- Associations
- Working Groups
- Escardio.org
- ESC eLearning
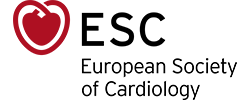

Show navigation Hide navigation
- European Society of Cardiology
- ESC Journal Family
Cardiovascular Research
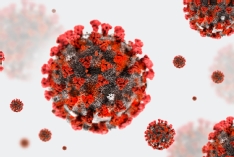
Resource library and new ESC Video Series.
The journal of basic, translational and clinical research
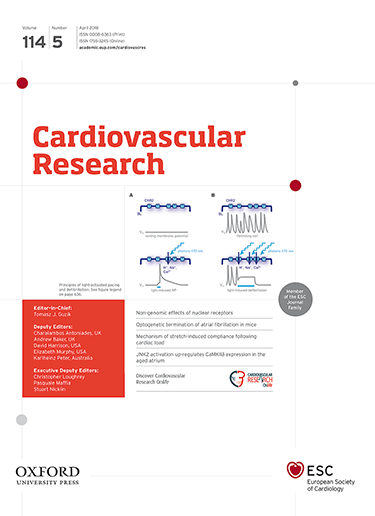
Cardiovascular Research (CVR) is the international journal of the European Society of Cardiology that deals with basic and translational research across different disciplines and areas.
The journal enhances insights into cardiovascular disease mechanisms and innovation.
Submit your paper
- Editor-in-Chief: Tomasz J. Guzik, UK
- Current volume: 120 (year 2024)
- Issues per subscription: 18
- Impact Factor 2023: 10.4
Advertising, Reprints and Supplements
Oxford University Press (OUP), publisher of this ESC journal, offers a number of services which help you reach ESC audiences or re-use the content published by the journals for educational purposes:
• Print and digital advertising puts your marketing message in front of ESC journal readers • A peer-reviewed supplement communicates scientific research via a themed collection of papers • Reprints and ePrints are an ideal format for dissemination of published papers • Licensing ESC content for excerpted local editions or CME ensures the best-possible content for your region
Please contact OUP at [email protected] for further information.
Editor-in-Chief History
Free access for esc professional members.
ESC Professional Members benefit from free online access to Cardiovascular Research .
ESC Professional Plus Members benefit from free online access and a print subscription to Cardiovascular Research .
Become an ESC Professional Member
Subscriptions
Connect with us on x.
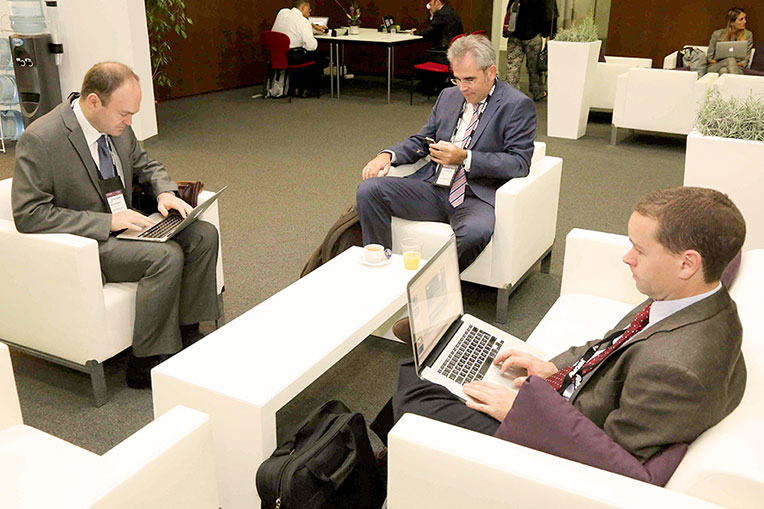
Updates on latest articles
Receive the table of contents in your mailbox.
Track topics and authors important to you and receive articles in your mailbox.
For Authors
Before being published, all manuscripts go through double-blind, peer review by experienced international experts.
Submit a Manuscript
Instructions for authors, publisher information, frequently-asked questions.
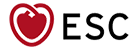
- ESC Board and Committees
- ESC Policies
- Statutes & Reports
- ESC Press Office
- Press Releases
- ESC Congress
- ESC Cardio Talk
- Our Offices
- Conference Facilities
- Jobs in Cardiology
- Terms & Conditions
- Update your cookie settings
Need help?
Help centre Contact us
© 2024 European Society of Cardiology. All rights reserved.
Progress in cardiac research: from rebooting cardiac regeneration to a complete cell atlas of the heart
Affiliations.
- 1 The Hatter Cardiovascular Institute, University College London, 67 Chenies Mews, London WC1E 6HX, UK.
- 2 Cardiovascular Program ICCC, Institut de Recerca de l'Hospital Santa Creu i Sant Pau-IIB Sant Pau, Barcelona, Spain.
- 3 CIBER Enfermedades Cardiovasculares (CIBERCV), Instituto de Salud Carlos III (ISCIII), Madrid, Spain.
- 4 Department of Experimental Medicine (DIMES), University of Genova, Genova, Italy.
- 5 Division of Experimental Cardiology, Department of Cardiology, Thoraxcenter, Erasmus University Medical Center, Rotterdam, The Netherlands.
- 6 Department of Infection, Immunity and Cardiovascular Disease and Insigneo Institute, University of Sheffield, Sheffield, UK.
- 7 British Heart Foundation Centre for Cardiovascular Research, Institute of Cardiovascular and Medical Sciences, University of Glasgow, Glasgow, UK.
- 8 Department of Medicine, Jagiellonian University, Collegium Medicum, Krakow, Poland.
- 9 Central Diagnostic Laboratory, University Medical Center Utrecht, Utrecht, The Netherlands.
- 10 Department of Cardiovascular Medicine, Medical Faculty, University of Muenster, Muenster, Germany.
- 11 Department of Internal Medicine II, Medical University of Vienna, Vienna, Austria.
- 12 Institute for Cardiovascular Prevention (IPEK), LMU Munich, DZHK (German Centre for Cardiovascular Research), partner site Munich Heart Alliance, and Munich Cluster for Systems Neurology (SyNergy), Munich, Germany.
- 13 Department of Biochemistry, Cardiovascular Research Institute Maastricht (CARIM), Maastricht University, Maastricht, The Netherlands.
- PMID: 34114614
- PMCID: PMC8344830
- DOI: 10.1093/cvr/cvab200
We review some of the important discoveries and advances made in basic and translational cardiac research in 2020. For example, in the field of myocardial infarction (MI), new aspects of autophagy and the importance of eosinophils were described. Novel approaches, such as a glycocalyx mimetic, were used to improve cardiac recovery following MI. The strategy of 3D bio-printing was shown to allow the fabrication of a chambered cardiac organoid. The benefit of combining tissue engineering with paracrine therapy to heal injured myocardium is discussed. We highlight the importance of cell-to-cell communication, in particular, the relevance of extracellular vesicles, such as exosomes, which transport proteins, lipids, non-coding RNAs, and mRNAs and actively contribute to angiogenesis and myocardial regeneration. In this rapidly growing field, new strategies were developed to stimulate the release of reparative exosomes in ischaemic myocardium. Single-cell sequencing technology is causing a revolution in the study of transcriptional expression at cellular resolution, revealing unanticipated heterogeneity within cardiomyocytes, pericytes and fibroblasts, and revealing a unique subpopulation of cardiac fibroblasts. Several studies demonstrated that exosome- and non-coding RNA-mediated approaches can enhance human induced pluripotent stem cell (iPSC) viability and differentiation into mature cardiomyocytes. Important details of the mitochondrial Ca2+ uniporter and its relevance were elucidated. Novel aspects of cancer therapeutic-induced cardiotoxicity were described, such as the novel circular RNA circITCH, which may lead to novel treatments. Finally, we provide some insights into the effects of SARS-CoV-2 on the heart.
Keywords: COVID-19; Cardiac; Cardiomyocyte division; Cardiotoxicity; Heart failure; Induce pluripotent stem cells; Myocardial infarction; Myocardial injury; Non-coding RNA; Single-cell RNA sequencing s.
Published on behalf of the European Society of Cardiology. All rights reserved. © The Author(s) 2021. For permissions, please email: [email protected].
Publication types
- Research Support, Non-U.S. Gov't
- Biomedical Research*
- COVID-19 / pathology
- COVID-19 / virology
- Cardiology*
- Cell Communication
- Cell Proliferation*
- Cellular Microenvironment
- Exosomes / metabolism
- Exosomes / pathology
- Heart Failure / metabolism
- Heart Failure / pathology*
- Heart Failure / physiopathology
- Mitochondria, Heart / metabolism
- Mitochondria, Heart / pathology
- Myocardial Infarction / metabolism
- Myocardial Infarction / pathology*
- Myocardial Infarction / physiopathology
- Myocardial Reperfusion Injury / metabolism
- Myocardial Reperfusion Injury / pathology*
- Myocardial Reperfusion Injury / physiopathology
- Myocytes, Cardiac / metabolism
- Myocytes, Cardiac / pathology*
- Myocytes, Cardiac / virology
- RNA, Untranslated / metabolism
- Regeneration*
- SARS-CoV-2 / pathogenicity
- RNA, Untranslated
Grants and funding
- PGC2018-094025-B-I00/Spanish Ministry of Science and Innovation
- CoG-2016-InflammaTENSION/ERC_/European Research Council/International
- RG/19/10/34506/BHF_/British Heart Foundation/United Kingdom
- CVON2014-RECONNECT/Netherlands CardioVascular Research Initiative financially supported by the Dutch Heart Foundation
- FEDER 'Una Manera de Hacer Europa
- Hatter Cardiovascular Institute
- PI19/01687/Spanish Ministry of Health-Institute of Health Carlos III
- PG/18/44/33790/BHF_/British Heart Foundation/United Kingdom
- University of Genova
Featured News
- CRF Announces 2024 SET-10 Rankings
- MedTech Innovation Takes Center Stage at TCT 2024
- CRF Announces TCT 2024 Late-Breaking Clinical Trials and Science
- New York Valves 2024 Late-Breaking Clinical Trials and Science Announced
- TCT 2024 Master Operator Award to Be Presented to Jeffrey W. Moses, MD
- Skirball Center for Innovation
- Investigate
- Clinical Trials Center
- Center for Education

We help doctors improve survival and quality of life for people suffering from heart and vascular disease.

INVESTIGATE

IMPROVED OUTCOMES
Our model to defy heart disease
We work to identify, develop and promote the latest advancements in the treatment of heart and vascular disease. By covering all aspects of the research continuum, we’re able to accelerate the speed with which breakthrough therapies reach patients through doctors, improving survival rates and quality of life, faster.
Drug-releasing Stents »
Disappearing stents ».

Your gift goes to help save and improve the lives of people living with heart and vascular disease. Find out more or:
For naming opportunities or to support CRF’s mission contact [email protected].
- The Problem
- Board of Directors
- Management Team
- Drug-eluting stents
- News Archive
- Events (Calendar)
- Recent Faculty Publications
- The Pulse of Progress Initiative
- Donate to WHHI
- Pulse of Progress Initiative Form
- Pulse of the City Gala

1700 Broadway, 9th Floor New York, NY 10019 646.434.4500
Please e-mail us if you would like more information: info@crf.org
Sign up for CRF Digest:
© 2024, Cardiovascular Research Foundation ® . All rights reserved.
Privacy Policy Terms and Conditions of Use Manage Cookie Settings
- Meetings Calendar
- TCT ® Conference
- TCTMD ®
- CRF ® Corporate
- MD | PhD Program
- Master's Programs
- PhD Programs
- Postdoctoral Fellows
- Residency & Fellowship
- Non-Degree Programs
- Visiting Students
- Campus Life at U-M
- Health & Wellness
- Building Your Community
- Accessibility & Disability
- Departments
- Centers & Institutes
- Interdisciplinary Programs
- Facts & Figures
- Medical School Leadership
- News & Stories
- Requirements
- Interview Day
- Admissions Chats
- AAMC Michigan's 35 Answers
- AAMC Michigan's 10 Financial Aid Answers
- Admitted Students
- Overview & Highlights
- Patient Interaction
- Chief Concern
- Years 3 & 4
- Learning Informatics
- Training Sites
- Leadership Program
- Global Health & Disparities
- Healthcare Innovation
- Health Policy
- Medical Humanities
- Patient Safety & Quality Improvement
- Scientific Discovery
- Doctoring Course
- Evidence-Based Medicine
- Interprofessional Education
- DEIAJ Curriculum
- Language Opportunities
- Curriculum Diagrams
- Grading & Assessments
- Guideline Budget
- Loans & Eligibility
- Scholarships & Grants
- Documents & Forms
- Tuition Refund Policies
- Consumer Information
- Extra Help for Current Students
- Contact the Office of Financial Aid
- Profiles & Demographics
- Culinary Connections
- Students with Disabilities
- Health & Wellbeing
- Arts & Humanities
- Diversity & Health Equity
- Dual Degrees
- More Possibilities
- Commencement
- Available PhD Programs
- Academic & Social Events
- MSTP Fellows
- Application Process
- Application Requirements
- MD | PhD Curriculum
- Undergrad Summer Program
- Contact the MD | PhD Program
- Bioinformatics
- Biological Chemistry
- Cancer Biology
- Cell & Developmental Biology
- Cellular & Molecular Biology
- Genetics and Genomics
- Health Infrastructures & Learning Systems
- Microbiology & Immunology
- Molecular, Cellular & Developmental Biology
- Molecular & Cellular Pathology
- Molecular & Integrative Physiology
- Neuroscience
- Pharmacology
- Recruitment Events
- Interview Weekends
- Certificates & Dual Degrees
- Quantitative & Computational Biology Emphasis
- Training Grants
- Facilities & Resources
- Stipend & Benefits
- Professional Development
- Finding a Position
- Funding Your Postdoc
- Hiring Process
- Postdoc Preview
- International Postdocs
- ACGME Fellowships
- Non-Accredited Fellowships
- Postdoctoral Physician Scientist Training
- Salary & Benefits
- Prerequisites
- Visiting Residents & Fellows
- Application Overview & Requirements
- Tuition & Fees
- Timeline & Curriculum
- Information Sessions
- Program Details
- Undergrad Summer Research
- First Days Survival Guide
- Health Services
- Mental Health
- Health, Spirituality & Religion Program
- For Partners & Families
- Things to Do in Ann Arbor
- Getting Around
- Guiding Tools
- Graduate Medical Education
- Office of Continuing Medical Education
- Office of Faculty Affairs & Faculty Development
- Office of Graduate & Postdoctoral Studies
- Physician Scientist Education & Training
- Office of Medical Student Education
- Points of Blue

The commitment to excellence through collaboration, teamwork and innovation is the basis for success for the Frankel Cardiovascular Center research programs . Continuing efforts to support a culture of collaboration, encourage creative thinking, and maintain infrastructure for both basic science and clinical research allow the Frankel Cardiovascular Center to remain at the cutting edge of science and discovery.
T32 Training Programs
- The NIH Postdoctoral Cardiovascular Research Training Grant provides intensive research training experience in basic or translational cardiovascular research. The program is designed for postdoctoral MD's or PhD's in preparation for independent investigative careers related to cardiovascular disease.
- The NIH Predoctoral Training Program in Translational Cardiovascular Research and Entrepreneurship trains the next generation of PhD scientists seeking careers in cardiovascular research in the ethical development and application of research results from the level of the molecule to the bedside.
Key Basic Science & Translational Research Areas
- Cardiac Myocyte Biology
- Cardiovascular Genetics
- Tissue and Cell Regeneration
- Vascular and Thrombosis Biology
Key Clinical Research Areas
- Acute and Chronic Cardiovascular Diseases
- Aging and the Cardiovascular System
- Aortic Disease
- Cardiac Imaging
- Heart Valve Disease
- Health Services and Clinical Effectiveness
- Heart Failure and LVAD
- Hypertension and Pulmonary Hypertension
- Structural Heart Disease
- Thrombosis and DVT
Learn more about our clinical research
The Cardiovascular Health Improvement Project is a biorepository of DNA, plasma, serum, and aortic tissue samples as well as an extensive clinical database of medical and family history information.
The Cardiovascular Regeneration Core Laboratory generates patient-specific human induced pluripotent stem cells (hiPSCs) for basic science research purposes.
The Center for Advanced Models for Translational Sciences and Therapeutics strives to accelerate the “bench to bedside” process in biomedical research and drug development with the mission of developing advanced models for translational sciences and therapeutics as part of the University of Michigan Medical School.
At the Center for Arrhythmia Research , scientists and physicians from a variety of disciplines work together to develop new methods of diagnosing and treating cardiovascular diseases, with the primary goal of preventing premature cardiac death. Our scientists have made major advances in understanding the molecular and cellular basis for and the fundamental mechanisms of complex, life-threatening arrhythmias and sudden cardiac death.
The Michigan Biological Research Initiative on Sex Differences in Cardiovascular Disease (M-BRISC) aims to increase investigation designed to understand the mechanisms of cardiovascular disease that differentially affect women and factors underlying the health of women.
Michigan Biology of Cardiovascular Aging is a multidisciplinary science program focused on aging and cardiovascular disease that enhances collaborative research knowledge between established investigators, team members, and others whose work and interests closely relate.
Launched in 2011 and based in the U-M Office of the Vice President for Research, the Michigan–Israel Partnership for Research and Education (MIPRE) is a collaboration among researchers at the University of Michigan, the Technion-Israel Institute of Technology, and the Weizmann Institute of Science. The MIPRE's mission is to facilitate relationships across disciplines and across the globe to advance the most promising areas of medicine, science, and engineering.
MAP is an interdisciplinary program with over 20 faculty representatives from cardiac surgery, vascular surgery, interventional radiology, cardiac imaging, medical genetics, and cardiovascular medicine. MAP faculty are conducting innovative research across a wide spectrum, including FDA-regulated trials, NIH-funded trials, Department of Defense contracts, and investigator-initiated projects, all specific to aortic disease.
Abdel-Latif Lab
- Research in the Abdel-Latif lab focuses on elucidating the molecular mechanisms underlying heart failure and investigating the intricate involvement of the immune system in this process. (Ahmed Abdel-Latif, MD, PhD)
- The Brody lab is broadly focused on the molecular signals that underlie cardiac disease onset and progression. (Matthew Brody, PhD)
- Research in the Byrd lab focuses on improving the diagnosis and treatment of high blood pressure through the identification of novel biomarkers of mineralocorticoid receptor activation. (J. Brian Byrd, MD, MS)
Espinoza-Foneseca Lab
- The goal of the Espinoza-Fonseca group is to understand the fundamental molecular motions and interactions that are responsible for regulating calcium transport in muscle cells. (L. Michel Espinoza-Fonseca, PhD)
- The Ganesh lab studies the genetics of vascular diseases leading to phenotypes such as fibromuscular dysplasia, spontaneous coronary artery dissection, visceral artery aneurysms, and other typically non-aortic arterial aneurysms and dissections. (Santhi Ganesh, MD)
Goldstein Lab
- The Goldstein lab is interested in how aging impacts innate immunity, focusing on aging and respiratory viral infections and aging and vascular diseases. (Daniel Goldstein, MBBS)
- Research in the Hayek lab centers on the novel use of biomarkers for predictive and prevention-based diagnoses and disease treatment. (Salim Hayek, MD)
- The Kanthi lab investigates the mechanisms of dysregulated vascular and innate immune signals in thrombosis and inflammation. (Yogen Kanthi, MD)
Lawrence Lab
- The Lawrence lab studies the role of proteases and their inhibitors in health and disease, primarily focusing on the vascular biology of the CNS and disorders such as stroke; and on the development of peripheral vascular disease. (Daniel A. Lawrence, PhD)
Morrissey Lab
- The research conducted in the Morrissey lab focuses on understanding how cells regulate blood clotting in health and disease. (James H. Morrissey, PhD)
- The Willer lab focuses on the analysis of high-throughput genetic data to understand the biological basis of cardiovascular and metabolic diseases. (Cristen Willer, PhD)
We transform lives through bold discovery, compassionate care and innovative education.
- Diversity, Equity & Inclusion
- Find a Doctor
- Conditions & Treatments
- Patient & Visitor Guide
- Patient Portal
- Clinical Trials
- Research Labs
- Research Centers
- Cores and Resources
- Programs & Admissions
- Our Community
- Departments, Centers & Offices
- About the Medical School
Global Footer Secondary Navigation
Editor's Choice: JAMA+ AI Site to Inform Medicine and Public Health

