Thank you for visiting nature.com. You are using a browser version with limited support for CSS. To obtain the best experience, we recommend you use a more up to date browser (or turn off compatibility mode in Internet Explorer). In the meantime, to ensure continued support, we are displaying the site without styles and JavaScript.
- View all journals
- My Account Login
- Explore content
- About the journal
- Publish with us
- Sign up for alerts
- Perspective
- Open access
- Published: 01 August 2024

Plastic recycling: A panacea or environmental pollution problem
- Nisha Singh 1 , 2 &
- Tony R. Walker 2
npj Materials Sustainability volume 2 , Article number: 17 ( 2024 ) Cite this article
12k Accesses
6 Citations
14 Altmetric
Metrics details
- Environmental sciences
- Materials science
Increasing plastic waste is a critical global challenge to ecological and human health requiring focused solutions to reduce omnipresent plastic pollution in the environment. While recycling has been touted as one solution to counter plastic waste and resource utilization, it has been largely ineffective in offsetting the impact of rising global plastic production of more than 400 million metric tonnes annually, due to low global recycling rates of only 9%. Over three decades since implementing plastic resin codes, recycling has favoured thermoplastics, neglecting thermoset plastics. There is a constant need to enhance overall recycling efficiency by exploring advanced methods, as enormous gaps exist in fully unlocking the potential of plastic recycling. We identify critical gaps associated with plastic waste recycling and its potential environmental impacts. We discuss substantial progress in recycling technology, designs-for-recyclability with controlled chemical use, and economic incentives to expand markets for recycled plastics and to curb plastic leakage into the environment. Additionally, we highlight some emerging strategies and legally binding international policy instruments, such as the Global Plastics Treaty that require further development to reduce plastic waste and improve plastic recyclability.
Similar content being viewed by others
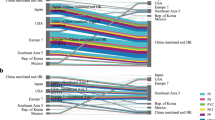
China’s plastic import ban increases prospects of environmental impact mitigation of plastic waste trade flow worldwide
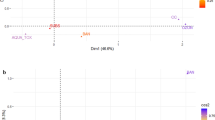
Insights from international environmental legislation and protocols for the global plastic treaty
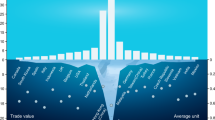
Economic viability requires higher recycling rates for imported plastic waste than expected
Introduction.
The versatile properties of plastics, in contrast to traditional materials such as paper, glass, and metals, facilitate innumerable applications across various sectors, including automobiles, agriculture, electronics, packaging, and healthcare 1 , 2 . For example, the incorporation of plastic in various vehicle components reduces weight and enhances performance in automobile industries. Our growing reliance on the convenience of consumer plastics has resulted in increased global production and consumption leading to unprecedented plastic waste generation and widespread plastic pollution. However, our infatuation with plastics is weakening due to its associated risks to environmental and human health 3 , 4 .
Globally, more than 9200 million metric tonnes (Mt) of plastic have been produced to date. Of this, a significant 6900 Mt has not undergone any type of recycling, resulting instead in accumulation in landfills or dispersal within the environment. This represents a missed economic opportunity and a substantial detriment to the environmental health 5 . To sustain the viability of this multi-billion-dollar material, it is crucial to address the complexity of plastic waste and take transformative steps to redesign plastic products focusing on sustainability and end-of-life (EoL). Among the recently available options to manage plastic waste are – (1) landfilling (waste-to-landfill), with its finite capacity, risks leaching toxic chemicals into the surrounding environment, (2) waste-to-energy through incineration with the potential to release hazardous chemicals and gases (e.g., dioxins and furans), and (3) recycling plastic waste into new products 6 , 7 (Fig. 1 ). Plastic waste in landfills is a reflection of unrealized economic potential and harm inflicted upon the environment. While energy recovery from plastics offers convenience without the labour-intensive sorting required for recycling, it limits material recovery to low energy conversion and intensifies atmospheric pollution and global warming. However, emerging carbon capture technologies in exhaust gases may be used so that CO 2 emissions can be minimized 6 . Conversely, recycling presents an opportunity to address the challenge of increasing global plastic waste.
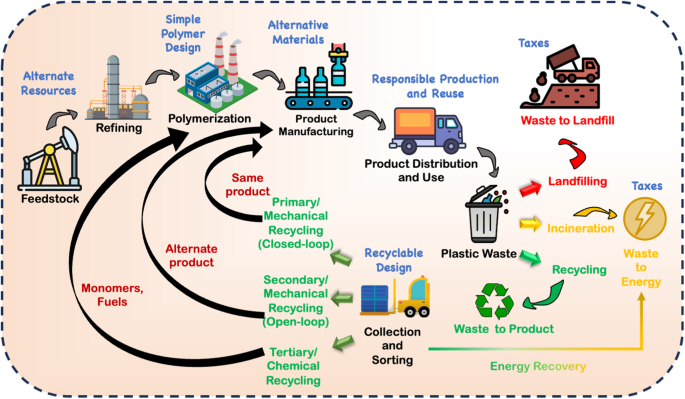
Artwork for this figure is original and created by the authors.
Plastic recycling encompasses the entire process from waste collection to reprocessing into valuable form 8 (Fig. 1 ). Plastics can undergo mechanical or chemical recycling to maintain their original chemical structure, or deliberately alter the chemical composition of the material, respectively 9 , 10 . Currently, mechanical recycling dominates plastic waste management 11 , 12 , with polyethylene or polythene (PE) and polyethylene terephthalate (PET) being the most commonly recycled 8 , 13 and valuable post-consumer plastics globally. Plastic recycling is performed using different approaches including primary, secondary, tertiary, and quaternary recycling 6 , 8 . A small fraction of mechanically recycled plastics undergo closed-loop material recycling to generate identical products as the original plastic and contribute to primary recycling. As a result, closed-loop recycling relies on high-quality waste inputs 14 , with pre-consumer manufacturing waste forming a crucial component 6 . Additionally, open-loop recycling creates products serving different purposes than the original material that enters into alternative markets 11 . The process of open-loop mechanical recycling can potentially lead to secondary recycling opportunities. Conversely, tertiary, or chemical cycling practices advance methods to depolymerize and recover monomers, and hydrocarbon products through pyrolysis, and gasification 15 . Chemical recycling, while efficient for mixed plastic waste, is quite limited due to high energy requirements and intense reaction conditions. Besides, a major burden of chemical recycling technologies such as gasification or pyrolysis is the need to clean the downstream output— to protect the equipment and keep the product valuable 16 . Further, the quaternary approach involves energy recovery by incineration, especially from mixed plastic waste instead of diverting it to landfills 6 .
Theoretically, most polymers are recyclable and some even have desirable cradle-to-cradle lifecycles, offering opportunities for a circular plastic economy 12 , 17 . Here, we discuss some major challenges of recycling such as the complexity of plastic products themselves, market forces that make fossil-fuel-derived virgin plastics cheaper than recycled plastic feedstock, the negative environmental, and social impacts, and inconsistent global policies, including the Global Plastics Treaty 18 , that influence international efforts for effective closed-loop plastic recycling 19 . Additionally, we call for prioritizing reduction in plastic production, consumption, and exploring alternative sustainable materials to tackle rising plastic waste 20 , 21 .
Challenges of plastic recycling
Acknowledging the presence of EoL plastics is crucial in addressing the intricacies of plastic recycling. While recycling is widely touted as a promising pathway to achieving a plastic waste-free future, there remain substantial barriers to making this a reality. For example, current global recycling rates, at only 9%, are simply ineffective in the face of increased plastic production. Over 400 Mt of plastics is produced annually 2 , primarily as single-use items, accounting for more than 50% of consumer-based plastics, which are difficult-to-recycle 22 , 23 . The intrinsic polymer and product design flows of plastic impede their EoL recyclability. Despite the recyclability of most consumed thermoplastics, only a small fraction of them find their way into the recycling stream. Besides, several plastics are incompatible during recycling resulting in a phase-separate mixture adding to the recycling cost and reducing profitability 6 . Meanwhile, the phase separation of the mismatching plastic waste stream can be controlled by polymer compatibilizers such as block copolymers, and graft copolymers 24 . The introduction of compatibilizers stabilizes the immiscible mixture and allows their interaction to produce advanced material 12 , 13 .
Contemporary recycling techniques predominantly address thermoplastics, omitting a substantial fraction of plastic types lacking circular design. Thermoset plastics exemplify this issue, where their valued rigidity from covalent cross-linking also confers significant recycling resistance 12 . While it is possible to grind into fine powders for certain downgrade applications, recycling thermosets, which currently constitute one-third of the total plastic manufactured, requires a distinct approach compared to thermoplastics 25 . Similarly, elastomers primarily composed of tires, represent one of the rapidly expanding industries, and encounter an uncertain fate 24 , 26 . Additionally, composite plastics, integrating polymers with fibrous substances such as fiberglass or carbon fibre, are increasingly used across various industries but present substantial separation hurdles. These challenges underscore the imperative for research into the design of easy-to-recycle plastic materials 17 , 27 .
The complexity and diversity of plastic compositions, exacerbated by chemical additives blended for versatility, lead to a low recycling rate due to the difficulty in recycling different grades together without degrading properties 11 . For instance, reprocessing different colours of 100% recyclable PET 28 together can lead to lower-quality recyclate 19 , 24 . High-value transparent plastics are preferred and hold higher market value, while pigmented ones may be discarded. Therefore, recycling necessitates extensive sorting facilities to maintain the quality of the end product. A notable challenge to sorting lies in the complex composition of most plastic waste generated today, compounded by contamination with labels, coatings, and food remains 8 , 29 . The immiscible plastic waste, combined with diverse materials, questions the efficacy of current recycling techniques, which are more inclined to pure waste polymers requiring efficient waste collection and extensive sorting 24 . Although sorting waste at the source has generally improved, the sorted waste is often underutilized or repurposed ineffectively 30 . If the waste stream is too contaminated, it is not recycled and diverted to landfills or incinerators 19 . Moreover, recycled plastics typically endure only a few recycling cycles 3 , with approximately 10%—undergoing multiple rounds 31 , and are often mixed with virgin materials to maintain the desired properties 24 .
About 90% of plastics production relies on oil and gas feedstocks 23 , and in 2019, this accounted for 6% of the world’s oil production used as raw material 32 , 33 . The surge in fossil fuel availability for plastic production, driven by global decarbonization efforts in the energy and transport sector, exacerbates the issue. Recent developments in creating alternative materials like bio-PET and bio-PE aim to promote reduction of fossil resource use and to reduce life-cycle CO 2 emissions. Incorporating these bioplastics, identical to their fossil fuel versions, into existing recycling methods, however, remains crucial to their positive impact and avoid waste problems and plastic pollution at EoL 34 . The readily available and inexpensive fossil fuels present a significant disincentive to building waste collection infrastructure, particularly in low-income countries where funding and planning are already insufficient. This poses substantial challenges to enhancing recycling efforts and developing a more robust waste management system 4 , 35 .
