. P., Dhanda, S., Jodha, D., & Singh, J. (2015). Purification and biochemical characterization of dipeptidyl peptidase-II (DPP7) homologue from germinated Vigna radiata seeds. Bioorganic Chemistry , 63 , 132–141.
King, A., & Young, G. (1999). Characteristics and occurrence of phenolic phytochemicals. Journal of the American Dietetic Association , 99 , 213–218.
Lai, F. R., Wen, Q. B., Li, A., Wu, L., & Li, H. (2010). Antioxidant activities of water-soluble polysaccharide extracted from mung bean ( Vigna radiata L.) hull with ultrasonic assisted treatment. Carbohydrate Polymers , 81 , 323–329.
Lee, J. H., Jeon, J. K., Kim, S. G., Kim, S. H., Chun, T., & Imm, J. (2011). Comparative analyses of total phenols, flavonoids, saponins and antioxidant activity in yellow soy beans and mung beans. International Journal of Food Science & Technology , 46 , 2513–2519.
Liu, C., Chen, X., Wang, S., Wang, L., Sun, L., Mei, L., & Xu, N. (2006). The genetic diversity of mungbean germplasm in China. Journal of Plant Genetic Resources , 7 , 459–463 (in Chinese with English abstract).
Luo, J., Cai, W., Wu, T., & Xu, B. (2016). Phytochemical distribution in hull and cotyledon of adzuki bean ( Vigna angularis L.) and mung bean ( Vigna radiate L.), and their contribution to antioxidant, anti-inflammatory and anti-diabetic activities. Food Chemistry , 201 , 350–360.
Mattila, P., Pihlava, J. M., & Hellström, J. (2005). Contents of phenolic acids, alkyl-and alkenylresorcinols, and avenanthramides in commercial grain products. Journal of Agricultural and Food Chemistry , 53 , 8290–8295.
Randhir, R., & Shetty, K. (2007). Mung beans processed by solid-state bioconversion improves phenolic content and functionality relevant for diabetes and ulcer management. Innovative Food Science & Emerging Technologies , 8 , 197–204.
Shi, Z., Yao, Y., Zhu, Y., & Ren, G. (2016). Nutritional composition and antioxidant activity of twenty mung bean cultivars in China. The Crop Journal , 4 , 398–406.
Soucek, J., Skvor, J., Pouckova, P., Matougek, J., Slavik, T., & Matousek, J. (2005). Mung bean sprout ( Phaseolus aureus ) nuclease and its biological and antitumor effects. Neoplasma , 53 , 402–409.
Tang, D., Dong, Y., Ren, H., Li, L., & He, C. (2014). A review of phytochemistry, metabolite changes, and medicinal uses of the common food mung bean and its sprouts ( Vigna radiata ). Chemistry Central Journal , 8 , 1–9.
Thiex, N. J., Manson, H., Anderson, S., & Persson, J. A. (2002). Determination of crude protein in animal feed, forage, grain, and oilseeds by using block digestion with a copper catalyst and steam distillation into boric acid: Collaborative study. Journal of AOAC International , 85 , 309–317.
Wang, L., Bai, P., Yuan, X., Chen, H., Wang, S., Chen, X., & Cheng, X. (2018). Genetic diversity assessment of a set of introduced mung bean accessions ( Vigna radiata L.). The Crop Journal , 6 , 207–213.
Wang, Y. K., Zhang, X., Chen, G. L., Yu, J., Yang, L. Q., & Gao, Y. Q. (2016). Antioxidant property and their free, soluble conjugate and insoluble-bound phenolic contents in selected beans. Journal of Functional Foods , 24 , 359–372.
Xiao, Y., Wang, L. X., Rui, X., Li, W., Chen, X. H., Jiang, M., & Dong, M. S. (2015). Enhancement of the antioxidant capacity of soy whey by fermentation with lactobacillus plantarum B1–6. Journal of Functional Foods , 12 , 33–44.
Xie, J. H., Dong, C. J., Nie, S. P., Li, F., Wang, Z. J., Shen, M. Y., & Xie, M. Y. (2015). Extraction, chemical composition and antioxidant activity of flavonoids from Cyclocarya paliurus (Batal.) Iljinskaja leaves. Food Chemistry , 186 , 97–105.
Yang, Q. Q., Ge, Y. Y., Gunaratne, A., Kong, K. W., Li, H. B., Gul, K., … Gan, R. Y. (2020). Phenolic profiles, antioxidant activities, and antiproliferative activities of different mung bean ( Vigna radiata ) varieties from Sri Lanka. Food Bioscience , 37 , 100705.
Yao, Y., Yang, X. S., Tian, J., Liu, C. Y., Cheng, X. Z., & Ren, G. X. (2013). Antioxidant and antidiabetic activities of black mung bean ( Vigna radiata L. ). Journal of Agricultural and Food Chemistry , 61 , 8104–8109.
Yao, Y., Zhu, Y. Y., & Ren, G. X. (2016). Antioxidant and immunoregulatory activity of alkali-extractable polysaccharides from mung bean. International Journal of Biological Macromolecules , 84 , 289–294.
Yates, C. M., Calder, P. C., & Rainger, G. E. (2014). Pharmacology and therapeutics of omega-3 polyunsaturated fatty acids in chronic inflammatory disease. Pharmacology & Therapeutics , 141 , 272–282.
Zhang, X. W., Shang, P. P., Qin, F., Zhou, Q., Gao, B. Y., Huang, H. Q., … Yu, L. L. (2013). Chemical composition and antioxidative and anti-inflammatory properties of ten commercial mung bean samples. LWT-Food Science and Technology , 54 , 171–178.
Zuk, M., Szperlik, J., Hnitecka, A., & Szopa, J. (2019). Temporal biosynthesis of flavone constituents in flax growth stages. Plant Physiology and Biochemistry , 142 , 234–245.
Download references
Acknowledgements
We wish to thank Dr. Wang Jialei from Central Laboratory in Jiangsu Academy of Agricultural Sciences for her help in the HPLC analysis.
This work was financed by earmarked fund for China Agriculture Research System (CARS-08-G15) and Jiangsu Agricultural Science and Technology Innovation Fund (CX (20) 2015).