Nicole D. Armstrong, PhD; Vinodh Srinivasasainagendra, MS; Amit Patki, MS; et al
Original Investigation | October 23, 2024
Just Published
- Microaxial Flow Pump Effects in Infarct-Related Cardiogenic Shock Nanna Louise Junker Udesen, MD, PhD; et al. Original Investigation free access Nanna Louise Junker Udesen, MD, PhD; et al. Editorial
- Utility of a Systolic Blood Pressure Polygenic Risk Score With Chlorthalidone Response Nicole D. Armstrong, PhD; et al. Original Investigation Nicole D. Armstrong, PhD; et al. Editor's Note
- Trends in Transcatheter Aortic Valve Replacement Outcomes Suzanne V. Arnold, MD, MHA; et al. Original Investigation Suzanne V. Arnold, MD, MHA; et al.
- Evidence for Mechanical Circulatory Support in Cardiogenic Shock Jason E. Bloom, MBBS; et al. Editorial free access Jason E. Bloom, MBBS; et al.
- Precision Medicine to Guide Blood Pressure Control Sadiya S. Khan, MD, MSc Editor's Note Sadiya S. Khan, MD, MSc
- Age-Stratified Risk Categories for Cardiovascular Disease Prevention Therapies Cian P. McCarthy, MB, BCh, BAO, SM; et al. Viewpoint Cian P. McCarthy, MB, BCh, BAO, SM; et al.
- Aortic Stenosis, Heart Failure, and Aortic Valve Replacement Siddhartha Mengi, MD; et al. Review has active quiz Siddhartha Mengi, MD; et al.
- Tombstone Pattern Electrocardiogram in a Young Woman Yun Chen, MD; et al. JAMA Cardiology Clinical Challenge has active quiz Yun Chen, MD; et al.
- Racial Disparities in Sports Cardiology Sheela Krishnan, MD; et al. Review has active quiz Sheela Krishnan, MD; et al.
- 49,010 Views Sex Differences in Blood Pressure Trajectories Over the Life Course
- 9,724 Views Estimated Long-Term Benefits of Finerenone in Heart Failure
- 9,129 Views Genetic Risk Factors Associated With Preeclampsia and Hypertensive Disorders of Pregnancy
- 8,259 Views Cardiac Myosin Inhibition in Heart Failure With Normal and Supranormal Ejection Fraction
- 6,160 Views Dual-Antiplatelet Therapy After Drug-Eluting Stenting in ACS
- 5,880 Views Aortic Stenosis, Heart Failure, and Aortic Valve Replacement
- 5,114 Views Amiodarone or Implantable Cardioverter-Defibrillator in Chagas Cardiomyopathy
- 4,105 Views Lipoprotein(a) and Long-Term Plaque Progression, Low-Density Plaque, and Pericoronary Inflammation
- 3,233 Views Leadless Ultrasound-Based Cardiac Resynchronization System in Heart Failure
- 2,630 Views Exploring the Link Between Genetic Predictors of Cardiovascular Disease and Psoriasis
- 165 Citations Clopidogrel Monotherapy After 1 to 2 Months of DAPT vs 12 Months of DAPT in Acute Coronary Syndrome
- 148 Citations TET2 -Driven Clonal Hematopoiesis and Response to Canakinumab
- 132 Citations Association of Myocarditis With BNT162b2 Vaccination in Children
- 124 Citations ApoB-Containing Lipoproteins and Risk of MI in Individuals With and Without Atherosclerosis
- 124 Citations SARS-CoV-2 Vaccination and Myocarditis in a Nordic Cohort Study of 23 Million Residents
- 114 Citations Lipoprotein(a) and its Significance in Cardiovascular Disease
- 103 Citations Treatment With Sacubitril/Valsartan in Patients With Advanced Heart Failure and Reduced Ejection Fraction
- 85 Citations Transthyretin Amyloid Cardiomyopathy in Patients With Heart Failure With Preserved Ejection Fraction
- 83 Citations Association Between HDL-C Levels and Adverse Cardiovascular Outcomes
- 83 Citations Early Mortality in Type A Acute Aortic Dissection
- Register for email alerts with links to free full-text articles
- Access PDFs of free articles
- Manage your interests
- Save searches and receive search alerts
Thank you for visiting nature.com. You are using a browser version with limited support for CSS. To obtain the best experience, we recommend you use a more up to date browser (or turn off compatibility mode in Internet Explorer). In the meantime, to ensure continued support, we are displaying the site without styles and JavaScript.
- View all journals
- Explore content
- About the journal
- Publish with us
- Sign up for alerts
- Published: 16 May 2022
A roadmap of strategies to support cardiovascular researchers: from policy to practice
- Niamh Chapman ORCID: orcid.org/0000-0001-6317-5594 1 na1 ,
- Emma E. Thomas 2 , 3 na1 ,
- Joanne T. M. Tan ORCID: orcid.org/0000-0003-1875-4882 4 , 5 ,
- Sally C. Inglis 6 ,
- Jason H. Y. Wu ORCID: orcid.org/0000-0003-2073-3562 7 ,
- Rachel E. Climie 1 , 8 , 9 ,
- Dean S. Picone 1 ,
- Lauren C. Blekkenhorst 10 , 11 , 12 ,
- Steven G. Wise 13 ,
- Katrina M. Mirabito Colafella 14 ,
- Anna C. Calkin ORCID: orcid.org/0000-0002-9861-0602 8 , 15 , 16 &
- Francine Z. Marques ORCID: orcid.org/0000-0003-4920-9991 8 , 17
Nature Reviews Cardiology volume 19 , pages 765–777 ( 2022 ) Cite this article
5641 Accesses
7 Citations
64 Altmetric
Metrics details
- Medical research
Cardiovascular disease remains the leading cause of death worldwide. Cardiovascular research has therefore never been more crucial. Cardiovascular researchers must be provided with a research environment that enables them to perform at their highest level, maximizing their opportunities to work effectively with key stakeholders to address this global issue. At present, cardiovascular researchers face a range of challenges and barriers, including a decline in funding, job insecurity and a lack of diversity at senior leadership levels. Indeed, many cardiovascular researchers, particularly women, have considered leaving the sector, highlighting a crucial need to develop strategies to support and retain researchers working in the cardiovascular field. In this Roadmap article, we present solutions to problems relevant to cardiovascular researchers worldwide that are broadly classified across three key areas: capacity building, research funding and fostering diversity and equity. This Roadmap provides opportunities for research institutions, as well as governments and funding bodies, to implement changes from policy to practice, to address the most important factors restricting the career progression of cardiovascular researchers.
Diversity is key to innovation, but the current system and culture of the cardiovascular research sector are driving researchers, particularly those from under-represented groups, out of the sector.
Our team conducted research to identify the key short-term, medium-term and long-term solutions to addressing issues faced by early-career and mid-career researchers, focusing on three key areas: capacity building, research funding and fostering diversity and equity.
Capacity building needs to support collaboration and team-based research, improve and incentivize mentorship, and provide training to cardiovascular researchers to develop management, financial and communication skills.
Research funding needs to be used to improve job security and the assessment of career disruptions and opportunities and to support early-career and mid-career cardiovascular researchers to prepare for leadership roles.
To improve diversity and equity in cardiovascular research, working parents, women and individuals from other under-represented groups need additional support.
Similar content being viewed by others
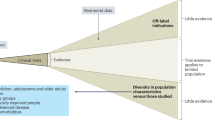
How to make cardiology clinical trials more inclusive
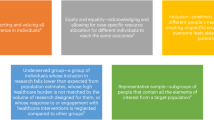
A toolkit for capturing a representative and equitable sample in health research
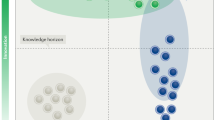
Key opinion leaders — a critical perspective
Introduction.
At present, cardiovascular disease (CVD) remains the main cause of morbidity and death worldwide 1 . In addition to new therapies, cost-effective policies and practices are urgently needed to prevent avoidable deaths from CVD. Research has shown that diversity in the workforce — which refers to involvement of individuals across the spectrum of gender, race or ethnicity, disability status, nationality, religious affiliation, sexual orientation and socioeconomic background in the workplace — drives innovation and performance 2 . Therefore, a diverse and stable cardiovascular research workforce is essential to identify solutions to tackle the global CVD burden. This diversity is particularly important to ensure that the solutions identified address the issues faced by the vast majority of the population, particularly those who are currently under-served by the existing health-care system 3 , 4 .
Some progress has been made in the past decade in improving diversity and equity in the sector, with several countries implementing national programmes to address these issues in research and academia. However, some evidence suggests that the cardiovascular research workforce is at imminent risk from low staff retention. In a 2019 survey involving >500 cardiovascular researchers in Australia, two-thirds of the participants had considered leaving the cardiovascular research sector or did not feel that they had long-term career prospects in cardiovascular research owing to the lack of long-term job security and funding 5 . Worryingly, these and other issues were twice as prevalent in women than in men 5 . Indirect evidence from other countries demonstrates that Australian cardiovascular researchers are not alone 6 , 7 , 8 . Although these concerns might reflect a broader issue affecting the biomedical research sector as a whole, such issues seem to be exacerbated in the cardiovascular research field owing to insufficient funding for cardiovascular research and development in the USA and Europe 6 , 7 , particularly for discovery research 8 . Moreover, a shortage in the cardiology workforce has remained for nearly two decades 9 , and women continue to be under-represented among cardiologists 10 . On the basis of this information and data from other areas of research 11 , 12 , these workforce challenges are likely to be occurring elsewhere in the world. Such challenges have been exacerbated by the coronavirus disease 2019 (COVID-19) pandemic, with evidence that the pandemic is worsening the gender and race or ethnicity disparities among researchers 13 , 14 and is driving an overall phenomenon called ‘the great resignation’, particularly among mid-career employees 15 . Consequently, solutions to stop the ‘brain drain’ of cardiovascular researchers and to improve retention and increase diversity are immediately needed.
To address this issue and identify potential solutions, focus groups involving 34 Australia-based early-career and mid-career researchers (EMCRs) working in cardiovascular research were formed in 2021 (ref. 16 ). These researchers were involved in different fields of cardiovascular research and represented different ethnicities, genders and LGBTIQA+ (lesbian, gay, bisexual, transgender, gender diverse, intersex, queer, asexual or questioning) identities, as well as differing levels of caregiving responsibilities. These focus groups resulted in 92 proposed solutions across three key areas: capacity building, research funding, and fostering of diversity and equity 17 (Table 1 ). By undertaking a review of the international literature, we have extended these solutions with practical examples from the global community. In this Roadmap article, we present short-term, medium-term and long-term strategies that could be implemented at an individual, organizational and sector level, from policy to practice, to support the careers of cardiovascular researchers worldwide (Fig. 1 ).
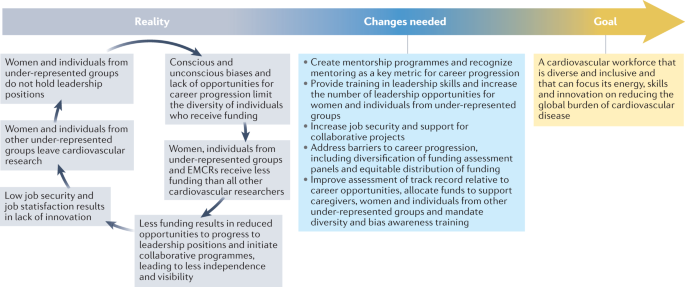
The figure provides a summary of the current reality and the future changes needed to support early-career and mid-career researchers (EMCRs) in the cardiovascular sector, with a focus on individuals from under-represented groups, including women and individuals from ethnic and other minorities.
Roadmap for capacity building
Reducing the burden of CVD globally in the short-term (<1 year), medium-term (1–5 years) and long-term (>5 years) requires a strong and secure pipeline of biomedical, clinical and public health researchers. Therefore, targeted investment in the career development of EMCRs is key to capacity building. In this section, we highlight strategies for building capacity with a specific focus on supporting collaborations, mentorship and training. Although the resources available to support and improve the career development of EMCRs might differ between low-income, middle-income and high-income countries 16 , 18 , many of the strategies suggested can be implemented without cost or are low cost and can be facilitated through local and international networks and societies.
Support multidisciplinary collaborations
Short-term strategies.
EMCRs could be supported to organize and attend regular meetings where clinical, biomedical and public health researchers can interact and share ideas. This approach would inform the direction of research and might lead to new research interests and collaborative projects. EMCRs could promote their research interests and skillset and identify potential collaborations through online platforms that allow cardiovascular researchers to connect and share ideas. For example, the Trainee Advocacy Committee of the American Heart Association (AHA) Council on Hypertension comprises EMCRs who contribute to all aspects of the organization of their annual scientific meeting, allowing them the opportunity to collaborate with researchers across different cardiovascular fields and levels of seniority 19 . Another example is the Global Cardiovascular Research Funders Forum, founded by 11 cardiovascular research bodies from Australia, Canada, Europe, New Zealand and the USA 20 . The forum aims to accelerate the pace of research progress by creating opportunities for cross-border coordination and collaboration between world-leading cardiovascular researchers and organizations to advance the prevention, diagnosis and treatment of CVD. Similar forums could be conducted at national and international levels through professional societies and organizations and could operate on an opt-in basis for EMCRs.
Medium-term strategies
EMCR-specific seed funding is invaluable in establishing job independence and collaborative research. Existing funding schemes could be redesigned to promote cross-disciplinary collaborations, support EMCR-only or EMCR-majority teams, encourage clinician–scientist collaborations, and stipulate mandatory inclusion of an EMCR as part of the principal investigator team or even as a co-lead investigator. Although some grant schemes focus on funding EMCRs, such as the NIH Pathway to Independence Award (K99/R00) programme 21 and the European Research Council starting grants (for early-career researchers) and consolidator grants (for mid-career researchers), these funding schemes target only individual researchers, rather than promote collaborations or team-based research. An innovative training programme called MINDSHIFT , funded by the European Commission for the years 2021–2026, aims to promote an interdisciplinary research programme across Europe to bridge knowledge gaps in hypertension research. This funding model could be adapted elsewhere in the world to improve collaborative efforts. Another important aspect of cardiovascular research is engagement with patients, who are experts by experience and can improve the relevance and applicability of research 22 , 23 . Facilitating connections between patients and EMCRs would increase awareness about the barriers faced by patients and their families, and cardiovascular researchers, and improve study design and effectiveness.
Long-term strategies
The development of infrastructure that facilitates the co-location of clinicians and non-clinician biomedical researchers might promote further cross-pollination of research interests and perspectives (Box 1 ). Capacity-building efforts should include collaboration between researchers at all levels of seniority, funding bodies and stakeholders (patients, programme organizers and policymakers) at the local, state, national and international levels 18 . The changes needed to reduce the burden of CVD require sustained awareness and advocacy among all stakeholders 24 . One example of an effective and broad collaborative effort is the regional cardiovascular research networks across Australia, such as the New South Wales Cardiovascular Research Network (Box 2 ). Moreover, the stagnation of cardiovascular drug development over the past two decades is a well-known issue 25 , which could be at least partially addressed by stronger partnerships between academia and industry. Such collaborations have already been established in other fields in the UK 26 , with Novo Nordisk investing up to £115 million in a new research centre at the University of Oxford for the development of innovative approaches to the treatment of type 2 diabetes mellitus 27 , and AstraZeneca locating one of its global research and development centres at the University of Cambridge 28 . In addition, India has approved its first industry–academia partnership programme (the Biopharma Mission) to accelerate discovery research and facilitate the development of products, including vaccines and medical devices 29 . The Biopharma Mission aims to improve technology transfer capabilities in the public and private sectors and to support start-up companies and small-sized and medium-sized enterprises to build the capacity for sharing innovative research 29 . Similar partnerships in the cardiovascular research sector would generate new opportunities for EMCRs, including more industry-funded fellowships.
Box 1 Building collaborations to maximize discovery and translation
Researchers and clinicians tend to have distinct but complementary perspectives, priorities and approaches to solving the same problem 84 . For example, a discovery researcher might want to know how or why a phenomenon is occurring, whereas a clinician might be more concerned about how the research findings will affect patient care. Breaking down research silos and bringing together stakeholders across the cardiovascular sectors to solve the same problem will maximize new discoveries and expedite translation of basic research into the clinic. Furthermore, clinical connectedness is likely to reduce researcher tunnel vision (that is, the tendency for researchers to focus on a research question that might not have clinical relevance), improve scientific rigour and reproducibility, and facilitate translation and reverse translation of research (whereby clinical needs and observations drive the focus of basic or discovery research) to ensure that the key questions being faced in the clinic today are the same questions being addressed in the laboratory.
Effective collaborative relationships take years to cultivate. Therefore, early-career and mid-career researchers should be encouraged to establish these connections early in their careers. Institutions and professional networks and societies can foster the development of these relationships by creating opportunities that bring together trainees from across the cardiovascular sectors, priming them for collaborations and providing incentives to do so (such as through small grants or seed funding). Such initiatives are especially important for institutions in which departments are situated across multiple floors or locations. In general, more focus and institutional support must be given to attract clinicians to engage in research. In certain countries, such as the Netherlands, the medical system is very supportive of those who hold dual degrees (such as clinician–researchers), and completion of a PhD is almost a necessary step to enter specialist training programmes, whereas in other parts of the world, such as in Australia, those who hold dual degrees are rare. Since clinicians tend to have their schedules dictated by their clinical duties, incentives must be given to encourage their participation in research 85 . Supervisors and mentors can further drive collaborative projects by involving junior faculty members in their existing collaborations.
Box 2 A case study for best practice for funding assessment and distribution
New South Wales (NSW), Australia’s most populous state, is leading the way in cardiovascular research funding after the state government established a Australian $150 million programme to invest in cardiovascular research over 10 years. Building on the work of the NSW Cardiovascular Research Network, a collaborative network of 13 umbrella member organizations and >50 affiliated institutions supporting cardiovascular research in the state, the Cardiovascular Research Capacity Program (CRCP) was established in 2018 (ref. 86 ). The injection of resources into a strained Australian funding system was most welcome.
Grants awarded in the first 3 years of the programme have been highly targeted, with categorized funding for senior researchers, clinical researchers and early-career and mid-career researchers (EMCRs), which was further stratified by years post-PhD: 0–2, 2–8 and 8–15 years post-PhD, and designed to build capacity across the sector. The grants last for 3 years and range between Australian $450,000 and $750,000, depending on seniority. For senior researchers, this grant is an extension of established programmes and protection of research time for clinicians. However, the greatest benefit of this initiative has been for EMCRs to begin a new project or to build further research independence, which will lead to improved job security and increased competitiveness for tenure track positions and will provide a unique opportunity for leadership. Of note, NSW Health had a clear strategy to award the funding to individuals of diverse backgrounds, by explicitly encouraging applications from clinician–researchers, researchers from culturally and linguistically diverse backgrounds, Aboriginal and Torres Strait Islander researchers, and primary caregivers who have experienced career disruptions. Review panellists were varied in level of seniority and research field, and a mixed-effects regression model was used to account for variability in reviewer scoring. Grant applicants need to demonstrate a pathway to translation (grants are split 60:40 for basic and clinical sciences), and previously successful applicants are excluded from applying to better distribute resources. The emphasis for funded applications so far has been on novelty, building research capacity and the potential benefit for consumers. Overall, the CRCP is a promising example of how a well-resourced programme with capacity building and diversity at its core can benefit EMCRs. After independent and rigorous evaluation for outcomes in the near future, this programme could be adopted more widely to solve some of the most pressing issues affecting the cardiovascular research sector.
Incentivize mentorship and sponsorship
Effective mentorship is a key factor in the development, success and retention of EMCRs in research settings 30 , 31 . At present, more investment in mentorship and sponsorship is needed to support career advancement for women and other under-represented groups 32 , 33 . Academic sponsors who advocate for the career development of EMCRs are crucial, particularly to bridge the equity gap by increasing the number of cardiovascular researchers from under-represented groups to be promoted to senior leadership positions 33 , 34 .
Cardiovascular institutions, networks and societies could establish small peer-mentoring groups made up of EMCRs who can provide support for each other. Importantly, all EMCRs should be encouraged to join mentoring programmes at institutional, national and international levels, such as the AHA Mentoring for Professionals 35 scheme and the International Society of Hypertension (ISH) Mentorship programme 36 . The MINDSHIFT programme also partners researchers with at least two independent research institutions across Europe to increase mentoring opportunities. Moreover, cross-sector mentoring programmes can provide opportunities to facilitate translation of basic science research to the clinic. For example, the Australian Cardiovascular Alliance offers a cross-sector mentoring programme that connects biomedical scientists, clinical researchers, health services and public health researchers, clinicians, policymakers and industry leaders 37 . EMCR mentees and mentors should undergo formal mentorship training to learn how to navigate and gain the most value from the mentor–mentee relationship. In particular, mentors should be provided with training to increase their awareness of diversity. At present, formal mentorship training programmes for academic researchers are scarce, particularly in low-income and middle-income countries (LMICs) 31 , 38 . A notable example is the Fogarty Global Health Program for Fellows and Scholars, which includes a mentorship workshop to train mid-level and senior-level investigators working in public health and clinical and basic science research across multiple academic institutions in LMICs 31 .
To encourage involvement from supervisors and senior mentors, funding bodies could build mentorship requirements into grant applications, whereby senior applicants would be required to describe how they will build research capacity and mentor EMCRs within and, importantly, across research groups. Additionally, institutions could provide career coaching and secondment opportunities to develop and improve mentoring skills. Career coaching, as distinct from mentorship, is designed to help individuals to set and reach personal goals and is advantageous for career planning and leadership training.
Periodic evaluation of mentoring and sponsorship schemes is crucial to ensure that these programmes are effective and are having a positive effect on the career development of cardiovascular researchers. Furthermore, a robust review of mentoring programmes encourages institutional leadership support and creates local ownership of the initiative 29 . In this regard, a six-point framework to review and evaluate mentorship programmes has been proposed, which encompasses aspects of the mentor–mentee relationship, career guidance, academic productivity, networking, wellness and organizational capacity 39 .
Provide training to improve professional skills
Early in their career, EMCRs are trained to become good scientists who are proficient at collecting, analysing and discussing their research. As EMCRs transition from postdoctoral fellows to independent research leaders, they are suddenly faced with a myriad of new challenges with little-to-no formal training. These new challenges can include recruitment and management of staff, finance administration, science communication and the balancing of clinical or teaching loads with research, while simultaneously trying to build their research programmes and secure research funding to establish themselves as leaders in their respective fields. Indeed, results from a survey of 3,200 scientists revealed that two-thirds of laboratory heads had not received any training on the managing and mentoring of staff and students 40 . The training and development of EMCRs, in both a research and a professional capacity, are crucial to provide a strong foundation on which to build an independent and sustainable research career in cardiovascular research.
Areas of training for EMCRs could include communication, leadership, and financial and project management. Such training programmes can improve general skills and are helpful in building a people-centred research culture. Training programmes should also extend beyond intra-institutional and inter-institutional opportunities to include local, regional and national agencies. For example, a training initiative for early-career researchers from LMICs that was developed by the National Heart, Lung, and Blood Institute–UnitedHealth Collaborating Centers of Excellence includes training in project management 21 , 41 , 42 . In addition, the World Heart Federation Salim Yusuf Emerging Leaders Programme provides professional development and mentoring opportunities alongside research capacity building activities 43 . However, these programmes are usually extremely competitive, with only a small number of researchers who can participate. Advocacy and support of these training programmes for a wider range of EMCRs are vital and should be prioritized by institutions to ensure they remain sustainable. Many societies and organizations offer training webinars involving established researchers who share their experience. The design of such sessions to have a clear learning outcome focused on professional development would provide accessible training that is currently not widely available to cardiovascular researchers.
Institutions should consider offering specific training to early-career researchers to support their transition from a PhD graduate to a postdoctoral scientist, and from postdoctoral to leadership positions. The responsibilities of postdoctoral fellows differ between countries, but commonly include tasks such as finance management, grant administration and staff recruitment, which are often expected of these researchers without provision of additional training. Institutions should also be encouraged to integrate professional skills training into Masters and PhD programmes to fully equip graduates with the skillset required as they transition into their next academic or non-academic role, or as part of an on-boarding programme during postdoctoral training.
Instituting training of professional skills across the short-term and medium-term, as described above, should help to build leadership capacity in EMCRs. This training should be made compulsory as part of the promotion process, so that future leaders are well equipped with the skills necessary to succeed in cardiovascular research and beyond. Of note, professional development and leadership training programmes must be well designed and have the backing of a strong and supportive institutional culture 44 . Therefore, the training should optimally be delivered by external parties or collaboratively between broad stakeholders and designed to suit local research environments.
Roadmap for research funding
The cardiovascular research sector is seeing lower success rates for competitive funding schemes than in previous decades, with overall less funding available for cardiovascular research and development 6 , 7 , 8 . Moreover, the average age of awardees is increasing. For example, in the USA, the average age at which a scientist secured their first NIH R01 grant increased from 40 years in 1995 to 44 years in 2020 (ref. 45 ). The distribution of NIH funding support is also highly skewed, with 10% of NIH-funded researchers receiving 40% of the total funding available 45 . This finding is particularly relevant given the reports suggesting that innovative research is driven by EMCRs 46 , who are disproportionately affected by lack of funding owing, in part, to biases against junior scientists 46 . To address these longstanding issues, the NIH launched an EMCR-specific biomedical scheme, the Next Generation Researchers Initiative 45 . Considering that cardiovascular research has one of the highest cost–benefit ratios of all biomedical research fields (Australian $9.8 return for every dollar invested in Australia 47 and a 20.6% internal rate of return in Canada 48 ), investing in funding that specifically benefits cardiovascular EMCRs should be an obvious choice. In this section, we discuss the major barriers faced by cardiovascular EMCRs in securing competitive funding and how these challenges can be overcome. An example of a well-designed and well-implemented funding scheme is described in Box 2 .
Increase job security
Many outstanding researchers with strong track records and notable contributions to health and medical research are appointed on short-term (6-month to 18-month) contracts 5 . This situation has evolved owing to many complex, interrelated factors, such as short-term research funding, and will not be improved quickly or without strong leadership and advocacy, underscoring the societal and economic cost of the lack of support for cardiovascular research to address our CVD burden.
EMCRs should be provided with access to objective and critical career development advice to increase awareness of different career pathways, including industry roles and entrepreneurship.
Mid-career researchers have a specific set of career challenges; they are expected to compete with established senior researchers for grant funding, while having a large administrative burden as they try to maintain research independence and acquire international recognition 49 . In this regard, funding schemes have been developed to specifically cater to mid-career researchers, such as the NIH Next Generation Researchers Initiative 45 and the European Research Council consolidator grant. The development of strong connections and formal collaborations with industry and policymakers is essential for the continuity of the cardiovascular research pipeline, including opportunities to develop co-funded, longer-term postdoctoral roles between research institutions, industry and the government. Such conjoint roles are essential in improving management and policy and to shift biomedical discoveries along the translational pipeline. In addition, little support exists for a team-based approach to research for mid-career researchers who are willing to contribute to a research programme, but are not necessarily ready to lead a research team. Grant schemes could be improved by better recognizing the team-based approach that is required to conduct high-quality research, rather than recognizing only an individual lead investigator.
Funding agencies can contribute to improving job security for researchers by requiring research institutions to set a minimum duration of employment contracts for research staff. Employers of grant recipients should also be contractually obliged to ensure that a predefined number of staff employed through the grant are provided with an employment contract that aligns with or extends beyond the term of the grant. Longer-term goals such as an extension in the research project, dissemination of results, applications for the next stage of research funding and investment in the translation or implementation of findings are all contingent on the researcher’s job security. Moreover, creating long-term research opportunities for professional researchers who provide essential training and increase the overall capacity of institutions would allow further employment opportunities while retaining expertise. For example, in France, a tenured research position with INSERM (the National Institute of Health and Medical Research) is possible by way of a nationally competitive examination. A nationally relevant set of expectations or minimum standards to secure ongoing employment would dramatically shift the research culture towards innovation that comes with job security. Finally, in a post-COVID-19 world, with new and re-imagined workplace practices and a greater understanding of career opportunities and disruptions, researchers who want to work part-time should be treated equitably with regard to expectations on output and workload compared with those in equivalent full-time positions.
Improve assessment of career opportunity
Australia’s major medical research funding body, the National Health and Medical Research Council (NHMRC), introduced the policy ‘relative to opportunity’ in recognition of the fact that over a given period of time, researchers might not have equitable opportunities to advance their career. This term is used to acknowledge that career breaks, carer responsibilities, stage of career, time dedicated to research and access to resources can all have an effect on scientific track record and productivity. Therefore, consideration of a researcher’s achievements relative to opportunity should take into account the differences in career progression and opportunity owing to differences in life circumstances. However, no formal infrastructure exists to guide peer-reviewers in the assessment of achievements that are relative to opportunity within funding applications. The solutions below describe how such a relative-to-opportunity policy could be fairly implemented and assessed, offering valuable guidance for other countries.
Peer-reviewers, promotion panels and institution leaders involved in the grant review process should receive training on how to assess the effect of relative opportunity and other factors contributing to scientific track record; this training needs to be developed, implemented and legislated. Just as a Good Clinical Practice certification is required to conduct clinical research, or a higher degree in research supervision training is required before being able to oversee doctoral students, grant reviewer training or certification should become compulsory for peer-reviewers involved in the assessment of funding applications and for all institutional leaders. National and international research organizations should provide leadership to develop and implement consistent cross-sector training and certification programmes, providing one course that is relevant to all funding bodies in the country.
Relative-to-opportunity considerations should involve a formalized, documented and standardized process during the application for grants, employment, promotion or leadership opportunities that are offered by research institutions and funding agencies. For applicants, this process could include mention of career disruptions and other factors affecting relative opportunity. At the peer-review stage, a standardized protocol should be developed to acknowledge that relative-to-opportunity considerations have been examined and considered for each applicant.
Longer-term strategies
Relative-to-opportunity considerations can be quantified by quartiles or tertiles on the basis of full-time-equivalent research time, with peer-review scores weighted accordingly. The allocation of additional funding to support near-miss funding applications from researchers who have experienced substantial career disruption and relative-to-opportunity barriers might also address existing inequalities in funding distribution.
EMCR leadership opportunities
Leadership in the research sector can be formal (such as on committees and boards or in institutional leadership positions) or less formal (as in grant and manuscript preparation in team and group roles). In this section, we describe how current cardiovascular research leaders can create opportunities for future leaders.
Access to opportunities for leadership roles are often reliant on supportive senior researchers and personal factors such as confidence, skills and capacity. However, many EMCRs struggle during the transition to a lead investigator role 50 . Measurable performance expectations need to be established to enable senior leaders to provide opportunities for the next generation of cardiovascular research leaders, such as co-supervision of students and a co-leadership role in preparing research grants or papers, with proper acknowledgement of the contribution by the EMCR (such as co-senior or co-corresponding authorship). These achievements have only a marginal value for the track record of senior leaders, but have an enormous effect on the career development of EMCRs.