Consequently, the low recycling rate leads to a disparity between the demand and supply of recycled plastic resins 36 . Additionally, market values of reprocessed resins are compromised by their reduced structural integrity. Advanced techniques, such as solid-state polymerization, offer solutions by enhancing polymer chain reassembly and strength by heating the polymer without reaching melting points. Often contaminated plastic waste from industries or agriculture chemical packaging limits the application of recycled products 1 . The ambition to incorporate more recycled plastics into products confronts the reality of the shortage of high-quality and volume plastic waste and reprocessed resins 37 . Regardless embracing plastic recycling, has the potential to generate substantial profits of up to USD$60 billion by 2030, within the petrochemicals and plastics sector 37 . However, utilizing recyclates as direct replacements for virgin plastics is crucial to undercut the production of the latter and to prevent the proliferation of low-end, disposable goods. A strategic shift in the market towards high-quality recyclable materials is essential for bridging the existing gap in the recycling ecosystem and for the realization of the sector’s financial potential.
Among other challenges to the unique composition of every plastic and availability of cheap virgin plastics, the lack of consistency and standardization in waste handling approaches are major obstacles across the globe. The Resin Identification Code (RIC), is defined for polymers under the 1-6 category, while category 7 includes all others 7 , with no dedicated class for nonrecyclable, biodegradable polymers such as polylactic acid, and elastomers including rubbers. Since the inception of RIC in 1988, the progress in polymer science has added several plastics into the market, emphasizing the need for a comprehensive tagging system including factors like colour for better material recycling. Similarly, certifications and permits associated with labelling should be updated to reflect modern scientific understanding and findings. Additionally, eco-labels, such as those indicating biodegradability, plastic-free, or eco-friendly, issued by third-party certifiers assist the plastic recycling ecosystem. For example, the label (green dot) introduced under the producer responsibility for plastic packaging products in Germany boosted the recovery of recyclable plastics 6 . In contrast, the positive impact can remain unrealized when the product features generic and self-declared misleading claims to greenwash and confuse consumer decision-making 38 . For example, “100% Recyclable” (Coca-Cola and Nestle) 39 , “Degradable” (Coco Thumb), and “Microplastics Free” (Wital tea) without scientific merit to attract green purchases amplify the gravity of the situation.
Environmental impacts of recycling
The use of plastic is anticipated to triple by 2060 compared to 2019, driven by the expanding global economy; however, the recycling rate may double during this period, creating a significant unintended environmental leakage 2 , 40 . Until now, the environment has been housing multiple layers of first-generation nonbiodegradable plastics that have transgressed different compartments 4 , which may unfold as a catastrophic environmental challenge. It is estimated that 19–23 Mt of plastic waste generated globally in 2016 entered aquatic ecosystems, but could reach up to 53 Mt annually by 2030 3 . Legacy plastic pollution is not just limited to marine and aquatic ecosystems. Due to the widespread use of plastics in agriculture and their limited recyclability, an estimated 12.5 Mt of plastics accumulate in agricultural soils annually 1 , 41 . Additionally, recycling alone cannot reverse the damage incurred due to the leakage of plastics already in the environment 21 , 35 .
Plastic recycling encompasses both positive and negative aspects, warranting a comprehensive evaluation to balance environmental benefits and burdens. Recycling plastic waste significantly reduces fossil fuel utilization, power consumption, and landfilling 30 , 42 . The ripple effect is a decline in the emission of greenhouse gases, thus lowering the carbon footprints while contributing to the global economy and direct jobs. In fact, it is emphasized that reprocessing 1 ton of plastic can save up to approximately 130 million kilojoules of energy 24 . A life cycle assessment (LCA) conducted on the environmental impact of 1) recycling plastic waste compared to alternative approaches and 2) application of secondary products instead of virgin materials marks a positive step toward climate control 30 . Similarly, several other LCA studies have confirmed the superiority of plastics as material over their alternative option such as aluminium bottles, paper, and cotton bags 43 , 44 . However, a notable limitation in several standard LCA methodologies lies in omitting a crucial factor—the long-term fate of chemicals and particulates released during EoL plastic 1 , 45 . The disadvantage of existing short-term LCAs in disregarding the consequences of chemical and particulate releases raises concerns about the overall efficacy of plastics and recycling as a solution to plastic pollution. This gap in evaluating the true ecological footprint of virgin and recyclate plastics (i.e., raw materials transported to a waste recycling facility for processing into a new materials or products) may result in unintended environmental and health costs.
Recycling facilities have been identified as potential hotspots and contributors of toxic and hazardous waste, however, there is limited attention to chemical or particle release from plastic recycling facilities. Despite the current and emerging technologies to recycle plastic waste, non-recoverable tiny plastic particles (microplastics) cannot be addressed with existing collection methods due to their exceptionally small size. Further, the size reduction and washing during mechanical recycling facilities tend to release significant microplastics into the environment 46 . About 13% of plastics infiltrate water or air as microplastics from recycling facilities in the UK 47 . A study on PET recycling facilities reveals microplastic releases range from approximately 23–1836 mg/L in wastewater that is distributed in the effluent (8–83 mg/L) and the sludge (52,166–68,866 mg/L) as it leaves the facility 48 . Microplastics generated during the recycling process are governed by the properties of plastics (polymer type or hardness) and environmental exposure 46 . Ideally, plastic recycling facilities are equipped with filters to prevent and mitigate environmental contamination, but it partially mitigates microplastic release and is not a comprehensive solution 47 . Additionally, the leaching of harmful plastic chemicals during and after recycling also poses a significant threat 29 . Recycled plastics exhibit higher levels of hazardous chemicals such as brominated flame retardants as legacy contaminants. The contamination not only hinders the wide application, it also poses health risks for workers and end-users 12 . With this, it is imperative to produce toxic chemical-free material through controls over what is being recycled and standards for recycled plastics and their usability in different sectors.
While chemical recycling can produce food-grade plastics and has been heralded to fix plastics recycling, it is financially risky and can have far-reaching environmental implications compared to virgin plastics production 8 , 21 . The damage to the environment through chemical recycling in terms of emissions, energy consumption, and water utilization surpasses those used in other technologies 49 . Meanwhile, mechanical recycling is believed to exhibit a lower overall impact on climate change than chemical recycling and energy recovery, which contributes to greenhouse gas emissions and photochemical ozone formation 42 . To address these concerns effectively, the transport and sorting of waste should be confined within closed spaces, filters should be installed and wastewater should be treated to prohibit the release of plastics and associated chemicals into the environment 36 , 50 . Despite an apparent increase in the plastic recycling rate, lower-grade polymers with a limited lifespan are eventually disposed of as waste, thus challenging the circular economy of plastics and environmental sustainability.
Inefficient waste collection, coupled with the necessity for sorting before recycling, requires transportation to dedicated waste handling facilities leading to inadvertent loss and an escalation in carbon footprints. However, the global plastic waste trade is built on the premise of exporting for recycling, often to lower-income countries 51 . Countries are also fraught with widespread environmental impacts and incredibly low recycling rates if accurately reported 52 . Further, regional policies have far-reaching effects on global plastics recycling dynamics. Until 2018, China had been the reprocessing house for more than 50% of PET bottles 53 , but the recent ban on foreign waste imports, including plastics, has left world recycling facilities scrambling 54 . High-income countries began exporting plastic waste to other low-income countries, particularly those in the global south 51 , 55 . Many of these low-income countries have become disproportionally impacted by plastic pollution due to overwhelming imports of plastic waste (for so-called “recycling”), as part of the global plastic waste trade 52 . These countries lack adequate recycling facilities, which has led to excessive open-dumping or burning of plastic waste, including waste-to-energy incineration 35 , 51 . Imported plastic, often of low quality, contaminated, or mislabelled, is diverted to landfilling and incineration, each contributing to negative environmental impacts. The other example of change in plastic waste dynamics includes the largest exporter of plastics (i.e., Japan), which saw a surge in reprocessing, while the use of virgin plastics increased in China which further increased the carbon footprint following the import ban 56 .
Achieving plastic circularity and plastic recycling in the Global Plastics Treaty
Currently, we are in the midst of a global plastic pollution problem driven by unsustainable plastic production and plastic consumption 20 . The plastics industry narrative has previously been framed around the unique recyclability of many plastic polymers, but the reality is that plastics have been grossly mismanaged 3 , 57 . While recycling plays a role in managing plastic waste, doubts linger if it is a holistic solution 21 . The combination of poor polymer and product design, the nature of mixed waste generated, inadequate and wide variations of waste management infrastructure, poor quality of post-recycling products, demand-supply gaps, and environmental, economic, and social impacts have resulted in unsustainable plastic waste generation 7 , 19 . With technological limitations and substandard industrial compliance, plastic recycling is not working. Globally, the recycling rates for plastic are paling in comparison to paper and metals, with a high recycling rate of aluminium at 76% 58 . Even if plastics are recycled, the environmental impacts are startling, particularly with chemical recycling 42 .
Addressing the challenge of reducing global plastic production is complex, particularly given the disparity in plastic consumption between developing and developed economies. With almost 4 billion people residing in developing countries utilizing considerably less plastic than their counterparts in developed nations, there exists a growing trend towards increased production and usage in these regions. Further, the global trade in plastic waste often involves shipping to countries with lower processing costs. The extended producer responsibility (EPR) schemes have the potential to internalize the environmental costs of production and waste management, providing incentives to reduce the use of virgin plastics and improve the quality of recyclables 59 .
The transformative shift to global plastic sustainability demands a 50% reduction in future plastic demand, coupled with phasing out of fossil-derived plastics, a remarkable 95% recycling rate for retrievable plastics, and a transition to renewable energy sources to establish a sustainable circular plastics economy 60 . Although current technology for plastic recycling is yet not circular, robust steps in tandem with changing regulations and research efforts are needed to encourage a decline in the impact of plastics. The time lag to achieve a complete closed-loop recycling for all plastic produced accentuates the need to cap production and explore design-for-recyclability, extending beyond mere reducing and reusing these materials. Bridging the gap between escalating plastic production and effective recycling demands substantial immediate investment in research and infrastructure to maintain the plastic waste within the value chain without resorting to down-cycling or disposal.