Author information
Fuhao Wang and Lu Huang contributed equally to this work.
Authors and Affiliations
Institute of Industrial Crops, Jiangsu Academy of Agricultural Sciences, Nanjing, Jiangsu, China
Fuhao Wang, Lu Huang, Xingxing Yuan, Xiaoyan Zhang, Chenchen Xue & Xin Chen
College of Food Science and Engineering, Nanjing University of Finance and Economics, Nanjing, Jiangsu, China
Fuhao Wang & Xin Chen
School of Food and Biological Engineering, Jiangsu University, Zhenjiang, Jiangsu, China
Luping Guo & Xin Chen
You can also search for this author in PubMed Google Scholar
Contributions
Wang F. and Huang L. conducted the experiments, analyzed the data and drafted the manuscript. Yuan X. and Zhang X. helped to process the data. Guo L. took care of the production of the samples. Chen X. and Xue C. supervised the project, conceived the project idea and reviewed the manuscript. All authors have read and approved the final manuscript.
Corresponding authors
Correspondence to Chenchen Xue or Xin Chen .
Ethics declarations
Ethical approval and consent to participate.
Not applicable.
Consent for publication
Competing interests.
The authors declare that they have no competing interests.
Additional information
Publisher’s note.
Springer Nature remains neutral with regard to jurisdictional claims in published maps and institutional affiliations.
Rights and permissions
Open Access This article is licensed under a Creative Commons Attribution 4.0 International License, which permits use, sharing, adaptation, distribution and reproduction in any medium or format, as long as you give appropriate credit to the original author(s) and the source, provide a link to the Creative Commons licence, and indicate if changes were made. The images or other third party material in this article are included in the article's Creative Commons licence, unless indicated otherwise in a credit line to the material. If material is not included in the article's Creative Commons licence and your intended use is not permitted by statutory regulation or exceeds the permitted use, you will need to obtain permission directly from the copyright holder. To view a copy of this licence, visit http://creativecommons.org/licenses/by/4.0/ .
Reprints and permissions
About this article
Cite this article.
Wang, F., Huang, L., Yuan, X. et al. Nutritional, phytochemical and antioxidant properties of 24 mung bean ( Vigna radiate L.) genotypes. Food Prod Process and Nutr 3 , 28 (2021). https://doi.org/10.1186/s43014-021-00073-x
Download citation
Received : 16 July 2021
Accepted : 31 August 2021
Published : 09 October 2021
DOI : https://doi.org/10.1186/s43014-021-00073-x
Share this article
Anyone you share the following link with will be able to read this content:
Sorry, a shareable link is not currently available for this article.
Provided by the Springer Nature SharedIt content-sharing initiative
- Phytochemicals
- Antioxidant properties
- Proximate analysis
- Mung bean genotypes
Food Production, Processing and Nutrition
ISSN: 2661-8974
- Submission enquiries: Access here and click Contact Us
- General enquiries: [email protected]

An official website of the United States government
The .gov means it’s official. Federal government websites often end in .gov or .mil. Before sharing sensitive information, make sure you’re on a federal government site.
The site is secure. The https:// ensures that you are connecting to the official website and that any information you provide is encrypted and transmitted securely.
- Publications
- Account settings
Preview improvements coming to the PMC website in October 2024. Learn More or Try it out now .
- Advanced Search
- Journal List
- Open Life Sci
- v.17(1); 2022

Agronomic and economic performance of mung bean ( Vigna radiata L.) varieties in response to rates of blended NPS fertilizer in Kindo Koysha district, Southern Ethiopia
Wolaita Zone Agricultural Department, Wolaita Zone Agricultural Office, Wolaita Sodo, Ethiopia
Dereje Shanka
Department of Plant Science, Wolaita Sodo University, P.O. Box 238, Wolaita Sodo, Ethiopia
Mesfin Bibiso
Department of Chemistry, Wolaita Sodo University, P.O. Box 238, Wolaita Sodo, Ethiopia
Mung bean is one of Ethiopia’s most important pulse crops in the lowlands. The main constraints to mung bean productivity in Ethiopia are low soil fertility and improved varieties. During the 2018 cropping season, a field experiment was conducted in Kindo Koysha woreda with the objective of evaluating the effects of NPS fertilizer rates on yield and yield attributing traits of four mung bean varieties. Treatments consisted of factorial combinations of four mung bean varieties (N26, Shewarobit, NVL-1, and Chinese) with four NPS fertilizer rates (0, 50, 100, and 150 kg ha −1 ) laid out in randomized complete block design with three replications. The combination of the N26 variety with 150 kg NPS produced the highest number of pods per plant (15.46), seeds per pod (10.93), grain yield (1240.70 kg ha −1 ), and biomass (3177.40 kg ha −1 ). Moreover, the combination of 100 kg NPS ha −1 with the variety N26 also generated the highest net return (31,734.30 Birr ha −1 ) with a marginal rate of return of 771.71%. Thus, it may be tentatively stated that the usage of 100 kg NPS ha −1 with the variety N26 was determined to be optimum for the development of mung bean in the study region.
1. Introduction
Mung bean ( Vigna radiata L.) is a Fabaceae plant that is one of the most important legume crops. It grows in tropical and subtropical regions around the world [ 1 ]. Mung bean is widely cultivated for human food consumption; it can be used as green manure and livestock feed. On average, mung bean seeds contain 26% protein, 62.5% carbohydrates, 1.4% fiber, vitamins, minerals, calcium, and phosphorus. Because they are easy to digest, they replace the scarce animal protein in the human diet in tropical regions of the world [ 2 ]. The most common characteristics of this crop are its short life cycle and ability to carry out biological nitrogen fixation that satisfies the nitrogen demand of the crop [ 3 ]. Due to its rapid growth and early maturity, this crop can be used to improve planting patterns because it can be planted as a catch and intercrop crop. It can be mainly planted in crop rotation with cereals [ 4 ].