Given the fiscal pressures on research funding, grants should be scaled to ensure EMCRs are encouraged and supported to be named as principal investigators or co-principal investigators in research projects. Indeed, funding agencies need policies that specify mandatory inclusion of EMCRs in grants.
Any instances of senior research leaders promoting EMCRs to research leadership positions, primarily through research funding opportunities, should be formally acknowledged through prestigious awards for research mentoring, such as those from the ISH 51 . Moreover, we propose that mentorship of more junior staff, particularly EMCRs, should be included as key performance indicators in annual and promotion reviews of senior cardiovascular researchers.
Diversify funding evaluation
Many high-income countries such as France 52 , Germany 53 and the USA 54 have announced boosts to their research budget in the next 5–10 years. However, research funding continues to be limited in most regions of the world, particularly for the cardiovascular sector 6 , 7 , 8 , and remains a major reason why cardiovascular researchers leave the sector 5 . Therefore, ensuring fair distribution of funding is crucial for retention of expertise.
To minimize the effect of unconscious bias and ensure alignment with funding priorities, peer-review panels need to be gender-balanced and include patients and carers, and industry-based experts, as well as researchers from different disciplines and ethnicities, career stage and backgrounds (including those from under-represented groups, such as indigenous persons, and culturally and linguistically diverse individuals). In addition, where possible, applications should be de-identified to reduce bias.
Ideas-based funding, whereby the applicant’s track record is peer-reviewed only to assess feasibility and not to rank applications, might help to overcome some of the biases experienced by women and individuals from other under-represented groups. Moreover, the outcomes of these funding schemes should be carefully reviewed to ensure they result in knowledge gain and are indeed distributed equitably. The NHMRC in Australia found that although the percentages of men and women awarded ideas-based funding in 2019–2021 were similar, female principal investigators received a total of Australian $44 million less per year than male counterparts 55 . Data on other under-represented groups or specific to the cardiovascular field are not available. With success rates in funding schemes as low as 5–15% in many instances (for the years 2019–2021), careful review of the value proposition to researchers of applying for several grants throughout the year needs to be considered. Streamlining the grant process and consolidating funding requirements across different funding schemes will improve efficiency. For example, shorter preliminary applications that are peer-reviewed, in which only a proportion (30–50%) of candidates are invited to submit a full application, might also improve efficiency. Furthermore, by staggering funding rounds throughout the year and restricting eligibility to those who have not already received funding in that year will result in more equitable distribution of research funding (Box 2 ).
Although national research priorities are often mentioned in calls for research funding applications, to what extent funding is awarded on the basis of alignment with research priorities, disease burden, contribution to health outcomes or delivery of care is unclear. For example, a 2021 international analysis of support for nursing and allied health research indicates that in the UK, nurses, midwives and allied health professionals account for more than two-thirds of the health-care workforce, yet only 1%, <1% and 4% of trainees from these professions, respectively, are supported by the National Institute of Health Research 56 . Similarly, in Australia, only 0.4% of NHMRC fellowships in 2020 were awarded to midwifery, 3% to nursing and 7% to allied health departments, despite these professions representing 80% of the registered health practitioner workforce 56 . Lottery-style funding programmes, especially for ideas-based grants, might be appropriate in settings with limited research funding to target applications that are deemed by peer-review to be worthy of funding, despite failure to secure funding. This approach has been adopted by the Health Research Council of New Zealand 57 . Although perhaps a controversial idea, if all research proposals and their feasibility are peer-reviewed and deemed fundable or not fundable, all those deemed fundable should have an equal chance of being funded, irrespective of other factors that might bias the ranking of funding applications. After a trial period, the outcomes of these funding schemes should be assessed to determine whether they deliver value, lead to knowledge gain and are distributed equitably.
Roadmap for diversity and equity
Equity is the acknowledgement that individuals with diverse circumstances require specific resources and opportunities to reach an equal outcome 58 . Growing evidence indicates that more diverse and equitable workplaces will achieve greater innovation, discovery and benefit 3 , 59 . Notably, historically excluded and marginalized groups continue to be under-represented and under-supported in cardiovascular research. Despite calls for change, the research sector still lacks leadership, policies and strategies to provide equitable access to opportunities. Cardiology is a male-dominated profession, and cardiovascular research, like many areas of academia, has disproportionately fewer women and individuals in ethnic minority groups in training fellowships, clinical roles and leadership positions 60 , 61 , 62 , 63 . Women are also under-represented in leadership roles, such as membership in cardiovascular guideline-writing committees 64 and cardiovascular clinical trial leadership committees 65 . Therefore, solutions to meaningfully tackle and reduce inequity and improve diversity are urgently needed. Some national programmes have been specifically designed to address this issue (Box 3 ), but the benefits imparted by these programmes remain to be seen. In this section, we present possible strategies and solutions to support diversity, equity and inclusivity in the cardiovascular research sector (Table 2 ).
Box 3 Addressing gender equity
A range of programmes implemented globally have been designed to address the lack of diversity and equity that exists in the science, technology, engineering, mathematics and medicine (STEMM) fields 66 . Although each programme has a unique framework, they all have the overarching goal of developing an equitable, diverse and inclusive STEMM sector in which all individuals can thrive. These programmes involve: self-reflection of STEMM workplace culture, policies and practice with regard to diversity, equity and inclusivity in a quantitative and qualitative manner; the development of an action plan to address inequities, barriers and knowledge gaps, with commitment to the principles of the respective charter; implementation of strategies to facilitate sustainable change; and an award system that is judged on the basis of commitment to change. Examples of these international programmes are listed below.
Athena Scientific Women’s Academic Network Charter
Established in 2005, the Athena Scientific Women’s Academic Network Charter initiative originally focused on advancing the careers of women in STEMM and has formed the basis of programmes internationally, with almost 1,000 institutional and departmental participants in the UK 87 . The effectiveness of the charter has been credited to both applicant accountability and the tools provided to implement change 88 .
Science in Australia Gender Equity
The Science in Australia Gender Equity programme is an initiative of the Australian Academy of Science and the Academy of Technology & Engineering that was launched in 2015 to pilot the Athena Swan Charter in Australia 89 . In 2020, 87% of universities, 12% of medical research institutions and 40% of publicly funded science research agencies had received an Athena Swan Bronze Award 90 .
STEMM Equity Achievement Change
The STEMM Equity Achievement Change is an initiative of the American Association for the Advancement of Science. The pilot programme was launched in 2018, with the first awards given in 2019 (ref. 91 ), and their mechanism for transformation is founded on three pillars: institute, community and awards.
In Canada, 17 institutions joined the Dimensions pilot programme that commenced in 2019. The programme aims to “foster increased research excellence, innovation and creativity within the post-secondary sectors across all disciplines, through greater equity, diversity and inclusion” 92 .
Support primary caregivers
Primary caregivers, both male and female, should be supported to maintain research engagement during parental and carer’s leave, upon return to work and for the long-term. Workplace policies should consider whether the timing of meetings and conferences is suitable for parents and carers and should optimally provide targeted support for parents and carers, such as financial support for childcare or an option of virtual meeting attendance. Practical support is required to allow the continuation of work during periods of leave, such as funds to employ a research assistant 17 , 66 . Parental leave, in particular, is a crucial period of high researcher attrition, with a survey of researchers in the USA revealing that 43% of new mothers and 23% of new fathers who were employed full-time before having children either did not return to academia or returned part-time after having children 67 .
To support the transition of parents and carers back to the workforce, flexibility is required in the duration of the secured grant funding period to allow for the time absent from research, as well as in allowing researchers to work part-time. Workplaces that provide such flexibility are more likely to retain employees with caregiving responsibilities 68 .
To shift the status quo, greater incentives are required to encourage more equitable sharing of parental leave, such as gender-neutral leave policies that encourage leave arrangements to be split between carers. Importantly, creating policies that increase opportunities for both men and women to combine paid employment with unpaid caring responsibilities can help to recalibrate gender roles 69 . Organizations that provide strong parental leave schemes have greater recruitment and retention of staff 70 . Sweden has been a long-standing leader in such progressive parental leave policies 71 . Further to these changes, an acknowledgement of the effect of carer responsibilities on career disruptions during grant funding reviews is also important.
Support women
Increasing the visibility of women and celebrating their research accomplishments are key in demonstrating to female EMCRs the possibility of a successful career as a female cardiovascular researcher. The Organization for Women in Science for the Developing World aims to increase the participation, opportunities for collaboration and leadership skills of women from LMICs 72 . Furthermore, the Future Professors Programme in South Africa focuses on supporting Black and female researchers to achieve professorial level by providing mentorship and skills training and fostering collaborative efforts 73 . Women-focused and structured mentoring programmes, such as those led by Franklin Women in Australia 74 , have been shown to improve the participants’ knowledge, skills and research metrics required for promotion. The framework of such initiatives could be implemented by cardiovascular research societies globally.
Grant and publication review processes need to be re-evaluated to minimize unconscious reviewer bias, such as by removing identifiable information from the applicants and authors (such as gender and region of origin) and through the use of gender-neutral language 17 . To extend this goal further, grant committees should aim to fund male-led and female-led projects equally and ensure that gender-specific data on grant success are collected and reported publicly. The South African government has developed projects, such as the Black Academics Advancement Programme 75 , to prioritize women and other under-represented groups in funding applications, resulting in the greater success of female-led initiatives across all career stages.
To unpick long-standing gender biases, group coaching and reverse mentoring (that is, when a female EMCR mentors a senior leader on the issues that EMCRs face) 76 are required to increase awareness of gender bias. Moreover, a study has shown that female faculty dedicate more time to service (such as service to the university, campus, or local and international communities) than male faculty 77 . Therefore, strategies should be in place to ensure service and social roles are distributed evenly across genders.
Support under-represented groups
In response to continued issues of racism in society, many institutions have mandated diversity and bias awareness training for all staff and students. Importantly, these training modules should not be undertaken as a one-off exercise, because evidence suggests that such modules result in limited behaviour change 78 . Therefore, training should be carefully designed and, if possible, undertaken at regular intervals, with outcomes assessed 79 . Scientific societies could also increase visibility of under-represented researchers, such as the Black in Cardio campaign, which celebrates exceptional Black cardiovascular researchers 80 , and Black in Physiology , which is associated with the American Physiological Society.
To address barriers to career progression and workplace participation for researchers from under-represented groups, grant schemes that fund discretionary items such as parental or carer support, visa fees or conference registration should be considered. Financial barriers associated with opportunities that are advantageous for career progression are often a limiting factor for those from low socioeconomic backgrounds and single-income households, as well as those with carer’s responsibilities.
Research produced by more ethnically diverse teams has been shown to have increased value compared with that from less ethnically diverse teams 59 . Given the globally connected nature of research, national funding bodies should consider funding schemes to attract and retain international talent. In Australia, most nationally competitive funding schemes will fund permanent residents or citizens, but not individuals on temporary work visas 17 . In addition, strategic partnerships with international institutions need to be carefully considered and developed to build capacity between and within high-income and low-income countries. Lastly, individuals with disabilities should be provided with a safe and accessible workplace.
Limitations
We acknowledge that several of the issues highlighted in this Roadmap article are not exclusive to the cardiovascular research sector. However, considering the reduced funding investment into cardiovascular research globally, these issues might be of increased relevance in this sector. The solutions proposed in this Roadmap article were identified by cardiovascular researchers from diverse backgrounds. Of note, however, these solutions originated in Australia and might not necessarily reflect the needs and views of cardiovascular researchers in LMICs and in some under-represented groups. To broaden the relevance of the solutions that we have proposed, we engaged with eight cardiovascular researchers who are a part of institutions in nine countries (see Acknowledgements). The researchers from high-income countries (Canada, Italy, New Zealand, the UK and the USA) agreed that our proposed roadmap accurately presents the major issues faced by EMCRs in their regions. Overall, those from LMICs (Brazil, India and South Africa) commented that although cardiovascular researchers from LMICs would benefit from the solutions proposed, these researchers also face other major issues specific to the region. In particular, they mention that inadequate research funding in their region limits conference participation and research publication and therefore does not support a sustainable research culture 81 . This issue might be addressed by increased international investment into local infrastructure and resources (such as local biobanks) and more international collaborations. Strategic and immediate solutions to support the inclusion of cardiovascular researchers from LMICs include free membership to professional societies, waived fees for online conferences and publications, training grants for doctoral and postdoctoral researchers, local mentoring programmes, and inclusion on editorial boards of journals and committees of professional societies. Given that LMICs are disproportionately affected by CVD 2 , inclusion of researchers from these regions in global research is key to reducing the global burden of CVD.
Conclusions
A diverse and collaborative cardiovascular research workforce is crucial to finding solutions to reduce the global burden of CVD. However, the current culture of cardiovascular research is not supportive of diversity and collaboration and often inhibits the career progression of EMCRs. These factors, together with the global downward trend in available funding for cardiovascular research, are driving researchers out of the sector. Immediate action needs to be taken to prevent the loss of an entire generation of cardiovascular researchers, particularly those from under-represented groups. The effect of this loss on the burden of CVD in the future will be substantial, after well-established research leaders retire without a strong and secure pipeline of EMCRs and succession planning. The proposed roadmap of changes in this article could improve the cardiovascular research culture and support EMCR career progression, allowing the cardiovascular research sector to thrive and, in turn, to reduce the burden of CVD globally.
Roth, G. A. et al. Global burden of cardiovascular diseases and risk factors, 1990–2019: update From the GBD 2019 Study. J. Am. Coll. Cardiol. 76 , 2982–3021 (2020).
Article PubMed PubMed Central Google Scholar
Hewlett, S. A., Marshall, M. & Sherbin, L. How diversity can drive innovation. Harvard Business Review https://hbr.org/2013/12/how-diversity-can-drive-innovation? (2013).
Schnabel, R. B. & Benjamin, E. J. Diversity 4.0 in the cardiovascular health-care workforce. Nat. Rev. Cardiol. 17 , 751–753 (2020).
Article CAS PubMed Google Scholar
Capers, Q. T., Johnson, A., Berlacher, K. & Douglas, P. S. The urgent and ongoing need for diversity, inclusion, and equity in the cardiology workforce in the United States. J. Am. Heart Assoc. 10 , e018893 (2021).
Climie, R. E. et al. Lack of strategic funding and long-term job security threaten to have profound effects on cardiovascular researcher retention in Australia. Heart Lung Circ. 29 , 1588–1595 (2020).
Garcia-Dorado, D. Insufficient cardiovascular research & development funding. Eur. Heart J. 38 , 10–11 (2017).
Article PubMed Google Scholar
Nicholls, M. Funding of cardiovascular research in the USA: Robert Califf and Peter Libby – speak about cardiovascular research funding in the United States and what the latest trends are with Mark Nicholls. Eur. Heart J. 39 , 3629–3631 (2018).
Nicholls, M. Funding cardiovascular research in Europe. Eur. Heart J. 40 , 80–82 (2019).
Fye, W. B. Cardiology’s workforce shortage: implications for patient care and research. Circulation 109 , 813–816 (2004).
Narang, A. et al. The supply and demand of the cardiovascular workforce: striking the right balance. J. Am. Coll. Cardiol. 68 , 1680–1689 (2016).
Milojevic, S., Radicchi, F. & Walsh, J. P. Changing demographics of scientific careers: the rise of the temporary workforce. Proc. Natl Acad. Sci. USA 115 , 12616–12623 (2018).
Article CAS PubMed PubMed Central Google Scholar
Wellcome Trust. What researchers think about the culture they work in (Wellcome, 2020).
Muric, G., Lerman, K. & Ferrara, E. Gender disparity in the authorship of biomedical research publications during the COVID-19 pandemic: retrospective observational study. J. Med. Internet Res. 23 , e2537 (2021).
Article Google Scholar
Staniscuaski, F. et al. Gender, race and parenthood impact academic productivity during the COVID-19 pandemic: from survey to action. Front. Psychol. 12 , 663252 (2021).
Cook, I. Who is driving the great resignation? Harvard Business Review https://hbr.org/2021/09/who-is-driving-the-great-resignation (2021).
Malekzadeh, A., Michels, K., Wolfman, C., Anand, N. & Sturke, R. Strengthening research capacity in LMICs to address the global NCD burden. Glob. Health Action. 13 , 1846904 (2020).
Thomas, E. E. et al. Strategies to support early- and mid-career cardiovascular researchers to thrive. Preprint at medRxiv https://doi.org/10.1101/2022.02.06.22270563 (2022).
Bloomfield, G. S. et al. Training and capacity building in LMIC for research in heart and lung diseases: the NHLBI–UnitedHealth Global Health Centers of Excellence program. Glob. Heart 11 , 17–25 (2016).
American Heart Association. Council hypertension trainee advocacy resources. Professional Heart Daily https://professional.heart.org/en/partners/scientific-councils/hypertension/trainee-advocacy-resources (2022).
National Heart, Lung, and Blood Institute. Global cardiovascular research funders forum. NHLBI https://www.nhlbi.nih.gov/funding/global-cardiovascular-research (2021).
National Institutes of Health. Early stage investigator policies. Grants and Funding https://grants.nih.gov/policy/early-stage/index.htm (2019).
Fitzsimons, D. Patient engagement at the heart of all European Society of Cardiology activities. Cardiovasc. Res. 115 , e99–e101 (2019).
Vandigo, J. et al. Continuous patient engagement in cardiovascular disease clinical comparative effectiveness research. Expert Rev. Pharmacoecon. Outcomes Res. 16 , 193–198 (2016).
Shilton, T., Champagne, B., Blanchard, C., Ibarra, L. & Kasesmup, V. Towards a global framework for capacity building for non-communicable disease advocacy in low- and middle-income countries. Glob. Health Promot. 20 , 6–19 (2013).
Fordyce, C. B. et al. Cardiovascular drug development: is it dead or just hibernating? J. Am. Coll. Cardiol. 65 , 1567–1582 (2015).
Schwartz, D. University-industry collaborations aspire to pharmaceutical innovation. Tech Transfer Central https://techtransfercentral.com/staged.techtransfercentral.com/2017/02/21/university-industry-collaborations-aspire-to-pharmaceutical-innovation/ (2017).
Novo Nordisk Global. Novo Nordisk Research Centre Oxford (NNRCO). Novo Nordisk https://www.novonordisk.com/science-and-technology/research-and-technology-centres/oxford-research-centre.html (2022).
AstraZeneca. AstraZeneca in Cambridge. AstraZeneca https://www.astrazeneca.com/our-company/our-locations/cambridge.html (2021).
Venkatasubramanian, K. V. India promotes industry-academia R&D collaborations. CEN Glob. Enterp. 95 , 15 (2017).
Noormahomed, E. et al. The evolution of mentorship capacity development in low- and middle-income countries: case studies from Peru, Kenya, India, and Mozambique. Am. J. Trop. Med. Hyg. 100 , 29–35 (2019).
Gandhi, M. et al. Mentoring the mentors: implementation and evaluation of four Fogarty-sponsored mentoring training workshops in low-and middle-income countries. Am. J. Trop. Med. Hyg. 100 , 20–28 (2019).
Sharma, G., Narula, N., Ansari-Ramandi, M. M. & Mouyis, K. The importance of mentorship and sponsorship: tips for fellows-in-training and early career cardiologists. JACC Case Rep. 1 , 232–234 (2019).
Ayyala, M. S. et al. Mentorship is not enough: exploring sponsorship and its role in career advancement in academic medicine. Acad. Med. 94 , 94–100 (2019).
Patton, E. W. et al. Differences in mentor-mentee sponsorship in male vs female recipients of National Institutes of Health grants. JAMA Intern. Med. 177 , 580–582 (2017).
American Heart Association. Mentoring for professionals. Prefessional Heart Daily https://professional.heart.org/en/partners/mentoring-for-professionals (2022).
International Society of Hypertension. Mentorship. ISH https://ish-world.com/mentorship/ (2021).
Figtree, G., Doyle, K., Hsu, M. P. & Freedman, B. The Australian Cardiovascular Alliance (ACvA). Eur. Heart J. 42 , 8–10 (2021).
PubMed Google Scholar
Cole, D. C. et al. Mentoring health researchers globally: diverse experiences, programmes, challenges and responses. Glob. Public Health 11 , 1093–1108 (2016).
Chi, B. H. et al. Evaluating academic mentorship programs in low- and middle-income country institutions: proposed framework and metrics. Am. J. Trop. Med. Hyg. 100 , 36–41 (2019).
Van Noorden, R. Some hard numbers on science’s leadership problems. Nature 557 , 294–296 (2018).
Nabel, E. G., Stevens, S. & Smith, R. Combating chronic disease in developing countries. Lancet 373 , 2004–2006 (2009).
UnitedHealth Group/National Heart, Lung, and Blood Institute Centres of Excellence. A global research network for non-communicable diseases. Lancet 383 , 1446–1447 (2014).
World Heart Federation. Emerging leaders programme. WHF https://world-heart-federation.org/emerging-leaders/ (2021).
Geerts, J. M., Goodall, A. H. & Agius, S. Evidence-based leadership development for physicians: a systematic literature review. Soc. Sci. Med. 246 , 112709 (2020).
Collins, F. New NIH approach to grant funding aimed at optimizing stewardship of taxpayer dollars. NIH https://www.nih.gov/about-nih/who-we-are/nih-director/statements/new-nih-approach-grant-funding-aimed-optimizing-stewardship-taxpayer-dollars (2017).
Levitt, M. & Levitt, J. M. Future of fundamental discovery in US biomedical research. Proc. Natl Acad. Sci. USA 114 , 6498–6503 (2017).
Deloitte Access Economics. Australia’s health and medical research workforce: expert people providing exceptional returns (Deloitte, 2016).
de Oliveira, C. et al. Estimating the payoffs from cardiovascular disease research in Canada: an economic analysis. CMAJ Open 1 , E83–E90 (2013).
Richards, G. C. et al. Challenges facing early-career and mid-career researchers: potential solutions to safeguard the future of evidence-based medicine. BMJ Evid. Based Med. 26 , 8–11 (2021).
Woolston, C. Work environment: when labs go bad. Nature 525 , 413–415 (2015).
Kruger, R. et al. Highlights from the International Society of Hypertension’s New Investigators Network during 2019. J. Hypertens. 38 , 968–973 (2020).
Casassus, B. Scientists disappointed by plan to boost France’s research prowess. Nature https://www.nature.com/articles/d41586-020-02217-4 (2020).
Boytchev, H. How Germany retains one of the world’s strongest research reputations. Nature 587 , S104–S105 (2020).
Article CAS Google Scholar
Remmel, A. Massive science-funding bill passes US Senate – but China focus worries researchers. Nature 594 , 485 (2021).
National Health and Medical Research Council. Outcomes of funding rounds. NHMRC https://www.nhmrc.gov.au/funding/data-research/outcomes (2021).
Ferguson, C., Henshall, C. & Albert, N. M. Global perspectives on under-funding for clinical research training fellowships in nursing. J. Clin. Nurs. 30 , e48–e50 (2021).
Adam, D. Science funders gamble on grant lotteries. Nature 594 , 485 (2019).
Google Scholar
Tan, T. Q. Principles of inclusion, diversity, access, and equity. J. Infect. Dis. 220 , S30–S32 (2019).
AlShebli, B. K., Rahwan, T. & Woon, W. L. The preeminence of ethnic diversity in scientific collaboration. Nat. Commun. 9 , 5163 (2018).
Jaijee, S. K., Kamau-Mitchell, C., Mikhail, G. W. & Hendry, C. Sexism experienced by consultant cardiologists in the United Kingdom. Heart 107 , 895–901 (2021).
Mehran, R. Women’s voices in cardiology: an uncomfortable silence. JAMA Cardiol. 3 , 676–677 (2018).
Curtis, A. B. & Rodriguez, F. Choosing a career in cardiology: where are the women? JAMA Cardiol. 3 , 691–692 (2018).
Rymer, J. A. et al. Evaluation of women and underrepresented racial and ethnic group representation in a general cardiology fellowship after a systematic recruitment initiative. JAMA Netw. Open 4 , e2030832 (2021).
Rai, D. et al. National trends of sex disparity in the American College of Cardiology/American Heart Association Guideline Writing Committee authors over 15 years. Circ. Cardiovasc. Qual. Outcomes 14 , e007578 (2021).
Denby, K. J. et al. Representation of women in cardiovascular clinical trial leadership. JAMA Intern. Med. 180 , 1382–1383 (2020).
Magliano, D. J., Macefield, V. G., Ellis, T. M. & Calkin, A. C. Addressing gender equity in senior leadership roles in translational science. ACS Pharmacol. Transl. Sci. 3 , 773–779 (2020).
Cech, E. A. & Blair-Loy, M. The changing career trajectories of new parents in STEM. Proc. Natl Acad. Sci. USA 116 , 4182–4187 (2019).
Pavalko, E. K. & Henderson, K. A. Combining care work and paid work: do workplace policies make a difference? Res. Aging 28 , 359–374 (2006).
King, T. et al. Reordering gender systems: can COVID-19 lead to improved gender equality and health? Lancet 396 , 80–81 (2020).
Workplace Gender Equality Agency. Designing and supporting gender equitable parental leave (WGEA, 2019).
Hagqvist, E., Nordenmark, M., Pérez, G., Trujillo Alemán, S. & Gillander Gådin, K. Parental leave policies and time use for mothers and fathers: a case study of Spain and Sweden. Soc. Health Vulnerability 8 , 1374103 (2017).
Organization for Women in Science for the Developing World. The OWSD–Elsevier Foundation awards for early career women scientists in the developing world. OWSD https://owsd.net/awards/awards (2021).
Department of Higher Education and Training. Future professors programme 01. FPP https://futureprofessorsprogramme.co.za/programme/ (2021).
Vassallo, A., Walker, K., Georgousakis, M. & Joshi, R. Do mentoring programmes influence women’s careers in the health and medical research sector? A mixed-methods evaluation of Australia’s Franklin Women Mentoring Programme. BMJ Open 11 , e052560 (2021).
Human and Infrastructure Capacity Development. Black Academics Advancement Programme (BAAP) framework (HICD, 2021).
Clarke, A. J., Burgess, A., van Diggele, C. & Mellis, C. The role of reverse mentoring in medical education: current insights. Adv. Med. Educ. Pract. 10 , 693–701 (2019).
Guarino, C. M. & Borden, V. M. H. Faculty service loads and gender: are women taking care of the academic family? Res. High. Educ. 58 , 672–694 (2017).
Chang, E. H. et al. The mixed effects of online diversity training. Proc. Natl Acad. Sci. USA 116 , 7778–7783 (2019).
Carnes, M. et al. The effect of an intervention to break the gender bias habit for faculty at one institution: a cluster randomized, controlled trial. Acad. Med. 90 , 221–230 (2015).
Saccoh, A., Tcheandjieu, C., Mukaz, D. K. & Amartey, J. #BlackInCardioWeek: How it started, what happened, and what is to come. BMC https://blogs.biomedcentral.com/on-medicine/2020/11/11/blackincardioweek-how-it-started-what-happened-and-what-is-to-come/ (2020).
Matsui, J. T. “Outsiders at the Table”–diversity lessons from the Biology Scholars Program at the University of California, Berkeley. CBE Life Sci. Educ. 17 , es11 (2018).
Gill, G. K., McNally, M. J. & Berman, V. Effective diversity, equity, and inclusion practices. Healthc. Manag. Forum 31 , 196–199 (2018).
Olzmann, J. A. Diversity through equity and inclusion: the responsibility belongs to all of us. Mol. Biol. Cell 31 , 2757–2760 (2020).
Green, B. N. & Johnson, C. D. Interprofessional collaboration in research, education, and clinical practice: working together for a better future. J. Chiropr. Educ. 29 , 1–10 (2015).
Williams, J., Craig, T. J. & Robson, D. Barriers and facilitators of clinician and researcher collaborations: a qualitative study. BMC Health Serv. Res. 20 , 1126 (2020).
NSW Ministry of Health. Cardiovascular Research Capacity Program. NSW Gov https://www.medicalresearch.nsw.gov.au/cardiovascular/ (2021).
Advance HE. Athena Swan Charter members. Advance HE https://www.advance-he.ac.uk/equality-charters/athena-swan-charter/members (2020).
Advance HE. The transformed UK Athena Swan Charter. Advance HE https://www.advance-he.ac.uk/equality-charters/transformed-uk-athena-swan-charter#report (2020).
Science in Australia Gender Equity. SAGE accreditation and awards. SAGE https://sciencegenderequity.org.au/sage-accreditation-and-awards/ (2021).
Science in Australia Gender Equity. Australian HER institutions stick with gender equity and diversity journey despite COVID-19 impact. SAGE https://www.sciencegenderequity.org.au/australian-her-institutions-stick-with-gender-equity-and-diversity-journey-despite-covid-19-impact/ (2020).
American Association for the Advancement of Science. What is SEA Change? AAAS https://seachange.aaas.org/about/what-is-sea-change (2021).
Natural Sciences and Engineering Research Council. Equity, diversity and inclusion: Dimensions charter. NSERC https://www.nserc-crsng.gc.ca/InterAgency-Interorganismes/EDI-EDI/Dimensions-Charter_Dimensions-Charte_eng.asp (2019).
Download references
Acknowledgements
The authors thank the Australian Cardiovascular Alliance (ACvA) Board for their support and acknowledge that the authors were members of the ACvA Emerging Leaders Committee at the time this paper was written. The authors acknowledge the supporting roles of the ACvA President G. Figtree, ACvA Chief Executive Officer K. Doyle and ACvA Project Officer M.-P. Hsu in the facilitation of this initiative. Finally, the authors acknowledge the following international cardiovascular researchers for their honest and generous feedback: M. Bertagnolli (McGill University, Canada), E. Bianchini (Institute of Clinical Physiology, Italy), D. Casarini (Federal University of São Paulo, Brazil), L. Gafane-Matamane (North-West University, South Africa), A. Kirabo (Vanderbilt University, USA), C. McCarthy (University of South Carolina, USA), A. Montezano (University of Glasgow, UK) and M. Patel (India Association for Parenteral and Enteral Nutrition Association, India). E.T. (105215), S.C.I. (102821), R.E.C. (102484), D.S.P. (104774), L.B. (102498), S.G.W. (105622), A.C.C. (105631) and F.Z.M. (101185, 105663) are funded by fellowships from the National Heart Foundation of Australia. The National Health and Medical Research Council of Australia supports K.M.M.C. through a C.J. Martin Fellowship (1112125) and L.B. through an Emerging Leader Investigator Grant (1172987). F.Z.M. is also supported by a Senior Medical Research Fellowship from the Sylvia and Charles Viertel Charitable Foundation.
Author information
These authors contributed equally: Niamh Chapman, Emma Thomas.
Authors and Affiliations
Menzies Institute for Medical Research, University of Tasmania, Hobart, Tasmania, Australia
Niamh Chapman, Rachel E. Climie & Dean S. Picone
Centre for Online Health, University of Queensland, Brisbane, Queensland, Australia
Emma E. Thomas
Centre for Health Services Research, University of Queensland, Brisbane, Queensland, Australia
Vascular Research Centre, Lifelong Health Theme, South Australian Health & Medical Research Institute, Adelaide, South Australia, Australia
Joanne T. M. Tan
Adelaide Medical School, University of Adelaide, Adelaide, South Australia, Australia
IMPACCT — Improving Palliative, Aged and Chronic Care through Clinical Research and Translation, Faculty of Health, University of Technology Sydney, Sydney, New South Wales, Australia
Sally C. Inglis
The George Institute for Global Health, Faculty of Medicine, University of New South Wales, Sydney, New South Wales, Australia
Jason H. Y. Wu
Baker Heart and Diabetes Institute, Melbourne, Victoria, Australia
Rachel E. Climie, Anna C. Calkin & Francine Z. Marques
Paris Cardiovascular Research Center, Integrative Epidemiology of Cardiovascular Disease, Université de Paris, Paris, France
Rachel E. Climie
Nutrition & Health Innovation Research Institute, School of Medical and Health Sciences, Edith Cowan University, Perth, Western Australia, Australia
Lauren C. Blekkenhorst
Royal Perth Hospital Research Foundation, Perth, Western Australia, Australia
Medical School, The University of Western Australia, Perth, Western Australia, Australia
School of Medical Sciences, Faculty of Health and Medicine, University of Sydney, Sydney, New South Wales, Australia
Steven G. Wise
Department of Physiology and Biomedicine Discovery Institute, Monash University, Melbourne, Victoria, Australia
Katrina M. Mirabito Colafella
Central Clinical School, Monash University, Melbourne, Victoria, Australia
Anna C. Calkin
Baker Department of Cardiometabolic Health, University of Melbourne, Melbourne, Victoria, Australia
Hypertension Research Laboratory, Monash University, Melbourne, Victoria, Australia
Francine Z. Marques
You can also search for this author in PubMed Google Scholar
Contributions
All the authors wrote parts of the manuscript, and reviewed and edited the manuscript before submission, all having a substantial role.
Corresponding author
Correspondence to Francine Z. Marques .
Ethics declarations
Competing interests.
The authors declare no competing interests.
Peer review
Peer-review information.
Nature Reviews Cardiology thanks Barbara Casadei, who co-reviewed with Rohan Wijesurendra, and the other, anonymous, reviewer(s) for their contribution to the peer review of this work.
Additional information
Publisher’s note.
Springer Nature remains neutral with regard to jurisdictional claims in published maps and institutional affiliations.
Related links
Athena Scientific Women’s Academic Network Charter: https://www.advance-he.ac.uk/equality-charters/athena-swan-charter
Black in Cardio: https://blackincardio.com/
Black in Physiology: https://blackinphysiology.com
Dimensions: https://www.nserc-crsng.gc.ca/InterAgency-Interorganismes/EDI-EDI/Dimensions_Dimensions_eng.asp
European Research Council: https://erc.europa.eu
MINDSHIFT: https://www.eumindshift.eu
Science in Australia Gender Equity: https://www.sciencegenderequity.org.au/the-athena-swan-accreditation-framework/
STEMM Equity Achievement Change: https://www.aaas.org/programs/sea-change
Rights and permissions
Reprints and permissions
About this article
Cite this article.
Chapman, N., Thomas, E.E., Tan, J.T.M. et al. A roadmap of strategies to support cardiovascular researchers: from policy to practice. Nat Rev Cardiol 19 , 765–777 (2022). https://doi.org/10.1038/s41569-022-00700-1
Download citation
Accepted : 27 March 2022
Published : 16 May 2022
Issue Date : November 2022
DOI : https://doi.org/10.1038/s41569-022-00700-1
Share this article
Anyone you share the following link with will be able to read this content:
Sorry, a shareable link is not currently available for this article.
Provided by the Springer Nature SharedIt content-sharing initiative
This article is cited by
An electrochemical immunosensor based on polyaniline microtubules and zinc gallinate for detection of human growth differentiation factor-15.
Microchimica Acta (2023)
Health research, development and innovation capacity building, enhancement and sustainability
- Marlon E. Cerf
Discover Social Science and Health (2023)
Quick links
- Explore articles by subject
- Guide to authors
- Editorial policies
Sign up for the Nature Briefing newsletter — what matters in science, free to your inbox daily.