Achieving sustainability and a circular economy requires recognizing the importance of methods beyond recycling, including product design, alternative materials, phasing out problematic plastics, curbing the consumption of virgin plastic materials, and adopting reduction and reuse strategies 23 (Fig. 1 ). The paradigm shift necessitates a decoupling from fossil fuel reliance and embracing recycled and biobased feedstock, towards CO 2 emission neutrality. Importantly, the focus extends to EoL considerations, where plastics should either be efficiently collected and economically recycled or designed to be completely biodegradable if dispersion is unavoidable 61 , 62 . Crucially, future polymer designs should not only meet traditional performance and cost but also incorporate safe and sustainable-by-design principles. A simplified plastic with a design-for-recyclability along with controlled chemicals, labels, and adhesive in finished products has the potential to encourage recycling rate 11 , 50 . Embracing a mono-material approach in product design, where single polymers are utilized without compromising performance, and innovative solutions such as debonding-on-demand techniques offer pathways to address the challenges posed by multilayer plastics products 61 . Additionally, establishing standards and global policies is crucial to capping plastic production and curbing the continuous flow of plastic waste into the environment 63 .
The reaction to the looming global threat of irreversible plastic pollution is through decreasing plastic emissions 64 . Life cycle analyses indicate net-zero emission plastics are achievable using current technology, through a synergistic approach that integrates biomass, CO 2 utilization, and attains a 70% effective recycling rate, which significantly reduces energy use and operational costs 65 . Further, addressing the global plastic waste crisis requires the implementation of internationally coordinated waste management strategies 64 . Countries are implementing economic instruments to stimulate plastic recycling via different methods under the polluter-pays principle including EPR 66 , deposit-refund schemes (DRS), tax on virgin plastics, landfill and incineration taxes, and pay-as-you-throw schemes 67 , 68 . For instance, DRS, a lucrative refund incentive once applied to glass bottles, successfully promotes collection and reduces plastic littering. DRS accumulates less contaminated plastics over the traditional single-stream recycling process. The scheme has incentivized as high as 95% of plastic bottle recycling in Norway whereas Ecuador reported an 80% collection of PET bottles in 2012 as compared to 30% in 2011 69 . Similarly, in 2019 plastic collection under DRS has increased in different countries including Denmark (94%), Croatia (89%), Estonia (87%), and Finland (90%) 69 .
The challenge of EoL plastic has been recognized by the international community with 175 United Nations member countries agreeing to eliminate plastic pollution with a legally binding plastic treaty instrument 70 . The international community with the ongoing Plastics Treaty negotiations have already established a zero draft document and an updated revised zero draft document, which includes elements to address inadequacies of current plastic recycling 50 . Those include primary plastic polymers, chemicals, and polymers of concern when recycling complex mixtures of plastic waste 71 . Additionally, problematic and avoidable single-use plastic products will be included in the Global Plastics Treaty as these are invariably difficult or impossible to recycle and should be phased out or replaced with sustainable alternatives 72 , 73 , 74 . Sustainable product design, performance, and practices such as reduction, reuse, refill, and repair will be emphasized.
Another important element of the Global Plastics Treaty includes the use of increased recycled plastic contents amidst the challenge of rising global plastic production, largely from virgin plastics 21 . To increase recycled plastic contents as part of the Global Plastics Treaty, governments could implement economic policy instruments to incentivise the price of recycled plastics compared to virgin plastics. For example, industries utilizing recycled plastics could be offered lower corporate taxes, whereas industries using virgin plastics would incur penalties (higher corporate taxes). The transition to a circular economy needs to reduce resource consumption and plastic pollution by moving away from the current linear economic model of plastic production 63 . Only focus on improved recycling and improvements in waste management facilities will promote increased production of waste as it will not cap production and will effectively lock-in the global community to business as usual.
Finally, the Global Plastics Treaty will also include elements of EPR, emissions and releases of plastic through its entire life cycle, transformational improvements to waste management, as well as a just transition for waste pickers who play a major role in driving the informal recycling sector in many jurisdictions. Overall, it will offer opportunities to improve plastic recycling and eliminate harmful chemicals used in plastic production, manufacture, and packaging.
Concluding remarks
An immense variety of plastic products comprising a complex mixture are used in every aspect of modern society. However, the sustainability of these invaluable materials has largely been ignored. A staggering 91% of plastic meets an alternate fate than recycling. To improve the sustainability of plastic recycling we need a coordinated global panacea of solutions, as there is no one silver bullet to solve the pervasive plastic pollution problem. Emerging recycling technologies will help contribute to the panacea of solutions, but without global coordination, such as the Global Plastics Treaty, they alone will not address the plastic pollution crisis until it is controlled at the source with plastic production caps. Under the Global Plastics Treaty, United Nations member countries could consider adjusting the international price of virgin plastics to reflect the true environmental and economic costs of plastic pollution on ecological and human health. Reducing global virgin plastic production and overall consumption will help the implementation of an effective Global Plastics Treaty that will comprise comprehensive elements to reduce plastic pollution and increase plastic recycling to achieve a circular economy.
Data availability
No datasets were generated or analysed during the current study.
Hofmann, T. et al. Plastics can be used more sustainably in agriculture. Commun. Earth Environ. 4 , 332 (2023).
Article Google Scholar
OECD. Plastics use projections to 2060. Global Plastics Outlook https://doi.org/10.1787/65863783-EN (2022).
Borrelle, S. B. et al. Predicted growth in plastic waste exceeds efforts to mitigate plastic pollution. Science 369 , 1515–1518 (2020).
Article CAS PubMed Google Scholar
Lebreton, L. & Andrady, A. Future scenarios of global plastic waste generation and disposal. Palgrave Commun. 5 , 1–11 (2019).
Geyer, R. Production, use, and fate of synthetic polymers. Plast. Waste Recycl. 13–32 https://doi.org/10.1016/B978-0-12-817880-5.00002-5 (2020).
Hopewell, J., Dvorak, R. & Kosior, E. Plastics recycling: challenges and opportunities. Philos. Trans. Royal Soc. B: Biol. Sci. 364 , 2115 (2009).
Article CAS Google Scholar
Vanapalli, K. R., Samal, B., Dubey, B. K. & Bhattacharya, J. Emissions and environmental burdens associated with plastic solid waste management. Plast. Energy: Fuel Chem. Sustain. Implicat. 313–342 https://doi.org/10.1016/B978-0-12-813140-4.00012-1 (2019).
Hou, Q. et al. Upcycling and catalytic degradation of plastic wastes. Cell Rep. Phys. Sci. 2 , 100514 (2021).
Ragaert, K. et al. Clarifying European terminology in plastics recycling. Curr. Opin. Green Sustain. Chem. 44 , 100871 (2023).
ISO 15270:2008 - Plastics — Guidelines for the recovery and recycling of plastics waste. https://www.iso.org/standard/45089.html .
Ragaert, K., Delva, L. & Van Geem, K. Mechanical and chemical recycling of solid plastic waste. Waste Manag. 69 , 24–58 (2017).
Ignatyev, I. A., Thielemans, W. & Vander Beke, B. Recycling of polymers: a review. ChemSusChem 7 , 1579–1593 (2014).
Schyns, Z. O. G. & Shaver M. P. Mechanical recycling of packaging plastics: a review. Macromol. Rapid Commun. 42 , 2000415 (2021).
Rahimi, A. R. & Garciá, J. M. Chemical recycling of waste plastics for new materials production. Nat. Rev. Chem. 1 , 1–11 (2017).
Vollmer, I. et al. Beyond mechanical recycling: giving new life to plastic waste. Angew. Chem. Int. Ed. 59 , 15402–15423 (2020).
Vasudeo, R. A., Abitha, V. K., Vinayak, K., Jayaja, P. & Gaikwad, S. Sustainable development through feedstock recycling of plastic wastes. Macromol. Symp. 362 , 39–51 (2016).
Vyncke, G., Onnekink, J., Feenstra, T. & Ragaert, K. Design from Recycling for post-consumer WEEE plastics. International Conference on Polymers and Moulds Innovations-PMI 2018 . (Institute of Polymers and Composites, University of Minho, Portugal, 2018).
Ammendolia, J. & Walker, T. R. Global plastics treaty must be strict and binding. Nature 611 , 236 (2022).
Syberg, K. Beware the false hope of recycling. Nature 611 , S6 (2022).
Walker, T. R. & Fequet, L. Current trends of unsustainable plastic production and micro(nano)plastic pollution. TrAC Trends Anal. Chem. 160 , 116984 (2023).
Bergmann, M. et al. A global plastic treaty must cap production. Science 376 , 469–470 (2022).
Article PubMed Google Scholar
Walker, T. R. & Xanthos, D. A call for Canada to move toward zero plastic waste by reducing and recycling single-use plastics. Resour. Conserv. Recycl. 133 , 99–100 (2018).
World Economic Forum, Ellen MacArthur Foundation & McKinsey & Company. The new plastics economy rethinking the future of plastics. World Economic Forum. 36 p (2016).
Garcia, J. M. & Robertson, M. L. The future of plastics recycling. Science 358 , 870–872 (2017).
Utekar, S., V. K., S., More, N. & Rao, A. Comprehensive study of recycling of thermosetting polymer composites – Driving force, challenges and methods. Compos. B Eng. 207 , 108596 (2021).
Fazli, A. & Rodrigue, D. Waste Rubber Recycling: A Review on the Evolution and Properties of Thermoplastic Elastomers. Materials 13 , 782 (2020).
Article CAS PubMed PubMed Central Google Scholar
Yang, Y. et al. Recycling of composite materials. Chemical Engineering and Processing: Process Intensification 51 , 53–68 (2012).
Cestari, S. P., Albitres, G. A. V., Pires, H. M., de França da Silva Freitas, D. & Mendes, L. C. Study of the interaction between oligomerised recycled Poly(ethylene terephtalate) and concrete waste. J. Polym. Environ. 27 , 2915–2924 (2019).
Hahladakis, J. N., Velis, C. A., Weber, R., Iacovidou, E. & Purnell, P. An overview of chemical additives present in plastics: Migration, release, fate and environmental impact during their use, disposal and recycling. J. Hazard. Mater. 344 , 179–199 (2018).
Tonini, D., Garcia-Gutierrez, P. & Nessi, S. Environmental effects of plastic waste recycling. Publications Office of the European Union, Luxembourg https://doi.org/10.2760/6309 (2021).
Geyer, R., Jambeck, J. R. & Law, K. L. Production, use, and fate of all plastics ever made. Sci Adv 3 , (2017).
Walker, T. R. & McGuinty, E. Plastics. The Palgrave Handbook of Global Sustainability 1–12 https://doi.org/10.1007/978-3-030-38948-2_55-1 (2021).
Siddique, R., Khatib, J. & Kaur, I. Use of recycled plastic in concrete: A review. Waste Manage. 28 , 1835–1852 (2008).