Despite its multiple uses, mung bean is considered a newly introduced crop in Ethiopia. It is grown locally as “Masho” and is grown in few areas of the country; the production is also very small, mainly in the North Shewa and South Wollo Zones of the Amhara region, Southern Nations, Nationalities, and People’s Region (SNNPR) (Gofa, Konso, and Derashe), Tigray Regions (Humara), Oromia Regions (Harrag), and some woredas in Benshangul Gummuz Region [ 5 ]. According to the Central Statistical Agency report [ 5 ], the area covered by Ethiopian mung bean in 2017/18 was 41630.20 ha, the average grain yield was 1,235 kg ha –1 , and the annual output was 51,413,297 kg. The area coverage of the crop in the SNNPRS is estimated to be 224.4 ha, and the average productivity is 931 kg ha –1 , which is far below the potential yield level of 1,500 kg ha –1 [ 6 ].
The reasons for the low productivity of mung beans in Ethiopia include biological and non-biological factors as well as other production restrictions. Among them, fertilizer management is an important factor affecting the growth and yield of mung beans [ 7 ]. However, productivity in the area is still low due to a number of production constraints, including lowland agronomic management practices, adequate nutrient supply, disease, poor ventilation, unbalanced nutrient supply, and a lack of high-yielding varieties. Among them, nutrient deficiency is the biggest factor limiting the yield of beans in Ethiopia. Nitrogen (N), phosphorus (P), and trace elements such as sulfur (S) are important factors that have a greater impact on the growth, development, and yield of mung beans [ 7 ]. In line with this, low production of mung bean has been shown in growing areas of the country as a result of limited nutrient supply [ 8 ]. In order to address these problems, maintaining soil fertility through a balanced fertilization program, including chemical, organic, and biological fertilizers, is quite important in the yield and quality improvement of agricultural crops [ 9 , 10 , 11 ].
Nitrogen is an important mineral whose nutritional management requires special attention due to its diverse roles in plant physiology and metabolites biosynthesis, as well as its dynamics in soil [ 12 , 13 , 14 , 15 ]. Mung beans require more N in the reproductive stage than in the vegetative stage. Moreover, P is the second most important phytonutrient in crop production after N. Phosphorus deficiency is exacerbated under dryland conditions, which affect fertilizer efficiency and successful crop production [ 16 ]. Sulfur promotes the formation of legume nodules and stimulates the production of seeds. With the application of S up to 20 kg ha −1 , the total number of nodules and active nodules increased significantly [ 17 ]. The soil fertility map of the study area showed that levels of N, P, K, S, and Zn, as well as elements such as B and Cu, are depleted, and deficiency symptoms are observed in major crops [ 18 ].
Research done so far to solve the problems in the study area and elsewhere in the country on the response of legumes, including mung beans, to blended NPS fertilizer showed promising results [ 19 , 20 ]. However, farmers in the study area have been using 100 kg of DAP ha −1 (18 kg N and 46 kg P 2 O 5 ha −1 ) for all legumes in a unified package to increase crop yield for approximately 50 years without considering the soil fertility status and crop demand. This emphasizes the importance of developing alternative methods to meet the nutrient needs of plants. In addition to the commonly used N and P fertilizers, mixed NPS contains S. However, the response of mung bean varieties to the amount of mixed NPS fertilizer in the Kindo Koysha district of the Wolaita district in Southern Ethiopia has not been studied. So, this study was done to investigate the effect of the NPS rate on yield and yield attributing traits of mung bean varieties.
2. Materials and methods
2.1. description of the experimental site.
The trial was carried out during the 2017/2018 harvest season at Kindo Koysha woreda (KKW), in the Wolaita Zone of Southern Ethiopia. KKW is geographically located at 6°79′7.06″ N latitude and 37°39′37.63″ E longitude 36 km southwest of the city of Wolaita Sodo to Jima. The altitude range of this area is 1,094 meter above sea level (m.a.s.l.). The study area is a semi-arid climate area with an average annual rainfall of around 400 mm. Rainfall has a bimodal pattern ( Meher and Belg ) of distribution, where the “ Belg ” planting season runs from February to June and the “Meher” season from July to October. The experimental site’s average maximum and minimum temperatures were 32 and 19.2°C, respectively, (unpublished report from the Kindo Koysha District Agricultural Office, 2018).
2.2. Treatments: design of experiments and field layout
Four NPS fertilizer rates (0, 50, 100, and 150 kg NPS ha −1 blended NPS (19% N, 38% P, and 7% S)) and four mung bean varieties were used in the experiments ( Table 1 ). The experiment was laid out using a randomized complete block design (RCBD), and the treatments were repeated three times. The size of each plot was 2.1 m wide and 3 m long (6.3 m 2 area), with a harvestable plot size of 3 m × 0.9 m (2.7 m 2 ). The spacing between the plots and between blocks was 1 and 1.5 m, respectively. The row spacing and plant spacings were 30 and 10 cm, respectively. Each block has 7 rows, each row has 30 plants, and each block has 210 plants. Each row was sown with two seeds at a specified interval, with a depth of about 5 cm, to ensure full emergence. When planting, fertilizer was applied in rows by hand.
Description of mung bean varieties used for the study
Varieties | ||||
---|---|---|---|---|
Characteristics | N26 | Shewarobit | NVL-1 | Chinese |
Altitude (m.a.s.l.) | 900–1,670 | 900–1,670 | 450–1,650 | 450–1,650 |
Rain fall (mm) | 350–550 | 350–550 | 350–750 | 350–750 |
Fertilizer rate (kg ha ) | P O = 46 | P O = 46 | P O = 46 | P O = 46 |
= 18 | = 18 | = 18 | = 18 | |
Maturity days | 65–80 | 75–90 | 60–70 | 75–90 |
Yield on research (kg ha ) | 800–1,500 | 800–1,500 | 750–1,500 | 750–1,500 |
Yield on farmer (kg ha ) | 500–1,000 | 500–1,000 | 500–1,000 | 500–1,000 |
Year of release | 2011 | 2011 | 2014 | |
Breeder | MARC | MARC | MARC |
2.3. Description of experimental materials
The blended NPS (19% N, 38% P, 7% S) used for this experiment was obtained from Woreda Agricultural Office. Mung bean varieties, namely, N26, Shewarobit, NVL-1, and Chinese, were used as planting materials. The description of the varieties used for this study is indicated in Table 1 .