An official website of the United States government
Official websites use .gov A .gov website belongs to an official government organization in the United States.
Secure .gov websites use HTTPS A lock ( Lock Locked padlock icon ) or https:// means you've safely connected to the .gov website. Share sensitive information only on official, secure websites.
- Publications
- Account settings
- Advanced Search
- Journal List

Precision Medicine and the future of Cardiovascular Diseases: A Clinically Oriented Comprehensive Review
Yashendra sethi, oroshay kaiwan, arsalan moinuddin, ashish goel, hitesh chopra, simona cavalu.
- Author information
- Article notes
- Copyright and License information
Correspondence: [email protected] (N.K.); [email protected] (A.M.); [email protected] (S.C.)
These authors contributed equally to this work.
Received 2023 Feb 1; Revised 2023 Feb 17; Accepted 2023 Feb 20; Collection date 2023 Mar.
Licensee MDPI, Basel, Switzerland. This article is an open access article distributed under the terms and conditions of the Creative Commons Attribution (CC BY) license ( https://creativecommons.org/licenses/by/4.0/ ).
Cardiac diseases form the lion’s share of the global disease burden, owing to the paradigm shift to non-infectious diseases from infectious ones. The prevalence of CVDs has nearly doubled, increasing from 271 million in 1990 to 523 million in 2019. Additionally, the global trend for the years lived with disability has doubled, increasing from 17.7 million to 34.4 million over the same period. The advent of precision medicine in cardiology has ignited new possibilities for individually personalized, integrative, and patient-centric approaches to disease prevention and treatment, incorporating the standard clinical data with advanced “omics”. These data help with the phenotypically adjudicated individualization of treatment. The major objective of this review was to compile the evolving clinically relevant tools of precision medicine that can help with the evidence-based precise individualized management of cardiac diseases with the highest DALY. The field of cardiology is evolving to provide targeted therapy, which is crafted as per the “omics”, involving genomics, transcriptomics, epigenomics, proteomics, metabolomics, and microbiomics, for deep phenotyping. Research for individualizing therapy in heart diseases with the highest DALY has helped identify novel genes, biomarkers, proteins, and technologies to aid early diagnosis and treatment. Precision medicine has helped in targeted management, allowing early diagnosis, timely precise intervention, and exposure to minimal side effects. Despite these great impacts, overcoming the barriers to implementing precision medicine requires addressing the economic, cultural, technical, and socio-political issues. Precision medicine is proposed to be the future of cardiovascular medicine and holds the potential for a more efficient and personalized approach to the management of cardiovascular diseases, contrary to the standardized blanket approach.
Keywords: precision medicine, cardiology, precision cardiology, hypertension, heart failure, myocardial infarction
1. Introduction
Over the past three decades, cardiovascular diseases (CVDs) have preponderated global disease burden––93% prevalence, 54% mortality, and 60% in disability-adjusted life years (DALY) [ 1 , 2 , 3 ]. This is further exacerbated by disparities in the inter- and intra-continental disease burden, costing USD 216 billion and USD 147 billion annually for healthcare and productivity loss, respectively [ 4 , 5 , 6 ].
The rectitude of medicine has always emphasized treating the patient rather than the disease. Today’s medicine is honing itself to be more precise and patient-centric. Precision medicine is an innovative clinical approach that uses individual genomic, environmental, and lifestyle information to guide medical management. It has already revolutionized oncology [ 7 ]; CVDs form their current epicenter owing to their heterogeneity and multi-causality, which leads to altered responses to treatment for each patient. The long-old treatment principles are succored by technological evolution in the “omics”—genomics, transcriptomics, epigenomics, metabolomics, proteomics, and microbiomics— which, together, help frame the position for future medicine [ 8 ]. “Omics” is aided by advanced “big data” analysis, which has helped in the development of in-depth clinical, biological, and molecular phenotyping, promoting better-integrated healthcare with early diagnosis, enhanced risk stratification, and disease management with the least possible side effects [ 9 ].
Most CVDs stem from a complex interplay of modifiable and non-modifiable factors which aggravate the set “omic” predisposition. In contemporary cardiology, most diagnostic criteria and therapeutic approaches rely on population-based studies, with less focus on approaches tailored to individualize patient treatment [ 10 ]. As such, a comprehensive analysis of phenotypes and the “omics” can help cluster patient groups sheathing disparities, simultaneously reinforcing patient-centric clinical care. Further, it will enhance patient quality of life (QOL) and help reduce complications via novel biomarkers, improved AI-assisted diagnostics, targeted therapeutics, and appropriate long-term risk assessment [ 11 ].
With technological advancements in data science and machine learning, the applicability of precision medicine in CVDs seems within reach, especially with the significant evolving literature in the pipeline over the past decade. As such, we aim to: 1. pool evolving clinically relevant information on precision medicine in cardiology, and 2. provide a comprehensive synthesis of the relevant literature to date. Thus, this will help with the evidence-based precise management of cardiac diseases and identification of possible challenges.
2. Precision Medicine and Cardiology
The advent of precision medicine has the potential to revolutionize the future of cardiovascular disease (CVD) healthcare via its application through “omics” in cardiology ( Figure 1 ). It empowers a physician to treat cardiac diseases on an individual basis—based on the patient’s unique profile. Recent times have seen a growing body of literature underlining the application of precision medicine in cardiology. Table 1 presents a compilation underlining the clinical significance of all “reviews” published over the past decade regarding the same, while Table 2 , Table 3 and Table 4 present an omic-stratified and disease-specific compilation of the literature for myocardial infarction, hypertension, and heart failure, respectively. As such, precision medicine in cardiology promises to improve health and revolutionize the management previously manifested in oncology. The evolution of precision medicine in cardiology has been remarkable ( Figure 2 ). Its applicability can have the best impact if enacted on the diseases with the highest impact (associated with the highest DALY), these include—myocardial infarction, hypertension, and heart failure.
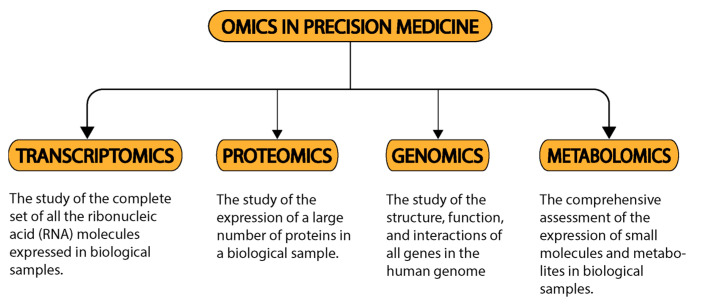
The OMICS in precision medicine.
A compilation of important reviews underlining the role of precision medicine in cardiology.
A compilation of the literature underlining role of precision medicine in hypertension.
A compilation of the literature underlining role of precision medicine in heart failure.
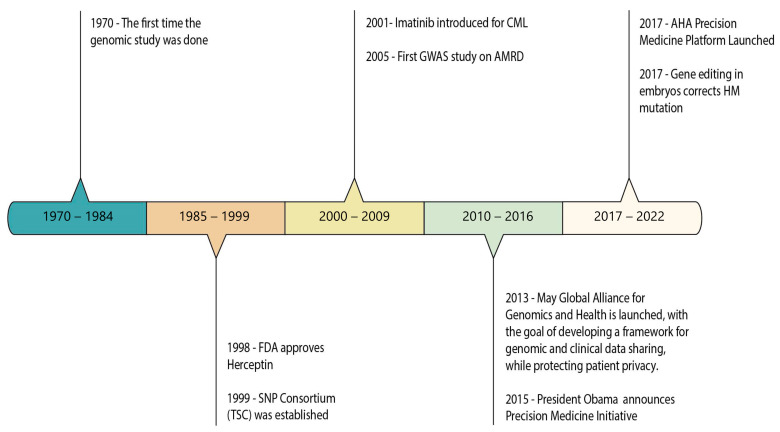
Timeline of the evolution of precision medicine in cardiology.
2.1. Myocardial Infarction
Myocardial infarction (MI) is the leading cause of death globally—16% of total deaths. Its pathogenesis is peculiar in terms of its heterogeneous causality and largely varied genetic predisposition. MI is a critical medical emergency, true to its scientific adage “Time is equal to Myocardium”. An opportune diagnosis with sensitive markers, optimal intervention, and the prevention of complications and recurrence is extremely consequential. Precision medicine may find its applications in all these areas ( Table 2 ) and may guide research and drug development to add to the pharmacotherapeutic armamentarium for this disease [ 29 , 30 ].
A compilation of the literature underlining the role of precision medicine in myocardial infarction.
2.1.1. Metabolomics
The association between an individual’s metabolic profile and MI has been explored a lot recently. Svati Shah et al. demonstrated an independent association between peripheral blood metabolites and the presence of CAD (coronary artery disease). They showed that simple metabolites such as factor 4 (branched-chain amino acid metabolites) and factor 9 (urea cycle metabolites) can help diagnose CAD [ 48 , 50 ]. Many new metabolites have recently been associated with CAD, including but not limited to: medium-chain acylcarnitines, short-chain dicarboxylacylcarnitines, long-chain dicarboxylacylcarnitines, long-chain acylcarnitines, short-chain acylcarnitines, medium-chain acylcarnitines, ketone related, cholesterol, lipids, fatty acids, glucose, and branched-chain amino acids [ 49 ]. Another recent observational study by Tanzilli G et al. found that low serum albumin levels are associated with adverse events in STEMI patients [ 46 ]. Uchino Y et al. and Ijichi C et al. showed that supplementing patients with BCAAs (branch-chain amino acids) showed increased serum albumin levels [ 51 , 52 ]. The combination of one or more newer metabolic markers can aid the diagnosis for a certain subset of patients, personalizing their care and increasing the sensitivity of MI detection.
Metabolomics can improve treatment options in addition to its predictive and diagnostic capabilities. Currently, the mainstay of acute coronary syndrome treatment is revascularization via emergency percutaneous coronary intervention (PCI). Tanzilli G et al. proposed ways to improve PCI by using early and prolonged glutathione infusions to blunt the inflammatory response via a chain of processes: 1. reduced NOX2 activation; 2. hsCRP generation; 3. TNF- levels; 4. cTpT release; 5. reduced neutrophil generation to protect myocardial cells; and 6. prevention of aberrant cardiac remodeling, allowing better left ventricular size and function post-PCI [ 46 ].