Lamberti, F. M., Román-Ramírez, L. A. & Wood, J. Recycling of bioplastics: routes and benefits. J. Polym. Environ. 28 , 2551–2571 (2020).
Jambeck, J. R. et al. Plastic waste inputs from land into the ocean. Science 347 , 768–771 (2015).
Santos, G., Esmizadeh, E. & Riahinezhad, M. Recycling construction, renovation, and demolition plastic waste: review of the status quo, challenges and opportunities. J. Polym. Environ. 1–31 https://doi.org/10.1007/S10924-023-02982-Z/TABLES/10 (2023).
Hundertmark, T., Mayer, M., Mcnally, C., Simons, T. J. & Witte, C. How plastics waste recycling could transform the chemical industry. McKinsey & Company. 11 p (2018).
Józwik-Pruska, J., Bobowicz, P., Hernández, C. & Szalczyńska, M. Consumer awareness of the eco-labeling of packaging. Fibres Textiles East. Eur. 30 , 39–46 (2022).
Coca-Cola and Nestle accused of misleading eco claims - BBC News. https://www.bbc.com/news/business-67343893
OECD. Projections of plastic leakage to the environment to 2060. Global Plastics Outlook https://doi.org/10.1787/6D147318-EN (2022).
Singh, N., Abdullah, M. M., Ma, X. & Sharma, V. K. Microplastics and nanoplastics in the soil-plant nexus: Sources, uptake, and toxicity. Crit. Rev. Environ. Sci. Technol. 53 , 1613–1642 (2023).
Jeswani, H. et al. Life cycle environmental impacts of chemical recycling via pyrolysis of mixed plastic waste in comparison with mechanical recycling and energy recovery. Sci. Total Environ. 769 , 144483 (2021).
Ahamed, A. et al. Life cycle assessment of plastic grocery bags and their alternatives in cities with confined waste management structure: A Singapore case study. J. Clean. Prod. 278 , 123956 (2021).
Tamburini, E. et al. Plastic (PET) vs bioplastic (PLA) or refillable aluminium bottles – What is the most sustainable choice for drinking water? A life-cycle (LCA) analysis. Environ. Res. 196 , 110974 (2021).
Gontard, N., David, G., Guilbert, A. & Sohn, J. Recognizing the long-term impacts of plastic particles for preventing distortion in decision-making. Nat. Sustain. 5 , 472–478 (2022).
Stapleton, M. J., Ansari, A. J., Ahmed, A. & Hai, F. I. Evaluating the generation of microplastics from an unlikely source: The unintentional consequence of the current plastic recycling process. Sci. Total Environ. 902 , 166090 (2023).
Brown, E., MacDonald, A., Allen, S. & Allen, D. The potential for a plastic recycling facility to release microplastic pollution and possible filtration remediation effectiveness. J. Hazard. Mater. Adv. 10 , 100309 (2023).
Guo, Y. et al. Ignored microplastic sources from plastic bottle recycling. Sci. Total Environ. 838 , 156038 (2022).
Uekert, T. et al. Technical, economic, and environmental comparison of closed-loop recycling technologies for common plastics. ACS Sustain. Chem. Eng. 11 , 965–978 (2023).
UNEP. Zero draft text of the international legally binding instrument on plastic pollution, including in the marine environment. UNEP/PP/INC.3/1. Nairobi. 31 p (2023).
Walker, T. R. The tropics should not become the world’s plastic pollution problem. J. Tropic. Futur. 1 , 12–14 (2024).
Google Scholar
Liu, Z., Adams, M. & Walker, T. R. Are exports of recyclables from developed to developing countries waste pollution transfer or part of the global circular economy? Resour. Conserv. Recycl. 136 , 22–23 (2018).
Aizenshtein, E. M. Bottle wastes − to textile yarns. Fibre Chem. 47 , 343–347 (2016).
Walker, T. R. China’s ban on imported plastic waste could be a game changer. Nature 553 , 405 (2018).
Brooks, A. L., Wang, S. & Jambeck, J. R. The Chinese import ban and its impact on global plastic waste trade. Sci. Adv. 4 , eaat0131 (2018).
Article PubMed PubMed Central Google Scholar
Sun, N. & Tabata, T. Environmental impact assessment of China’s waste import ban policies: An empirical analysis of waste plastics importation from Japan. J. Clean. Prod. 329 , 129606 (2021).
Lau, W. W. Y. et al. Evaluating scenarios toward zero plastic pollution. Science 369 , 1455–1461 (2020).
Geyer, R., Jambeck, J. R. & Law, K. L. Production, use, and fate of all plastics ever made. Sci. Adv. 3 , e1700782 (2017).
Diggle, A., Walker, T. R. & Adams, M. Examining potential business impacts from the implementation of an extended producer responsibility program for printed paper and packaging waste in Nova Scotia. Canada. Circular Economy 2 , 100039 (2023).
Vidal, F. et al. Designing a circular carbon and plastics economy for a sustainable future. Nature 626 , 45–57 (2024).
von Vacano, B. et al. Sustainable design of structural and functional polymers for a circular economy. Angew. Chem. Int. Ed. 62 , e202210823 (2023).
Law, K. L. & Narayan, R. Reducing environmental plastic pollution by designing polymer materials for managed end-of-life. Nat. Rev. Mater. 7 , 104–116 (2021).
Policy Brief: Transitioning to a safe and sustainable circular economy for plastics - Ikhapp. https://ikhapp.org/stories-and-research-brief/policy-brief-transitioning-to-a-safe-and-sustainable-circular-economy-for-plastics/
MacLeod, M., Arp, H. P. H., Tekman, M. B. & Jahnke, A. The global threat from plastic pollution. Science 373 , 61–65 (2021).
Meys, R. et al. Achieving net-zero greenhouse gas emission plastics by a circular carbon economy. Science 374 , 71–76 (2021).
OECD. Extended Producer Responsibility: A Guidance Manual for Governments . (Organisation for Economic Co-operation and Development, 2001).
Dubois, M. & Eyckmans, J. Economic policy instruments. Handbook of Recycling 615–626 https://doi.org/10.1016/B978-0-323-85514-3.00021-X (2024).
European Environment Agency (EEA). Economic instruments and separate collection systems — key strategies to increase recycling – European Sources Online. Publications Office of the European Union https://www.europeansources.info/record/economic-instruments-and-separate-collection-systems-key-strategies-to-increase-recycling/ (2023).
Norway - Bottle Bill Resource Guide. https://www.bottlebill.org/index.php/current-and-proposed-laws/worldwide/norway .
Walker, T. R. Calling for a decision to launch negotiations on a new global agreement on plastic pollution at UNEA5.2. Mar. Pollut. Bull. 176 , 113447 (2022).
Dey, T. et al. Global plastic treaty should address chemicals. Science 378 , 841–842 (2022).
Schnurr, R. E. J. et al. Reducing marine pollution from single-use plastics (SUPs): A review. Mar. Pollut. Bull. 137 , 157–171 (2018).
Walker, T. R. Canada must uphold its classification of single-use plastics. Nature 624 , 522 (2023).
Xanthos, D. & Walker, T. R. International policies to reduce plastic marine pollution from single-use plastics (plastic bags and microbeads): A review. Mar. Pollut. Bull. 118 , 17–26 (2017).
Download references
Acknowledgements
Nisha Singh acknowledges the financial support received through the JAMSTEC Young Researcher Fellowship and the Ocean Frontier Institute Visiting Researcher Fellowship. This perspective was also supported by the Natural Sciences and Engineering Research Council of Canada (NSERC), Grant/Award Number: RGPIN-2018-04119 to Tony R. Walker.
Author information
Authors and affiliations.
Japan Agency for Marine-Earth Science and Technology (JAMSTEC), 2-15 Natsushimacho, Yokosuka, Kanagawa, 237-0061, Japan
Nisha Singh
School for Resource and Environmental Studies, Dalhousie University, Halifax, NS, B3H 4R2, Canada
Nisha Singh & Tony R. Walker
You can also search for this author in PubMed Google Scholar
Contributions
N.S.: Conceptualization, Methodology, Discussion, Funding acquisition, Writing-original draft, review & editing. T.R.W.: Conceptualization, Methodology, Discussion, Funding acquisition, Writing-review & editing. All authors reviewed the manuscript.
Corresponding author
Correspondence to Tony R. Walker .
Ethics declarations
Competing interests.
The authors declare no competing interests.
Additional information
Publisher’s note Springer Nature remains neutral with regard to jurisdictional claims in published maps and institutional affiliations.
Rights and permissions
Open Access This article is licensed under a Creative Commons Attribution 4.0 International License, which permits use, sharing, adaptation, distribution and reproduction in any medium or format, as long as you give appropriate credit to the original author(s) and the source, provide a link to the Creative Commons licence, and indicate if changes were made. The images or other third party material in this article are included in the article’s Creative Commons licence, unless indicated otherwise in a credit line to the material. If material is not included in the article’s Creative Commons licence and your intended use is not permitted by statutory regulation or exceeds the permitted use, you will need to obtain permission directly from the copyright holder. To view a copy of this licence, visit http://creativecommons.org/licenses/by/4.0/ .
Reprints and permissions
About this article
Cite this article.
Singh, N., Walker, T.R. Plastic recycling: A panacea or environmental pollution problem. npj Mater. Sustain. 2 , 17 (2024). https://doi.org/10.1038/s44296-024-00024-w
Download citation
Received : 25 January 2024
Accepted : 28 March 2024
Published : 01 August 2024
DOI : https://doi.org/10.1038/s44296-024-00024-w
Share this article
Anyone you share the following link with will be able to read this content:
Sorry, a shareable link is not currently available for this article.
Provided by the Springer Nature SharedIt content-sharing initiative

This article is cited by
Chemiluminescence-based evaluation of styrene block copolymers’ recyclability.
- Marius Bumbac
- Traian Zaharescu
- Istvan Borbath
Scientific Reports (2024)
Quick links
- Explore articles by subject
- Guide to authors
- Editorial policies
Sign up for the Nature Briefing newsletter — what matters in science, free to your inbox daily.