2.4. Agronomic p ractice
The experimental area was ploughed with a tractor-driven disc plough and cross-harrowed with the help of a disc harrow. Sowing was done in rows, with furrows opened by hand plough to a depth of 5 cm at a row spacing of 30 cm and plant to plant spacing of 10 cm. Fertilizers were applied as per treatments in each plot. Plant spacing was maintained by thinning out extra plants 15 days after sowing when all the plants emerged. Weed management is often done manually as needed. In addition, shallow cultivation was performed 25 days after emergence.
Harvesting was done at harvest maturity when the bottom of the mung bean pods started to dry [ 21 ]. The border area was first harvested and then removed from the experimental field. Later on, the net plot area was harvested. Produce from each net plot area was then bundled, tagged, and brought to the threshing floor for sun drying and threshing. After drying, the weight of the bundles (grain + straw) was recorded. Threshing was also done by beating the plants with the help of sticks, plot by plot. The weight of grain and straw was recorded treatment-wise and converted into kg ha −1 .
2.5. Data collection and measurements
2.5.1. soil sampling and analysis.
Prior to planting, 12 representative soil samples were randomly collected in a zigzag pattern from the entire experiment, with a depth of 0–30 cm. The sample was packed in bulk into a composite sample. The soil sample was air dried, crushed with a mortar, and passed through a 2 mm sieve.
The composite samples were analyzed for the soil texture, pH, organic carbon (OC), total N, cation exchange capacity (CEC), available K, P, S, B, and zinc in the Laboratory of Soil and Water Analysis in Debere Zeit, Ethiopia. A standard glass electrode pH meter was used to potentiometrically measure the soil pH value in a 1:2:5 soil water suspension [ 22 ]. The Walkley and Black method [ 23 ] was used to determine the organic matter content of the soil. The Kjeldahl [ 24 ] method was used to determine the total N in the soil. The Olsen extraction method was used to determine soil available P as described by Olsen and Dean [ 25 ]. Available S was analyzed by the turbidimetric method. The Bouyoucas hydrometer method [ 26 ] was used to determine soil texture. Electrical conductivity was measured with a standard glass electrode using an EC meter. The CEC of the soil was determined by the ammonium acetate method [ 27 ].
2.5.2. Crop data
2.5.2.1. yield components and yield.
The number of pods per plant was counted from the pods of the five plants sampled, and the average number was calculated and expressed as the number of pods per plant. Number of seeds per pod was recorded from ten pods of five sampled plants, and the average number was calculated and expressed as the number of seeds per pod. A representative sample of 1,000 grains of mung bean from each plot, sun-dried and moisture content adjusted to 10 %, was weighed in grams using a sensitive balance. Grain yield (kg ha −1 ) was estimated by weighing grains obtained after threshing, cleaning, and sun drying, and finally recorded in kg ha −1 . Aboveground dry biomass yield (kg ha −1 ) was recorded by harvesting the three central rows in each subplot. The material was sun-dried up to a constant weight, weighed, and then converted into kg ha −1 . Harvest index (HI) (%) was calculated as the ratio of economic yield (grain) and aboveground dry biomass yield. Its value was expressed as a percentage using the following formula:
2.6. Economic analysis
The economic analysis was performed using the partial budget analysis procedure described by CIMMYT [ 28 ]. The cost of NPS and labor costs involved in applying NPS fertilizer and using it for this analysis are listed below. The current price of mung bean is calculated based on the average open market price of Kindo Koysha, which was 30 ETB per kg. The net income and other economic analyses are based on the formula developed by CIMMYT [ 28 ] and are given as follows:
The unadjusted grain yield (kg ha −1 ) was the average yield per treatment.
Adjusted grain yield (AGY) (kg ha −1 ) was the average yield adjusted downward by 10% to reflect the difference between the experimental and farmer yields. The total field profit (gross field benefits [GFB]) (ETB ha −1 ) was calculated by multiplying the field/farm price obtained by farmers when they sell their crops by the adjusted yield. GFB = AGY × The field/farm price of the crop.
Total variable cost (TVC) (ETB ha −1 ) was calculated by adding up the costs that vary, including the cost of NPS (14 ETB kg −1 ) fertilizers at the time of planting (August 1, 2018). The costs of other inputs and production practices, such as labor costs for land preparation, planting, weeding, chemical spraying, harvesting, and threshing, were considered the same for all treatments or plots.
Net benefit (NB) (ETB ha −1 ) was calculated by subtracting the TVCs from GFB for each treatment. GFB – TVC = NB
By dividing the change in NB by the change in TVC and multiplying by 100, the marginal rate of return (MRR) (%) was calculated.
2.7. Data analysis
Analysis of variance of the data collected was performed according to the general linear model procedure of SAS version 9.0 [ 29 ] appropriate to factorial experiments in RCBD, and interpretations were made following the procedure described by Gomez and Gomez [ 30 ]. Whenever a treatment effect was found to be significant, the least significant difference (LSD) test at the 5% significance level was used to compare the average.
3.1. Physico-chemical characteristics of the experimental soil
Study site soil’s physical and chemical properties analysis results before planting, such as particle size distribution (texture), pH, OC, total N, available P, available K, available Zn and B, available S, and CEC are shown in Table 2 . The particle size distribution of the experimental soil was found to be 26% sand, 38% silt, and 36% clay ( Table 2 ). According to Rayan and Rashid [ 31 ], the soil at experimental fields was clay loam in textural class.