2.1.2. Genomics
Advances in genomics are helping the knowledge gained to be incorporated into the treatment and diagnosis of MI. An RCT by Scott R et al. discovered six genes (CNR2, DPP4, GLP1R, SLC5A1, HTR2C, MCHR1) that could be potentially used to develop drugs to treat type 2 diabetes or obesity without incremental CVD risk [ 45 ]. At present, post-PCI, dual antiplatelet therapy (DAPT) is initiated to reduce the risk of thrombosis or MI. DAPT usually consists of aspirin and clopidogrel. However, patients with loss-of-function CYP2C19 mutation have elevated chances of ischemic events if treated with standard DAPT therapy [ 44 ]. Pereira N et al. showed that the genotype CYP2C19-guided selection of P2Y12 inhibitor was superior to standard treatment concerning thrombotic events and resulted in a lower incidence of bleeding. They also proposed that a genotype-guided P2Y12 inhibitor such as ticagrelor should be selected for such patients [ 43 ].
2.1.3. Biomarkers
The development of biomarkers in precision medicine has been astounding and is still evolving. Mangion K et al. suggested the use of computational modeling to provide precise diagnostic care to patients and improve individual risk prediction. Computed biomechanical parameters such as contractility, stiffness, myofilament kinetics, strain, and stress provide information about LV function, thus determining the extent of cardiac damage and future prognosis [ 37 ]. Crea F et al. established biomarkers such as myeloperoxidase and hyaluronidase-2 to identify plaque erosion among ACS patients [ 35 ]. The CD44v6 splicing variant of the hyaluronan receptor was significantly higher in patients with plaque erosion than in plaque fissures, which can help detect silent myocardial infarctions. Adding to the evidence of the benefit of therapy individualization, Pasea L et al. concluded that the decision to prolong DAPT therapy should be assessed individually for each patient [ 38 ]. For assessment, prognostic models should look at demographics and behavior, cardiovascular history, non-cardiovascular history, biomarkers, and drugs. Tong G et al. explored the use of basic fibroblast growth factor (bFGF) in myocardial ischemia injury [ 33 ]. Oxidative stress plays a major role in myocardial injury, and bFGF can reduce oxidative stress by promoting the activation of NrF2 via the Akt/GSK3b/Fyn pathway, which reduces cardiomyocytes apoptosis and thus the infarct size to a larger extent, ultimately alleviating the heart injury. More studies are being conducted to study the cardioprotective effect of bFGF, which is certainly a novel preventive tool to treat MI. Further, Oni-Orisan A et al. found that epoxyeicosatrienoic acid (EET) elicits potent anti-inflammatory, vasodilatory, anti-apoptotic, pro-angiogenic, fibrinolytic, and smooth muscle cell anti-migratory effects with-in the cardiovascular system [ 41 ], and thus can be used in the treatment of acute MI. Wen Z et al. investigated the application of telmisartan-doped co-assembly nanofibers (TDCNfs), dual-ligand supramolecular nanofibers that synergistically counter-regulate RAS through targeted delivery, and presented it as an option for combined therapy against cardiac deterioration post-MI [ 32 ]. It reduces apoptosis, alleviates inflammatory response, and inhibits fibrosis to potentially mitigate post-MI outcomes [ 32 ]. Aside from MI, the treatment for myocardial infarction with no obstructive coronary arteries (MINOCA) also requires an investigation of cause to determine the patient-specific treatment [ 36 ]. Collectively, precision medicine helps to individualize the therapy by blending various diagnostic modalities such as ECG, cardiac enzymes, other biomarkers, echocardiography, coronary angiography, coronary vasomotion, and intravascular imaging techniques.
2.2. Hypertension
Hypertension (HTN) is a major modifiable risk factor for cardiovascular morbidity and mortality. Globally, it forms a major share of NCD (Non-communicable diseases); about 1.28 billion adults aged 30–79 years have HTN [ 53 ]. Due to its insidious onset, the majority of HTN cases remain unidentified and run a silent but catastrophic course. Early detection and optimal control can considerably reduce the cardiovascular burden associated with it. The pathophysiology of hypertension includes an interplay between genetic, physiological, biochemical, and environmental factors, which vary amongst individuals [ 54 ]. Precision medicine in hypertension can specifically identify patient subgroups with distinct disease causation mechanisms and their differential responses to diverse antihypertensive treatments. A compilation of recent evidence for the application of PM in hypertension is listed in Table 3 .
2.2.1. Hypertension Pharmacogenomics
Genetic polymorphisms and response to diuretics: Current evidence identifies maximum target polymorphisms in response to thiazide diuretics. As opposed to the findings of the GenHAT study, the homozygous carriers of ACE I/D polymorphisms, ACE II, showed a small reduction in blood pressure response to hydrochlorothiazide, compared to homozygous carriers of ACE DD alleles [ 72 , 75 ]. Another study reported the response to thiazide diuretic in African Americans with SNP rs7297610 CC located on chromosome 12q15 [ 76 ]. Other genetic association studies showed that PRKCAA allele carriers had a better blood pressure response than GG homozygote patients. Another study targeting the UMOD gene polymorphism showed differential BP response to loop diuretics in hypertensives. They divided patients into two groups: 1. UMOD group (AA genotype), which showed a good response to loop diuretics, and 2. “Low” UMOD group, which exhibited a lower BP response to loop diuretics [ 70 ].
Genetic polymorphisms and response to other antihypertensives: Blood pressure regulation in humans involves at least 70 genes and presents with a complex set of individual differences. The human beta (1)-adrenergic receptor (ADRB1) has two common functional polymorphisms (Ser49Gly and Gly389Arg), which are associated with varied responses to metoprolol in essential hypertension. Furthermore, 49Ser389Arg/49Ser389Arg and 49Ser389Arg/49Gly389Arg polymorphisms have been seen as good responders, whilst 49Ser389Gly/49Gly389Arg and 49Ser389Gly/49Ser389Gly polymorphisms have been seen to be non-responders [ 77 ]. ADRB1 polymorphisms further revealed that C allele homozygotes showed a better response to metoprolol than G allele carriers [ 78 ]. Another study reported a possible link between nephrin (NPNS1) gene variants and a good response to the angiotensin receptor antagonist, losartan, in hypertensive patients [ 79 ]. Other less commonly studied genetic polymorphisms include GRK4 polymorphisms, namely R65L, A142V, and A486V. Studies have revealed that homozygote double variants of 65 L and 142 V require more aggressive antihypertensive therapy than the homozygous single variants or heterozygous carriers to achieve a target mean arterial blood pressure [ 71 ].
Another study aimed at finding rare and common variants associated with hypertension identified 31 novel genetic regions—rare missense variants in RBM47, COL21A1, and RRAS [ 67 ]. These allelic variations can lay the foundation for newer drug targets. Furthermore, by using polygenic risk score (based on the total number of genetic loci required to be assessed to estimate the risk of developing a disease), five potential loci (PKD2L1, SLC12A2, CACNA1C, CACNB4, and CA7) have been reported as novel therapeutic targets for hypertensive therapy. More than 1000 hypertension-associated loci have now been identified, with drug-target genes expected to expand in the future [ 65 ]. Loganathan et al. identified other hypertension-associated loci and SNPs (Single Nucleotide Polymorphisms) such as RAAS signaling and Cytochrome P (CYP) genes, which govern individual and population differences in drug tolerance [ 61 ]. In 2018, the GWAS catalog found seven candidate genes with an established pathophysiological role in hypertension, namely ACE1, ACE2, ADRB1, ADRB2, MME, CACNA2D2, and UMOD [ 63 ].
Recently, newer drugs such as Rostafuroxin have disrupted the binding of mutant alpha-adducin and the ouabain-activated Na-K pump with the Src-SH2 domain, in rats as well as in human cell cultures, posing as potential antihypertensives [ 80 ]. Evidence also suggests that riboflavin, a co-factor for MTHFR, also has an antihypertensive effect via the MTHFR 677TT genotype-specific mechanism [ 81 ]. Another potential choice is aldosterone, which targets the epigenetically modified sodium channel epithelial 1α subunit (SCNN1A). It hypomethylates the histone protein (H3) at lysine 79 (H3K79) at subregions of the promoter in a subgroup of hypertensives [ 68 ].
2.2.2. Hypertension Metabolomics
The effect of metabolomic factors in hypertension is another limb to study for the precision medicine domain. The most commonly identified metabolomic factors include sex, gender, race, and plasma renin activity; their response to antihypertensives was studied. A growing body of literature found that hypertensive women have lower plasma renin activity as opposed to men, and thus were more responsive to diuretics and Calcium Channel Blockers (CCB) as compared to angiotensin-converting enzyme inhibitors (ACEI) and beta blockers [ 82 ]. Another study comparing renin profiling-guided (plasma renin activity) treatment to clinical judgment in uncontrolled hypertensive patients showed equal or better hypertension control while using the renin profile-guided treatment approach [ 83 ]. Adverse drug events following antihypertensive medications were more common in females, though a notable exception was aldosterone antagonists [ 56 ]. It has been shown that African Americans respond to diuretics and CCBs better than ACEIs, probably due to their RAAS genes and increased plasma level in conjunction with suppressed plasma renin activity. These factors are hypothesized to cause variations in drug responses, Additionally, the African race is predisposed to severe hypertension, courtesy of their enhanced vascular contractility and salt-retaining capacity [ 59 ]. Furthermore, a raised sympathetic tone amongst obese patients responds better to beta blockers [ 57 ]. Ongoing clinical trials are investigating biochemical pathways, pharmaco-metabolomics, and pharmacogenomics in antihypertensive drug responses.
2.2.3. Resistant Hypertension
A recent experimental study by Bazzell et al., on hypertension transcriptomics, measured mRNA transcripts in the human urine supernatant to detect mineralocorticoid receptor activation and predict its response to mineralocorticoid receptor antagonists in hypertensive patients. The results of the RNA sequencing of urine extracellular vesicles match those of the human kidney. Alterations in mRNA in urine supernatant were associated with changes in human endocrine signaling (MR activation). These findings can aid in individualizing pharmaceutical therapy in patients with mineralocorticoid signaling abnormalities, such as resistant hypertension. These findings could be utilized to noninvasively discover possible indicators of abnormal renal and cardiorenal physiology [ 74 ]. In light of the current evidence available, it can be concluded that what we know about the pharmacogenomics of hypertension is only the tip of the iceberg, and finding more precise targets and therapies is imperative.
2.3. Heart Failure
Heart failure (HF) is one of the most challenging cardiovascular disorders to manage. Despite recent advances in symptom management and the possibility of halting disease progression, the structural and functional impairment associated with HF is irreversible. It is a disorder with heterogenous causality and a strong genetic predisposition. Thus, risk assessment, prevention, and early screening are key in its management. Precision medicine poses to fill the existing gap in preventive medical management and risk stratification ( Table 4 ).
2.3.1. Genomics
The relevance of common genetic variation in the susceptibility to and heritability of HF has recently been investigated through large-scale genome-wide techniques. F Dominguez found that DCM (dilated cardiomyopathy) caused by mutations in BAG3 has high penetrance in carriers >40 years of age and increases the risk of progressive heart failure [ 103 ]. Shah S et al. found that loci KLHL3 and SYNPOL2–AGAP5 are implicated in HF, and also BAG3 and CDKN1A are associated with LV systolic dysfunction [ 104 ]. Maurer, MS et al., documented that tafamidis reduced death and hospitalizations associated with cardiovascular events in patients with transthyretin-associated cardiomyopathy [ 85 ]. Dumeny et al. found that NR3C2, which codes the target protein of spironolactone, or CYP11B2, which is involved in aldosterone synthesis, was associated with better spironolactone response in diastolic HF patients [ 84 ].
2.3.2. Proteomics
Glick D found that among older adults without HF with initially low cardiac troponin T(cTnT) and N-terminal pro-brain natriuretic peptide (NT-proBNP), the long-term trajectory of both biomarkers predicts systolic dysfunction, incident HF, and CV death [ 93 ]. Pozsonyi et al. defined that copeptin predicted 5-year all-cause mortality in heart failure patients. Drum et al., found that plasma TB4 is elevated in women with HFpEF, which predicts mortality independent of clinical risk factors and NT-proBNP in women with HF [ 92 ]. Feng SD et al. found that β-endorphin (β-EP) and brain natriuretic peptide BNP have both high specificity and sensitivity to detecting early acute left heart failure and atrial fibrillation in patients [ 89 ]. Pellicori et al., investigated the effects of spironolactone on the serum markers of collagen metabolism and cardiovascular structure and function in people at risk of developing HF, as well as the potential interactions with a marker of fibrogenic activity, galectin-3 [ 88 ]. G Michael Felker found that Hs-cTnT was elevated in the majority of acute heart failure (AHF) patients. Baseline, peak, and peak change hs-cTnT were associated with worse outcomes, mainly 180-day cardiovascular mortality [ 91 ]. Shah et al. found that MR-proANP seems accurate in diagnosing acute decompensated heart failure (ADHF), whilst both mid-regional pro-atrial natriuretic peptide (MR-proANP) and mid-regional pro-adrenomedullin (MR-proADM) acclimatize prognosis [ 50 ]. Sjoukje I Lok found that growth differentiation factor 15 (GDF) levels are increased in patients with HF and correlate with the extent of myocardial fibrosis, hence they are used as a biomarker for cardiac remodeling [ 95 ]. Natalia Lopez-Andrès et al. found that increased galectin-3 (Gal-3) and N-terminal propeptide III procollagen (PIIINP), and low metallic metalloproteinase-1 (MMP-1) are associated with adverse long-term heart failure outcomes [ 96 ]. Julio Núñez et al. suggested that CA125 is a surrogate of fluid overload, hence it is potentially valuable for guiding decongestion therapy, and a CA125-guided diuretic strategy improved eGFR in patients with acute heart failure with renal dysfunction [ 87 ]. Hanna K Gaggin et al. found that the soluble suppression of tumorigenesis (sST2) measurement identifies patients with chronic heart failure in whom higher beta-blocker doses may be beneficial [ 94 ].
2.3.3. Metabolomics
Metabolomics is the study of tiny, organic compounds within metabolic pathways. With the improvement of technology, nuclear magnetic resonance, gas chromatography, and mass spectrometry have enabled the discovery and analysis of enormous databases of metabolites implicated in heart failure. The molecular pathways implicated in cardiac failure show that a metabolic transition occurs in the failing myocardium. Metabolic profiles of patients with systolic heart failure have been developed by examining patient serum and breath. These profiles can be used clinically for diagnosis and prognosis in this population [ 105 ]. Du Z et al. suggested that 3-hydroxybutyrate, acetone, and succinate were elevated in patients with HFrEF and can predict outcomes in patients with HF [ 99 ]. Additionally, Hunter WG et al. found that levels of metabolites in medium- and long-chain acylcarnitines and ketone bodies are higher in patients with HFpEF compared to patients with HfrEF [ 98 ]. Wang Li et al. suggested that patients with HFrEF with ischemic causes had higher levels of lactate, alanine, creatinine, proline, isoleucine, and leucine in plasma than healthy subjects [ 100 ]. Desmoulin F et al. found that an increased ratio of plasma lactate to total cholesterol is a significant predictor of 30-day mortality in patients with acute decompensated HF (ADHF) [ 101 ]. Ahmad T et al. found that increased circulating long-chain acylcarnitine metabolite levels in patients with chronic HF were associated with adverse clinical outcomes [ 106 ]. Treating patients with end-stage HF with long-term mechanical circulatory support resulted in significantly decreased circulating long-chain acylcarnitine levels, suggesting that levels of long-chain acylcarnitine can be used to prognosticate HF outcomes. Olivotto I et al. found that treatment with mavacamten, a small molecule modulator of β-cardiac myosin, improved exercise capacity, LVOT obstruction, NYHA functional class, and health status in patients with obstructive hypertrophic cardiomyopathy [ 97 ].
2.3.4. Microbiomics
W H Wilson Tang et al. suggested that high trimethylamine N-oxide (TMAO) levels were observed in patients with HF, and elevated TMAO levels portended higher long-term mortality risk [ 102 ].
2.4. Precision Medicine and Aortic Diseases
The genetic basis of aortic diseases has long been known. Twenty percent of patients with thoracic aortic aneurysms and aortic dissection either have a family history or are associated with a syndrome such as Marfan syndrome, vascular Ehlers–Danlos syndrome, and Loeys–Dietz syndrome. Mutations of ACTA2, MYLK , and MYH11 have been found to be associated with aortic disease [ 107 ]. Furthermore, genetic variants of genes FBN1, SMAD3 , and ACTA2 have also been shown to cause either syndromic or non-syndromic thoracic aortic aneurysm and dissection [ 108 , 109 ]. The recent updates have added evidence to support the role of pathogenic variants in COL3A1, FBN1, MYH11, SMAD3, TGFB2, TGFBR1, TGFBR2, MYLK, LOX , and PRKG1, predisposing to hereditary thoracic aortic disease. The above insight into the genes associated with aortic diseases can be added to the clinical database. Patients with clinical suspicion can be tested for genetic predisposition, and the results can be saved on their EHR (electronic health records) to help personalize their treatment [ 109 , 110 ].
3. Precision Cardiology and Artificial Intelligence
The evolution in tools of artificial intelligence (AI) and machine learning models has made it possible to incorporate multimodal and multidimensional omics, which promise enhanced diagnosis and treatment modalities for tomorrow. AI has the potential to usher in the next medical revolution and enhance precision medicine to stratify patients according to their phenotypic characteristics. The incorporation of AI into laboratory medicine and diagnostics can aid in better performing screening and confirmatory tests. AI can be used to generate insights by integrating powerful computing and analysis, thus allowing the system to think, learn and empower clinical decision-making with augmented intelligence [ 111 ]. The advances in artificial intelligence and data science have allowed for the automation of various critical thinking processes in medicine, including diagnosis, risk classification, and management, easing the workload of doctors and decreasing the possibility of making errors. It has many different uses in the workplace and the care of patients, from making doctors’ life easier to facilitating research. As a field that relies heavily on abstract reasoning and interpretation, cardiology is a natural fit for the introduction of AI. Clinical evaluation, imaging interpretation, diagnosis, prognosis, risk stratification, precision medicine, and therapy for various cardiac diseases have all benefited from the use of artificial intelligence. Clinical diagnostic accuracy, especially for pediatric cardiac diseases, has been bolstered by the application of neural networks and machine learning. AI has helped increase the diagnostic utility of imaging modalities such as cardiac magnetic resonance imaging, echocardiograms, computer tomography scans, and electrocardiograms. In pediatric cardiac surgeries, the introduction of AI-based prediction algorithms greatly improves post-operative outcomes and prognosis. Important clinical results can be used with suitable computer algorithms for risk classification and predicting treatment outcomes [ 112 ]. Although artificial intelligence has made medicine more precise and accurate, it still has a long way to go and has some serious limitations. The acceptability is hampered by difficulties such as a lack of adequate algorithms and their infancy, a lack of physician training, a concern of over-mechanization, and dread of missing the “human touch”. The generalizability of algorithms developed in standardized research environments employing high-quality data to heterogeneous real-world populations must be rigorously evaluated. Biases in training data, model overfitting, insufficient statistical correction for multiple testing, and limited accountability around the processes by which deep learning algorithms reach their outcome (“black box” systems) are just a few of the pitfalls of AI that can have serious consequences for the patients, and they necessitate careful consideration by researchers, clinicians, and regulatory bodies. Despite the challenges, we believe that AI will be the perfect assistant to clinicians in directing adult and pediatric cardiology in the future [ 112 , 113 ]. Table 5 underlines the clinical applicability of AI in Precision cardiology.
Clinical applicability of artificial intelligence in precision cardiology [ 11 , 111 ].
4. Cardiovascular Pharmacology and Precision Medicine
The clinical trials help us judge and predict drug outcomes with the best representative samples that evolve with phases of clinical trials, but the genetic variations, environmental factors, and idiosyncrasies are still strong enough to cause a fair number of adverse events and treatment failures.
The evolving approach of precision medicine advocates the individualization of therapy, directed by local regulations and guidelines based on novel markers and gene targets, which can help us define reasons for failure, thus evolving a better tailored patient-centric approach to curing diseases. Numerous examples of genetic diversity and DNA variants determining the response to a drug are already in common parlance and are continually used to modify treatment. For instance, warfarin, the most commonly prescribed anticoagulant medicine, has a narrow therapeutic window and has shown wide inter-individual variations [ 114 , 115 ]. Studies document around 10% to 50% variability in warfarin dose requirements per the patient genotype, notably SNPs in CYP2C9 ( CYP2C9*2, CYP2C9*3 ) and VKORC1 (rs9923231) [ 115 , 116 , 117 ]. Additionally, genetic variants have been identified that show differences in the response to β-blockers ( ADRB1, ADRB2, GRK5, GRK4 ); angiotensin-converting enzyme inhibitors ( ACE, AGTR1 ); diuretics ( ADD1, NPPA, NEDD4L ); and Calcium Channel Blockers ( CACNB2, CACNA1C ) [ 26 ]. Further, clopidogrel, an antiplatelet medicine, is a P2Y12 inhibitor, and it shows great inter-individual variability–the clopidogrel non-responders [ 106 , 118 , 119 ]. The genetics purported behind this involve loss-of-function alleles in CYP2C19 (CYP2C19*2 and CYP2C19*3), which is thought to be associated with poor drug responsiveness, whilst the gain-of-function allele CYP2C19*17 is associated with increased bleeding risk [ 119 , 120 ]. Now, the COAG (Clarification of Optimal Anticoagulation through Genetics) trial and the EU-PACT (European Pharmacogenetics and Anticoagulant Therapy-Warfarin) have produced RCTs advocating genotype-guided drug dosing for warfarin, which prescribe dosing based on CYP2C9 and VKORC1 genotyping [ 121 , 122 ]. Precision medicine aided by pharmacogenomics and pharmacogenetic profiling poses to refine this area, bringing in next-generation care using enhanced phenotyping for disease stratification [ 123 ].
5. Precision Cardiology and the Omics
Cardiovascular research is increasingly a part of the vast, digital, data-driven world made possible by the plethora of molecular, physiological, and environmental data generated by a variety of “omics” technologies. Clinical research and practice will advance from focusing on the “typical patient” to gaining a more sophisticated understanding of specific individuals and populations [ 124 ]. With this review, we underline the key areas where the domains of precision medicine ( Figure 3 ) can be implemented in cardiology diagnostics, stratification, therapeutics, and prognostics, and compare its novelty to the existing norm. Precision medicine empowers a physician to treat cardiac diseases individualistically, based on the patient’s unique genetic, metabolic, proteomic, or symptomatic profile. The strength of precision medicine lies in the synthesis and analysis of “data” that is rapidly changing from standard clinical, imaging, and laboratory testing to next-generation sequencing, metabolomics, and proteomic studies [ 8 ]. Modern-day cardiology is evolving to adopt new genetic, molecular, metabolic, and proteomic tools. In the case of myocardial infarction, newer biomarkers such as bFGF, hsCRP, hs Troponins, and miRNAs have emerged, which have great potential for detecting disease processes with more accuracy and at an earlier stage. Additionally, recent advances have shown that metabolites (such as acylcarnitines, fatty acids, BCAAs) are strong predictors of cardiovascular diseases and can be paired with standard metabolomics such as troponin and lipid levels to promptly predict the occurrence of MI/death in patients with heart disease. Similarly for heart failure, various markers such as 3-hydroxybutyrate, acetone, succinate 2-oxoglutarate, pseudouridine alanine, creatinine, proline, isoleucine, and leucine in plasma have shown usability for the prediction of outcome; various genes have also been identified that can serve the purpose of early risk stratification in the near future [ 125 ]. Despite its applicability challenges, genomics has contributed greatly to our understanding of the variability of disease processes, risk propensity, and response to treatment. Future advancements in genetic data generation and tools of application will enable its implementation in the routine management of common diseases. Next-generation sequencing and genome-wide association studies using a variety of computational biology technologies offer hope for improving the diagnosis and treatment of cardiovascular diseases. The mass spectrophotometric characterization of human cardiac proteins may expand the applicability of proteomics methods to CVD. Furthermore, transcriptomics approaches reveal novel information about gene expression, and metabolomics represents the tail end of multi-omics efforts to tackle CVDs early on. The “omics” can thus play a core role in the individualization of therapy in cardiac diseases [ 125 ].
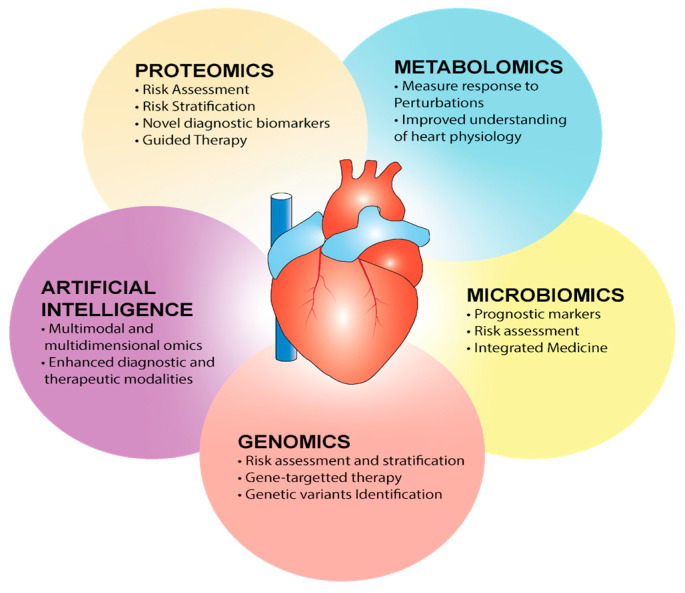
Domains of precision medicine in cardiology.
6. Evolving Understanding of the Immune Cells and the Future of Precision Cardiology
The rupture of atherosclerotic plaques appears to be the leading primary cause of CVD. Atherosclerosis, the leading cause of CVD, is a chronic inflammatory condition in which immuno-competent cells in lesions produce primarily pro-inflammatory cytokines. One key target for atherogenic immune responses is heat shock proteins, with other mediators being: pro-inflammatory cytokines, chemokines, and lipid mediators [ 126 ].
The evidence has evolved significantly in this domain, highlighting role of immune cells in various cardiac diseases. To cite an example, in the pathophysiology of heart failure, regulatory T cells (Tregs) play a role in immunoregulation and tissue healing. Tregs help the heart by limiting excessive inflammatory response and encouraging stable scar formation in the early stages of cardiac damage. However, Treg phenotypes and functions are altered in chronic heart failure by these cells being mutated into antiangiogenic and profibrotic cells. In addition, tumour necrosis factor (TNF)- and tumour necrosis factor receptor (TNFR1) expression rises in HF-activated CD4+ T cells. Immunotherapy for heart failure is now conceivable because of advances in next-generation sequencing and gene editing technologies [ 127 , 128 , 129 , 130 , 131 ].
The majority of pharmaceutical therapies have focused on changing hemodynamics (lowering afterload, regulating blood pressure and volume) or cardiac myocyte function. However, significant contributions of the immune system to normal cardiac function and damage response have lately emerged as attractive research fields. Therapeutic approaches that harness the strength of immune cells have the potential to open up new therapeutic pathways for various cardiac diseases, and these form important targets for providing individualized therapy by exploiting the “omics” and tailoring therapy in line with the immune makeup of the patients [ 126 , 131 , 132 ].
7. Challenges to PM in Cardiology
Various experts question the applicability and accessibility of precision medicine, believing that it lacks a global impact on cardiovascular disease management and will merely serve a small group of patients in the developed world, relegating its role to a selected niche only. However, this concern seems implausible due to the limited literature attesting to the validity of this claim [ 133 ]. Another challenge is the dearth of acceptability and neophobia to the growing methods both by the providers and the recipients. Precision medicine was historically considered complex, expensive, and inaccessible to underserved populations.
Genomics has undoubtedly accelerated the discovery of mutations underlying cardiac diseases. Exploring genetic sequences, assembly, and the identification of genes is still evolving and seems to have a promising future, although the technology needed to translate this data into clinical interpretation and practice is still challenging. While major research work is focused on the exome (protein-coding DNA), another area of interest in present-day genetic sequencing is the non-protein-coding DNA and its impact on major clinical diseases, which are largely under-discovered. Moreover, the research/development of testing for genetic variants associated with the risk of developing a certain cardiac disease and its role in prevention is encouraging, but affordability and feasibility remain a concern even in developed countries [ 133 , 134 ].