Advertisement
Practical ways to recycle plastic: current status and future aspects
- Published: 15 February 2023
- Volume 25 , pages 1249–1266, ( 2023 )
Cite this article
- Kailash Pati Pandey 1 ,
- Ujjwal R. Jha 2 ,
- Jeetesh Kushwaha 1 ,
- Madhumita Priyadarsini 1 ,
- Siddharth U. Meshram 3 &
- Abhishek S. Dhoble ORCID: orcid.org/0000-0003-4889-0885 1
2840 Accesses
16 Citations
Explore all metrics
With sky-rocketing demand and unrestricted global production, plastics have become an inseparable part of daily human life and the circular economy at large. Notwithstanding, it is crucial to consider that they lead to substantial economic losses, disrupt the ecological equilibrium, and cause environmental pollution. In this regard, several strategies have been employed in the past, such as recycling techniques, waste management systems, extended producer responsibility, reduction of incineration, plastic prohibition, and globular thinking. These methods work toward the more sustainable usage of plastics in the future, but so far, none have scaled up to the industries’ growing demands. It also reflects the current state of the art of these methods concerning the status of scientific research and gap areas in the recycling pathways. Chemical recycling seems to be one of the most efficient techniques, as it is less time consuming and the least waste is generated, but the requirement of efficient sorting makes it time consuming. While other methods generate waste and are comparatively more time consuming. Thus, each recycling method has its limitations, indicating that much work is needed to tackle the growing problem of plastic pollution. In addition, in the context of the present drawbacks of the methods, this review discusses a concomitant solution to the problem of plastic pollution via sustainable development by offering an alternative to fossil fuel-based plastic materials, i.e., biodegradable plastics.
Graphical abstract
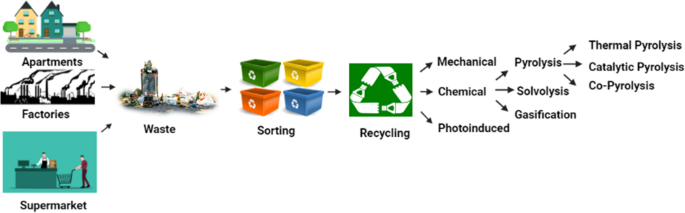
This is a preview of subscription content, log in via an institution to check access.
Access this article
Subscribe and save.
- Get 10 units per month
- Download Article/Chapter or eBook
- 1 Unit = 1 Article or 1 Chapter
- Cancel anytime
Price includes VAT (Russian Federation)
Instant access to the full article PDF.
Rent this article via DeepDyve
Institutional subscriptions
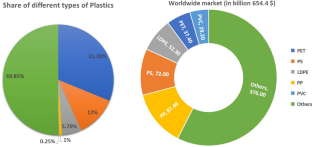
Similar content being viewed by others
Advances and Current Approach to Recycled Plastic Waste
Plastic Waste Management: Current Overview and Future Prospects
Plastic Waste and Its Eco-Friendly Management
Abbreviations.
Polyethylene terephthalate
Polystyrene
Low-density polyethylene
Polypropylene
Polyvinyl chloride
High density polyethylene
CetylTrimethyl Ammonium-PolyOxoMetalate
Fluid Catalytic Cracking
Zeolite Socony Mobil #5
Isotactic polypropylene
Polyethylene
Polyurethane
Bis (hydroxyethyl) terephthalate
Dimethyl terephthalate
Ethylenediamine
Bis(2-aminoethyl) terephthalamide
Polydiketoenamine
Lambert S, Wagner M (2017) Environmental performance of bio-based and biodegradable plastics: the road ahead. Chem Soc Rev 46(22):6855–6871. https://doi.org/10.1039/c7cs00149e
Article Google Scholar
López-Rubio A, Almenar E, Hernandez-Muñoz P, Lagarón JM, Catalá R, Gavara R (2004) Overview of active polymer-based packaging technologies for food applications. Food Rev Int 20(4):357–387. https://doi.org/10.1081/FRI-200033462
Mrowiec B (2018) Plastics in the circular economy (CE). Ochrona Srodowiska i Zasobow Naturalnych 29(4):16–19. https://doi.org/10.2478/oszn-2018-0017
World Economic Forum and 2016, The new plastics economy rethinking the future of plastics. coscienzeinrete.net, 2016 [Online]. Available: http://coscienzeinrete.net/wp-content/uploads/2017/02/WEF_The_New_Plastics_Economy.pdf . Accessed 19 Feb 2022
Sardon H, Dove AP (2018) Plastics recycling with a difference: a novel plastic with useful properties can easily be recycled again and again. Science (1979) 360(6387):380–381. https://doi.org/10.1126/SCIENCE.AAT4997/ASSET/0B77E5AE-50EE-4F68-92CC-9DB43A9A9E0F/ASSETS/GRAPHIC/360_380_F1.JPEG
Jang YC, Hong S, Lee J, Lee MJ, Shim WJ (2014) Estimation of lost tourism revenue in Geoje Island from the 2011 marine debris pollution event in South Korea. Mar Pollut Bull 81(1):49–54. https://doi.org/10.1016/j.marpolbul.2014.02.021
B Gervet, B Nordell, The use of crude oil in plastic making contributes to global warming Fluid dynamics View project Renewable Energy View project. [Online]. Available: https://www.researchgate.net/publication/266469821 . Accessed 17 Feb 2022
DKA Barnes, F Galgani, RC Thompson, M Barlaz (2009) Accumulation and Fragmentation of Plastic Debris in Global Environments. [Online]. Available: https://www.jstor.org/stable/40485977 . Accessed 17 Feb 2022
Gregory MR (2009) Environmental implications of plastic debris in marine settings- entanglement, ingestion, smothering, hangers-on, hitch-hiking and alien invasions. Philos Trans Royal Soc B 364(1526):2013–2025. https://doi.org/10.1098/rstb.2008.0265
Barboza LGA, Gimenez BCG (2015) Microplastics in the marine environment: current trends and future perspectives. Marine Pollut Bull 97(1–2):5–12. https://doi.org/10.1016/j.marpolbul.2015.06.008
Mato Y, Isobe T, Takada H, Kanehiro H, Ohtake C, Kaminuma T (2001) Plastic resin pellets as a transport medium for toxic chemicals in the marine environment. Environ Sci Technol 35(2):318–324. https://doi.org/10.1021/es0010498
Browne MA, Dissanayake A, Galloway TS, Lowe DM, Thompson RC (2008) Ingested microscopic plastic translocates to the circulatory system of the mussel, Mytilus edulis (L.). Environ Sci Technol 42(13):5026–5031. https://doi.org/10.1021/es800249a
Hammer J, Kraak MHS, Parsons JR (2012) Plastics in the marine environment: the dark side of a modern gift. Rev Environ Contam Toxicol 220:1–44. https://doi.org/10.1007/978-1-4614-3414-6_1
Okunola AA, Kehinde IO, Oluwaseun A, Olufiropo AE (2019) Public and environmental health effects of plastic wastes disposal: a review. J Toxicol Risk Assess. https://doi.org/10.23937/2572-4061.1510021
Okoffo ED, O’Brien S, O’Brien JW, Tscharke BJ, Thomas KV (2019) Wastewater treatment plants as a source of plastics in the environment: a review of occurrence, methods for identification, quantification and fate. Environ Sci 5(11):1908–1931. https://doi.org/10.1039/c9ew00428a
Tetu SG et al (2019) Plastic leachates impair growth and oxygen production in Prochlorococcus the ocean’s most abundant photosynthetic bacteria. Commun Biol. https://doi.org/10.1038/s42003-019-0410-x
Andrade AJM, Grande SW, Talsness CE, Grote K, Chahoud I (2006) A dose-response study following in utero and lactational exposure to di-(2-ethylhexyl)-phthalate (DEHP): non-monotonic dose-response and low dose effects on rat brain aromatase activity. Toxicology 227(3):185–192. https://doi.org/10.1016/j.tox.2006.07.022
Prata JC, da Costa JP, Lopes I, Duarte AC, Rocha-Santos T (2020) Environmental exposure to microplastics: an overview on possible human health effects. Sci Total Environ 702:134455. https://doi.org/10.1016/J.SCITOTENV.2019.134455
Mason SA, Welch VG, Neratko J (2018) Synthetic polymer contamination in bottled water. Front Chem. https://doi.org/10.3389/fchem.2018.00407
Anastas PT, Beach ES (2007) Green chemistry: the emergence of a transformative framework. Green Chem Lett Rev 1(1):9–24. https://doi.org/10.1080/17518250701882441
European Commission (2019) A circular economy for plastics—insights from research and innovation to inform policy and funding decisions. European Commission, Brussels, p 206. https://doi.org/10.2777/269031
Book Google Scholar
Leal Filho W et al (2019) An overview of the problems posed by plastic products and the role of extended producer responsibility in Europe. J Clean Prod 214:550–558. https://doi.org/10.1016/j.jclepro.2018.12.256
Rebeiz KS, Craft AP (1995) Plastic waste management in construction: technological and institutional issues. Resour Conserv Recycl 15:245–257
Singh P, Sharma VP (2016) Integrated plastic waste management: environmental and improved health approaches. Procedia Environ Sci 35:692–700. https://doi.org/10.1016/j.proenv.2016.07.068
Single-use plastics: A roadmap for sustainability | UNEP - UN Environment Programme. https://www.unep.org/resources/report/single-use-plastics-roadmap-sustainability . Accessed 16 Feb 2022
E Watkins, S Gionfra, J-P Schweitzer, M Pantzar, CJ Patrick Ten Brink, (2017) EPR in the EU plastics strategy and the circular economy: a focus on plastic packaging. [Online]. Available: www.ieep.eu . Accessed 17 Feb 2022
Thompson RC, Moore CJ, Saal FSV, Swan SH (2009) Plastics, the environment and human health: current consensus and future trends. Philos Trans R Soc B 364(1526):2153–2166. https://doi.org/10.1098/rstb.2009.0053
Satapathy S (2017) An analysis of barriers for plastic recycling in the Indian plastic industry. Benchmarking 24(2):415–430. https://doi.org/10.1108/BIJ-11-2014-0103
Scott G (1976) Some chemical problems in the recycling of plastics. Resour Recover Conserv 1(4):381–395. https://doi.org/10.1016/0304-3967(76)90027-5
Alabi OA, Bakare AA, Xu X, Li B, Zhang Y, Huo X (2012) Comparative evaluation of environmental contamination and DNA damage induced by electronic-waste in Nigeria and China. Sci Total Environ 423:62–72. https://doi.org/10.1016/J.SCITOTENV.2012.01.056