Physico-chemical properties of the experimental site soil before planting 3
Soil properties | Results | Rating | Reference |
---|---|---|---|
Soil particle size | Sand (%) 26 | ||
Silt (%) 38 | |||
Clay (%) 36 | |||
Textural class | Clay Loam | ||
Soil pH | 6.23 | Moderate | [ ] |
OC (%) | 2.08 | Moderate | [ ] |
Available N (kg ha ) | 0.13 | Moderate | [ ] |
Available P (mg kg ) (ppm) | 9.48 | Low | [ ] |
Available S (mg kg ) (ppm) | 37.76 | Moderate | [ ] |
CEC (meq/100 g soil) | 20.39 |
The soil reaction of the experimental site was 6.23. This indicates suitability of the soil reaction in the experimental site for optimum mung bean growth and yield. Soil analysis before sowing indicated that the soil has medium level of total N, available S, organic matter, and low level of available P. The OC content (2.08 %) and total N (0.13 %) of the soil were medium. The level of available P content of the experimental soil was low (9.48 ppm) ( Table 2 ). According to the rating of Landon [ 32 ] and Hazelton and Murphy [ 33 ], the N and P of the study site were poor requiring application of nitrogenous and phosphoric fertilizers. Overall, the fertility status of the study sites was low ( Table 2 ).
3.2. Yield components and yield
3.2.1. number of pods per plant.
Data analysis shows the interaction between NPS and varieties also significantly affected the number of pods plant −1 . The average of the data shows that the variety N26 (15.46) produced the largest number of pods plant −1 at 150 kg ha −1 , followed by the Chinese pod (14.96) plant at 150 kg ha −1 , while the variety NVL1 provided the lowest number of pods per plant (9.83) with 0 kg NPS ha −1 ( Figure 1 ).

Interaction effects of varieties and NPS fertilizer rate on pod number per plant of mung bean at Kindo Koysha in 2018. LSD (0.05) = Least significant difference at 5% level; CV (%) = Coefficient of variation. Means in the table followed by the same letters are not significantly different at 5% level of significance.
3.2.2. Number of seeds per pod
The interaction between NPS level and mung bean variety had a significant ( P < 0.05) effect on the number of seeds per pod. The highest number of seeds per pod (10.93) was recorded in the 150 kg NPS ha −1 level for variety N26, which was statistically the same at 100 kg ha −1 for the same variety (10.53), followed by all other combined treatments. The lowest number of seeds per pod (8.66) was recorded in the 0 kg ha −1 NPS level for Chinese variety ( Figure 2 ). The change in the NPS level relative to the control (0 kg ha −1 ) indicated that the number of seeds per pod increased with the increase in the NPS level for the N26 variety.

Interaction effects of varieties and NPS fertilizer rate on seed number per pod of mung bean at Kindo Koysha in 2018. LSD (0.05) = Least significant difference at 5% level; CV (%) = Coefficient of variation. Means in the table followed by the same letters are not significantly different at 5% level of significance.
3.2.3. 100-seed weight
The results revealed that thousand seed weight (TSW) was significantly affected by the rate of NPS and variety. The highest TSW (48.81 g) was recorded at a rate of 150 kg ha −1 NPS, which was statistically equivalent to 100 kg ha −1 NPS (48.16 g) and 50 kg ha −1 NPS (46.91 g), while the lowest TSW (43.57 g) was recorded for plots supplied with nil NPS fertilizer ( Table 3 ). Further, the improved variety Chinese had the highest TSW (53.96 g), while the variety Shewarobit had the lowest TSW (40.19 g) ( Table 3 ). This variance could be due to genotypic differences between the types.
The main effect of NPS fertilizer rates and varieties on TSW of mung bean at Kindo Koysha in 2018
Treatment | TSW |
---|---|
) | |
0 | 43.57 |
50 | 46.91 |
100 | 48.16 |
150 | 48.81 |
LSD (0.05) | 1.99 |
N26 | 50.55 |
Shewarobit | 40.19 |
NVL-1 | 42.75 |
Chinese | 53.96 |
LSD (0.05) | 1.99 |
CV(%) | 5.11 |
LSD (0.05) = Least significant difference at 5% level; CV (%) = Coefficient of variation. Means.
In the table followed by the same letters are not significantly different at 5% level.
3.2.4. Grain yield
Grain yield was significantly ( P < 0.05) affected by the interaction effect of the NPS level and the mung bean variety. Compared with the control, with the increase in NPS level, grain yield gradually increased. Variety N26 (1240.7 kg ha −1 ) recorded the highest grain yield at 150 kg NPS ha −1 , followed by the same variety N26 (1230.9 kg ha −1 ) at a NPS blending level of 100 kg ha −1 , while the lowest grain yield (702.4 kg ha −1 ) was from the control for Chinese variety ( Figure 3 ).

Interaction effects of varieties and NPS rates (kg ha −1 ) on grain yield of mung bean at Kindo Koysha in 2018. LSD (0.05) = Least significant difference at 5% level; CV (%) = Coefficient of variation. Means in the table followed by the same letters are not significantly different at 5% level of significance.
3.2.5. Above ground biomass
Results revealed that the interaction between mung bean variety and NPS fertilizer rate had high significant impact ( P ≤ 0.01) on the aboveground biomass yield. Variety N26 with 150 kg ha −1 NPS produced significantly higher aboveground biomass yield (3177.40 kg ha −1 ), which was statistically equivalent to the same variety N26 (3121.50 kg ha −1 ) applied with 100 kg ha −1 NPS, while variety NVL1 applied with 0 kg ha −1 NPS provided the lowest aboveground biomass yield per hectare (2193.4 ha −1 ), which is statistically equivalent to other varieties at the control level ( Figure 4 ).

The interaction effect of varieties and NPS fertilizer rate on aboveground biomass (kg ha −1) of mung bean at Kindo Koysha in 2018. LSD (0.05) = Least significant difference at 5% level; CV (%) = Coefficient of variation. Means in the table followed by the same letters are not significantly different at 5% level of significance.
The results showed that interaction between variety and NPS rate had significant impact on the HI. Accordingly, the highest HI value (39.40%) came from the N26 variety at rate of 150 kg ha −1 NPS, while the lowest HI (32.02%) was for the NVL1 variety at the control plot ( Figure 5 ).

Interaction effects of varieties and NPS fertilizer rate on HI of mung bean at Kindo Koysha in 2018.