Another challenge to PM in cardiology is the education and training of the stakeholders, including the providers and the general public [ 135 , 136 ]. Education must be aimed at training to use an integrated system approach, allowing healthcare providers and patients to be in congruency to accept and trust the new evolving techniques [ 136 ]. An added challenge is the apparent lack of available cohorts with relevant phenotypes to demonstrate statistically meaningful associations. Moreover, the absence of a replication cohort and differences in epigenomic patterns also make research difficult.
8. Future Perspectives and Conclusions
Precision medicine is the future of medicine and holds promise for the more efficient management of cardiovascular diseases, owing to their gradual onset and heterogeneous, multimorbid, and chronic nature. The pathogenesis of these diseases may begin decades before any ultimate disease manifestation. Therefore, the use of precisely targeted tools for diagnosis and personalized treatment can revolutionize management by allowing the prevention, early diagnosis, and tailored treatment of cardiovascular diseases. Precision medicine is still an evolving field and many of the technologies needed for its implementation are in nascent stages. Moreover, the research and data on precision medicine are limited because of the ethical, social, legal, and economic issues, which may have produced an unavoidable bias in this review as well. This review explored the literature on precision medicine in cardiology and tried to outline and summarize the most clinically relevant sections of the evolving field. As we evolve in our capacity and infrastructure to employ tools exploring the genomics, proteomics, and metabolomics of cardiovascular diseases, we stand to see a future where a more precise therapy tailored to the needs, demands and limitations of an individual patient would no longer be a dream but a responsibility. The future of cardiology is here; we need to assimilate, adapt and make it more accessible by educating the providers about the evolving field and making infrastructure more equitable to the public.
Author Contributions
Conceptualization, Y.S., N.K. and N.P.; methodology, Y.S., N.P. and N.K.; software, N.P., Y.S. and N.K.; investigation, Y.S., N.P., O.K. and N.K.; data curation, N.K., N.P., Y.S. and O.K.; writing—original draft preparation, Y.S., N.K., N.P., O.K., J.K., A.M., A.G., H.C. and S.C.; writing—review and editing, Y.S., N.K., N.P., O.K., J.K. and A.M.; visualization, Y.S., N.K., N.P. and O.K.; supervision, Y.S., N.K., N.P., O.K. and A.M.; project administration, Y.S. and N.K.; funding acquisition, S.C. All authors have read and agreed to the published version of the manuscript.
Institutional Review Board Statement
Not applicable.
Informed Consent Statement
Data availability statement, conflicts of interest.
The authors declare no conflict of interest.
Funding Statement
This research was funded by University of Oradea.
Disclaimer/Publisher’s Note: The statements, opinions and data contained in all publications are solely those of the individual author(s) and contributor(s) and not of MDPI and/or the editor(s). MDPI and/or the editor(s) disclaim responsibility for any injury to people or property resulting from any ideas, methods, instructions or products referred to in the content.
- 1. Vos T., Allen C., Arora M., Barber R.M., Bhutta Z.A., Brown A., Carter A., Casey D.C., Charlson F.J., Chen A.Z., et al. Global, Regional, and National Incidence, Prevalence, and Years Lived with Disability for 310 Diseases and Injuries, 1990–2015: A Systematic Analysis for the Global Burden of Disease Study 2015. Lancet. 2016;388:1545–1602. doi: 10.1016/S0140-6736(16)31678-6. [ DOI ] [ PMC free article ] [ PubMed ] [ Google Scholar ]
- 2. Roth G.A., Johnson C.O., Abajobir A.A., Abd-Allah F., Abera S.F., Abyu G.Y., Ahmed M.B., Aksut B., Alam T., Alam K., et al. Global, Regional, and National Burden of Cardiovascular Diseases for 10 Causes, 1990 to 2015. J. Am. Coll. Cardiol. 2017;70:1–25. doi: 10.1016/j.jacc.2017.04.052. [ DOI ] [ PMC free article ] [ PubMed ] [ Google Scholar ]
- 3. Roth G.A., Mensah G.A., Johnson C.O., Addolorato G., Ammirati E., Baddour L.M., Barengo N.C., Beaton A.Z., Benjamin E.J., Benziger C.P., et al. Global Burden of Cardiovascular Diseases and Risk Factors, 1990–2019. J. Am. Coll. Cardiol. 2020;76:2982–3021. doi: 10.1016/j.jacc.2020.11.010. [ DOI ] [ PMC free article ] [ PubMed ] [ Google Scholar ]
- 4. WHO . WHO Top10 Causes of Death. WHO; Geneva, Switzerland: 2020. [ Google Scholar ]
- 5. CDC Health and Economic Costs of Chronic Diseases. [(accessed on 10 February 2023)]; Available online: https://www.cdc.gov/chronicdisease/about/costs/index.htm .
- 6. Masaebi F., Salehi M., Kazemi M., Vahabi N., Azizmohammad Looha M., Zayeri F. Trend Analysis of Disability Adjusted Life Years Due to Cardiovascular Diseases: Results from the Global Burden of Disease Study 2019. BMC Public Health. 2021;21:1268. doi: 10.1186/s12889-021-11348-w. [ DOI ] [ PMC free article ] [ PubMed ] [ Google Scholar ]
- 7. Krzyszczyk P., Acevedo A., Davidoff E.J., Timmins L.M., Marrero-Berrios I., Patel M., White C., Lowe C., Sherba J.J., Hartmanshenn C., et al. The Growing Role of Precision and Personalized Medicine for Cancer Treatment. Technology. 2018;06:79–100. doi: 10.1142/S2339547818300020. [ DOI ] [ PMC free article ] [ PubMed ] [ Google Scholar ]
- 8. Leopold J.A., Loscalzo J. Emerging Role of Precision Medicine in Cardiovascular Disease. Circ. Res. 2018;122:1302–1315. doi: 10.1161/CIRCRESAHA.117.310782. [ DOI ] [ PMC free article ] [ PubMed ] [ Google Scholar ]
- 9. Antman E.M., Loscalzo J. Precision Medicine in Cardiology. Nat. Rev. Cardiol. 2016;13:591–602. doi: 10.1038/nrcardio.2016.101. [ DOI ] [ PubMed ] [ Google Scholar ]
- 10. Currie G., Delles C. Precision Medicine and Personalized Medicine in Cardiovascular Disease. Adv. Exp. Med. Biol. 2018;1065:589–605. doi: 10.1007/978-3-319-77932-4_36. [ DOI ] [ PubMed ] [ Google Scholar ]
- 11. Krittanawong C., Zhang H., Wang Z., Aydar M., Kitai T. Artificial Intelligence in Precision Cardiovascular Medicine. J. Am. Coll. Cardiol. 2017;69:2657–2664. doi: 10.1016/j.jacc.2017.03.571. [ DOI ] [ PubMed ] [ Google Scholar ]
- 12. GALLONE G., BRUNO F., D’ASCENZO F., de Ferrari G.M. What Will We Ask to Artificial Intelligence for Cardiovascular Medicine in the next Decade? Minerva Cardiol. Angiol. 2022;70:92–101. doi: 10.23736/S2724-5683.21.05753-7. [ DOI ] [ PubMed ] [ Google Scholar ]
- 13. Simeon M., Dangwal S., Sachinidis A., Doss M.X. Application of the Pluripotent Stem Cells and Genomics in Cardiovascular Research-What We Have Learnt and Not Learnt until Now. Cells. 2021;10:3112. doi: 10.3390/cells10113112. [ DOI ] [ PMC free article ] [ PubMed ] [ Google Scholar ]
- 14. Pullamsetti S.S., Tello K., Seeger W. Utilising Biomarkers to Predict Right Heart Maladaptive Phenotype: A Step toward Precision Medicine. Eur. Respir. J. 2021;57:2004506. doi: 10.1183/13993003.04506-2020. [ DOI ] [ PubMed ] [ Google Scholar ]
- 15. Iqbal F., Lupieri A., Aikawa M., Aikawa E. Harnessing Single-Cell RNA Sequencing to Better Understand How Diseased Cells Behave the Way They Do in Cardiovascular Disease. Arterioscler. Thromb. Vasc. Biol. 2021;41:585. doi: 10.1161/ATVBAHA.120.314776. [ DOI ] [ PMC free article ] [ PubMed ] [ Google Scholar ]
- 16. Schiano C., Benincasa G., Franzese M., della Mura N., Pane K., Salvatore M., Napoli C. Epigenetic-Sensitive Pathways in Personalized Therapy of Major Cardiovascular Diseases. Pharmacol. Ther. 2020;210:107514. doi: 10.1016/j.pharmthera.2020.107514. [ DOI ] [ PubMed ] [ Google Scholar ]
- 17. Schulte C., Zeller T. Biomarkers in Primary Prevention. Herz. 2019;45:10–16. doi: 10.1007/s00059-019-04874-2. [ DOI ] [ PubMed ] [ Google Scholar ]
- 18. Gruson D., Bernardini S., Dabla P.K., Gouget B., Stankovic S. Collaborative AI and Laboratory Medicine Integration in Precision Cardiovascular Medicine. Clin. Chim. Acta. 2020;509:67–71. doi: 10.1016/j.cca.2020.06.001. [ DOI ] [ PubMed ] [ Google Scholar ]
- 19. Visco V., Ferruzzi G.J., Nicastro F., Virtuoso N., Carrizzo A., Galasso G., Vecchione C., Ciccarelli M. Artificial Intelligence as a Business Partner in Cardiovascular Precision Medicine: An Emerging Approach for Disease Detection and Treatment Optimization. Curr. Med. Chem. 2021;28:6569–6590. doi: 10.2174/0929867328666201218122633. [ DOI ] [ PubMed ] [ Google Scholar ]
- 20. AMBROSINI S., MOHAMMED S.A., COSTANTINO S., PANENI F. Disentangling the Epigenetic Landscape in Cardiovascular Patients: A Path toward Personalized Medicine. Minerva Cardiol. Angiol. 2021;69:331–345. doi: 10.23736/S2724-5683.20.05326-8. [ DOI ] [ PubMed ] [ Google Scholar ]
- 21. Pan Z., Ebert A., Liang P. Human-Induced Pluripotent Stem Cells as Models for Rare Cardiovascular Diseases: From Evidence-Based Medicine to Precision Medicine. Pflugers Arch. 2021;473:1151–1165. doi: 10.1007/s00424-020-02486-y. [ DOI ] [ PubMed ] [ Google Scholar ]
- 22. Gualandro D.M., Twerenbold R., Boeddinghaus J., Nestelberger T., Puelacher C., Müller C. Biomarkers in Cardiovascular Medicine: Towards Precision Medicine. Swiss Med. Wkly. 2019;149:w20125. doi: 10.4414/smw.2019.20125. [ DOI ] [ PubMed ] [ Google Scholar ]
- 23. Rodríguez-Carrio J., López P., Suárez A. EPC Dysfunction and Immune Networks: Translating Opportunities for Clinical Setting in Personalized Medicine. Curr. Med. Chem. 2018;25:4497–4506. doi: 10.2174/0929867324666170606101823. [ DOI ] [ PubMed ] [ Google Scholar ]
- 24. Spence J.D., Rayner B.L. Hypertension in Blacks. Hypertension. 2018;18:607–622. doi: 10.1161/HYPERTENSIONAHA.118.11064. [ DOI ] [ PubMed ] [ Google Scholar ]
- 25. Halushka P.V., Goodwin A.J., Halushka M.K. Opportunities for MicroRNAs in the Crowded Field of Cardiovascular Biomarkers. Annu. Rev. Pathol. Mech. Dis. 2019;14:211–238. doi: 10.1146/annurev-pathmechdis-012418-012827. [ DOI ] [ PMC free article ] [ PubMed ] [ Google Scholar ]
- 26. Zaiou M., el Amri H. Cardiovascular Pharmacogenetics: A Promise for Genomically-guided Therapy and Personalized Medicine. Clin. Genet. 2017;91:355–370. doi: 10.1111/cge.12881. [ DOI ] [ PubMed ] [ Google Scholar ]
- 27. MacRae C.A., Roden D.M., Loscalzo J. The Future of Cardiovascular Therapeutics. Circulation. 2016;133:2610–2617. doi: 10.1161/CIRCULATIONAHA.116.023555. [ DOI ] [ PubMed ] [ Google Scholar ]
- 28. Nishikimi T., Kuwahara K., Nakagawa Y., Kangawa K., Nakao K. Adrenomedullin in Cardiovascular Disease: A Useful Biomarker, Its Pathological Roles and Therapeutic Application. Curr. Protein. Pept. Sci. 2013;14:256–267. doi: 10.2174/13892037113149990045. [ DOI ] [ PubMed ] [ Google Scholar ]
- 29. Pollard T.J. THE ACUTE MYOCARDIAL INFARCTION. Prim. Care Clin. Off. Pract. 2000;27:631–649. doi: 10.1016/S0095-4543(05)70167-6. [ DOI ] [ PubMed ] [ Google Scholar ]
- 30. Mohan S., Lynch S., Cummings T.A. Time Equals Myocardium: Are We in Time? West Indian Med. J. 2010;59:680–685. [ PubMed ] [ Google Scholar ]
- 31. Andreotti F., Maggioni A.P., Scambia G. Sex- and Gender-specific Precision Medicine for Chronic Coronary Syndromes: Challenges and Opportunities. Kardiol. Pol. 2021;79:373–375. doi: 10.33963/KP.15948. [ DOI ] [ PubMed ] [ Google Scholar ]
- 32. Wen Z., Zhan J., Li H., Xu G., Ma S., Zhang J., Li Z., Ou C., Yang Z., Cai Y., et al. Dual-Ligand Supramolecular Nanofibers Inspired by the Renin-Angiotensin System for the Targeting and Synergistic Therapy of Myocardial Infarction. Theranostics. 2021;11:3725–3741. doi: 10.7150/thno.53644. [ DOI ] [ PMC free article ] [ PubMed ] [ Google Scholar ]
- 33. Tong G., Liang Y., Xue M., Chen X., Wang J., An N., Wang N., Chen Y., Wang Y., Jin L., et al. The Protective Role of BFGF in Myocardial Infarction and Hypoxia Cardiomyocytes by Reducing Oxidative Stress via Nrf2. Biochem. Biophys. Res. Commun. 2020;527:15–21. doi: 10.1016/j.bbrc.2020.04.053. [ DOI ] [ PubMed ] [ Google Scholar ]
- 34. Shrivastava A., Marzolla V., Weidmann H., Caprio M., Tregouet D.-A., Zeller T., Karakas M. Design and Rationale of the ERA-CVD Consortium PREMED-CAD—Precision Medicine in Coronary Artery Disease. Biomolecules. 2020;10:125. doi: 10.3390/biom10010125. [ DOI ] [ PMC free article ] [ PubMed ] [ Google Scholar ]
- 35. Crea F., Vergallo R. Plaque Erosion: Towards Precision Medicine in Acute Coronary Syndromes. Int. J. Cardiol. 2019;288:22–24. doi: 10.1016/j.ijcard.2019.04.058. [ DOI ] [ PubMed ] [ Google Scholar ]
- 36. Montone R.A., Russo M., Niccoli G. MINOCA: Current Perspectives. Aging. 2018;10:3044–3045. doi: 10.18632/aging.101618. [ DOI ] [ PMC free article ] [ PubMed ] [ Google Scholar ]
- 37. Mangion K., Gao H., Husmeier D., Luo X., Berry C. Advances in Computational Modelling for Personalised Medicine after Myocardial Infarction. Heart. 2018;104:550–557. doi: 10.1136/heartjnl-2017-311449. [ DOI ] [ PubMed ] [ Google Scholar ]
- 38. Pasea L., Chung S.-C., Pujades-Rodriguez M., Moayyeri A., Denaxas S., Fox K.A.A., Wallentin L., Pocock S.J., Timmis A., Banerjee A., et al. Personalising the Decision for Prolonged Dual Antiplatelet Therapy: Development, Validation and Potential Impact of Prognostic Models for Cardiovascular Events and Bleeding in Myocardial Infarction Survivors. Eur. Heart J. 2017;38:1048–1055. doi: 10.1093/eurheartj/ehw683. [ DOI ] [ PMC free article ] [ PubMed ] [ Google Scholar ]
- 39. Goretti E., Wagner D.R., Devaux Y. MiRNAs as Biomarkers of Myocardial Infarction: A Step Forward towards Personalized Medicine? Trends Mol. Med. 2014;20:716–725. doi: 10.1016/j.molmed.2014.10.006. [ DOI ] [ PubMed ] [ Google Scholar ]
- 40. Kaschina E., Lauer D., Schmerler P., Unger T., Steckelings U.M. AT2 Receptors Targeting Cardiac Protection Post-Myocardial Infarction. Curr. Hypertens Rep. 2014;16:441. doi: 10.1007/s11906-014-0441-0. [ DOI ] [ PubMed ] [ Google Scholar ]
- 41. Oni-Orisan A., Alsaleh N., Lee C.R., Seubert J.M. Epoxyeicosatrienoic Acids and Cardioprotection: The Road to Translation. J. Mol. Cell Cardiol. 2014;74:199–208. doi: 10.1016/j.yjmcc.2014.05.016. [ DOI ] [ PMC free article ] [ PubMed ] [ Google Scholar ]
- 42. Arenja N., Mueller C., Ehl N.F., Brinkert M., Roost K., Reichlin T., Sou S.M., Hochgruber T., Osswald S., Zellweger M.J. Prevalence, Extent, and Independent Predictors of Silent Myocardial Infarction. Am. J. Med. 2013;126:515–522. doi: 10.1016/j.amjmed.2012.11.028. [ DOI ] [ PubMed ] [ Google Scholar ]
- 43. Pereira N.L., Farkouh M.E., So D., Lennon R., Geller N., Mathew V., Bell M., Bae J.-H., Jeong M.H., Chavez I., et al. Effect of Genotype-Guided Oral P2Y12 Inhibitor Selection vs Conventional Clopidogrel Therapy on Ischemic Outcomes After Percutaneous Coronary Intervention. JAMA. 2020;324:761. doi: 10.1001/jama.2020.12443. [ DOI ] [ PMC free article ] [ PubMed ] [ Google Scholar ]
- 44. Claassens D.M.F., Vos G.J.A., Bergmeijer T.O., Hermanides R.S., van ’t Hof A.W.J., van der Harst P., Barbato E., Morisco C., Tjon Joe Gin R.M., Asselbergs F.W., et al. A Genotype-Guided Strategy for Oral P2Y12 Inhibitors in Primary PCI. N. Engl. J. Med. 2019;381:1621–1631. doi: 10.1056/NEJMoa1907096. [ DOI ] [ PubMed ] [ Google Scholar ]
- 45. Scott R.A., Freitag D.F., Li L., Chu A.Y., Surendran P., Young R., Grarup N., Stancáková A., Chen Y., Varga T.v., et al. A Genomic Approach to Therapeutic Target Validation Identifies a Glucose-Lowering GLP1R Variant Protective for Coronary Heart Disease. Sci. Transl. Med. 2016;8:341ra76. doi: 10.1126/scitranslmed.aad3744. [ DOI ] [ PMC free article ] [ PubMed ] [ Google Scholar ]
- 46. Tanzilli G., Arrivi A., Placanica A., Viceconte N., Cammisotto V., Nocella C., Barillà F., Torromeo C., Pucci G., Acconcia M.C., et al. Glutathione Infusion Before and 3 Days After Primary Angioplasty Blunts Ongoing NOX2-Mediated Inflammatory Response. J. Am. Heart Assoc. 2021;10:e020560. doi: 10.1161/JAHA.120.020560. [ DOI ] [ PMC free article ] [ PubMed ] [ Google Scholar ]
- 47. Bicciré F.G., Pastori D., Tanzilli A., Pignatelli P., Viceconte N., Barillà F., Versaci F., Gaudio C., Violi F., Tanzilli G. Low Serum Albumin Levels and In-Hospital Outcomes in Patients with ST Segment Elevation Myocardial Infarction. Nutr. Metab. Cardiovasc. Dis. 2021;31:2904–2911. doi: 10.1016/j.numecd.2021.06.003. [ DOI ] [ PubMed ] [ Google Scholar ]
- 48. Bhattacharya S., Granger C.B., Craig D., Haynes C., Bain J., Stevens R.D., Hauser E.R., Newgard C.B., Kraus W.E., Newby L.K., et al. Validation of the Association between a Branched Chain Amino Acid Metabolite Profile and Extremes of Coronary Artery Disease in Patients Referred for Cardiac Catheterization. Atherosclerosis. 2014;232:191–196. doi: 10.1016/j.atherosclerosis.2013.10.036. [ DOI ] [ PMC free article ] [ PubMed ] [ Google Scholar ]
- 49. Cavalu S., Popa A., Bratu I., Borodi G., Maghiar A. New Evidences of Key Factors Involved in “Silent Stones” Etiopathogenesis and Trace Elements: Microscopic, Spectroscopic, and Biochemical Approach. Biol. Trace Elem. Res. 2015;168:311–320. doi: 10.1007/s12011-015-0361-0. [ DOI ] [ PubMed ] [ Google Scholar ]
- 50. Shah R.V., Truong Q.A., Gaggin H.K., Pfannkuche J., Hartmann O., Januzzi J.L. Mid-Regional pro-Atrial Natriuretic Peptide and pro-Adrenomedullin Testing for the Diagnostic and Prognostic Evaluation of Patients with Acute Dyspnoea. Eur. Heart J. 2012;33:2197–2205. doi: 10.1093/eurheartj/ehs136. [ DOI ] [ PMC free article ] [ PubMed ] [ Google Scholar ]
- 51. Cavalu S., Damian G., Dansoreanu M. EPR study of non-covalent spin labeled serum albumin and hemoglobin. Biophys. Chem. 2002;99:181–188. doi: 10.1016/S0301-4622(02)00182-5. [ DOI ] [ PubMed ] [ Google Scholar ]
- 52. WHO Global Health Observatory (GHO) Data World Health Organization. [(accessed on 10 February 2023)]. Available online: https://www.who.int/data/gho .
- 53. Mozaffarian D., Benjamin E.J., Go A.S., Arnett D.K., Blaha M.J., Cushman M., Das S.R., de Ferranti S., Després J.-P., Fullerton H.J., et al. Heart Disease and Stroke Statistics—2016 Update. Circulation. 2016;133:e38–e360. doi: 10.1161/CIR.0000000000000350. [ DOI ] [ PubMed ] [ Google Scholar ]
- 54. Research Councils UK. King’s College London Ancestry and Biological Informative Markers for Stratification of HYpertension: The AIM HY Study. [(accessed on 10 February 2023)]. Available online: https://gtr.ukri.org/projects?ref=MR%2FM016560%2F1 .
- 55. Rydberg D.M., Mejyr S., Loikas D., Schenck-Gustafsson K., von Euler M., Malmström R.E. Sex Differences in Spontaneous Reports on Adverse Drug Events for Common Antihypertensive Drugs. Eur. J. Clin. Pharmacol. 2018;74:1165–1173. doi: 10.1007/s00228-018-2480-y. [ DOI ] [ PMC free article ] [ PubMed ] [ Google Scholar ]
- 56. Gong Y., Wang Z., Beitelshees A.L., McDonough C.W., Langaee T.Y., Hall K., Schmidt S.O.F., Curry R.W., Gums J.G., Bailey K.R., et al. Pharmacogenomic Genome-Wide Meta-Analysis of Blood Pressure Response to β-Blockers in Hypertensive African Americans. Hypertension. 2016;67:556–563. doi: 10.1161/HYPERTENSIONAHA.115.06345. [ DOI ] [ PMC free article ] [ PubMed ] [ Google Scholar ]
- 57. Zhao Y., Peng J., Lu C., Hsin M., Mura M., Wu L., Chu L., Zamel R., Machuca T., Waddell T., et al. Metabolomic Heterogeneity of Pulmonary Arterial Hypertension. PLoS ONE. 2014;9:e88727. doi: 10.1371/journal.pone.0088727. [ DOI ] [ PMC free article ] [ PubMed ] [ Google Scholar ]
- 58. Brewster L.M., Seedat Y.K. Why Do Hypertensive Patients of African Ancestry Respond Better to Calciumblockers and Diuretics than to ACE Inhibitors and β-Adrenergic Blockers? Asystematic Review. BMC Med. 2013;11:141. doi: 10.1186/1741-7015-11-141. [ DOI ] [ PMC free article ] [ PubMed ] [ Google Scholar ]
- 59. Wikoff W.R., Frye R.F., Zhu H., Gong Y., Boyle S., Churchill E., Cooper-Dehoff R.M., Beitelshees A.L., Chapman A.B., Fiehn O., et al. Pharmacometabolomics Reveals Racial Differences in Response to Atenolol Treatment. PLoS ONE. 2013;8:e57639. doi: 10.1371/journal.pone.0057639. [ DOI ] [ PMC free article ] [ PubMed ] [ Google Scholar ]
- 60. Loganathan L., Gopinath K., Sankaranarayanan V.M., Kukreti R., Rajendran K., Lee J.-K., Muthusamy K. Computational and Pharmacogenomic Insights on Hypertension Treatment: Rational Drug Design and Optimization Strategies. Curr. Drug Targets. 2019;21:18–33. doi: 10.2174/1389450120666190808101356. [ DOI ] [ PubMed ] [ Google Scholar ]
- 61. Kronish I.M., Cheung Y.K., Shimbo D., Julian J., Gallagher B., Parsons F., Davidson K.W. Increasing the Precision of Hypertension Treatment Through Personalized Trials: A Pilot Study. J. Gen. Intern. Med. 2019;34:839–845. doi: 10.1007/s11606-019-04831-z. [ DOI ] [ PMC free article ] [ PubMed ] [ Google Scholar ]
- 62. Hoh B.-P., Abdul Rahman T., Yusoff K. Natural Selection and Local Adaptation of Blood Pressure Regulation and Their Perspectives on Precision Medicine in Hypertension. Hereditas. 2019;156:1. doi: 10.1186/s41065-019-0080-1. [ DOI ] [ PMC free article ] [ PubMed ] [ Google Scholar ]
- 63. Kokubo Y., Padmanabhan S., Iwashima Y., Yamagishi K., Goto A. Gene and Environmental Interactions According to the Components of Lifestyle Modifications in Hypertension Guidelines. Environ. Health Prev. Med. 2019;24:19. doi: 10.1186/s12199-019-0771-2. [ DOI ] [ PMC free article ] [ PubMed ] [ Google Scholar ]
- 64. Evangelou E., Warren H.R., Mosen-Ansorena D., Mifsud B., Pazoki R., Gao H., Ntritsos G., Dimou N., Cabrera C.P., Karaman I., et al. Genetic Analysis of over 1 Million People Identifies 535 New Loci Associated with Blood Pressure Traits. Nat. Genet. 2018;50:1412–1425. doi: 10.1038/s41588-018-0205-x. [ DOI ] [ PMC free article ] [ PubMed ] [ Google Scholar ]
- 65. Mattson D.L., Liang M. From GWAS to Functional Genomics-Based Precision Medicine. Nat. Rev. Nephrol. 2017;13:195–196. doi: 10.1038/nrneph.2017.21. [ DOI ] [ PMC free article ] [ PubMed ] [ Google Scholar ]
- 66. Surendran P., Drenos F., Young R., Warren H., Cook J.P., Manning A.K., Grarup N., Sim X., Barnes D.R., Witkowska K., et al. Trans-Ancestry Meta-Analyses Identify Rare and Common Variants Associated with Blood Pressure and Hypertension. Nat. Genet. 2016;48:1151–1161. doi: 10.1038/ng.3654. [ DOI ] [ PMC free article ] [ PubMed ] [ Google Scholar ]
- 67. Zhang W. Epigenetics of Epithelial Na + Channel-Dependent Sodium Uptake and Blood Pressure Regulation. World J. Nephrol. 2015;4:363. doi: 10.5527/wjn.v4.i3.363. [ DOI ] [ PMC free article ] [ PubMed ] [ Google Scholar ]
- 68. Chittani M., Zaninello R., Lanzani C., Frau F., Ortu M.F., Salvi E., Fresu G., Citterio L., Braga D., Piras D.A., et al. TET2 and CSMD1 Genes Affect SBP Response to Hydrochlorothiazide in Never-Treated Essential Hypertensives. J. Hypertens. 2015;33:1301–1309. doi: 10.1097/HJH.0000000000000541. [ DOI ] [ PMC free article ] [ PubMed ] [ Google Scholar ]
- 69. Trudu M., Janas S., Lanzani C., Debaix H., Schaeffer C., Ikehata M., Citterio L., Demaretz S., Trevisani F., Ristagno G., et al. Common Noncoding UMOD Gene Variants Induce Salt-Sensitive Hypertension and Kidney Damage by Increasing Uromodulin Expression. Nat. Med. 2013;19:1655–1660. doi: 10.1038/nm.3384. [ DOI ] [ PMC free article ] [ PubMed ] [ Google Scholar ]
- 70. Muskalla A.M., Suter P.M., Saur M., Nowak A., Hersberger M., Krayenbuehl P.-A. G-Protein Receptor Kinase 4 Polymorphism and Response to Antihypertensive Therapy. Clin. Chem. 2014;60:1543–1548. doi: 10.1373/clinchem.2014.226605. [ DOI ] [ PubMed ] [ Google Scholar ]
- 71. Choi H.D., Suh J.H., Lee J.Y., Bae S.K., Kang H.E., Lee M.G., Shin W.G. Effects of ACE and ADD1 Gene Polymorphisms on Blood Pressure Response to Hydrochlorothiazide: A Meta-Analysis. Int. J. Clin. Pharmacol. Ther. 2013;51:718–724. doi: 10.5414/CP201899. [ DOI ] [ PubMed ] [ Google Scholar ]
- 72. Duarte J.D., Turner S.T., Tran B., Chapman A.B., Bailey K.R., Gong Y., Gums J.G., Langaee T.Y., Beitelshees A.L., Cooper-Dehoff R.M., et al. Association of Chromosome 12 Locus with Antihypertensive Response to Hydrochlorothiazide May Involve Differential YEATS4 Expression. Pharm. J. 2013;13:257–263. doi: 10.1038/tpj.2012.4. [ DOI ] [ PMC free article ] [ PubMed ] [ Google Scholar ]
- 73. Bazzell B.G., Rainey W.E., Auchus R.J., Zocco D., Bruttini M., Hummel S.L., Byrd J.B. Human Urinary MRNA as a Biomarker of Cardiovascular Disease. Circ. Genom. Precis. Med. 2018;11:e002213. doi: 10.1161/CIRCGEN.118.002213. [ DOI ] [ PMC free article ] [ PubMed ] [ Google Scholar ]
- 74. Arnett D.K., Davis B.R., Ford C.E., Boerwinkle E., Leiendecker-Foster C., Miller M.B., Black H., Eckfeldt J.H. Pharmacogenetic Association of the Angiotensin-Converting Enzyme Insertion/Deletion Polymorphism on Blood Pressure and Cardiovascular Risk in Relation to Antihypertensive Treatment. Circulation. 2005;111:3374–3383. doi: 10.1161/CIRCULATIONAHA.104.504639. [ DOI ] [ PubMed ] [ Google Scholar ]
- 75. Turner S.T., Bailey K.R., Fridley B.L., Chapman A.B., Schwartz G.L., Chai H.S., Sicotte H., Kocher J.-P., Rodin A.S., Boerwinkle E. Genomic Association Analysis Suggests Chromosome 12 Locus Influencing Antihypertensive Response to Thiazide Diuretic. Hypertension. 2008;52:359–365. doi: 10.1161/HYPERTENSIONAHA.107.104273. [ DOI ] [ PMC free article ] [ PubMed ] [ Google Scholar ]
- 76. LIU J., LIU Z., YU B., XU F., MO W., ZHOU G., LIU Y., LI Q., ZHOU H. B1-Adrenergic Receptor Polymorphisms Influence the Response to Metoprolol Monotherapy in Patients with Essential Hypertension. Clin. Pharmacol. Ther. 2006;80:23–32. doi: 10.1016/j.clpt.2006.03.004. [ DOI ] [ PubMed ] [ Google Scholar ]
- 77. Ganesh S.K., Tragante V., Guo W., Guo Y., Lanktree M.B., Smith E.N., Johnson T., Castillo B.A., Barnard J., Baumert J., et al. Loci Influencing Blood Pressure Identified Using a Cardiovascular Gene-Centric Array. Hum. Mol. Genet. 2013;22:1663–1678. doi: 10.1093/hmg/dds555. [ DOI ] [ PMC free article ] [ PubMed ] [ Google Scholar ]
- 78. Rimpelä J.M., Kontula K.K., Fyhrquist F., Donner K.M., Tuiskula A.M., Sarin A.P., Mohney R.P., Stirdivant S.M., Hiltunen T.P. Replicated evidence for aminoacylase 3 and nephrin gene variations to predict antihypertensive drug responses. Pharmacogenomics. 2017;18:445–458. doi: 10.2217/pgs-2016-0204. [ DOI ] [ PubMed ] [ Google Scholar ]
- 79. Ferrandi M., Molinari I., Torielli L., Padoani G., Salardi S., Rastaldi M.P., Ferrari P., Bianchi G. Adducin- and Ouabain-Related Gene Variants Predict the Antihypertensive Activity of Rostafuroxin, Part 1: Experimental Studies. Sci. Transl. Med. 2010;2:e01815. doi: 10.1126/scitranslmed.3001815. [ DOI ] [ PubMed ] [ Google Scholar ]
- 80. Horigan G., McNulty H., Ward M., Strain J., Purvis J., Scott J.M. Riboflavin Lowers Blood Pressure in Cardiovascular Disease Patients Homozygous for the 677C→T Polymorphism in MTHFR. J. Hypertens. 2010;28:478–486. doi: 10.1097/HJH.0b013e328334c126. [ DOI ] [ PubMed ] [ Google Scholar ]
- 81. Alderman M. Plasma Renin Activity Levels in Hypertensive Persons: Their Wide Range and Lack of Suppression in Diabetic and in Most Elderly Patients. Am. J. Hypertens. 2004;17:1–7. doi: 10.1016/j.amjhyper.2003.08.015. [ DOI ] [ PubMed ] [ Google Scholar ]
- 82. Egan B.M., Basile J.N., Rehman S.U., Davis P.B., Grob C.H., Riehle J.F., Walters C.A., Lackland D.T., Merali C., Sealey J.E., et al. Plasma Renin Test-Guided Drug Treatment Algorithm for Correcting Patients With Treated but Uncontrolled Hypertension: A Randomized Controlled Trial. Am. J. Hypertens. 2009;22:792–801. doi: 10.1038/ajh.2009.63. [ DOI ] [ PubMed ] [ Google Scholar ]
- 83. Dumeny L., Vardeny O., Edelmann F., Pieske B., Duarte J.D., Cavallari L.H. NR3C2 Genotype Is Associated with Response to Spironolactone in Diastolic Heart Failure Patients from the Aldo-DHF Trial. Pharmacother. J. Hum. Pharmacol. Drug Ther. 2021;41:978–987. doi: 10.1002/phar.2626. [ DOI ] [ PMC free article ] [ PubMed ] [ Google Scholar ]
- 84. Maurer M.S., Schwartz J.H., Gundapaneni B., Elliott P.M., Merlini G., Waddington-Cruz M., Kristen A.V., Grogan M., Witteles R., Damy T., et al. Tafamidis Treatment for Patients with Transthyretin Amyloid Cardiomyopathy. N. Engl. J. Med. 2018;379:1007–1016. doi: 10.1056/NEJMoa1805689. [ DOI ] [ PubMed ] [ Google Scholar ]
- 85. Dominiczak A., Delles C., Padmanabhan S. Genomics and Precision Medicine for Clinicians and Scientists in Hypertension. Hypertension. 2017;69:e08252. doi: 10.1161/HYPERTENSIONAHA.116.08252. [ DOI ] [ PubMed ] [ Google Scholar ]
- 86. Núñez J., Llàcer P., García-Blas S., Bonanad C., Ventura S., Núñez J.M., Sánchez R., Fácila L., de la Espriella R., Vaquer J.M., et al. CA125-Guided Diuretic Treatment Versus Usual Care in Patients With Acute Heart Failure and Renal Dysfunction. Am. J. Med. 2020;133:370–380.e4. doi: 10.1016/j.amjmed.2019.07.041. [ DOI ] [ PubMed ] [ Google Scholar ]
- 87. Pellicori P., Ferreira J.P., Mariottoni B., Brunner-La Rocca H., Ahmed F.Z., Verdonschot J., Collier T., Cuthbert J.J., Petutschnigg J., Mujaj B., et al. Effects of Spironolactone on Serum Markers of Fibrosis in People at High Risk of Developing Heart Failure: Rationale, Design and Baseline Characteristics of a Proof-of-concept, Randomised, Precision-medicine, Prevention Trial. The Heart OMics in AGing (HOMAGE) Trial. Eur. J. Heart Fail. 2020;22:1711–1723. doi: 10.1002/ejhf.1716. [ DOI ] [ PubMed ] [ Google Scholar ]
- 88. Feng S.-D., Jiang Y., Lin Z.-H., Lin P.-H., Lin S.-M., Liu Q.-C. Diagnostic Value of Brain Natriuretic Peptide and β-Endorphin Plasma Concentration Changes in Patients with Acute Left Heart Failure and Atrial Fibrillation. Medicine. 2017;96:e7526. doi: 10.1097/MD.0000000000007526. [ DOI ] [ PMC free article ] [ PubMed ] [ Google Scholar ]
- 89. Drum C.L., Tan W.K.Y., Chan S., Pakkiri L.S., Chong J.P.C., Liew O., Ng T., Ling L., Sim D., Leong K.G., et al. Thymosin Beta-4 Is Elevated in Women With Heart Failure With Preserved Ejection Fraction. J. Am. Heart Assoc. 2017;6:05586. doi: 10.1161/JAHA.117.005586. [ DOI ] [ PMC free article ] [ PubMed ] [ Google Scholar ]
- 90. Felker G.M., Mentz R.J., Teerlink J.R., Voors A.A., Pang P.S., Ponikowski P., Greenberg B.H., Filippatos G., Davison B.A., Cotter G., et al. Serial High Sensitivity Cardiac Troponin T Measurement in Acute Heart Failure: Insights from the RELAX-AHF Study. Eur. J. Heart Fail. 2015;17:1262–1270. doi: 10.1002/ejhf.341. [ DOI ] [ PubMed ] [ Google Scholar ]
- 91. Pozsonyi Z., Förhécz Z., Gombos T., Karádi I., Jánoskuti L., Prohászka Z. Copeptin (C-Terminal pro Arginine-Vasopressin) Is an Independent Long-Term Prognostic Marker in Heart Failure with Reduced Ejection Fraction. Heart Lung Circ. 2015;24:359–367. doi: 10.1016/j.hlc.2014.10.008. [ DOI ] [ PubMed ] [ Google Scholar ]
- 92. Glick D., deFilippi C.R., Christenson R., Gottdiener J.S., Seliger S.L. Long-Term Trajectory of Two Unique Cardiac Biomarkers and Subsequent Left Ventricular Structural Pathology and Risk of Incident Heart Failure in Community-Dwelling Older Adults at Low Baseline Risk. JACC Heart Fail. 2013;1:353–360. doi: 10.1016/j.jchf.2013.04.007. [ DOI ] [ PMC free article ] [ PubMed ] [ Google Scholar ]
- 93. Gaggin H.K., Motiwala S., Bhardwaj A., Parks K.A., Januzzi J.L. Soluble Concentrations of the Interleukin Receptor Family Member ST2 and β-Blocker Therapy in Chronic Heart Failure. Circ. Heart Fail. 2013;6:1206–1213. doi: 10.1161/CIRCHEARTFAILURE.113.000457. [ DOI ] [ PubMed ] [ Google Scholar ]