Sepúlveda A et al (2010) A review of the environmental fate and effects of hazardous substances released from electrical and electronic equipments during recycling: examples from China and India. Environ Impact Assess Rev 30(1):28–41. https://doi.org/10.1016/j.eiar.2009.04.001
Zhang XL, Luo XJ, Liu HY, Yu LH, Chen SJ, Mai BX (2011) Bioaccumulation of several brominated flame retardants and dechlorane plus in waterbirds from an e-waste recycling region in south China: associated with trophic level and diet sources. Environ Sci Technol 45(2):400–405. https://doi.org/10.1021/es102251s
V Chandegara, MT Kumpavat, K Patel, (2015) Plastic Packaging Waste Impact on Climate Change and its Mitigation Design and Fabrication of Evaporative Cooling Transportation System View project Response of wheat water use efficiency, water productivity and yield attributes to soil moisture tension based irrigation scheduling in clay loam soils of semi arid region View project. [Online]. Available: https://www.researchgate.net/publication/284726839 . Accessed 17 Feb 2022
Balwada J, Samaiya S, Mishra RP (2021) Packaging plastic waste management for a circular economy and identifying a better waste collection system using analytical hierarchy process (AHP). Procedia CIRP 98:270–275. https://doi.org/10.1016/J.PROCIR.2021.01.102
Browning S, Beymer-Farris B, Seay JR (2021) Addressing the challenges associated with plastic waste disposal and management in developing countries. Curr Opin Chem Eng 32:100682. https://doi.org/10.1016/J.COCHE.2021.100682
Hahladakis JN, Purnell P, Iacovidou E, Velis CA, Atseyinku M (2018) Post-consumer plastic packaging waste in England: assessing the yield of multiple collection-recycling schemes. Waste Manag 75:149–159. https://doi.org/10.1016/J.WASMAN.2018.02.009
Tong YD, Huynh TDX, Khong TD (2021) Understanding the role of informal sector for sustainable development of municipal solid waste management system: a case study in Vietnam. Waste Manag 124:118–127. https://doi.org/10.1016/J.WASMAN.2021.01.033
Hopewell J, Dvorak R, Kosior E (2009) Plastics recycling: challenges and opportunities. Philos Trans R Soc B 364(1526):2115–2126. https://doi.org/10.1098/RSTB.2008.0311
Arvanitoyannis IS, Bosnea LA (2001) Recycling of polymeric materials used for food packaging: current status and perspectives. Food Rev Intl 17(3):291–346. https://doi.org/10.1081/FRI-100104703
Silveira AVM, Cella M, Tanabe EH, Bertuol DA (2018) Application of tribo-electrostatic separation in the recycling of plastic wastes. Process Saf Environ Prot 114:219–228. https://doi.org/10.1016/J.PSEP.2017.12.019
Censori M, la Marca F, Carvalho MT (2016) Separation of plastics: the importance of kinetics knowledge in the evaluation of froth flotation. Waste Manag 54:39–43. https://doi.org/10.1016/J.WASMAN.2016.05.021
Serranti S, Luciani V, Bonifazi G, Hu B, Rem PC (2015) An innovative recycling process to obtain pure polyethylene and polypropylene from household waste. Waste Manag 35:12–20. https://doi.org/10.1016/J.WASMAN.2014.10.017
Ozdemir ME, Ali Z, Subeshan B, Asmatulu E (2021) Applying machine learning approach in recycling. J Mater Cycles Waste Manag 23:855–871. https://doi.org/10.1007/s10163-021-01182-y
Taylor RL, Villas-Boas SB (2016) Bans vs. fees: disposable carryout bag policies and bag usage. Appl Econ Perspect Pol 38(2):351–372. https://doi.org/10.1093/AEPP/PPV025
Schnurr REJ et al (2018) Reducing marine pollution from single-use plastics (SUPs): a review. Mar Pollut Bull 137:157–171. https://doi.org/10.1016/J.MARPOLBUL.2018.10.001
Article MathSciNet Google Scholar
Omondi I, Asari M (2021) A study on consumer consciousness and behavior to the plastic bag ban in Kenya. J Mater Cycles Waste Manag 23:425–435. https://doi.org/10.1007/s10163-020-01142-y
Ragaert K, Delva L, van Geem K (2017) Mechanical and chemical recycling of solid plastic waste. Waste Manag 69:24–58. https://doi.org/10.1016/J.WASMAN.2017.07.044
Lee J, Kwon EE, Lam SS, Chen WH, Rinklebe J, Park YK (2021) Chemical recycling of plastic waste via thermocatalytic routes. J Clean Prod 321:128989. https://doi.org/10.1016/J.JCLEPRO.2021.128989
Sharuddin SDA, Abnisa F, Daud WMAW, Aroua MK (2018) Pyrolysis of plastic waste for liquid fuel production as prospective energy resource. IOP Conf Ser Mater Sci Eng 334(1):012001. https://doi.org/10.1088/1757-899X/334/1/012001
Qin L, Xu Z, Zhao B, Zou C, Chen W, Han J (2022) Kinetic study on high-temperature gasification of medical plastic waste coupled with hydrogen-rich syngas production catalyzed by steel-converter ash. J Energy Inst 102:14–21. https://doi.org/10.1016/J.JOEI.2022.02.005
Hou Q et al (2021) Upcycling and catalytic degradation of plastic wastes. Cell Rep Phys Sci 2(8):100514. https://doi.org/10.1016/J.XCRP.2021.100514
Shen M et al (2021) Can incineration completely eliminate plastic wastes? An investigation of microplastics and heavy metals in the bottom ash and fly ash from an incineration plant. Sci Total Environ 779:146528. https://doi.org/10.1016/J.SCITOTENV.2021.146528
Coates GW, Getzler YDYL (2020) Chemical recycling to monomer for an ideal, circular polymer economy. Nat Rev Mater 5(7):501–516. https://doi.org/10.1038/s41578-020-0190-4
Rahimi AR, Garciá JM (2017) Chemical recycling of waste plastics for new materials production. Nat Rev Chem 1(6):1–11. https://doi.org/10.1038/s41570-017-0046
Banu JR, Sharmila VG, Ushani U, Amudha V, Kumar G (2020) Impervious and influence in the liquid fuel production from municipal plastic waste through thermo-chemical biomass conversion technologies—a review. Sci Total Environ. https://doi.org/10.1016/j.scitotenv.2020.137287
Bartolome L, Imran M, Cho BG, Al-Masry WA, Kim DH (2012) Recent developments in the chemical recycling of PET. Mater Recycl Trends Perspect. https://doi.org/10.5772/33800
Sinha V, Patel MR, Patel JV (2010) Pet waste management by chemical recycling: a review. J Polym Environ 18(1):8–25. https://doi.org/10.1007/s10924-008-0106-7
Kannan P, Al Shoaibi A, Srinivasakannan C (2014) Temperature effects on the yield of gaseous olefins from waste polyethylene via flash pyrolysis. Energy Fuels 28(5):3363–3366. https://doi.org/10.1021/ef500516n
Zhang Y et al (2020) Exergy and energy analysis of pyrolysis of plastic wastes in rotary kiln with heat carrier. Process Saf Environ Prot 142:203–211. https://doi.org/10.1016/j.psep.2020.06.021
Fakhrhoseini SM, Dastanian M (2013) Predicting pyrolysis products of PE, PP, and PET using NRTL activity coefficient model. J Chem. https://doi.org/10.1155/2013/487676
Ahmad I et al (2015) Pyrolysis study of polypropylene and polyethylene into premium oil products. Int J Green Energy 12(7):663–671. https://doi.org/10.1080/15435075.2014.880146
S Kumar, RK Singh Recovery of hydrocarbon liquid from waste high density polyethylene by thermal pyrolysis. 28(04):659–667 [Online]. Available: www.abeq.org.br/bjche . Accessed 17 Feb 2022
Marcilla A, Beltrán MI, Navarro R (2009) Thermal and catalytic pyrolysis of polyethylene over HZSM5 and HUSY zeolites in a batch reactor under dynamic conditions. Appl Catal B 86(1–2):78–86. https://doi.org/10.1016/j.apcatb.2008.07.026
van der Westhuizen S, Collard FX, Görgens J (2022) Pyrolysis of waste polystyrene into transportation fuel: effect of contamination on oil yield and production at pilot scale. J Anal Appl Pyrolysis. https://doi.org/10.1016/j.jaap.2021.105407
López A, de Marco I, Caballero BM, Laresgoiti MF, Adrados A, Torres A (2011) Pyrolysis of municipal plastic wastes II: influence of raw material composition under catalytic conditions. Waste Manag 31(9–10):1973–1983. https://doi.org/10.1016/j.wasman.2011.05.021
Elordi G et al (2009) Catalytic pyrolysis of HDPE in continuous mode over zeolite catalysts in a conical spouted bed reactor. J Anal Appl Pyrolysis 85(1–2):345–351. https://doi.org/10.1016/J.JAAP.2008.10.015
Fuentes-Ordóñez EG, Salbidegoitia JA, Ayastuy JL, Gutiérrez-Ortiz MA, González-Marcos MP, González-Velasco JR (2014) High external surface Pt/zeolite catalysts for improving polystyrene hydrocracking. Catal Today 227:163–170. https://doi.org/10.1016/j.cattod.2013.09.004
Ochoa R, van Woert H, Lee WH, Subramanian R, Kugler E, Eklund PC (1996) Catalytic degradation of medium density polyethylene over silica-alumina supports. Fuel Process Technol 49:119–136
Buekens AG, Huang H (1998) Catalytic plastics cracking for recovery of gasoline-range hydrocarbons from municipal plastic wastes. Resour Conserv Recycl 23:163–181
Stelmachowski M (2010) Thermal conversion of waste polyolefins to the mixture of hydrocarbons in the reactor with molten metal bed. Energy Convers Manag 51(10):2016–2024. https://doi.org/10.1016/j.enconman.2010.02.035
J Schirmer, JS Kim, E Klemm (2001) Catalytic degradation of polyethylene using thermal gravimetric analysis and a cycled-spheres-reactor. [Online]. Available: www.elsevier.com/locate/jaap . Accessed 17 Feb 2022
Liu X, Burra KG, Wang Z, Li J, Che D, Gupta AK (2020) On deconvolution for understanding synergistic effects in co-pyrolysis of pinewood and polypropylene. Appl Energy. https://doi.org/10.1016/j.apenergy.2020.115811
Čepelioʇullar Ö, Pütün AE (2014) Products characterization study of a slow pyrolysis of biomass-plastic mixtures in a fixed-bed reactor. J Anal Appl Pyrolysis 110(1):363–374. https://doi.org/10.1016/j.jaap.2014.10.002