3.2.7. Partial budget analysis
Cost-benefit analysis results showed that the maximum NB (31,734.30 Birr ha −1 ) was obtained from variety N26 at an application rate of 100 kg ha −1 NPS with MRR of 771.71% ( Table 4 ). The feasibility of any method can be judged on the basis of additional performance generated by practice that exceeds established practice. Therefore, this is the ultimate deciding factor when choosing a profitable production technology. Furthermore, the use of any crop production technology by farmers to increase crop yield is completely dependent on their economy.
Summary of partial budget analysis of the response of mung bean varieties to the application of NPS fertilizer at Kindo Koysha in 2018
Treatment combination | Unadjusted yield (kg ha ) | Adjusted yield (kg ha ) | Total revenue (Birr ha ) | TVC (Birr ha ) | Net return (Birr ha ) | MRR (%) |
---|---|---|---|---|---|---|
N26 + 0 | 787.7 | 708.93 | 21267.90 | 0 | 21267.90 | |
N26 + 50 | 1004.9 | 904.41 | 27132.30 | 800 | 26332.30 | 633.05 |
N26 + 100 | 1230.9 | 1107.81 | 33234.30 | 1,500 | 31734.30 | 771.71 |
N26 + 150 | 1240.7 | 1116.63 | 33498.90 | 2,200 | 31298.90 | D |
Shewarobit + 0 | 734.6 | 661.14 | 19834.20 | 0 | 19834.20 | D |
Shewarobit + 50 | 921 | 828.9 | 24867.00 | 800 | 24067.00 | 529.1 |
Shewarobit + 100 | 1016.1 | 914.49 | 27434.70 | 1,500 | 25934.70 | 266.81 |
Shewarobit + 150 | 1164.2 | 1047.78 | 31433.40 | 2,200 | 29233.40 | 471.24 |
NVL-1 + 0 | 702.5 | 632.25 | 18967.50 | 0 | 18967.50 | D |
NVL-1 + 50 | 812.3 | 731.07 | 21932.10 | 800 | 21132.10 | 270.58 |
NVL-1 + 100 | 1130.9 | 1017.81 | 30534.30 | 1,500 | 29034.30 | 1,128.89 |
NVL-1 + 150 | 1167.9 | 1051.11 | 31533.30 | 2,200 | 29333.30 | 42.71 |
Chinese + 0 | 761.7 | 685.53 | 20565.90 | 0 | 20565.90 | D |
Chinese + 50 | 901.2 | 811.08 | 24332.40 | 800 | 23532.40 | 370.81 |
Chinese + 100 | 1130.9 | 1017.81 | 30534.30 | 1,500 | 29034.30 | 785.99 |
Chinese + 150 | 1167.9 | 1051.11 | 31533.30 | 2,200 | 29333.30 | 42.71 |
MRR (%) = Marginal rate of return; Fertilizer application cost = 100 Birr ha −1 ; NPS cost = 14.00 Birr kg −1 ; Mung bean grain local selling price = 30 Birr kg −1 ; TVC = Total variable cost; D = Dominated treatment.
4. Discussion
4.1. yield components and yield.
Correcting soil nutrient deficiencies is among the strategies to ensure the higher productivity of legumes in Ethiopia. It is also well documented that N, P, and S are indispensable plant nutrients for plant growth and productivity [ 35 , 36 , 37 ], and their deficiency can severely affect plant productivity. Nevertheless, the pre-plant analysis of the soils of the study area indicated inadequate availability of N, P, and S nutrients ( Table 2 ). In this regard, applying the optimum rate of the newly introduced blended NPS fertilizer was considered an immediate solution to correct the soil nutrient deficiencies in the country in general and in the study area in particular. To this end, the present study also showed that, averaged across varieties, the maximum number of pods per plant, number of seeds per pod, 100-seed weight, aboveground biomass weight, and grain yield were produced at the highest blended NPS (150 kg ha −1 ) rate ( Table 3 , Figures 6 – 9 ). Regardless of the varietal difference, NPS application improved the productivity of mung bean in the study area.

Number of pods per plant averaged across NPS level.

Mean aboveground dry biomass averaged across NPS level.

The mean number of seeds per pod averaged across NPS level.

Mean grain yield (kg ha −1 ) averaged across NPS level.
The results also revealed that mung bean varieties produced a significantly varying number of pods and seeds per plant across different levels of NPS fertilizer ( Figures 1 and and2). 2 ). This indicates a difference in nutrient requirements among the varieties. Previous studies also indicated a significant interactive effect of blended NPS fertilizer level and varieties on the number of pods per plant [ 38 , 39 ] and seeds per pod [ 40 ]. Number of pods per plant showed a 57% increase in pods per plant at 150 kg ha −1 NPS over nil rates of NPS for variety NVL-1, which is a low yielding variety. However, N26 attained the optimum number of pods per plant at 100 kg ha −1 NPS. There is an increasing trend in the number of pods per plant as the NPS level increases from zero to 150 kg ha −1 . This differential response among the varieties might be related to their genotypic differences.
The results also revealed that mung bean varieties also produced significantly different(TSW) ( Table 3 ). A 34% increase in 100-seed weight was recorded for the variety producing the higher 100-seed weight than the variety producing the lower 100-seed weight. This variation in TSW could be due to genotypic differences between the varieties. Supporting the present finding, Oljirra and Temesgen [ 41 ] also reported a significant difference among soybean varieties in 100-seed weight. Further, the findings of this study also indicated that the application of NPS significantly affected the TSW of mung bean. Corroborating the results, different scholars reported that application of N and P fertilizers can significantly influence the seed weight of different legumes, including mung bean [ 42 , 43 , 44 ]. For instance, Yin et al. [ 42 ] reported that N and P fertilizers had extremely significant ( P < 0.01) effects on the 100-seed weight of mung bean.