- 94. Lok S.I., Winkens B., Goldschmeding R., van Geffen A.J.P., Nous F.M.A., van Kuik J., van der Weide P., Klöpping C., Kirkels J.H., Lahpor J.R., et al. Circulating Growth Differentiation Factor-15 Correlates with Myocardial Fibrosis in Patients with Non-Ischaemic Dilated Cardiomyopathy and Decreases Rapidly after Left Ventricular Assist Device Support. Eur. J. Heart Fail. 2012;14:1249–1256. doi: 10.1093/eurjhf/hfs120. [ DOI ] [ PubMed ] [ Google Scholar ]
- 95. Lopez-Andrès N., Rossignol P., Iraqi W., Fay R., Nuée J., Ghio S., Cleland J.G.F., Zannad F., Lacolley P. Association of Galectin-3 and Fibrosis Markers with Long-Term Cardiovascular Outcomes in Patients with Heart Failure, Left Ventricular Dysfunction, and Dyssynchrony: Insights from the CARE-HF (Cardiac Resynchronization in Heart Failure) Trial. Eur. J. Heart Fail. 2012;14:74–81. doi: 10.1093/eurjhf/hfr151. [ DOI ] [ PubMed ] [ Google Scholar ]
- 96. Olivotto I., Oreziak A., Barriales-Villa R., Abraham T.P., Masri A., Garcia-Pavia P., Saberi S., Lakdawala N.K., Wheeler M.T., Owens A., et al. Mavacamten for Treatment of Symptomatic Obstructive Hypertrophic Cardiomyopathy (EXPLORER-HCM): A Randomised, Double-Blind, Placebo-Controlled, Phase 3 Trial. Lancet. 2020;396:759–769. doi: 10.1016/S0140-6736(20)31792-X. [ DOI ] [ PubMed ] [ Google Scholar ]
- 97. Hunter W.G., Kelly J.P., McGarrah R.W., Khouri M.G., Craig D., Haynes C., Ilkayeva O., Stevens R.D., Bain J.R., Muehlbauer M.J., et al. Metabolomic Profiling Identifies Novel Circulating Biomarkers of Mitochondrial Dysfunction Differentially Elevated in Heart Failure With Preserved Versus Reduced Ejection Fraction: Evidence for Shared Metabolic Impairments in Clinical Heart Failure. J. Am. Heart Assoc. 2016;5:e03190. doi: 10.1161/JAHA.115.003190. [ DOI ] [ PMC free article ] [ PubMed ] [ Google Scholar ]
- 98. Du Z., Shen A., Huang Y., Su L., Lai W., Wang P., Xie Z., Xie Z., Zeng Q., Ren H., et al. 1H-NMR-Based Metabolic Analysis of Human Serum Reveals Novel Markers of Myocardial Energy Expenditure in Heart Failure Patients. PLoS ONE. 2014;9:e88102. doi: 10.1371/journal.pone.0088102. [ DOI ] [ PMC free article ] [ PubMed ] [ Google Scholar ]
- 99. Wang J., Li Z., Chen J., Zhao H., Luo L., Chen C., Xu X., Zhang W., Gao K., Li B., et al. Metabolomic Identification of Diagnostic Plasma Biomarkers in Humans with Chronic Heart Failure. Mol. Biosyst. 2013;9:2618. doi: 10.1039/c3mb70227h. [ DOI ] [ PubMed ] [ Google Scholar ]
- 100. Desmoulin F., Galinier M., Trouillet C., Berry M., Delmas C., Turkieh A., Massabuau P., Taegtmeyer H., Smih F., Rouet P. Metabonomics Analysis of Plasma Reveals the Lactate to Cholesterol Ratio as an Independent Prognostic Factor of Short-Term Mortality in Acute Heart Failure. PLoS ONE. 2013;8:e60737. doi: 10.1371/journal.pone.0060737. [ DOI ] [ PMC free article ] [ PubMed ] [ Google Scholar ]
- 101. Tang W.H.W., Wang Z., Fan Y., Levison B., Hazen J.E., Donahue L.M., Wu Y., Hazen S.L. Prognostic Value of Elevated Levels of Intestinal Microbe-Generated Metabolite Trimethylamine-N-Oxide in Patients With Heart Failure. J. Am. Coll. Cardiol. 2014;64:1908–1914. doi: 10.1016/j.jacc.2014.02.617. [ DOI ] [ PMC free article ] [ PubMed ] [ Google Scholar ]
- 102. Domínguez F., Cuenca S., Bilińska Z., Toro R., Villard E., Barriales-Villa R., Ochoa J.P., Asselbergs F., Sammani A., Franaszczyk M., et al. Dilated Cardiomyopathy Due to BLC2-Associated Athanogene 3 (BAG3) Mutations. J. Am. Coll. Cardiol. 2018;72:2471–2481. doi: 10.1016/j.jacc.2018.08.2181. [ DOI ] [ PMC free article ] [ PubMed ] [ Google Scholar ]
- 103. Shah S., Henry A., Roselli C., Lin H., Sveinbjörnsson G., Fatemifar G., Hedman Å.K., Wilk J.B., Morley M.P., Chaffin M.D., et al. Genome-Wide Association and Mendelian Randomisation Analysis Provide Insights into the Pathogenesis of Heart Failure. Nat. Commun. 2020;11:163. doi: 10.1038/s41467-019-13690-5. [ DOI ] [ PMC free article ] [ PubMed ] [ Google Scholar ]
- 104. Albert C.L., Tang W.H.W. Metabolic Biomarkers in Heart Failure. Heart Fail. Clin. 2018;14:109–118. doi: 10.1016/j.hfc.2017.08.011. [ DOI ] [ PMC free article ] [ PubMed ] [ Google Scholar ]
- 105. Ahmad T., Voora D., Becker R.C. The Pharmacogenetics of Antiplatelet Agents: Towards Personalized Therapy? Nat. Rev. Cardiol. 2011;8:560–571. doi: 10.1038/nrcardio.2011.111. [ DOI ] [ PubMed ] [ Google Scholar ]
- 106. Biddinger A., Rocklin M., Coselli J., Milewicz D.M. Familial Thoracic Aortic Dilatations and Dissections: A Case Control Study. J. Vasc. Surg. 1997;25:506–511. doi: 10.1016/S0741-5214(97)70261-1. [ DOI ] [ PubMed ] [ Google Scholar ]
- 107. Brownstein A., Kostiuk V., Ziganshin B., Zafar M., Kuivaniemi H., Body S., Bale A., Elefteriades J. Genes Associated with Thoracic Aortic Aneurysm and Dissection: 2018 Update and Clinical Implications. AORTA. 2018;06:013–020. doi: 10.1055/s-0038-1639612. [ DOI ] [ PMC free article ] [ PubMed ] [ Google Scholar ]
- 108. Renard M., Francis C., Ghosh R., Scott A.F., Witmer P.D., Adès L.C., Andelfinger G.U., Arnaud P., Boileau C., Callewaert B.L., et al. Clinical Validity of Genes for Heritable Thoracic Aortic Aneurysm and Dissection. J. Am. Coll. Cardiol. 2018;72:605–615. doi: 10.1016/j.jacc.2018.04.089. [ DOI ] [ PMC free article ] [ PubMed ] [ Google Scholar ]
- 109. Wolford B.N., Hornsby W.E., Guo D., Zhou W., Lin M., Farhat L., McNamara J., Driscoll A., Wu X., Schmidt E.M., et al. Clinical Implications of Identifying Pathogenic Variants in Individuals With Thoracic Aortic Dissection. Circ. Genom. Precis. Med. 2019;12:e02476. doi: 10.1161/CIRCGEN.118.002476. [ DOI ] [ PMC free article ] [ PubMed ] [ Google Scholar ]
- 110. Johnson K.B., Wei W., Weeraratne D., Frisse M.E., Misulis K., Rhee K., Zhao J., Snowdon J.L. Precision Medicine, AI, and the Future of Personalized Health Care. Clin. Transl. Sci. 2021;14:86–93. doi: 10.1111/cts.12884. [ DOI ] [ PMC free article ] [ PubMed ] [ Google Scholar ]
- 111. Sethi Y., Patel N., Kaka N., Desai A., Kaiwan O., Sheth M., Sharma R., Huang H., Chopra H., Khandaker M.U., et al. Artificial Intelligence in Pediatric Cardiology: A Scoping Review. J. Clin. Med. 2022;11:7072. doi: 10.3390/jcm11237072. [ DOI ] [ PMC free article ] [ PubMed ] [ Google Scholar ]
- 112. de Marvao A., Dawes T.J., Howard J.P., O’Regan D.P. Artificial Intelligence and the Cardiologist: What You Need to Know for 2020. Heart. 2020;106:399–400. doi: 10.1136/heartjnl-2019-316033. [ DOI ] [ PMC free article ] [ PubMed ] [ Google Scholar ]
- 113. Johnson K.W., Torres Soto J., Glicksberg B.S., Shameer K., Miotto R., Ali M., Ashley E., Dudley J.T. Artificial Intelligence in Cardiology. J. Am. Coll Cardiol. 2018;71:2668–2679. doi: 10.1016/j.jacc.2018.03.521. [ DOI ] [ PubMed ] [ Google Scholar ]
- 114. Estimation of the Warfarin Dose with Clinical and Pharmacogenetic Data. N. Engl. J. Med. 2009;360:753–764. doi: 10.1056/NEJMoa0809329. [ DOI ] [ PMC free article ] [ PubMed ] [ Google Scholar ]
- 115. Jorgensen A.L., FitzGerald R.J., Oyee J., Pirmohamed M., Williamson P.R. Influence of CYP2C9 and VKORC1 on Patient Response to Warfarin: A Systematic Review and Meta-Analysis. PLoS ONE. 2012;7:e44064. doi: 10.1371/journal.pone.0044064. [ DOI ] [ PMC free article ] [ PubMed ] [ Google Scholar ]
- 116. Cooper G.M., Johnson J.A., Langaee T.Y., Feng H., Stanaway I.B., Schwarz U.I., Ritchie M.D., Stein C.M., Roden D.M., Smith J.D., et al. A Genome-Wide Scan for Common Genetic Variants with a Large Influence on Warfarin Maintenance Dose. Blood. 2008;112:1022–1027. doi: 10.1182/blood-2008-01-134247. [ DOI ] [ PMC free article ] [ PubMed ] [ Google Scholar ]
- 117. Takeuchi F., McGinnis R., Bourgeois S., Barnes C., Eriksson N., Soranzo N., Whittaker P., Ranganath V., Kumanduri V., McLaren W., et al. A Genome-Wide Association Study Confirms VKORC1, CYP2C9, and CYP4F2 as Principal Genetic Determinants of Warfarin Dose. PLoS Genet. 2009;5:e1000433. doi: 10.1371/journal.pgen.1000433. [ DOI ] [ PMC free article ] [ PubMed ] [ Google Scholar ]
- 118. Oqueli E., Hiscock M., Dick R. Clopidogrel Resistance. Heart Lung Circ. 2007;16:S17–S28. doi: 10.1016/j.hlc.2007.03.012. [ DOI ] [ PubMed ] [ Google Scholar ]
- 119. Collet J.-P., Hulot J.-S., Pena A., Villard E., Esteve J.-B., Silvain J., Payot L., Brugier D., Cayla G., Beygui F., et al. Cytochrome P450 2C19 Polymorphism in Young Patients Treated with Clopidogrel after Myocardial Infarction: A Cohort Study. Lancet. 2009;373:309–317. doi: 10.1016/S0140-6736(08)61845-0. [ DOI ] [ PubMed ] [ Google Scholar ]
- 120. Mega J.L., Hochholzer W., Frelinger A.L., Kluk M.J., Angiolillo D.J., Kereiakes D.J., Isserman S., Rogers W.J., Ruff C.T., Contant C., et al. Dosing Clopidogrel Based on CYP2C19 Genotype and the Effect on Platelet Reactivity in Patients With Stable Cardiovascular Disease. JAMA. 2011;306:2221–2228. doi: 10.1001/jama.2011.1703. [ DOI ] [ PubMed ] [ Google Scholar ]
- 121. Kimmel S.E., French B., Kasner S.E., Johnson J.A., Anderson J.L., Gage B.F., Rosenberg Y.D., Eby C.S., Madigan R.A., McBane R.B., et al. A Pharmacogenetic versus a Clinical Algorithm for Warfarin Dosing. N. Engl. J. Med. 2013;369:2283–2293. doi: 10.1056/NEJMoa1310669. [ DOI ] [ PMC free article ] [ PubMed ] [ Google Scholar ]
- 122. Pirmohamed M., Burnside G., Eriksson N., Jorgensen A.L., Toh C.H., Nicholson T., Kesteven P., Christersson C., Wahlström B., Stafberg C., et al. A Randomized Trial of Genotype-Guided Dosing of Warfarin. N. Engl. J. Med. 2013;369:2294–2303. doi: 10.1056/NEJMoa1311386. [ DOI ] [ PubMed ] [ Google Scholar ]
- 123. Garmaroudi F.S., Handy D.E., Liu Y.-Y., Loscalzo J. Systems Pharmacology and Rational Polypharmacy: Nitric Oxide−Cyclic GMP Signaling Pathway as an Illustrative Example and Derivation of the General Case. PLoS Comput. Biol. 2016;12:e1004822. doi: 10.1371/journal.pcbi.1004822. [ DOI ] [ PMC free article ] [ PubMed ] [ Google Scholar ]
- 124. Lau E., Wu J.C. Omics, Big Data, and Precision Medicine in Cardiovascular Sciences. Circ. Res. 2018;122:1165–1168. doi: 10.1161/CIRCRESAHA.118.313161. [ DOI ] [ PMC free article ] [ PubMed ] [ Google Scholar ]
- 125. Sohag M.M.H., Raqib S.M., Akhmad S.A. OMICS Approaches in Cardiovascular Diseases: A Mini Review. Genom. Inform. 2021;19:e13. doi: 10.5808/gi.21002. [ DOI ] [ PMC free article ] [ PubMed ] [ Google Scholar ]
- 126. Frostegård J. Immunity, Atherosclerosis and Cardiovascular Disease. BMC Med. 2013;11:117. doi: 10.1186/1741-7015-11-117. [ DOI ] [ PMC free article ] [ PubMed ] [ Google Scholar ]
- 127. Rurik J.G., Aghajanian H., Epstein J.A. Immune Cells and Immunotherapy for Cardiac Injury and Repair. Circ. Res. 2021;128:1766–1779. doi: 10.1161/CIRCRESAHA.121.318005. [ DOI ] [ PMC free article ] [ PubMed ] [ Google Scholar ]
- 128. Lu Y., Xia N., Cheng X. Regulatory T Cells in Chronic Heart Failure. Front. Immunol. 2021;12:732794. doi: 10.3389/fimmu.2021.732794. [ DOI ] [ PMC free article ] [ PubMed ] [ Google Scholar ]
- 129. Kumar V., Rosenzweig R., Asalla S., Nehra S., Prabhu S.D., Bansal S.S. TNFR1 Contributes to Activation-Induced Cell Death of Pathological CD4+ T Lymphocytes During Ischemic Heart Failure. JACC Basic Transl. Sci. 2022;7:1038–1049. doi: 10.1016/j.jacbts.2022.05.005. [ DOI ] [ PMC free article ] [ PubMed ] [ Google Scholar ]
- 130. Zhong Z., Zhang H., Xu T., Hao J., Chen X., Sun S., Yang J., Sun J., Lin H., Guo H. Identification and Verification of Immune-Related Biomarkers and Immune Infiltration in Diabetic Heart Failure. Front. Cardiovasc. Med. 2022;9:931066. doi: 10.3389/fcvm.2022.931066. [ DOI ] [ PMC free article ] [ PubMed ] [ Google Scholar ]
- 131. Kumar V., Prabhu S.D., Bansal S.S. CD4+ T-Lymphocytes Exhibit Biphasic Kinetics Post-Myocardial Infarction. Front. Cardiovasc. Med. 2022;9:992653. doi: 10.3389/fcvm.2022.992653. [ DOI ] [ PMC free article ] [ PubMed ] [ Google Scholar ]
- 132. Hayward S.L., Bautista-Lopez N., Suzuki K., Atrazhev A., Dickie P., Elliott J.F. CD4 T Cells Play Major Effector Role and CD8 T Cells Initiating Role in Spontaneous Autoimmune Myocarditis of HLA-DQ8 Transgenic IAb Knockout Nonobese Diabetic Mice. J. Immunol. 2006;176:7715–7725. doi: 10.4049/jimmunol.176.12.7715. [ DOI ] [ PubMed ] [ Google Scholar ]
- 133. Fuster V. A Second Dilemma in Cardiovascular Medicine. J. Am. Coll Cardiol. 2014;64:1292–1293. doi: 10.1016/j.jacc.2014.08.006. [ DOI ] [ PubMed ] [ Google Scholar ]
- 134. National Human Genome Research Institute Inter-Society Coordinating Committee for Practitioner Education in Genomics (ISCC) [(accessed on 10 February 2023)]; Available online: https://www.genome.gov/For-Health-Professionals/Inter-Society-Coordinating-Committee-for-Practitioner-Education-in-Genomics .
- 135. Korf B.R., Berry A.B., Limson M., Marian A.J., Murray M.F., O’Rourke P.P., Passamani E.R., Relling M.v., Tooker J., Tsongalis G.J., et al. Framework for Development of Physician Competencies in Genomic Medicine: Report of the Competencies Working Group of the Inter-Society Coordinating Committee for Physician Education in Genomics. Genet. Med. 2014;16:804–809. doi: 10.1038/gim.2014.35. [ DOI ] [ PubMed ] [ Google Scholar ]
- 136. Dickson D., Pfeifer J. Real-World Data in the Molecular Era-Finding the Reality in the Real World. Clin. Pharmacol. Ther. 2016;99:186–197. doi: 10.1002/cpt.300. [ DOI ] [ PubMed ] [ Google Scholar ]
Associated Data
This section collects any data citations, data availability statements, or supplementary materials included in this article.
- View on publisher site
- PDF (2.5 MB)
- Collections
Similar articles
Cited by other articles, links to ncbi databases.
- Download .nbib .nbib
- Format: AMA APA MLA NLM
Add to Collections
Global Cardiovascular Research: Gaps and Opportunities
- Global Cardiovascular Health (L Sperling and D Gaita, Section Editors)
- Published: 20 November 2023
- Volume 25 , pages 1831–1838, ( 2023 )
Cite this article
- Maha Inam 1 ,
- Zainab Samad 2 ,
- Elizabeth M. Vaughan 3 , 4 ,
- Aysha Almas 5 ,
- Bashir Hanif 6 ,
- Abdul Mannan Minhas 4 ,
- Zeerak Jarrar 7 ,
- Fatima Zohra Habib 1 ,
- Sana Sheikh 7 ,
- Dongshan Zhu 8 &
- Salim S. Virani 1 , 2 , 4 , 9
838 Accesses
4 Citations
1 Altmetric
Explore all metrics
Purpose of Review
Cardiovascular disease (CVD) is the leading cause of morbidity and mortality worldwide. CVDs contribute to a large health and economic burden on a global scale. We aim to describe the current landscape of global cardiovascular research, highlight significant findings, and identify potential opportunities for further studies.
Recent Findings
There has been remarkable research output regarding cardiovascular health in recent decades. Large-scale collaborative studies have made impactful strides in identifying modifiable risk factors and forming evidence-based guidelines to facilitate improved cardiovascular care and outcomes. However, there are significant CVD disparities between high- and low- income countries which require interventions to mitigate these inequalities.
Encouraging collaborative partnerships, strengthening research capacity in low-resource settings, and promoting equity in research are fundamental strategic approaches to help improve global cardiovascular research.
This is a preview of subscription content, log in via an institution to check access.
Access this article
Subscribe and save.
- Get 10 units per month
- Download Article/Chapter or eBook
- 1 Unit = 1 Article or 1 Chapter
- Cancel anytime
Price includes VAT (Russian Federation)
Instant access to the full article PDF.
Rent this article via DeepDyve
Institutional subscriptions
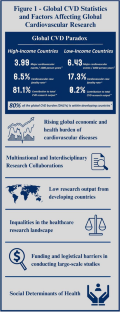
Similar content being viewed by others
Cardiovascular disease research in saudi arabia: a bibliometric analysis.
Priority Areas for CVD Research
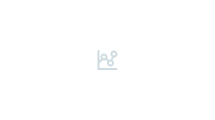
Cardiovascular Risk in South America Compared to Other Countries
Data availability.
No datasets were generated or analyzed during the current study.
Papers of particular interest, published recently, have been highlighted as: • Of importance •• Of major importance
Wang H, Naghavi M, Allen C, Barber RM, Bhutta ZA, Carter A, et al. Global, regional, and national life expectancy, all-cause mortality, and cause-specific mortality for 249 causes of death, 1980–2015: a systematic analysis for the Global Burden of Disease Study 2015. The Lancet. 2016;388(10053):1459–544.
Google Scholar
•• Roth GA, Mensah GA, Johnson CO, Addolorato G, Ammirati E, Baddour LM, et al. Global burden of cardiovascular diseases and risk factors, 1990–2019. J Am Coll Cardiol. 2020;76(25):2982–3021. This study forms the prime resource which outlines the global magnitude of total CVD burden (incidence, prevalence, case fatality, mortality, health risks), identifies underlying causes of CVD death, and links them to relevant risk factors .
PubMed PubMed Central Google Scholar
The top 10 causes of death [Internet]. World Health Organization; 2020 Dec 9 [cited 2023 Sep 1]. Available from: https://www.who.int/news-room/fact-sheets/detail/the-top-10-causes-of-death .
Murray CJL, Lopez AD. Measuring the global burden of disease. N Engl J Med. 2013;369(5):448–57.
CAS PubMed Google Scholar
Birger M, Kaldjian AS, Roth GA, Moran AE, Dieleman JL, Bellows BK. Spending on cardiovascular disease and cardiovascular risk factors in the United States: 1996 to 2016. Circulation. 2021;144(4):271–82.
CAS PubMed PubMed Central Google Scholar
Tsao CW, Aday AW, Almarzooq ZI, Anderson CAM, Arora P, Avery CL, et al. Heart disease and stroke statistics—2023 update: a report from the American Heart Association. Circulation. 2023;147(8):e93-621.
PubMed Google Scholar
Gaziano TA. Reducing the growing burden of cardiovascular disease in the developing world. Health Aff Proj Hope. 2007;26(1):13–24.
Huffman MD, Baldridge A, Bloomfield GS, Colantonio LD, Prabhakaran P, Ajay VS, et al. Global cardiovascular research output, citations, and collaborations: a time-trend, bibliometric analysis (1999–2008). PLoS ONE. 2013;8(12):e83440.
Yusuf S, Hawken S, Ounpuu S, Dans T, Avezum A, Lanas F, et al. Effect of potentially modifiable risk factors associated with myocardial infarction in 52 countries (the INTERHEART study): case-control study. Lancet Lond Engl. 2004;364(9438):937–52.
O’Donnell MJ, Chin SL, Rangarajan S, Xavier D, Liu L, Zhang H, et al. Global and regional effects of potentially modifiable risk factors associated with acute stroke in 32 countries (INTERSTROKE): a case-control study. Lancet Lond Engl. 2016;388(10046):761–75.
Yusuf S, Islam S, Chow CK, Rangarajan S, Dagenais G, Diaz R, et al. Use of secondary prevention drugs for cardiovascular disease in the community in high-income, middle-income, and low-income countries (the PURE Study): a prospective epidemiological survey. Lancet Lond Engl. 2011;378(9798):1231–43.
Joseph P, Kutty VR, Mohan V, Kumar R, Mony P, Vijayakumar K, et al. Cardiovascular disease, mortality, and their associations with modifiable risk factors in a multi-national South Asia cohort: a PURE substudy. Eur Heart J. 2022;43(30):2831–40.
Classifying countries by income [Internet]. The World Bank; 2019 Sep 9 [cited 2023 Sep 24]. Available from: https://datatopics.worldbank.org/world-development-indicators/stories/the-classification-of-countries-by-income.html .
World Bank Country and Lending Groups [Internet]. World Bank Data Help Desk [cited 2023 Sep 24]. Available from: https://datahelpdesk.worldbank.org/knowledgebase/articles/906519-world-bank-country-and-lending-groups .
Liu J, Bu X, Wei L, Wang X, Lai L, Dong C, et al. Global burden of cardiovascular diseases attributable to hypertension in young adults from 1990 to 2019. J Hypertens. 2021;39(12):2488–96.
Sun J, Qiao Y, Zhao M, Magnussen CG, Xi B. Global, regional, and national burden of cardiovascular diseases in youths and young adults aged 15–39 years in 204 countries/territories, 1990–2019: a systematic analysis of Global Burden of Disease Study 2019. BMC Med. 2023;21(1):222.
Wu S, Xu W, Guan C, Lv M, Jiang S, Jinhua Z. Global burden of cardiovascular disease attributable to metabolic risk factors, 1990–2019: an analysis of observational data from a 2019 Global Burden of Disease study. BMJ Open. 2023;13(5):e069397.
Vaduganathan M, Mensah GA, Turco JV, Fuster V, Roth GA. The global burden of cardiovascular diseases and risk: a compass for future health. J Am Coll Cardiol. 2022;80(25):2361–71.
Dong C, Bu X, Liu J, Wei L, Ma A, Wang T. Cardiovascular disease burden attributable to dietary risk factors from 1990 to 2019: a systematic analysis of the Global Burden of Disease study. Nutr Metab Cardiovasc Dis NMCD. 2022;32(4):897–907.
Yusuf S, Joseph P, Rangarajan S, Islam S, Mente A, Hystad P, et al. Modifiable risk factors, cardiovascular disease and mortality in 155,722 individuals from 21 high-, middle-, and low-income countries. Lancet Lond Engl. 2020;395(10226):795–808.
• Yusuf S, Rangarajan S, Teo K, Islam S, Li W, Liu L, et al. Cardiovascular risk and events in 17 low-, middle-, and high-income countries. N Engl J Med. 2014;371(9):818–27. Findings from this study reveal significant metrics to compare CVD risk and events between LICs and HICs, suggesting a substantially highest risk of major CVD and death in LICs. The results highlight the disparity between these regions and identify a lack of implementation of evidence-based practice in LICs .
Lloyd-Jones DM, Allen NB, Anderson CAM, Black T, Brewer LC, Foraker RE, et al. Life’s Essential 8: updating and enhancing the American Heart Association’s construct of cardiovascular health: a presidential advisory from the American Heart Association. Circulation. 2022;146(5):e18-43.
Arnett DK, Blumenthal RS, Albert MA, Buroker AB, Goldberger ZD, Hahn EJ, et al. 2019 ACC/AHA guideline on the primary prevention of cardiovascular disease: a report of the American College of Cardiology/American Heart Association Task Force on Clinical Practice Guidelines. Circulation. 2019;140(11):e596-646.
Visseren FLJ, Mach F, Smulders YM, Carballo D, Koskinas KC, Bäck M, et al. 2021 ESC Guidelines on cardiovascular disease prevention in clinical practice. Eur Heart J. 2021;42(34):3227–337.
Teo KK, Rafiq T. Cardiovascular risk factors and prevention: a perspective from developing countries. Can J Cardiol. 2021;37(5):733–43.
Franco M, Cooper RS, Bilal U, Fuster V. Challenges and opportunities for cardiovascular disease prevention. Am J Med. 2011;124(2):95–102.
•• Qureshi NQ, Mufarrih SH, Bloomfield GS, Tariq W, Almas A, Mokdad AH, et al. Disparities in cardiovascular research output and disease outcomes among high-, middle- and low-income countries — an analysis of global cardiovascular publications over the last decade (2008–2017). Glob Heart. 2021;16(1):4. This study outlines global cardiovascular publications over the last decade according to country development indicators. The results reveal a distinct disparity in research output and CVD burden between LICs and HICs and identify an urgent need to strengthen research capacity within LICs to bridge this gap.
Myers L, Mendis S. Cardiovascular disease research output in WHO priority areas between 2002 and 2011. J Epidemiol Glob Health. 2013;4(1):23–8.
Abushouk AI, Sayed A, Ghanem E, Hassanin A, Mentias A, Bendary A, et al. Africa on the global stage: analyzing 30 years of African-led clinical trials in cardiovascular medicine. Circ Cardiovasc Qual Outcomes. 2023;16(9):e009895.
Mendis S, Yach D, Bengoa R, Narvaez D, Zhang X. Research gap in cardiovascular disease in developing countries. Lancet. 2003;361(9376):2246–7.
Drubin DG, Kellogg DR. English as the universal language of science: opportunities and challenges. Mol Biol Cell. 2012;23(8):1399.
Pascual-Leone N, Liu JW, Beschloss A, Chenna SS, Saifi C. The language of all medical publications and spine publications from 1950 to 2020. North Am Spine Soc J. 2022;21(10):100118.
Amano T, González-Varo JP, Sutherland WJ. Languages are still a major barrier to global science. PLOS Biol. 2016;14(12):e2000933.
Ransing R, Vadivel R, Halabi SE, Jatchavala C, Shalbafan M, Noël C, et al. Language as multi-level barrier in health research and the way forward. Indian J Psychol Med. 2023;45(1):65–8.
Pearson TA. Cardiovascular disease in developing countries: myths, realities, and opportunities. Cardiovasc Drugs Ther. 1999;13(2):95–104.
Many Low- or Middle-Income Countries unprepared for the battle against cardiovascular disease [Internet]. University of Birmingham; 2023 Sep 22 [cited 2023 Sep 24]. Available from: https://www.birmingham.ac.uk/news/2023/many-low-or-middle-income-countries-unprepared-for-the-battle-against-cardiovascular-disease .
The 17 Goals - Sustainable Development [Internet]. United Nations [cited 2023 Aug 25]. Available from: https://sdgs.un.org/goals .
Alemayehu C, Mitchell G, Nikles J. Barriers for conducting clinical trials in developing countries — a systematic review. Int J Equity Health. 2018;17(1):37.
Nicholls M. The PURE study implications. Eur Heart J. 2018;39(48):4227–9.
Pena MSB, Bloomfield GS. Cardiovascular disease research and the development agenda in low- and middle-income countries. Glob Heart. 2015;10(1):71–3.
Public consultation on WHO guidance for best practices for clinical trials [Internet]. World Health Organization; 2023 Jul 19 [cited 2023 Sep 23]. Available from: https://www.who.int/news-room/articles-detail/public-consultation-on-who-guidance-for-best-practices-for-clinical-trials .
Social determinants of health [Internet]. World Health Organization [cited 2023 Sep 1]. Available from: https://www.who.int/health-topics/social-determinants-of-health .
Islam MM. Social determinants of health and related inequalities: confusion and implications. Front Public Health. 2019;8(7):11.
Powell-Wiley TM, Baumer Y, Baah FO, Baez AS, Farmer N, Mahlobo CT, et al. Social determinants of cardiovascular disease. Circ Res. 2022;130(5):782–99.
Mannoh I, Hussien M, Commodore-Mensah Y, Michos ED. Impact of social determinants of health on cardiovascular disease prevention. Curr Opin Cardiol. 2021;36(5):572–9.
Angell SY, McConnell MV, Anderson CAM, Bibbins-Domingo K, Boyle DS, Capewell S, et al. The American Heart Association 2030 impact goal: a presidential advisory from the American Heart Association. Circulation. 2020;141(9):e120–38.
Gheorghe A, Griffiths U, Murphy A, Legido-Quigley H, Lamptey P, Perel P. The economic burden of cardiovascular disease and hypertension in low- and middle-income countries: a systematic review. BMC Public Health. 2018;18(1):975.
McClellan M, Brown N, Califf RM, Warner JJ. Call to action: urgent challenges in cardiovascular disease: a presidential advisory from the American Heart Association. Circulation. 2019;139(9):e44-54.
Download references
Zainab Samad reports research grants from the National Institutes of Health D43 (D43TW011625), BMGF (INV-021944 & INV-050389), Certara (POCUSA3543), NIH Fogarty International Center (1D43 TW011625), Patrick McGovern Foundation — subaward through Duke University (383000239), and NIHR Global Health Research Center NIHR UK (NIHR203248). Zeerak Jarrar reports working as an Instructor Research and a NIH Fogarty Fellow under 5D43TW011625-03. Salim S. Virani reports grants from the National Institutes of Health (5R01DK129474-04) and the Department of Veterans Affairs, USA (IIR- 19–069); research endowment from Tahir and Jooma Family; honorarium in his role as Associate Editor for Innovations, acc.org for the American College of Cardiology (ended in June 2021), and honoraria as a Section Editor for Current Atherosclerosis Reports and Current Cardiology Reports , outside the submitted work.
Author information
Authors and affiliations.
Office of the Vice Provost, Research, Aga Khan University, Karachi, Pakistan
Maha Inam, Fatima Zohra Habib & Salim S. Virani
Section of Cardiology, Department of Medicine, Aga Khan University, Stadium Road, PO Box 3500, Karachi, Pakistan
Zainab Samad & Salim S. Virani
Department of Internal Medicine, UTMB, Galveston, TX, USA
Elizabeth M. Vaughan
Section of Cardiovascular Research, Department of Medicine, Baylor College of Medicine, Houston, TX, USA
Elizabeth M. Vaughan, Abdul Mannan Minhas & Salim S. Virani
Section of Internal Medicine, Department of Medicine, Aga Khan University, Karachi, Pakistan
Aysha Almas
Tabba Heart Institute, Karachi, Pakistan
Bashir Hanif
Department of Medicine, Aga Khan University, Karachi, Pakistan
Zeerak Jarrar & Sana Sheikh
Department of Epidemiology, School of Public Health, Cheeloo College of Medicine, Shandong University, Jinan, China
Dongshan Zhu
The Texas Heart Institute, Houston, TX, USA
Salim S. Virani
You can also search for this author in PubMed Google Scholar
Contributions
All authors contributed to the study. The first draft of the manuscript was written by M.I. and SSV. All authors reviewed and commented on all versions of the manuscript. All authors read and approved the final manuscript.
Corresponding author
Correspondence to Salim S. Virani .
Ethics declarations
Conflict of interest.
The other authors declare that they have no conflict of interest.
Human and Animal Rights and Informed Consent
This article does not contain any studies with human or animal subjects performed by any of the authors.
Additional information
Publisher's note.
Springer Nature remains neutral with regard to jurisdictional claims in published maps and institutional affiliations.
Rights and permissions
Springer Nature or its licensor (e.g. a society or other partner) holds exclusive rights to this article under a publishing agreement with the author(s) or other rightsholder(s); author self-archiving of the accepted manuscript version of this article is solely governed by the terms of such publishing agreement and applicable law.
Reprints and permissions
About this article
Inam, M., Samad, Z., Vaughan, E.M. et al. Global Cardiovascular Research: Gaps and Opportunities. Curr Cardiol Rep 25 , 1831–1838 (2023). https://doi.org/10.1007/s11886-023-01996-2
Download citation
Accepted : 08 November 2023
Published : 20 November 2023
Issue Date : December 2023
DOI : https://doi.org/10.1007/s11886-023-01996-2
Share this article
Anyone you share the following link with will be able to read this content:
Sorry, a shareable link is not currently available for this article.
Provided by the Springer Nature SharedIt content-sharing initiative
- Cardiovascular research
- Cardiovascular disease burden
- Global cardiovascular health
- Healthcare disparities
Advertisement
- Find a journal
- Publish with us
- Track your research
- Search Menu
- Sign in through your institution
- Advance Articles
- Editor's Choice
- Supplements
- Spotlight Issues
- Image Gallery
- ESC Journals App
- ESC Content Collections
- Author Guidelines
- Submission Site
- Why publish with CVR?
- Open Access Options
- Read & Publish
- Author Resources
- Self-Archiving Policy
- About Cardiovascular Research
- About the European Society of Cardiology
- ESC Publications
- Journal Career Network
- Editorial Board
- ESC membership
- Advertising and Corporate Services
- Developing Countries Initiative
- Dispatch Dates
- Terms and Conditions
- Journals on Oxford Academic
- Books on Oxford Academic
Browse issues