Chen W, Shi S, Chen M, Zhou X (2017) Fast co-pyrolysis of waste newspaper with high-density polyethylene for high yields of alcohols and hydrocarbons. Waste Manag 67:155–162. https://doi.org/10.1016/j.wasman.2017.05.032
Wang X et al (2019) Synergistic effect of biomass and polyurethane waste co-pyrolysis on soot formation at high temperatures. J Environ Manag 239:306–315. https://doi.org/10.1016/j.jenvman.2019.03.073
Duan D et al (2017) Ex-situ catalytic co-pyrolysis of lignin and polypropylene to upgrade bio-oil quality by microwave heating. Bioresour Technol 241:207–213. https://doi.org/10.1016/J.BIORTECH.2017.04.104
Zhang X, Lei H, Chen S, Wu J (2016) Catalytic co-pyrolysis of lignocellulosic biomass with polymers: a critical review. Green Chem 18(15):4145–4169. https://doi.org/10.1039/c6gc00911e
Muneer B, Zeeshan M, Qaisar S, Razzaq M, Iftikhar H (2019) Influence of in-situ and ex-situ HZSM-5 catalyst on co-pyrolysis of corn stalk and polystyrene with a focus on liquid yield and quality. J Clean Prod. https://doi.org/10.1016/j.jclepro.2019.117762
Li X et al (2013) Improving the aromatic production in catalytic fast pyrolysis of cellulose by co-feeding low-density polyethylene. Appl Catal A Gen 455:114–121. https://doi.org/10.1016/j.apcata.2013.01.038
Rutkowski P (2012) Chemical composition of bio-oil produced by co-pyrolysis of biopolymer/polypropylene mixtures with K2CO3 and ZnCl 2 addition. J Anal Appl Pyrolysis 95:38–47. https://doi.org/10.1016/j.jaap.2012.01.003
Alvarez-Castillo A, Castafio VM (1995) Modification of polyester resins by an oligomeric additive. Polym Bull 35:187–194
Pang K, Kotek R, Tonelli A (2006) Review of conventional and novel polymerization processes for polyesters. Progress Polym Sci (Oxford) 31(11):1009–1037. https://doi.org/10.1016/j.progpolymsci.2006.08.008
J Clark, J Alonso, J Villalba, J Aguado, D Serrano (1999) Feedstock recycling of plastic wastes. [Online]. Available: https://books.google.co.in/books?hl=en&lr=&id=HUlLAvuGsoQC&oi=fnd&pg=PR5&dq=eedstock+Recycling+of+Plastic+Wastes+-+James+H.+Clark,+Jos%C3%A9+Aguado+Alonso,+Jos%C3%A9+Aguado+Villalba,+Jos%C3%A9+Aguado,+David+P.+Serrano,+D.+A.+Serrano+-+Google+Books&ots=AAxC7sB36J&sig=wq_OLqzZOwxfdoEJp_McNVi5JiQ . Accessed 19 Feb 2022
Dietz HJ, Freese RE, Ter R, Assignors to Eastman Kodak Company (1968) Sub removal from polyester scrap. Patent 105(568):504
Kurokawa H, Ohshima MA, Sugiyama K, Miura H (2003) Methanolysis of polyethylene terephthalate (PET) in the presence of aluminium tiisopropoxide catalyst to form dimethyl terephthalate and ethylene glycol. Polym Degrad Stab 79(3):529–533. https://doi.org/10.1016/S0141-3910(02)00370-1
Campanelli JR, Kamal MR, Cooper DG (1993) A kinetic study of the hydrolytic degradation of polyethylene terephthalate at high temperatures. J Appl Polym Sci 48(3):443–451. https://doi.org/10.1002/APP.1993.070480309
Tawfik ME, Eskander SB (2010) Chemical recycling of poly(ethylene terephthalate) waste using ethanolamine. Sorting of the end products. Polym Degrad Stab 95(2):187–194. https://doi.org/10.1016/J.POLYMDEGRADSTAB.2009.11.026
Hoang CN, Dang YH (2013) Aminolysis of poly(ethylene terephthalate) waste with ethylenediamine and characterization of α, ω-diamine products. Polym Degrad Stab 98(3):697–708. https://doi.org/10.1016/J.POLYMDEGRADSTAB.2012.12.026
M Khallaf (2011) The impact of air pollution on health, economy, environment and agricultural sources. [Online]. Available: https://books.google.co.in/books?hl=en&lr=&id=-eqcDwAAQBAJ&oi=fnd&pg=PR11&dq=(The+Impact+of+Air+Pollution+on+Health,+Economy,+Environment+and+Agricultural+Sources%3B+Khallaf,+M.,+Ed.%3B+Intech+Open:+London,+UK,+2011.)&ots=elT7JpSiQP&sig=GbgEiMRXl7sCNmwq710Nb3qsnh8 . Accessed 19 Feb 2022
Lopez G, Artetxe M, Amutio M, Alvarez J, Bilbao J, Olazar M (2018) Recent advances in the gasification of waste plastics. A critical overview. Renew Sustain Energy Rev 82:576–596. https://doi.org/10.1016/J.RSER.2017.09.032
Elordi G, Olazar M, Lopez G, Artetxe M, Bilbao J (2011) Product yields and compositions in the continuous pyrolysis of high-density polyethylene in a conical spouted bed reactor. Ind Eng Chem Res 50(11):6650–6659. https://doi.org/10.1021/IE200186M
Jung SH, Kim SJ, Kim JS (2013) The influence of reaction parameters on characteristics of pyrolysis oils from waste high impact polystyrene and acrylonitrile–butadiene–styrene using a fluidized bed reactor. Fuel Process Technol 116:123–129. https://doi.org/10.1016/J.FUPROC.2013.05.004
Chai Y, Gao N, Wang M, Wu C (2020) H2 production from co-pyrolysis/gasification of waste plastics and biomass under novel catalyst Ni-CaO-C. Chem Eng J 382:122947. https://doi.org/10.1016/J.CEJ.2019.122947
Sommerhuber PF, Welling J, Krause A (2015) Substitution potentials of recycled HDPE and wood particles from post-consumer packaging waste in wood-plastic composites. Waste Manag 46:76–85. https://doi.org/10.1016/J.WASMAN.2015.09.011
Aurrekoetxea J, Sarrionandia MA, Urrutibeascoa I, Maspoch ML (2001) Fracture behaviour of virgin and recycled isotactic polypropylene. J Mater Sci 36(21):5073–5078. https://doi.org/10.1023/A:1012460804698
Gahleitner M, Fiebig J, Wolfschwenger J, Dreiling G, Paulik C (2007) Post-crystallization and physical aging of polypropylene: material and processing effects. J Macromol Sci Part B 41 B(4–6):833–849. https://doi.org/10.1081/MB-120013068
Yang K, Yang Q, Li G, Sun Y, Feng D (2006) Morphology and mechanical properties of polypropylene/calcium carbonate nanocomposites. Mater Lett 60(6):805–809. https://doi.org/10.1016/J.MATLET.2005.10.020
Chan CM, Wu J, Li JX, Cheung YK (2002) Polypropylene/calcium carbonate nanocomposites. Polymer (Guildf) 43(10):2981–2992. https://doi.org/10.1016/S0032-3861(02)00120-9
Suzuki G et al (2022) Mechanical recycling of plastic waste as a point source of microplastic pollution. Environ Pollut. https://doi.org/10.1016/J.ENVPOL.2022.119114
Day M, Wiles DM (1972) Photochemical degradation of poly(ethylene terephthalate). I. Irradiation experiments with the xenon and carbon arc. J Appl Polym Sci 16(1):175–189. https://doi.org/10.1002/APP.1972.070160116
Hoffmann MR, Martin ST, Choi W, Bahnemann DW (1995) Environmental applications of semiconductor photocatalysis. Chem Rev 95(1):69–96. https://doi.org/10.1021/CR00033A004/ASSET/CR00033A004.FP.PNG_V03
Gusain R, Gupta K, Joshi P, Khatri OP (2019) Adsorptive removal and photocatalytic degradation of organic pollutants using metal oxides and their composites: a comprehensive review. Adv Colloid Interface Sci 272:102009. https://doi.org/10.1016/J.CIS.2019.102009
Kolek Z (2001) Recycled polymers from food packaging in relation to environmental protection. Pol J Environ Stud 10:73–76
Google Scholar
Garcia JM, Robertson ML (2017) The future of plastics recycling. Science (1979) 358(6365):870–872. https://doi.org/10.1126/SCIENCE.AAQ0324/ASSET/53AC3A00-0BBA-41D7-A05A-BE986DAF5476/ASSETS/GRAPHIC/358_870_F1.JPEG
Foti S, Giuffrida M, Maravigna P, Montaudo G (1984) Direct mass spectrometry of polymers. XI. Primary thermal fragmentation processes in aromatic–aliphatic polyesters. J Polym Sci 22(6):1217–1229. https://doi.org/10.1002/POL.1984.170220602
Zhao XU, Li Z, Chen Y, Shi L, Zhu Y (2007) Solid-phase photocatalytic degradation of polyethylene plastic under UV and solar light irradiation. J Mol Catal A Chem 268(1–2):101–106. https://doi.org/10.1016/J.MOLCATA.2006.12.012
Miandad R, Barakat MA, Rehan M, Aburiazaiza AS, Ismail IMI, Nizami AS (2017) Plastic waste to liquid oil through catalytic pyrolysis using natural and synthetic zeolite catalysts. Waste Manag 69:66–78. https://doi.org/10.1016/J.WASMAN.2017.08.032
Vermeulen I, van Caneghem J, Block C, Baeyens J, Vandecasteele C (2011) Automotive shredder residue (ASR): reviewing its production from end-of-life vehicles (ELVs) and its recycling, energy or chemicals’ valorisation. J Hazard Mater 190(1–3):8–27. https://doi.org/10.1016/J.JHAZMAT.2011.02.088
Meys R, Frick F, Westhues S, Sternberg A, Klankermayer J, Bardow A (2020) Towards a circular economy for plastic packaging wastes—the environmental potential of chemical recycling. Resour Conserv Recycl 162:105010. https://doi.org/10.1016/J.RESCONREC.2020.105010
Volk R et al (2021) Techno-economic assessment and comparison of different plastic recycling pathways: a German case study. J Ind Ecol 25(5):1318–1337. https://doi.org/10.1111/JIEC.13145
Demirbas A (2007) Biodegradable plastics from renewable resources. Energy Sour Part A 29(5):419–424. https://doi.org/10.1080/009083190965820
Elordi G, Olazar M, Lopez G, Castaño P, Bilbao J (2011) Role of pore structure in the deactivation of zeolites (HZSM-5, Hβ and HY) by coke in the pyrolysis of polyethylene in a conical spouted bed reactor. Appl Catal B 102(1–2):224–231. https://doi.org/10.1016/J.APCATB.2010.12.002
Kunwar B, Cheng HN, Chandrashekaran SR, Sharma BK (2016) Plastics to fuel: a review. Renew Sustain Energy Rev 54:421–428. https://doi.org/10.1016/J.RSER.2015.10.015
Panda AK, Singh RK, Mishra DK (2010) Thermolysis of waste plastics to liquid fuel: a suitable method for plastic waste management and manufacture of value added products—a world prospective. Renew Sustain Energy Rev 14(1):233–248. https://doi.org/10.1016/J.RSER.2009.07.005