Mung bean varieties also produced significantly different aboveground biomass yield across NPS level ( Figure 4 ). The higher aboveground biomass yield (3177.40 kg ha −1 ) was produced by variety N26 at 150 kg ha −1 NPS. Averaged across mung bean varieties, the highest aboveground biomass was produced by N26. Nearly 45% increase in aboveground biomass over variety NVL-1 at nil NPS rate was recorded for variety N26 at 150 kg ha −1 NPS. This result is consistent with that of Girma [ 45 ], who found that increasing NP fertilizer rates from 0 to 27 kg ha −1 N, 0 to 69 kg ha −1 P 2 O 5 (150 kg DAP) enhanced common bean biological yield. In line with this finding, Shanka et al. [ 46 ] found that the three-factor interaction impact of site X cultivar X P was significant for aboveground dry biomass production and different dry matters at different P levels on common bean cultivars at varied P levels. The increased biomass yield of cultivars could be attributed to genotypic variations in cultivars across blended NPS rates, or to the fact that increased N availability increased plant height, number of pods per plant, and overall vegetative growth of the plants, all of which contributed to higher aboveground dry biomass yield.
Grain yield is a function of yield attributing traits such as number of pods per plant and seeds per pod, and 100-seed weight. The results indicated that nearly 77% higher grain yield over the low yielding variety NVL-1 at nil rates of NPS was produced by N26 variety at the highest rates of NPS ( Figure 3 ). However, applying NPS in excess of 100 kg ha −1 to variety Shewarobit did not result in a significant yield enhancement. In other words, using more than 100 kg of NPS to increase production of the Shewarobit mung bean variety at Kindo Koysha is not recommended. Further, averaged across mung bean varieties, N26 produced the highest grain yield, while the lowest was produced by Chinese variety. Averaged across the NPS levels also the highest gained yield was produced at 150 kg ha −1 NPS, while the lowest was produced at nil application rates. The findings are consistent with Nebret [ 47 ], who found that greater rates of N above 46 kg ha −1 have negative impacts on grain production of common bean. This could be due to a tendency to increase vegetative growth, which could have resulted in self-shading, lowering overall output.
Significantly different HI was recorded for mung bean varieties supplied with different levels of NPS fertilizer ( Figure 5 ). The reason for the higher value of HI may be due to the greater physiological potential of the crop in partitioning efficiency of dry matter into economic yield and increase in seed yield following application of NPS, accompanied by increased HI. Further, this study is consistent with the study by Kawte et al. [ 20 ], who recorded higher HI, at higher NPS rate for mung bean varieties.
In general, averaged across the NPS level, the highest number of pods per plant, number of seeds per pod, 100-seed weight aboveground biomass, and grain yield were recorded at the highest NPS level (150 kg ha −1 ). The production of a higher number of pods per plant and seeds per pod, 100-seed weight, and grain yield at the highest NPS rates (150 kg ha −1 ) could be due to enhanced photosynthetic activity, leaf area development, and dry matter production [ 36 , 46 ]. As a result, this in turn could have enhanced better photo-assimilate production and translocation into reproductive parts, enhancing the formation of pods, seed setting, seed weight, and economic or grain yield [ 20 , 47 ]. For instance, Seid et al. [ 48 ] noted an increase in the number of pods per plant of chickpea as a result of increased leaf area, with more N being associated with more reproductive nodes.
There are immense research reports regarding the important roles of N, P, and S in photosynthetic processes [ 49 , 36 , 37 ]. In their study, Jiaying et al. [ 36 ] noted higher net photosynthetic rate, ATP content, as well as NADH dehydrogenase, cytochrome oxidase, and ATPase activities, for plants supplied with adequate N and P. Phosphorus is involved in the energy transfer process in photosynthesis and the synthesis of chlorophyll [ 50 , 51 ], whereas S plays an important role in the biosynthesis of chlorophyll and proteins and enzyme activation. This indicates that the improvement in yield and yield related traits could be attributed to the improvement in photosynthetic processes following application of blended NPS fertilizer.
Besides, P is thought to be vital for seed formation and development. It is also a component of phytin, which is a major storage form of P in seeds [ 51 ]. Hence, the increase in the 100-seed weight with the levels of NPS might be attributed to a direct and positive contribution of P to the formation and development of the seed.
From an economical point of view, producers or farmers should maximize their profit from their produce. In other words, they should get an economically acceptable return for their investment. The minimum acceptable MRR should be 100%, according to CIMMYT [ 28 ]. Based on this, the economic analysis of this study also indicated that the higher net incomes with acceptable marginal returns were obtained using the N26 mung bean variety. The most attractive NPS fertilizer application rate is 100 kg ha −1 . In agreement to this study, Shumi et al. [ 52 ] reported that the economic analysis showed that the application of 150 kg ha −1 NPS can obtain the highest NB (34,167.56 Birr ha −1 ), while application of nil NPS resulted in the lowest NB (19,228.69 Birr ha −1 ). Therefore, the amount of fertilization mentioned above is recommended as the best economic return.
5. Conclusion
The results of this study evidenced that mung bean varieties can vary in terms of their blended NPS fertilizer requirement. Hence, the NPS fertilizer management should be designed accordingly. Further, the overall improvement in, yield determining traits such as number of pods per plant, seeds per pod and 100-seed weight resulted in higher grain yield by the best performing variety N26 at the highest blended NPS fertilizer rate (150 kg ha −1 ). Hence, based on the present findings it can be concluded that application of optimum rate of blended NPS fertilizer along with best performing mung bean varieties can boast of high grain yield of mung bean and income to the farmers in the study area. However, based on economic analysis, applying NPS at 100 kg ha −1 resulted in higher NB. Accordingly, growing variety N26 at application rate of 100 kg ha −1 was found to be economically optimum rate for mung bean production in the study area and other similar agroecologies elsewhere in the country or around the globe.
Acknowledgment
The authors would like to thank Wolaita Sodo University for providing financial support to execute this research and Melkasa Research Center for providing the improved seeds of mung bean.
Funding information: The research was supported by Wolaita Sodo University
Conflict of interest: Authors state no conflict of interest.
Data availability statement: The datasets generated during and/or analyzed during the current study are available from the corresponding author on reasonable request.

IMAGES
VIDEO
COMMENTS
PDF | Mung bean (Vigna radiata L. Wilczek) popularly known as green gram, believed to be native crop of India, is a tiny circular shaped bean in green... | Find, read and cite all the research you ...