Cover image

Volume 118, Issue 1, January 2022
Cardiovascular research onlife, spotted by the scientists of tomorrow, sex matters sex matters.
- View article
Clinical Commentaries
Atrial fibrillation and stroke: are we looking in the right direction, scientists on the spot, scientists on the spot: tracing the potential in electrophysiology.

Expert Opinion
Racial/ethnic health disparities in cardiovascular disease during the covid-19 pandemic: revisiting a long-lasting problem, the 2021 esc/eacts guidelines for the management of valvular heart disease: a new template for heart teams and their patients, extracellular role of s100 calcium-binding protein a4 in atherosclerosis, metabolic memory in diabetes: permanent scar, legacy, or ongoing domino effect, prevention or therapy of the diabetic cardiomyopathy by fine o-glcnacylation balance: hopes and concerns, the relationship between tnf-alpha driven inflammation, lipids, and endothelial function in rheumatoid arthritis: a complex puzzle continues, grandfather’s moonlighting: hydralazine’s novel liaison with mitochondria, cyclophilin d and p66shc contribute to kcl-induced ca 2+ increase in pulmonary artery smooth muscle cells: a potentially relevant phenomenon awaiting a definite mechanism, research letter, prolonged endothelial-dysfunction in human arterioles following infection with sars-cov-2, invited reviews, human-induced pluripotent stem cells in cardiovascular research: current approaches in cardiac differentiation, maturation strategies, and scalable production, immuno-metabolic interfaces in cardiac disease and failure, the vasculature: a therapeutic target in heart failure, current progress in clinical, molecular, and genetic aspects of adult fibromuscular dysplasia.

- Supplementary data
TNAP as a therapeutic target for cardiovascular calcification: a discussion of its pleiotropic functions in the body

Updated perspectives on vascular cell specification and pluripotent stem cell-derived vascular organoids for studying vasculopathies

The role of CD36 in cardiovascular disease
Role of the vascular endothelial sodium channel activation in the genesis of pathologically increased cardiovascular stiffness, original articles, atherosclerosis and lipid biology, neutralization of s100a4 induces stabilization of atherosclerotic plaques: role of smooth muscle cells.

Deficiency of inactive rhomboid protein 2 (iRhom2) attenuates diet-induced hyperlipidaemia and early atherogenesis

Cardiac remodelling and heart failure
G protein-coupled receptor kinase 5 (grk5) contributes to impaired cardiac function and immune cell recruitment in post-ischemic heart failure, clinical track articles, atrial nitroso-redox balance and refractoriness following on-pump cardiac surgery: a randomized trial of atorvastatin.

Co-morbidites and cardiovascular disease
Endothelial cell metabolic memory causes cardiovascular dysfunction in diabetes.

Fine-tuning the cardiac O-GlcNAcylation regulatory enzymes governs the functional and structural phenotype of the diabetic heart

Developmental cardiology
Asymmetric hapln1a drives regionalized cardiac ecm expansion and promotes heart morphogenesis in zebrafish development.

Titin-truncating mutations associated with dilated cardiomyopathy alter length-dependent activation and its modulation via phosphorylation

Immunity and inflammation (auto-immune/sepsis/etc.)
Tnfα induces endothelial dysfunction in rheumatoid arthritis via lox-1 and arginase 2: reversal by monoclonal tnfα antibodies.

Myocardial infarction and ischaemia/reperfusion injury
Selectin-targeting glycosaminoglycan-peptide conjugate limits neutrophil-mediated cardiac reperfusion injury.

Hydralazine protects the heart against acute ischaemia/reperfusion injury by inhibiting Drp1-mediated mitochondrial fission
Neurobiology and stroke, stroke risk prediction in patients with atrial fibrillation with and without rheumatic heart disease.

Pulmonary circulation
Genetic deletion of p66shc and/or cyclophilin d results in decreased pulmonary vascular tone.

Renal biology
Microrna-mediated vascular intercellular communication is altered in chronic kidney disease.

Risk in cardiovascular disease
Sex differences in cardiorespiratory fitness are explained by blood volume and oxygen carrying capacity.

Email alerts
- X (formerly Twitter)
- Recommend to Your Librarian
- Journals Career Network
Affiliations
- Online ISSN 1755-3245
- Print ISSN 0008-6363
- Copyright © 2024 European Society of Cardiology
- About Oxford Academic
- Publish journals with us
- University press partners
- What we publish
- New features
- Open access
- Institutional account management
- Rights and permissions
- Get help with access
- Accessibility
- Advertising
- Media enquiries
- Oxford University Press
- Oxford Languages
- University of Oxford
Oxford University Press is a department of the University of Oxford. It furthers the University's objective of excellence in research, scholarship, and education by publishing worldwide
- Copyright © 2024 Oxford University Press
- Cookie settings
- Cookie policy
- Privacy policy
- Legal notice
This Feature Is Available To Subscribers Only
Sign In or Create an Account
This PDF is available to Subscribers Only
For full access to this pdf, sign in to an existing account, or purchase an annual subscription.
To improve cardiovascular health, invest in prevention, say experts

October 18, 2024 – A crisis in primary care is one of the main reasons that the U.S. isn’t making enough headway in improving cardiovascular health, according to experts.
Cardiologists and clinicians interviewed in a pair of October 15 articles in STAT said that the nation’s health system needs to focus more on preventive care. The article noted that, according to the American Heart Association, cardiovascular diseases and their risk factors are likely to increase by 2050, fueled by racial and ethnic disparities and an aging population.
Asaf Bitton , executive director of Ariadne Labs , a health systems innovation center at Brigham and Women’s Hospital and Harvard T.H. Chan School of Public Health, was one of the experts quoted. He said there hasn’t been enough investment in preventive systems. He believes that it’s possible to instill healthier behaviors if health systems prioritize it.
“We know how to deliver better cardiology, outpatient, and primary care,” he said. “And we know how to integrate behavioral health into patient care. It requires investment, focus, and discipline on the part of a health system to deliver what it ostensibly promises.”
Read the STAT articles: 11 experts on why gains in cardiovascular disease are stalling and what we can do about it Heart disease experts in their own words: ‘This is solvable and this is preventable’

IMAGES
VIDEO