Zhang Y, Duan D, Lei H, Villota E, Ruan R (2019) Jet fuel production from waste plastics via catalytic pyrolysis with activated carbons. Appl Energy 251:113337. https://doi.org/10.1016/J.APENERGY.2019.113337
Cleetus C, Thomas S, Varghese S (2013) Synthesis of petroleum-based fuel from waste plastics and performance analysis in a CI engine. J Energy. https://doi.org/10.1155/2013/608797
Celik G et al (2019) Upcycling single-use polyethylene into high-quality liquid products. ACS Cent Sci 5(11):1795–1803. https://doi.org/10.1021/ACSCENTSCI.9B00722/SUPPL_FILE/OC9B00722_SI_003.PDF
Mishra RK, Maria HJ, Joseph K, Thomas S (2017) Basic structural and properties relationship of recyclable microfibrillar composite materials from immiscible plastics blends: an introduction. Micro and nano fibrillar composites (MFCs and NFCs) from polymer blends. Elsevier, Amsterdam, pp 1–25
Stein RS (1992) Emerging technologies in plastics recycling. American Chemical Society, Washington. https://doi.org/10.1021/bk-1992-0513.ch004
Bates FS, Hillmyer MA, Lodge TP, Bates CM, Delaney KT, Fredrickson GH (2012) Multiblock polymers: panacea or Dandora’s box? Science (1979) 336(6080):434–440. https://doi.org/10.1126/SCIENCE.1215368/SUPPL_FILE/1215368.BATES.SM.PDF
Article MATH Google Scholar
Creton C (2017) Molecular stitches for enhanced recycling of packaging: an additive creates tough blends from waste polyethylene and polypropylene. Science (1979) 355(6327):797–798. https://doi.org/10.1126/SCIENCE.AAM5803/ASSET/FDFC0458-E3C5-40A9-853E-C047DAA4D5E2/ASSETS/GRAPHIC/355_797_F1.JPEG
Lin Y, Yakovleva V, Chen H, Hiltner A, Baer E (2009) Comparison of olefin copolymers as compatibilizers for polypropylene and high-density polyethylene. J Appl Polym Sci 113(3):1945–1952. https://doi.org/10.1002/APP.30190
Nwabunma D, Kyu T (2007) Polyolefin blends. John Wiley & Sons Inc, Hoboken, pp 1–667. https://doi.org/10.1002/9780470199008
Eagan JM et al (2017) Combining polyethylene and polypropylene: enhanced performance with PE/iPP multiblock polymers. Science (1979) 355(6327):814–816. https://doi.org/10.1126/SCIENCE.AAH5744/SUPPL_FILE/EAGAN.SM.PDF
Huysman S, de Schaepmeester J, Ragaert K, Dewulf J, de Meester S (2017) Performance indicators for a circular economy: a case study on post-industrial plastic waste. Resour Conserv Recycl 120:46–54. https://doi.org/10.1016/J.RESCONREC.2017.01.013
Morseletto P (2020) Targets for a circular economy. Resour Conserv Recycl 153:104553. https://doi.org/10.1016/J.RESCONREC.2019.104553
Stahel WR (2016) The circular economy. Nature 531(7595):435–438. https://doi.org/10.1038/531435a
Thunman H et al (2019) Circular use of plastics-transformation of existing petrochemical clusters into thermochemical recycling plants with 100% plastics recovery. Sustain Mater Technol 22:e00124. https://doi.org/10.1016/J.SUSMAT.2019.E00124
Sardon H, Dove AP (2018) Plastics recycling with a difference: a novel plastic with useful properties can easily be recycled again and again. Science 360(6387):380–381. https://doi.org/10.1126/science.aat4997
Zhu JB, Watson EM, Tang J, Chen EYX (2018) A synthetic polymer system with repeatable chemical recyclability. Science (1979) 360(6387):398–403. https://doi.org/10.1126/SCIENCE.AAR5498/SUPPL_FILE/AAR5498-ZHU-SM.PDF
Christensen PR, Scheuermann AM, Loeffler KE, Helms BA (2019) Closed-loop recycling of plastics enabled by dynamic covalent diketoenamine bonds. Nat Chem 11(5):442–448. https://doi.org/10.1038/s41557-019-0249-2
Baker MS, Kim H, Olah MG, Lewis GG, Phillips ST (2015) Depolymerizable poly(benzyl ether)-based materials for selective room temperature recycling. Green Chem 17(9):4541–4545. https://doi.org/10.1039/C5GC01090J
DiLauro AM, Lewis GG, Phillips ST (2015) Self-immolative Poly(4,5-dichlorophthalaldehyde) and its applications in multi-stimuli-responsive macroscopic plastics. Angew Chem 127(21):6298–6303. https://doi.org/10.1002/ANGE.201501320
García JM et al (2014) Recyclable, strong thermosets and organogels via paraformaldehyde condensation with diamines. Science (1979) 344(6185):732–735. https://doi.org/10.1126/SCIENCE.1251484/SUPPL_FILE/GARCIA.SM.PDF
Sagi A, Weinstain R, Karton N, Shabat D (2008) Self-immolative polymers the increasing need for effective diagnostic. J Am Chem Soc. https://doi.org/10.1021/ja801065d
Fan B, Trant JF, Wong AD, Gillies ER (2014) Polyglyoxylates: a versatile class of triggerable self-immolative polymers from readily accessible monomers. J Am Chem Soc. https://doi.org/10.1021/ja504727u
Ogden WA, Guan Z (2018) Recyclable, strong, and highly malleable thermosets based on boroxine networks. J Am Chem Soc. https://doi.org/10.1021/jacs.8b03257
Patel V, Popli S, Bhatt D (2014) Utilization of plastic waste in construction of roads. Int J Sci Res 3(4):161–163
Appiah JK, Berko-Boateng VN, Tagbor TA (2017) Use of waste plastic materials for road construction in Ghana. Case Stud Constr Mater 6:1–7. https://doi.org/10.1016/J.CSCM.2016.11.001
Royer SJ, Ferrón S, Wilson ST, Karl DM (2018) Production of methane and ethylene from plastic in the environment. PLoS ONE 13(8):e0200574. https://doi.org/10.1371/JOURNAL.PONE.0200574
I Bayrakdar (2021) Japanese Inventor Built a Machine That Turns Plastic Bags into Oil, Irmak Bayrakdar . https://interestingengineering.com/japanese-invention-converts-plastic-into-oil . Accessed 12 Mar 2022
K Systems, Large Scale Plastics Pyrolysis System to Diesel Fuel | Cogeneration & Recycling Technology | Klean Industries. https://kleanindustries.com/waste-processing-projects/plastic-pyrolysis-recycling/spr-japan/ . Accessed 12 Mar 2022
Turning plastic pollution into green energy—Who we Are—Newcastle University. https://www.ncl.ac.uk/who-we-are/vision/green-energy/ . Accessed 12 Mar 12 2022
Divya VC, Ameen Khan M, Nageshwar Rao B, Sailaja RRN (2015) High density polyethylene/cenosphere composites reinforced with multi-walled carbon nanotubes: mechanical, thermal and fire retardancy studies. Mater Design (1980–2015) 65:377–386. https://doi.org/10.1016/J.MATDES.2014.08.076
Download references
Acknowledgements
This work was partly supported by the Indian Institute of Technology (Banaras Hindu University) Varanasi’s Seed Grant Ref. No. IIT (BHU)/Budget/19-(14)/2021-22/2268 dated 06-July-2021 as well as Department of Science and Technology (DST), Government of India’s Project No. DST/SEED/SUTRA/2020/132(G) dated 24-June-2021, both to ASD.
Author information
Authors and affiliations.
School of Biochemical Engineering, Indian Institute of Technology (BHU), Varanasi, Uttar Pradesh, 221005, India
Kailash Pati Pandey, Jeetesh Kushwaha, Madhumita Priyadarsini & Abhishek S. Dhoble
Department of Chemical Engineering, Birla Institute of Technology and Science (BITS), Pilani, Rajasthan, India
Ujjwal R. Jha
Department of Applied Chemistry, Laxminarayan Institute of Technology (LIT), Nagpur, Maharashtra, India
Siddharth U. Meshram
You can also search for this author in PubMed Google Scholar
Contributions
KPP: Conceptualization-Equal, Investigation-Equal, Data curation-Equal, Formal analysis-Lead, Methodology-Equal, Validation-Equal, Visualization-Equal, Writing—original draft-Lead; URJ: Methodology-Equal, Resources-Equal, Validation-Equal, Writing—review and editing-Equal; JK: Writing review and editing, Investigation-Equal, Data curation-Equal; MP: Writing review and editing, Investigation-Equal, Formal analysis-Supporting; SUM: Data curation-Supporting, Formal analysis-Supporting, Investigation-Supporting; ASD: Conceptualization-Lead, Data curation-Supporting, Formal analysis-Supporting, Funding acquisition-Lead, Investigation-Supporting, Methodology-Supporting, Project administration-Lead, Resources-Lead, Supervision-Lead, Validation-Lead, Visualization-Supporting, Writing—original draft-Supporting, Writing—review and editing-Equal.
Corresponding author
Correspondence to Abhishek S. Dhoble .
Ethics declarations
Conflict of interest.
The authors declare that they have no known competing financial interests or personal relationships that could have appeared to influence the work reported in this paper.
Additional information
Publisher's note.
Springer Nature remains neutral with regard to jurisdictional claims in published maps and institutional affiliations.
Rights and permissions
Springer Nature or its licensor (e.g. a society or other partner) holds exclusive rights to this article under a publishing agreement with the author(s) or other rightsholder(s); author self-archiving of the accepted manuscript version of this article is solely governed by the terms of such publishing agreement and applicable law.
Reprints and permissions
About this article
Pandey, K.P., Jha, U.R., Kushwaha, J. et al. Practical ways to recycle plastic: current status and future aspects. J Mater Cycles Waste Manag 25 , 1249–1266 (2023). https://doi.org/10.1007/s10163-023-01611-0
Download citation
Received : 10 July 2022
Accepted : 30 January 2023
Published : 15 February 2023
Issue Date : May 2023
DOI : https://doi.org/10.1007/s10163-023-01611-0
Share this article
Anyone you share the following link with will be able to read this content:
Sorry, a shareable link is not currently available for this article.
Provided by the Springer Nature SharedIt content-sharing initiative
- Plastic pollution
- Chemical recycling
- Mechanical recycling
- Recyclable polymers
- Circular economy
- Cold-plasma pyrolysis
- Find a journal
- Publish with us
- Track your research

IMAGES