Mung bean (Vigna radiata L.) is an important pulse consumed all over the world, especially in Asian countries, and has a long history of usage as traditional medicine.It has been known to be an excellent source of protein, dietary fiber, minerals, vitamins, and significant amounts of bioactive compounds, including polyphenols, polysaccharides, and peptides, therefore, becoming a popular ...
The overall nutritional properties of mung beans have been recently reviewed by Dahiya et al. ( 4 ). Due to its high nutritional value, ( 5) especially in seeds, mung bean has served as an important food/feed source for humans and animals. Mung bean seeds contain about 20.97-31.32% protein ( 6 ), compared to 18-22% ( 7) and 20-30% ( 8 ...
PDF | The mungbean is one of the important leguminous crops, holds great importance in agriculture and nutrition in Asian countries. It is a vital... | Find, read and cite all the research you ...
Mung bean can adapt to a wide range of climatic conditions. The best environment is a tropical climate with temperatures ranging from 25 to 35 °C and with moderate precipitation between 400 and 550 mm, during its life cycle of 60-90 days [28]. Mung bean is adaptable to various soil types and grows best on well-drained loamy to sandy loam soil.
The mung bean (Vigna radiata L.) is a leguminous plant widely cultivated in Asia, including Korea, India, and China because of its nutritional value, such as a high protein content of 20-27%, low ...
Wet milling involves cleaning of mung bean followed by soaking in water for 4-12 h. After draining the water, soaked mung beans are mixed with red earth (2-3%) and heaped for overnight for effective moisture diffusion and equilibrium. Alternate sun drying (thin layers) and tempering of mung beans for 2-4 days have Mung Bean
The concentration of -aminobutyric acid in the fermented mung bean increased by 7.6-fold to 0.122 g /100 g, while its content in the non-fermented mung bean was 0.016 g /100 g [52,53]. 3. Polysaccharides. Polysaccharides are proved to play an important role in physiological activities [54].
Plant-based functional foods have gained wider attention in current scenario with mung bean harboring several bioactive compounds with promising gut health benefits and pharmacological importance. Consumption of mung bean has a positive impact on beneficial gut microbes and microbial metabolite production. The effects of dietary mung bean on gut microbial homeostasis and the management of gut ...
advancement in mung bean farming. Astanakulov et al., 2022 [10], addressing the mechanical harvesting challenges in mung bean production, this research aimed at developing and adapting technology and equipment suitable for the efficient harvesting of mung bean. This study highlights the importance of mechanization
mung bean. Thus the protein nutritive value of the legume may be improved by supplementing with these amino acids or by consumption of foods high in these amino acids along with mung bean. Further research is required to identify varieties of these beans with high nutritional quality. Other studies aimed at improving the protein
Mungbean sprouts contain high amounts of thiamine, niacin, and ascorbic acid. Yield potential of mungbean is in the range of 2.5-3.0 t/ha, however, the average productivity of mungbean is staggering low at 0.5 t/ha. The low productivity is due to abiotic and biotic constraints, poor crop management practices and non-availability of quality ...
A limited number of reports have revealed other useful bioactivities for proteins and hydrolysed peptides, including angiotensin-converting enzyme inhibitory activity, anti-fungal activity and trypsin inhibitoryActivity, which have served as effective food additives to prevent proteolysis during storage. To date, no extensive literature review exists regarding potential uses of mung bean ...
Data on physical, chemical, food processing and nutritional properties were collected for whole mung bean grains and reviewed to assess the crop's potential as food and to set research priorities ...
The popularity of plant-based proteins has increased, and mung bean protein (MBP) has gained immense attention due to its high yield, nutritional value, and health benefits. MBP is rich in lysine and has a highly digestible indispensable amino acid score. Dry and wet extractions are used to extract MBP flours and concentrates/isolates, respectively. To enhance the quality of commercial MBP ...
Abstract. Mung bean (Vigna radiataL.) is a pulse crop with multiple uses, yet the crop was not introduced in the study area.Further, there is a lack of information on improved agronomic technologies for the crop in the study area. Hence, a field trial was conducted to evaluate the effects of inter-row spacing and fertilizer rate on the performance of mung bean varieties at Kindo Koysha ...
The seeds and sprouts of mung bean (Vigna radiata), a common food, contain abundant nutrients with biological activities.This review provides insight into the nutritional value of mung beans and its sprouts, discussing chemical constituents that have been isolated in the past few decades, such as flavonoids, phenolic acids, organic acids, amino acids, carbohydrates, and lipids.
Physiology of Mungbean and Development of the Mungbean Crop Model. Y. Chauhan, Col Douglas, +3 authors. A. Skerman. Published 2010. Agricultural and Food Sciences. Stepwise yield improvements in mungbean since the first introduction of varieties such as Emerald and White Gold have transformed the crop into an important summer legume and the ...
Cultivation of Mung bean. Five level of potassium (0,30,60,90 and 120 Kg/ha) were studied for growth and yield response of three cultivar varieties of mung bean (HUM-1, HUM-2 and JM-721) during autumn sea- son 2016. Layout design of mung bean was randomized complete block design (RCBD) with factorial arrangements of 3 replications.
Abstract. This chapter provides an overview of the economic importance of mungbean globally and the status of mungbean improvement research. The global mungbean area is about 7.3 million ha, and the average yield is 721 kg/ha. India and Myanmar each account for 30% of global output of 5.3 million t. Other large producers are China, Indonesia ...
Mung bean (Vigna radiate L.) is a species of Fabaceae plant which is well-known as green gram (Ganesan & Xu 2018).It has been widely grown in the Southeast Asia and is very common in consumer products around the world. Mung beans are rich in protein, starch, cellulose, minerals, and vitamins (Khaket et al. 2015).Studies have shown that mung bean has physiological functions such as anti-obesity ...
Average in vitro iron, zinc and calcium accessibility of the mung bean products were 16, 9 and 418 mg kg−1 dry weight. Phytic acid and polyphenols averaged 2.1 and 1.8 g kg−1 dry weight ...
1. Introduction. Mung bean (Vigna radiata L.) is a Fabaceae plant that is one of the most important legume crops.It grows in tropical and subtropical regions around the world [].Mung bean is widely cultivated for human food consumption; it can be used as green manure and livestock feed.